Abstract
Free full text

Purification and Characterization of the Coniferyl Aldehyde Dehydrogenase from Pseudomonas sp. Strain HR199 and Molecular Characterization of the Gene
Abstract
The coniferyl aldehyde dehydrogenase (CALDH) of Pseudomonas sp. strain HR199 (DSM7063), which catalyzes the NAD+-dependent oxidation of coniferyl aldehyde to ferulic acid and which is induced during growth with eugenol as the carbon source, was purified and characterized. The native protein exhibited an apparent molecular mass of 86,000 ± 5,000 Da, and the subunit mass was 49.5 ± 2.5 kDa, indicating an α2 structure of the native enzyme. The optimal oxidation of coniferyl aldehyde to ferulic acid was obtained at a pH of 8.8 and a temperature of 26°C. The Km values for coniferyl aldehyde and NAD+ were about 7 to 12 μM and 334 μM, respectively. The enzyme also accepted other aromatic aldehydes as substrates, whereas aliphatic aldehydes were not accepted. The NH2-terminal amino acid sequence of CALDH was determined in order to clone the encoding gene (calB). The corresponding nucleotide sequence was localized on a 9.4-kbp EcoRI fragment (E94), which was subcloned from a Pseudomonas sp. strain HR199 genomic library in the cosmid pVK100. The partial sequencing of this fragment revealed an open reading frame of 1,446 bp encoding a protein with a relative molecular weight of 51,822. The deduced amino acid sequence, which is reported for the first time for a structural gene of a CALDH, exhibited up to 38.5% amino acid identity (60% similarity) to NAD+-dependent aldehyde dehydrogenases from different sources.
Pseudomonas sp. strain HR199 is able to grow with eugenol as the sole carbon and energy source. Eugenol, which is the main component of the essential oil of the clove tree, Syzygium aromaticum, is most probably degraded via coniferyl alcohol, coniferyl aldehyde, ferulic acid, vanillin, and vanillic acid to protocatechuic acid (16, 26, 27), which is further metabolized by ortho cleavage (23). Coniferyl aldehyde is also described as an intermediate in the catabolism of eugenol by a Corynebacterium sp. (35) and a Pseudomonas sp. (36) and in the catabolism of coniferyl alcohol by Rhodococcus erythropolis (6) and a Xanthomonas sp. (13).
We are currently investigating the catabolism of eugenol by Pseudomonas sp. strain HR199 in detail, since there is an interest in producing vanillin by biotransformation of this natural raw material. One important step in this biotransformation is the oxidation of coniferyl aldehyde to ferulic acid (Fig. (Fig.1),1), which is catalyzed by coniferyl aldehyde dehydrogenase (CALDH). This enzyme has not been purified before, and there are no detailed molecular data available for such a dehydrogenase. In the present study, we describe the purification of the NAD+-dependent CALDH of Pseudomonas sp. strain HR199 and the molecular characterization of the corresponding structural gene, calB.
MATERIALS AND METHODS
Bacterial strains, plasmids, and growth conditions.
Cells of Pseudomonas sp. strain HR199 (DSM 7063) (27) were grown at 30°C either in a nutrient broth medium (0.8%, wt/vol; Bacto, Difco) or in mineral salts medium (MM or HR-MM [27, 30]) supplemented with carbon sources as indicated in the text. Coniferyl alcohol, coniferyl aldehyde, and ferulic acid were dissolved in dimethyl sulfoxide and were added to the medium at a final concentration of 0.1% (wt/vol). Eugenol was directly added to the medium at a final concentration of 0.1% (vol/vol). For mass cultivation, cells were grown aerobically in 20-liter fermentors (type F0020; CHEMAP AG, Männedorf, Switzerland) containing 15 liters of HR-MM supplemented with 0.5% (wt/vol) gluconate and 0.1% (vol/vol) eugenol. Growth was monitored photometrically at 436 nm. Samples were taken and catabolic intermediates were analyzed by high-performance liquid chromatography as described previously (26). If exhausted, eugenol was added to the culture to a final volume of 0.1% (vol/vol). Cells were harvested at a turbidity of about 6 when coniferyl alcohol, coniferyl aldehyde, and ferulic acid were still detectable in the medium.
Cells of Escherichia coli XL1-Blue (3) harboring hybrid plasmids of pBluescript SK(−) (Stratagene, San Diego, Calif.) or of E. coli S17-1 (32) harboring hybrid plasmids of pVK101 (14) were grown at 37°C in Luria-Bertani (LB) medium or in M9 mineral salts medium (29), both containing tetracycline or ampicillin at final concentrations of 12.5 and 100 μg/ml, respectively.
Qualitative and quantitative determination of catabolic intermediates.
Analysis of culture supernatants for excreted intermediates of the eugenol catabolism was performed by liquid chromatography without prior extraction, as described previously (26).
Preparation of the soluble fraction of the crude extract.
The cells were disrupted by a twofold French press passage at 96 MPa. The soluble fraction of the crude extract was obtained by centrifugation at 100,000 × g at 4°C for 1 h.
Enzyme assay.
CALDH was assayed photometrically at 400 nm at 26°C in 100 mM Tris-HCl buffer, pH 8.8, in the presence of 2.7 mM NAD+ and 0.08 mM coniferyl aldehyde by measuring the initial absorbancy changes due to the consumption of coniferyl aldehyde ( = 34 cm2 μmol−1) or at 340 nm by measuring the initial absorbancy changes due to the formation of NADH (
= 6.3 cm2 μmol−1).
In the assay to determine the optimum pH, 100 mM sodium phosphate buffer was used in the pH range from 6 to 8, 25 mM 3-(N-morpholino)propanesulfonic acid buffer was used in the pH range from 6.5 to 7.9, and 100 mM Tris-HCl buffer was used in the pH range from 7 to 10.
For the investigation of the pH stability, the enzyme was incubated in the aforementioned buffers at different pH values at 0°C. Samples were taken after appropriate incubation times, and CALDH activity was determined at 26°C by using the assay with 100 mM Tris-HCl buffer, pH 8.8.
For the determination of the substrate specificity of the purified enzyme, coniferyl aldehyde was replaced by other substrates. The reaction was started by addition of NAD+ (assayed photometrically at 400 nm) or the aromatic substrate (assayed at 340 nm). Stock solutions of coniferyl aldehyde or the other tested substrates were prepared in dimethyl sulfoxide. One unit of enzyme activity was defined as the consumption of 1 μmol of coniferyl aldehyde per min or as the formation of 1 μmol of NADH per min. The amount of soluble protein was determined as described by Lowry et al. (19).
Purification of CALDH.
All steps were carried out at 4°C with 10 mM sodium phosphate buffer (pH 7). A soluble fraction of a crude extract (about 3 g of protein) was derived from about 55 g of wet cells, dialyzed for 12 h against buffer, and then applied to a column (120-ml bed volume [BV]) of DEAE-Sephacel equilibrated with buffer. The column was washed with 2.5 BVs of buffer, and the bound proteins were eluted with a linear NaCl gradient (0 to 500 mM; 600 ml) at a flow rate of 2 ml/min. Fractions (10 ml each) exhibiting high CALDH activity were combined and dialyzed for 4 h against buffer. The enzyme solution was then applied onto a buffer-equilibrated column (80-ml BV) of hydroxylapatite. CALDH did not bind to this matrix and was eluted with 250 ml of buffer at a flow rate of 2 ml/min, whereas other proteins bound to the matrix under these conditions. Fractions (10 ml each) exhibiting high CALDH activity were combined and concentrated by ultrafiltration in a Diaflo chamber equipped with a YM 30 membrane. The enzyme solution was then applied onto a buffer-equilibrated Superdex 200 HiLoad 26/60 column (330-ml BV), at a flow rate of 1 ml/min. CALDH was eluted after a retention volume of 160 ml was reached. Fractions (2 ml each) exhibiting high CALDH activity were combined.
Molecular weight determination by gel filtration.
A Superdex 200HR 10/30 column (24-ml BV; Pharmacia LKB Biotechnologie) was equilibrated with 2.5 BVs of buffer. Purified CALDH and calibration proteins (1.0 mg each) were applied onto the column at a flow rate of 0.5 ml/min. Relative molecular masses were calculated from semilogarithmic plots of the molecular masses of the calibration proteins (bovine serum albumin, yeast alcohol dehydrogenase, sweet potato β-amylase, and horse spleen apoferritin [Sigma, Deisenhofen, Germany]) against the elution volume.
Electrophoretic methods.
Proteins were separated under denaturing conditions in 11.5% (wt/vol) polyacrylamide gels by the method of Laemmli (17) and stained with Serva Blue R.
N-terminal sequence analysis.
N-terminal sequence analysis was performed as described in detail previously (24).
Transformation of DNA.
Competent cells of E. coli were prepared and the DNA was transformed by using the CaCl2 procedure as described by Hanahan (8).
DNA sequence determination and analysis.
The nucleotide sequences were determined nonradioactively with a model 4000L DNA sequencer (LI-COR Inc., Biotechnology Division, Lincoln, Nebr.) and a Thermo Sequenase fluorescence-labelled primer cycle sequencing kit with 7-deaza-dGTP (Amersham Life Science, Amersham International plc, Little Chalfont, Buckinghamshire, United Kingdom) according to the instructions of the manufacturers. Fragment E94 was isolated and digested with Sau3a and HaeIII. The resulting subfragments were cloned in pBluescript SK(−), and the corresponding hybrid plasmids were used as template DNA together with the universal and reverse sequencing primers. Additional sequences were obtained with synthetic fluorescence-labelled oligonucleotides as primers, by the primer-hopping strategy (34). Nucleotide and amino acid sequences were analyzed with the Genetic Computer Group sequence analysis software package (version 6.2; June 1990) by the method of Devereux et al. (5).
Nucleotide sequence accession number.
The nucleotide sequence and amino acid sequence data reported in this paper have been submitted to the EMBL, GenBank, and DDBJ nucleotide sequence databases under accession no. AJ006231.
RESULTS
Purification of CALDH.
The CALDH from eugenol-grown cells of Pseudomonas sp. strain HR199 was purified 79-fold in a three-step procedure (Table (Table1).1). The soluble fraction of the crude extract exhibited a specific CALDH activity of 0.46 U/mg of protein. Chromatography on DEAE-Sephacel resulted in a 6.5-fold increase of the specific activity, with a 65% recovery of the enzyme activity. The subsequent chromatography on hydroxylapatite resulted in a 69.7-fold increase of the specific activity, with a 34% recovery of the enzyme activity. The final gel filtration chromatography on a Superdex 200 HiLoad 26/60 column resulted in a 79-fold increase of the specific activity, with a 13% recovery of the enzyme activity. Sodium dodecyl sulfate-polyacrylamide gel electrophoresis (SDS-PAGE) of the purified enzyme revealed only a single band corresponding to the subunit of CALDH (Fig. (Fig.2).2).
TABLE 1
Purification of CALDH from Pseudomonas sp. strainHR199
Fraction | Vol (ml) | Protein (mg/ml) | Total protein (mg) | Total activity (U) | Sp act (U/mg of protein) | Purification (fold) | Recovery (%) |
---|---|---|---|---|---|---|---|
Crude extract | 90 | 34.2 | 3,078 | 1,404 | 0.46 | 1 | 100 |
DEAE-Sephacel | 78 | 3.9 | 304 | 917 | 3.01 | 6.5 | 65.3 |
Hydroxylapatite | 5 | 3.0 | 15 | 481 | 32.06 | 69.7 | 34.3 |
Superdex 200 | 10 | 0.5 | 5 | 183 | 36.54 | 79.4 | 13.0 |
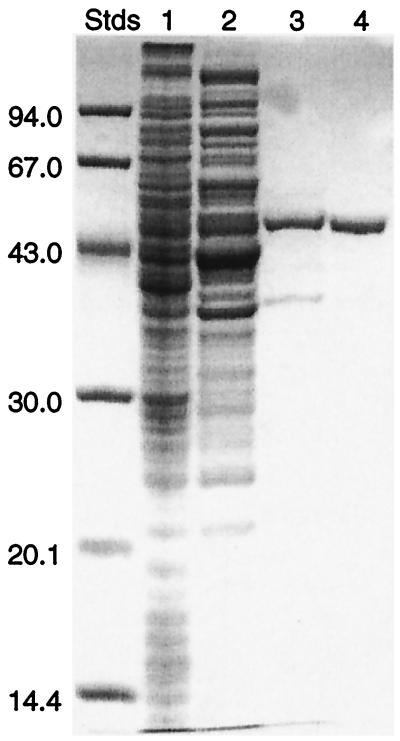
Results of SDS-PAGE of CALDH from Pseudomonas sp. strain HR199 grown in the presence of eugenol. Samples collected at various levels of purification were applied to an 11.5% (wt/vol) SDS-polyacrylamide gel as described in Materials and Methods. Lane 1, 46 μg of protein from the soluble fraction of the crude extract; lane 2, 34 μg of protein after chromatography on DEAE-Sephacel; lane 3, 4 μg of protein after chromatography on hydroxylapatite; lane 4, 2 μg of purified CALDH after chromatography on a Superdex 200 HiLoad column. The molecular masses of standard proteins (Stds) are given on the left in kilodaltons. Protein was stained with Serva Blue R.
Properties of CALDH.
SDS-PAGE of purified CALDH resulted in one band, indicating the presence of only one type of subunit, with an apparent molecular weight (Mr) of 49,500 ± 2,500 (Fig. (Fig.2).2). The nondenatured protein exhibited an apparent Mr of 86,000 ± 5,000 as revealed by gel filtration. These results indicated a homodimeric structure of the native CALDH.
The optimum pH for the NAD+-dependent oxidation of coniferyl aldehyde to ferulic acid catalyzed by CALDH was 8.8 in 100 mM Tris-HCl buffer. However, at this pH the enzyme was quite unstable and lost 87% of its activity within 5 min. Even at a pH of 7.5 the CALDH activity was reduced to 50% within 10 min. At a pH of 6.0 the loss of enzymatic activity was less dramatic, and 50% of the initial activity was left after 4 h.
The optimum temperature for the NAD+-dependent oxidation of coniferyl aldehyde to ferulic acid catalyzed by CALDH was 26°C, and the stability of the enzyme drastically decreased at increasing temperatures. The half-lives of CALDH activity at 31, 34, and 38°C were 5, 2.5, and 1 min, respectively. At temperatures higher than 46°C, the enzyme had a total loss of activity within less than 1 min.
For coniferyl aldehyde and NAD+, Km values of 7 to 12 μM and 0.334 mM, respectively, were calculated from Lineweaver-Burk plots. NADP+ was used as an electron acceptor at 4.3% of the rate of NAD+ reduction. In addition to coniferyl aldehyde, trans-cinnamaldehyde, sinapyl aldehyde, and benzaldehyde were substrates of the enzyme, with maximum rates of about 97, 77, and 18% relative to coniferyl aldehyde, respectively, whereas acrolein and vanillin were not oxidized by the enzyme.
The NH2-terminal amino acid sequence of CALDH was determined by Edman degradation to be 1-Ser-Ile-Leu-Gly-Leu-Asn-Gly-Ala-Pro-Val-Gly-Ala-Glu-Gln-Leu-Gly-Ser-Ala-Leu-19.
Induction of CALDH activity during growth on eugenol.
Pseudomonas sp. strain HR199 is able to utilize eugenol as the sole carbon source for growth. Cells grown on this substrate exhibited a specific CALDH activity of about 0.5 U/mg of protein, whereas no CALDH activity was detectable in cells grown on gluconate. Therefore, the expression of the structural gene of CALDH (calB) seems to be specifically induced during growth on eugenol. This result indicates that coniferyl aldehyde represents an intermediate in the catabolism of eugenol by Pseudomonas sp. strain HR199.
Identification and cloning of the structural gene of CALDH (calB).
During our group’s investigations of the eugenol catabolism by Pseudomonas sp. strain HR199, complementation of a CALDH-deficient mutant was achieved with a hybrid cosmid (pE5-1) from a genomic library in pVK100 (16). This hybrid cosmid harbored five EcoRI fragments with sizes of 1.2, 1.8, 3.0, 5.8, and 9.4 kbp (E12, E18, E30, E58, and E94, respectively). Comparison of the NH2-terminal amino acid sequence obtained from the purified CALDH with amino acid sequences deduced from partial nucleotide sequences of these fragments revealed the localization of the CALDH structural gene (calB) on fragment E94, whereas the structural gene of the coniferyl alcohol dehydrogenase (calA) corresponded to a region covered by fragments E12 and E18 (16).
Nucleotide sequence of calB.
To determine the nucleotide sequence of calB, fragment E94 and HpaI and Sau3a subfragments of E94 were cloned separately in pBluescript SK(−). First, nucleotide sequence data were obtained by using the universal and the reverse sequencing primers together with the aforementioned hybrid plasmids as template DNA. To complete the nucleotide sequences of calB and of adjacent regions, specific synthetic fluorescence-labelled oligonucleotides were applied as primers together with E94 cloned in pBluescript SK(−) as template DNA. The DNA sequence of this 2,000-bp region exhibited a G+C content of 55.1 mol%. An open reading frame of 1,446 bp, which was referred to as calB, was identified. Its putative translational start codon (ATG) at position 375 was preceded by a putative Shine-Dalgarno sequence (AGGAGGT) at a distance of five nucleotides. There was a stem loop structure (CGGGCCCAGGAGC-ATGC-GCTTCTGGGCCCG) 22 bp downstream of the calB gene, which was followed by a T-rich sequence, possibly representing a factor-independent transcriptional terminator. The free energy of this structure is approximately −90 kJ/mol, according to Tinoco et al. (37). The G+C content of calB was 56.6 mol% and the biases of the G+C contents for the different codon positions corresponded well with the theoretical values calculated according to the method of Bibb et al. (1). In addition, the codon usage of calB was very similar to the codon usages of other sequenced genes of Pseudomonas sp. strain HR199 (16, 23, 26). These data indicated that calB represented a coding region.
Properties of the calB gene product.
The relative molecular weight of the protein, calculated from the amino acid sequence deduced from the calB gene, was 51,953. This value corresponded well with the apparent molecular weight of 49,500 ± 2,500 determined by SDS-PAGE for the CALDH purified from Pseudomonas sp. strain HR199. Comparison of the amino acid sequence of CALDH with those in the GenBank database revealed homologies with aldehyde dehydrogenases (ALDHs) from different sources. The alignment of the amino acid sequence of CALDH from Pseudomonas sp. strain HR199 with those of nine representative ALDHs is shown in Fig. Fig.3.3.
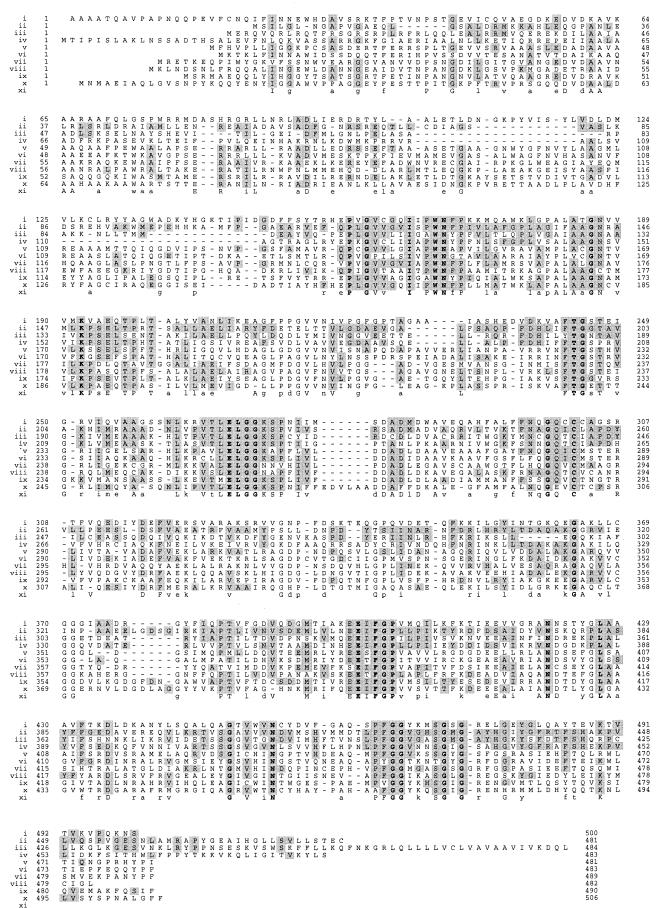
Homology of Pseudomonas sp. strain HR199 CALDH with ALDHs from other sources. The amino acid sequences of human liver mitochondrial ALDH (9) (row i), rat liver microsomal ALDH (21) (row iii), ALDH (alkH gene product) from Pseudomonas oleovorans (15) (row iv), vanillin dehydrogenase (vdh gene product) from Pseudomonas sp. strain HR199 (26) (row v), salicylaldehyde dehydrogenase (doxF gene product) from Pseudomonas sp. strain C18 (4) (row vi), benzaldehyde dehydrogenase (xylC gene product encoded by TOL plasmid pWW0) from P. putida (11) (row vii), succinic semialdehyde dehydrogenase (gabD gene product) from E. coli (22) (row viii), betaine ALDH (betB gene product) from E. coli (2) (row ix), and acetaldehyde dehydrogenase (acoD gene product) from A. eutrophus (25) (row x) were aligned with the CALDH amino acid sequence of Pseudomonas sp. strain HR199 deduced from calB (row ii). Amino acids are specified by standard one-letter abbreviations. Gaps (−) were introduced into the sequences in order to improve the alignment. Amino acid residues which are identical with those of the Pseudomonas sp. strain HR199 CALDH at particular sequence positions are shaded. Amino acids conserved across all 10 sequences are shown in bold. The consensus sequence is given in row xi.
DISCUSSION
Pseudomonas sp. strain HR199 is able to utilize eugenol, which is catabolized via coniferyl alcohol and coniferyl aldehyde as the sole carbon source for growth (27). Coniferyl aldehyde is oxidized to ferulic acid in an NAD+-dependent reaction catalyzed by CALDH. The corresponding gene is expressed only in the presence of coniferyl aldehyde or substrates which are catabolized via this intermediate (e.g., eugenol). No expression is achieved in the presence of unrelated substrates, such as gluconate (16).
This is the first report on the purification of CALDH and the cloning and molecular characterization of the corresponding gene, calB. The CALDH purified from cells of Pseudomonas sp. strain HR199 grown in the presence of eugenol exhibited a specific activity of about 37 U/mg of protein, corresponding to an 80-fold enrichment compared to the soluble fraction of the crude extract. The enzyme showed a high affinity for the substrate coniferyl aldehyde (Km = 7 to 12 μM), combined with a broad substrate specificity towards propenal-substituted aromatic compounds. Nonaromatic aldehydes like propionaldehyde or 2-propenal (acrolein) were not accepted as substrates. Such relatively low Km values for the corresponding substrates are shared by the majority of ALDHs characterized so far, e.g., the 2-hydroxymuconic semialdehyde dehydrogenase from Pseudomonas putida (Km, 17 ± 6 μM [11]), the acetaldehyde dehydrogenase from Saccharomyces cerevisiae (Km, 20 μM [28]), the acetaldehyde dehydrogenase II from Alcaligenes eutrophus (Km, 4 μM [12]), and the human liver mitochondrial ALDH (Km for the propionaldehyde, 0.53 μM [31]). For NAD+, the CALDH exhibited a Km value of 310 μM, which was of the same order of magnitude as those reported for the benzaldehyde dehydrogenases from Acinetobacter calcoaceticus (250 μM [20]) and P. putida (330 ± 13 μM [11]) and acetaldehyde dehydrogenase II from A. eutrophus (200 μM [12]). Moreover, CALDH shared a broad substrate specificity with most of the other ALDHs.
The structural gene calB, encoding CALDH, was localized on an EcoRI fragment harbored by a hybrid cosmid, which was capable of complementing a mutant of Pseudomonas sp. strain HR199 that does not grow with eugenol as the sole carbon and energy source. The amino acid sequence deduced from calB showed significant homology to NAD+-dependent ALDHs from different sources (Fig. (Fig.3).3). Interestingly, CALDH exhibited greater homologies to ALDHs preferring aliphatic aldehydes as substrates than to ALDHs catalyzing the dehydrogenation of benzaldehyde or substituted benzaldehydes. This might reflect that the propenal moiety of coniferyl aldehyde is the target site of CALDH. Nevertheless, the aromatic nature of the substrate is essential for CALDH activity. Recently, the three-dimensional structures of the tetrameric enzyme bovine liver mitochondrial ALDH (33) and a dimeric class 3 ALDH (18) have been determined. These investigations together with detailed site-directed mutagenesis experiments involving exchange of conserved amino acids in human liver mitochondrial ALDH (31) confirmed that Cys-302 (positions are given according to the amino acid sequence of human liver mitochondrial ALDH [9]) is the active-site nucleophile (7) and that Glu-268 is the general locus of a charge relay triad (including a water molecule [33]) for the activation of Cys-302 by ionization (38). These residues were likely conserved in the amino acid sequence of CALDH (Cys-255 and Glu-221). The conservation of other amino acid residues was most probably due to other catalytic and structural requirements, as investigated in detail by Hempel et al. (10) and Sheikh et al. (31).
ACKNOWLEDGMENTS
Amino-terminal sequence analysis of the CALDH protein by Bernhard Schmidt from the Zentrum Biochemie, Abteilung Biochemie II der Georg-August-Universität zu Göttingen, Göttingen, Germany, and skillful technical assistance with DNA sequencing by Daniela Rehder are gratefully acknowledged.
H.P. and A.S. are indebted to Haarmann & Reimer GmbH for providing a collaborative research grant.
REFERENCES
Articles from Journal of Bacteriology are provided here courtesy of American Society for Microbiology (ASM)
Full text links
Read article at publisher's site: https://doi.org/10.1128/jb.180.17.4387-4391.1998
Read article for free, from open access legal sources, via Unpaywall:
https://jb.asm.org/content/jb/180/17/4387.full.pdf
Free after 4 months at jb.asm.org
http://jb.asm.org/cgi/content/full/180/17/4387
Free to read at jb.asm.org
http://jb.asm.org/cgi/content/abstract/180/17/4387
Free after 4 months at jb.asm.org
http://jb.asm.org/cgi/reprint/180/17/4387
Citations & impact
Impact metrics
Citations of article over time
Alternative metrics
Smart citations by scite.ai
Explore citation contexts and check if this article has been
supported or disputed.
https://scite.ai/reports/10.1128/jb.180.17.4387-4391.1998
Article citations
Resolving the metabolism of monolignols and other lignin-related aromatic compounds in Xanthomonas citri.
Nat Commun, 15(1):7994, 12 Sep 2024
Cited by: 0 articles | PMID: 39266555 | PMCID: PMC11393088
Genomic analysis of dibenzofuran-degrading Pseudomonas veronii strain Pvy reveals its biodegradative versatility.
G3 (Bethesda), 11(2):jkaa030, 01 Feb 2021
Cited by: 4 articles | PMID: 33693598 | PMCID: PMC8022969
Functional and genomic characterization of Komagataeibacter uvaceti FXV3, a multiple stress resistant bacterium producing increased levels of cellulose.
Biotechnol Rep (Amst), 30:e00606, 05 Mar 2021
Cited by: 7 articles | PMID: 33747802 | PMCID: PMC7970039
Investigation of microbial community interactions between Lake Washington methanotrophs using -------genome-scale metabolic modeling.
PeerJ, 8:e9464, 30 Jun 2020
Cited by: 4 articles | PMID: 32655999 | PMCID: PMC7333651
Identification of enzymatic genes with the potential to reduce biomass recalcitrance through lignin manipulation in Arabidopsis.
Biotechnol Biofuels, 13:97, 29 May 2020
Cited by: 10 articles | PMID: 32514309 | PMCID: PMC7260809
Go to all (26) article citations
Other citations
Data
Data behind the article
This data has been text mined from the article, or deposited into data resources.
BioStudies: supplemental material and supporting data
Nucleotide Sequences
- (1 citation) ENA - AJ006231
Similar Articles
To arrive at the top five similar articles we use a word-weighted algorithm to compare words from the Title and Abstract of each citation.
Molecular characterization of genes of Pseudomonas sp. strain HR199 involved in bioconversion of vanillin to protocatechuate.
J Bacteriol, 179(8):2595-2607, 01 Apr 1997
Cited by: 103 articles | PMID: 9098058 | PMCID: PMC179009
Identification and molecular characterization of the eugenol hydroxylase genes (ehyA/ehyB) of Pseudomonas sp. strain HR199.
Arch Microbiol, 172(6):354-363, 01 Dec 1999
Cited by: 23 articles | PMID: 10591845
Biotransformation of eugenol to vanillin by a mutant of Pseudomonas sp. strain HR199 constructed by disruption of the vanillin dehydrogenase (vdh) gene.
Appl Microbiol Biotechnol, 52(6):820-828, 01 Nov 1999
Cited by: 61 articles | PMID: 10616715
Biochemical and genetic analyses of ferulic acid catabolism in Pseudomonas sp. Strain HR199.
Appl Environ Microbiol, 65(11):4837-4847, 01 Nov 1999
Cited by: 93 articles | PMID: 10543794 | PMCID: PMC91652