Abstract
Free full text

Methyridine (2-[2-methoxyethyl]-pyridine]) and levamisole activate different ACh receptor subtypes in nematode parasites: a new lead for levamisole-resistance
Abstract
The development of resistance to all chemotherapeutic agents increases and needs to be addressed. We are interested in resistance in parasitic nematodes to the anthelmintic levamisole. During studies on methyridine, we found that it gave us a new insight into pharmacological changes associated with levamisole resistance. Initially, electrophysiological investigation using a two-micropipette current-clamp recording technique revealed that methyridine acts as a cholinergic agonist on nematode muscle receptors (Ascaris suum). Methyridine (>30 μM) produced reversible concentration-dependent depolarizations and increases in input conductance. Mecamylamine (30 μM) and paraherquamide (0.3 μM) produced reversible antagonism of the depolarization and conductance responses to methyridine. These observations suggest that methyridine, like acetylcholine and levamisole, gates ion channels on the muscle of parasitic nematodes.
The antagonistic effects of dihydro-β-erythroidine and paraherquamide on methyridine-induced contractions of A. suum muscle flaps were then examined to determine if methyridine showed subtype selectivity for N-subtype (nicotine-sensitive) or L-subtype (levamisole-sensitive) acetylcholine receptors. Dihydro-β-erythroidine weakly antagonized the effects of methyridine (but had no effect on levamisole responses). The antagonism of methyridine (pA2, 5.9) and nicotine (pA2, 6.1) by paraherquamide was similar, but was less than the antagonism of levamisole (pA2, 7.0). The antagonist profiles suggested that methyridine has a selective action on the N-subtype rather than on the L-subtype.
A novel use for a larval inhibition migration assay was made using L3 larvae of Oesophagostomum dentatum. Inhibitory effects of nicotine, levamisole, pyrantel and methyridine on the migration of larvae of levamisole-sensitive (SENS) and levamisole-resistant (LEV-R) isolates were tested at different concentrations. Levamisole and pyrantel (putative L-subtype-selective agonists) concentration–response plots were displaced to the right in LEV-R isolates. Nicotine (an N-subtype-selective agonist) and methyridine produced little shift in concentration–response plots in the LEV-R isolates. Resistance dose ratios were used to calculate the relative selectivity, ρL, for the L-type receptor (levamisole ρL=1.0; pyrantel ρL=0.93; methyridine ρL=0.17; nicotine ρL=0.06). These observations reveal an N-subtype-selective action of methyridine and suggest that levamisole resistance may be associated with a loss of the L-subtype, but not the N-subtype receptors. The pharmacology of methyridine suggests an approach for the treatment of levamisole-resistant parasites.
Introduction
Many people are aware of the development of resistance to modern antibiotics by bacteria, but resistance to all chemotherapeutic agents is of concern, including drugs directed against parasites. Interestingly, resistance to modern chemotherapeutic agents was first recognized using parasites. Trypan red was used by Paul Erlïch (Ehrlich & Shiga, 1904) to cure mice infected with mal de Caderas (trypanosomiasis) around 1900 at the beginning of modern ‘chemotherapy'. Very soon after the discovery of the action of Trypan red, acquired resistance was observed. In 1905, Franke and Roehl (Browning, 1907) in their experiments with Ehrlïch discovered that mice infected with trypanosomiasis, and treated initially with Trypan red at low doses, became unresponsive to the original curative dose.
We (Robertson et al., 1999; 2000) have been interested in the development of resistance in nematode parasites and have focused our attention on the development of resistance to levamisole, a drug that acts as a selective agonist of nematode acetylcholine-gated ion-channel receptors (AChRs). In Caeno-rhabditis elegans and Ascaris suum, there are at least two pharmacological AChR subtypes associated with body muscle contraction (Richmond & Jorgensen, 1999; Robertson et al., 2002). (1) There is a nicotine-sensitive subtype, which we refer to here as the N-subtype, which is insensitive to levamisole and that is antagonized by dihydro-β-erythroidine. (2) There is a levamisole-sensitive subtype, we refer to here as the L-subtype, which is insensitive to nicotine and dihydro-β-erythroidine, and that is more sensitive to paraherquamide as an antagonist. In muscle contraction studies of A. suum, we separated the L-subtype further and also distinguished a bephenium-sensitive subtype (Robertson et al., 2002). In this paper, we focus on the anthelmintic, methyridine, and show that it has selectivity for the N-subtype of AChR.
Methyridine (Figure 1; 2-(2-methoxyethyl) pyridine) was first described by Broome & Greenhalgh (1961) as an injectable antiparasitic agent effective against roundworm parasites. It is not a potent drug and has an ED50 (subcutaneous) in mice of 108–280 mg/ kg−1 against intestinal parasites. This dose is equivalent to a concentration of 0.8–2.0 mM if we assume an even distribution in the body water. Although a number of studies have characterized the range of parasites that methyridine is effective against, information concerning the mode of action of this compound is incomplete and based on three studies. Broome (1961) first suggested that the mode of action of methyridine was as a neuromuscular blocking agent; and then Eyre (1970), using rat isolated phrenic nerve diaphragm and chick isolated biventer cervicis muscle preparations, observed that methyridine behaved like the depolarizing muscle relaxant, succinylcholine. However, in the most recent study (Raymond et al., 2000), methyridine in concentrations up to 1 mM was reported to have no effect on two AChR subtypes (chicken α-7 and C. elegans ACR-16) expressed in Xenopus oocytes. These observations might be explained if methyridine acts as a cholinergic agonist with AChR subtype selectivity, with effects on particular nematode AChRs and limited effects on vertebrate AChRs.
Methyridine is a pyrimidine, Figure 1. In this paper, we describe the electrophysiological effects of methyridine and examine the subtype selectivity of the drug in nematodes. We report that methyridine is a selective agonist for the nematode N-subtype of AChRs and that its effects are hardly changed in levamisole-resistant parasites. These observations are important because they suggest that levamisole resistance is associated with a reduction in the L-subtype of AChR and that methyridine could be effective in developing treatments for levamisole-resistant parasites.
Methods
Collection of Ascaris
Adult A. suum were collected weekly from the Storm Lake packing plant in Iowa and returned to the laboratory in Locke's solution maintained at 25–35°C in a metal vacuum flask. The Ascaris were used for our electrophysiological studies within 4 days of collection. Contraction responses were less robust and we used preparations within 72 h of collection for our experiments.
Electrophysiological preparation
A ~1 cm long section was taken from the anterior third of the worm and was cut along one lateral line. The body flap of tissue was then pinned out, cuticle side down, onto the Sylgard-lined recording chamber of volume 3 ml. The intestine was removed, exposing the bag regions of the body muscle cells.
The muscle flap preparation was microperfused at a rate of 2–3 ml min−1 with Ascaris Ringer (composition in mM: NaCl (23 mM), Na acetate (110 mM), KCl (24 mM), CaCl2 (6 mM), MgCl2 (5 mM), glucose (11 mM) and 4-(2-hydroxyethyl)-1-piperazineethanesulfonic acid (5 mM), pH 7.6 with NaOH). The temperature of the preparation was maintained at 32°C with a water jacket and a Warner Instruments TC 324B controlled heating system that warmed the temperature of the incoming solution. The microperfusion was accomplished using a 19-gauge needle plastic catheter positioned within 500 μM and over the bag cell being recorded, see Figure 2 inset. Drug solutions were prepared at the concentrations specified in the results and changed by switching the perfusing using a Warner Instruments VC-6 multichannel system.
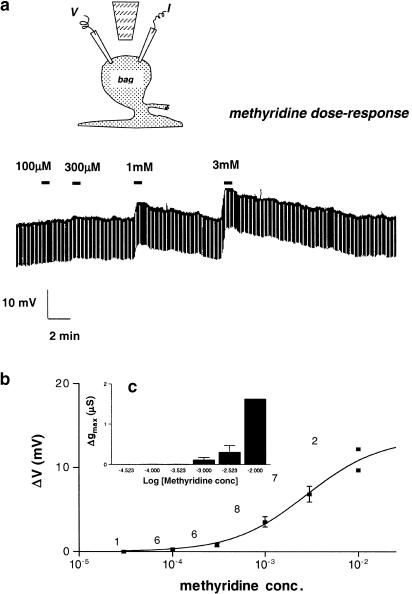
(a) Diagram of the placement of the current-injection (I) and voltage-recording (V) micropipettes and catheter for microperfusion of the bag region of the somatic muscle cell of Ascaris suum and sample electrophysiological responses to the application of different concentrations of methyridine. (b) Methyridine membrane potential dose–response plot. Except for the 10−2 M concentration, all observations are the means±s.e. The number of observations is shown. At 10−2 M, the two observations are shown. (c) (Inset) Methyridine conductance dose–response plot. All observations are the means±s.e. of 6–10 observations.
A two-micropipette current-clamp technique was used for measuring the membrane potential and input conductance changes of the Ascaris muscle cell bags. Glass micropipettes made from borosilicate glass (Clarke Electromedical, U.K.) with resistances in the range 20–40 MΩ when filled with 2 M potassium acetate were used for recording. Two micropipettes were carefully inserted into one muscle cell bag with minimum damage. An Axoclamp 2B amplifier, 1320A Digidata Interface, Clampex software (8.0) all Axon Instruments and a Pentium IV PC computer were used to display, record and analyze the membrane potential and injected current. One micropipette was used for recording of the membrane potential, and the second was used for injection of current pulses (hyperpolarizing: 40 nA, 500 ms at 0.25 Hz).
Muscle flap for contraction studies
We followed the methods we have used previously (Robertson et al., 2002). Two 1-cm body-flap preparations, one dorsal and one ventral, were made from each Ascaris female from the region anterior to the genital pore. The lateral line was removed from the edge of the flaps. Each flap was monitored isometrically by attaching a force transducer in an experimental bath maintained at 37°C containing 10 ml Ascaris Ringer and bubbled with nitrogen. Eight baths were used simultaneously. After dissection, the preparations were allowed to equilibrate for 15 min under an initial tension of 2.0 g. The antagonist was then added to the preparation 15 min before the application of the first concentration of the agonist. We ran two controls (no antagonist) and three replicate antagonist concentrations during each experiment (eight preparations). The dorsal and ventral flaps were assigned randomly in the experiments to reduce any potential error associated with differences in receptor populations. The agonists were added cumulatively with 2–3 min intervals between applications and the responses were steady changes in tension. The results for individual flaps were rejected if the maximum change in tension, at the highest agonist concentration, did not exceed 0.5 g. The mean maximum tension of the preparations, produced at each concentration of the antagonist, was not significantly different (P>0.05, f-test). The responses for each concentration were expressed as % of the maximum tension produced by each individual flap preparation.
Contraction studies: recording and analysis
Changes in isometric muscle tension responses were monitored using a PowerLab System (AD Instruments) that consists of the PowerLab hardware unit and Chart for Windows software. The system allows for recording, displaying and analysis of experimental data. Sigmoid dose–response curves for each individual flap preparation at each concentration of antagonist were described by the Hill equation

where EC50 is the concentration of agonist (Xa) producing 50% of the maximum response and nH is the Hill coefficient (slope); Rmin is the % response at zero Xa. In preparations where desensitization was evident, the maximum response, Rmax, was taken as 100% and responses at higher concentrations were set at 100% for the analysis. Prism 2.01 (GraphPad Software, San Diego, CA, U.S.A.) was used to estimate the constants EC50 and nH in Equation (1) by nonlinear regression for each preparation. The pEC50 was calculated as the negative logarithm of EC50. Statistical analysis was carried out by analysis of variance and t-tests using the GraphPad Software. To illustrate the agonist dose–response relationship at each concentration of antagonist, responses are plotted using the means±s.e.m.% responses (n=6–10 flap preparations), and the lines of fit obtained for the figure display nonlinear regression without constraining the lines to be parallel. These lines of fit were not used for the estimation of the pA2's. The competitive model of antagonism for the mode of action of paraherquamide as an antagonist of methyridine was not adequate: the slope of the Schild plot was significantly different from 1. So to quantitate and compare the antagonism of methyridine with nicotine, levamisole and bephenium by paraherquamide, we estimated the pA2, using the equation

where pEC50 is the same as before, Xb is the concentration of the antagonist, N is equivalent to the slope of the Schild plot, pA2 is the concentration of the antagonist producing a dose ratio (dr) of 2 and C is a constant (–log C is the difference between [pA2 × N] and the agonist control curve pEC50). N, pA2 and log C were estimated as before by nonlinear regression. The advantage of estimating pA2 with this method is that there is no over-reliance on the control dose–response relationship for estimating dr's for the Schild plot. Also, an error estimate for pA2 can be made from the covariance matrix and is expected to be normally distributed (Lew & Angus, 1995). We used the data from Robertson et al. (2002) for nicotine, levamisole and bephenium for comparison.
Larval migration studies
Levamisole-sensitive (SENS) and levamisole-resistant isolates (LEV-R) of Oesophagostomum dentatum were originally supplied by the Royal Veterinary and Agricultural School, Frederiksberg, Copenhagen and then reproduced at 6–9-month intervals by passage in pigs (Ames, IA, U.S.A.). The L3 larvae isolates were maintained between passages in tap water refrigerated at 11°C (changed every 2–4 months).
Between 1500 and 3000 L3's were exsheathed by a 5–10 min incubation in 1.5% sodium hypochlorite solution. The larvae were then washed three times in migration buffer (composition: 0.85% NaCl, 5 mM Tris-HCl, pH to 7.0 with 1 M NaOH) with the aid of centrifugation (5 min 1000 × g). Approximately 150 larvae were collected with a pipette and placed in each of the drug concentrations to be tested for 2 h at 37°C. After this incubation, the larvae were resuspended in fresh test solutions. A migration apparatus consisting of two tightly fitting plastic tubes (~10 mM length) that secured a 20 μM nylon filter (Small Parts Inc. Miami Lakes, FL, U.S.A.) was placed in each test solution of a 24-well plate. The resuspended larvae were added slowly to the top of each filter and allowed to migrate through the filters and into the wells during a 2 h incubation at 37°C. At the end of the incubation period, the number of larvae remaining within each of the filter tubes and the number of larvae entering into the 24-well plate were counted under a microscope, and the % of the total larvae not migrating was calculated for each of the concentrations. The relationship between the drug concentration of levamisole (or pyrantel) and the %-inhibited larvae was then examined. The sigmoid dose–response curves for the effect of levamisole (and pyrantel) concentration on % inhibition of migration were described by Equation (1).
Drugs
Paraherquamide was obtained from Pharmacia Animal Health, Kalamazoo, MI, U.S.A. Nicotine hemi-sulfate, levamisole hydrochloride, pyrantel citrate, dihydro-β-erythroidine and methyridine were purchased from Sigma Chemical Co, St Louis, MO, U.S.A. Pyrantel tartrate and paraherquamide were dissolved in dimethylsulfoxide (DMSO) and added to the microperfusing solution in the electrophysiological experiments and to the bath in the contraction studies. Concentrations of DMSO did not exceed 0.1% in the bath. Control experiments demonstrated that this concentration of DMSO had no effect on dose–response relationships (data not shown).
Results
In the following electrophysiological experiments, we show that the application of methyridine activates acetylcholine ion channels. The experiments on nine A. suum were analyzed for the preparation of the electrophysiological results presented in this paper. Recordings were made from muscle cells with membrane potentials more negative than –25 mV and with a resting input conductance less than 4.0 μS.
Electrophysiology of methyridine
Figure 2a shows the effect of 16 s applications of concentrations of 0.1, 0.3, 1 and 3 mM methyridine to the bag region of A. suum muscle. The muscle cell had a resting membrane potential of –27 mV and input conductance of 3.4 μS. The application of methyridine produced peak depolarizing responses of 0.4, 1.2, 5.1 and 10.1 mV that were associated with reversible conductance changes of 0.01, 0.01, 0.02 and 0.11 μS, respectively. After the application of the methyridine, the membrane potential and input conductance returned towards the resting level with a time course of a few minutes. Similar observations were made from a total of nine preparations.
The effect of methyridine on the membrane potential and input conductance is qualitatively similar to that produced by the nicotinic anthelmintics, levamisole and pyrantel (Harrow & Gration, 1985). Figure 2b shows the methyridine concentration–depolarization response plot. This dose–response plot was described by Equation (1) to obtain the EC50. ΔVmax was 13.5±3.3 mV; the pEC50 was 2.6±0.2 (or 2.6 mM) and nH was 1.1±0.3. Since the EC50 for the electrophysiological effect of levamisole on A. suum muscle is 20 μM (Harrow & Gration, 1985), it follows that methyridine is ~100 less potent than levamisole. Figure 2c shows the methyridine concentration–conductance change plots obtained from the same preparations. We were not able to obtain estimates of the maximum conductance response because of the very high concentrations of the drugs involved.
Antagonism by mecamylamine and paraherquamide
The effects of the nicotinic antagonists mecamylamine and paraherquamide on the electrophysiological responses of methyridine are shown in Figure 3. Mecamylamine is an antagonist of vertebrate neuronal nicotinic receptors and nematode nicotinic receptors (Colquhoun et al., 1991). Figure 3a shows that the depolarizing response of 3 mM methyridine, 7 mV, was abolished in the presence of 30 μM mecamylamine. After washing for 10 min the response returned to 3 mV. In five preparations, 3 mM methyridine produced a depolarization of 5.9±1.3 mV; there was no detectable effect of methyridine in the presence of 30 μM mecamylamine; and after washing for 20 min the mean±s.e. response was 1.2±0.3 mV. There was a significant difference between the mean control membrane potential response and the response in the presence of 30 μM mecamylamine (P=0.01, t-test).
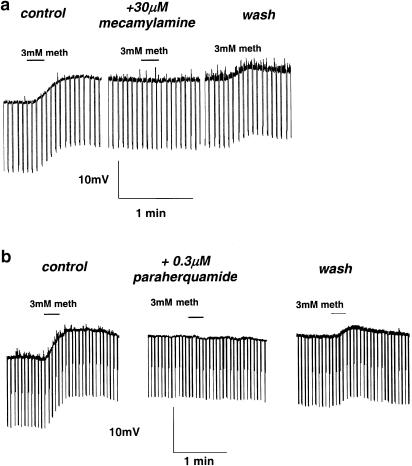
(a) Antagonism by 30 μM mecamylamine of the electrophysiological response to 3 mM methyridine in A. suum. The depolarizing response of 3 mM methyridine, 7 mV, was abolished in the presence of 30 μM mecamylamine. After washing for 10 min, the response returned to 3 mV. (b) Antagonism by 0.3 μM paraherquamide of electrophysiological responses in A. suum to 3 mM methyridine. The effect of 3 mM methyridine is to produce a 6.5 mV depolarization and this is completely abolished in the presence of 0.3 μM paraherquamide. After washing the preparation for 10 min, the depolarizing response returned to 3 mV.
We also tested the effect of paraherquamide, an oxindole antagonist, with selectivity for nematode nicotinic receptors (Robertson et al., 2002). Figure 3b shows a representative experiment. The effect of 3 mM methyridine is to produce a 6.5 mV depolarization and this is completely abolished in the presence of 0.3 μM paraherquamide. After washing the preparation for 10 min, the depolarizing response returned to 3 mV. In six preparations, the control 3 mM methyridine response was 4.7±1.2 mV; in the presence of 0.3 μM paraherquamide, the response was 0.4±0.2 mV; and after washing for 20 min the response returned to 2.2±0.3 mV. There was a significant difference between the mean control membrane potential response and the response in the presence of 0.3 μM paraherquamide (P=0.01, t-test).
The antagonist actions of mecamylamine and paraherquamide on the effects of methyridine are consistent with methyridine acting as an agonist on nematode ionotropic acetylcholine receptors.
Effects of dihydro-β-erythroidine on muscle contraction
We have separated subtypes of AChR in the parasitic nematode, A. suum, using contraction of body muscle strips (Robertson et al, 2002); these include the N- and L-subtypes. The N-subtype is weakly, but selectively antagonized by dihydro-β-erythroidine. Figure 4a shows control contraction methyridine–concentration plots and plots in the presence of 100 μM dihydro-β-erythroidine. There was a small, but statistically significant antagonistic effect of dihydro-β-erythroidine on the response to methyridine (P=0.02, f-test). The control methyridine plot was described using Equation (1), with a pEC50 of 3.41±0.05 (0.39 mM) and nH 1.18±0.14. In the presence of 100 μM dihydo-β-erythroidine, the pEC50 was 3.33±0.12 (or 0.59 mM) and nH 1.31±0.12. There was a shift in the EC50, giving a dr of 1.51. If the antagonism is taken to be competitive, we can make an estimate for the antagonist pKB using

where dr is the dose ratio and Xb is the concentration of dihydro-β-erythroidine. The pKB for methyridine was 3.7. This is similar for the pKB obtained with nicotine (4.1; Robertson et al., 2002), suggesting that methyridine is selective for the N-subtype. The effects of levamisole are not antagonized by dihydro-β-erythroidine (Robertson et al., 2002).
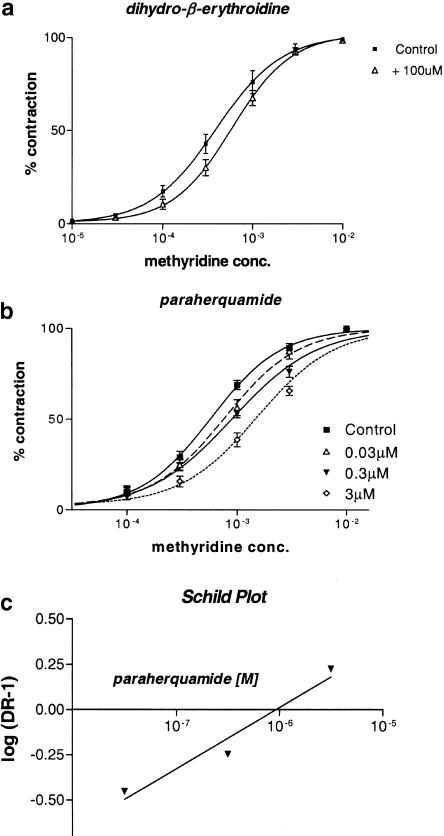
(a) A. suum muscle contraction dose–response plots in the absence (control) and presence of 100 μM dihydro-β-erythroidine. Each observation is the mean±of nine (control) and 10 (100 μM dihydro-β-erythroidine) observations. The line was fitted to Equation (1). Control pEC50 3.41±0.05, nH 1.18±0.14, Rmin 0.2±1.8; dihydro-β-erythroidine pEC50 3.23±0.04, nH 1.30±0.11, Rmin 0.9±1.2. (b) A. suum methyridine dose–response plots in the absence (control) and presence of 0.03, 0.3 and 3 μM paraherquamide. The lines were fitted using Equation (1) to determine the pEC50. Each observation is the mean±s.e. five observations. The observed mean responses to 10–2 M methyridine in the control and in the presence of paraherquamide were similar, close to 100% and superimposed, but the lines of best fit did not. (c) Schild plot of the antagonism shown in (b) The slope of the plot was 0.3, suggesting that the antagonism is not competitive and the intercept was 5.9 on the abscissa giving a value of 5.9 for the pA2. Each observation is the mean±s.e. of five observations.
Effect of paraherquamide
Figure 4b shows the concentration-dependent effect of paraherquamide on the effect of methyridine and Figure 4c shows a conventional Schild plot. The slope of the Schild plot was 0.3, suggesting that the antagonism is not competitive. To compare the antagonism of methyridine with nicotine, levamisole and bephenium, we estimated the pA2's using Equation (2).
The pA2 of methyridine, when estimated using nonlinear regression, was 5.95±0.33, a value close to the intersection on the abscissa of the Schild plot, Figure 4c. N was 0.36±0.12 (significantly less than 1, P<0.001, t-test), confirming deviation from the simple competitive model of antagonism. The pA2 for methyridine is close to the pA2 for nicotine (6.1), but further from the pA2 of levamisole and pyrantel (7.00 and 6.6). The pA2 values for levamisole and pyrantel were calculated using Equation (2) from the data of Robertson et al. (2002) without imposing the simple competitive model. The pA2 of methyridine suggests a selective action of methyridine on N-subtypes, but not on L-subtypes of AChR.
Effect of levamisole, pyrantel, nicotine, bephenium and methyridine in SENS and LEV-R migration assays
Figure 5a shows the concentration effect plots for levamisole in the L3 larval migration assays for SENS and LEV-R isolates. It can be seen that the pEC50 for levamisole was 4.58±0.01 for SENS, which is significantly (P=0.0001) less than the pEC50, 3.94±0.01, for LEV-R. The resistance ratio (EC50/EC50: SENS/LEV-R) is then 4.4. The constants of the concentration–response plot fits are given in Table 1 . We also tested the effect of pyrantel, Figure 5b, which has been shown to be selective for the L-subtype of receptors (Robertson et al., 2002), and found a similar resistance ratio, 4.2, when comparing SENS and LEV-R. Thus, the two isolates show a clear and important change in sensitivity to the L-subtype agonists. Bephenium did not produce inhibition of migration when tested up to a concentration of 1 mM; the lack of effect was interpreted as poor penetration of bephenium through the cuticle of the larvae.

(a) % inhibition larval migration assays by levamisole for SENS and LEV-R O. dentatum larvae. Table 1 gives the estimates for the constants of Equation (1). Note that there is a 4.38-fold shift in the dr's when SENS and LEV-R are compared. The inset shows a photograph of larvae × 50. Each observation is the mean±s.e. of five observations. (b) % inhibition larval migration assays by pyrantel for SENS and LEV-R O. dentatum larvae. Table 1 gives the estimates for the constants of Equation (1). Note that there is a 4.16-fold shift in the dr's when SENS and LEV-R are compared. Each observation is the means±s.e. of five observations. (c) % inhibition larval migration assays by nicotine for SENS and LEV-R O. dentatum larvae. Table 1 gives the estimates for the constants of Equation (1). Note that there is a 1.2-fold shift in the dr's when SENS and LEV-R are compared. The difference between SENS and LEV-R for the pEC50 was not significant. Each observation is the means±s.e. of six observations. (d) % inhibition larval migration assays by methyridine for SENS and LEV-R O. dentatum larvae. Table 1 gives the estimates for the constants of Equation (1). Note that there is a 1.51-fold shift in the dr's when SENS and LEV-R are compared. This difference, although small, did reach statistical significance. Each observation is the mean±s.e. of six observations.
Table 1
Values of the constants of Equation (1), Imax, Imin, pEC50, EC50 and nH used to describe the concentration–response relationships of the SENS and LEV-R L3 larvae of O. dentatum
SENS, n=5 | LEV-R, n=5 | |
---|---|---|
Levamisole | ||
Rmax | 100 | 100 |
Rmin±s.e. | 12.01±0.12 | 6.37±0.01 |
pEC50±s.e. | 4.581±0.002 | 3.943±0.0003 |
EC50 | 26 μM | 114 μM |
Res. ratio=4.38 | ||
P<0.0001*** | ||
nH | 1.364±0.009 | 1.380±0.001 |
Pyrantel | ||
Rmax | 100 | 100 |
Rmin±s.e. | 6.29±3.36 | 8.88±1.89 |
pEC50±s.e. | 4.44±0.06 | 3.82±0.040 |
EC50 | 36 μM | 150 μM |
Res. ratio=4.16 | ||
P=0.0001*** | ||
nH | 1.00±0.0.10 | 1.13±0.11 |
Nicotine | ||
Rmax | 100 | 100 |
Rmin±s.e. | 13.77±5.11 | 16.37±4.99 |
PEC50±s.e. | 2.50±0.11 | 2.44±0.12 |
EC50 | 3.1 mM | 3.6 mM |
Res. ratio=1.2 | ||
NS | ||
nH | 0.89±0.18 | 0.92±0.19 |
Methyridine | ||
Rmax | 100 | 100 |
Rmin±s.e. | 11.20±2.59 | 12.81±2.95 |
pEC50±s.e. | 3.15±0.047 | 2.947±0.057 |
EC50 | 0.7 mM | 1.1 mM |
P=0.008** | ||
Res. ratio=1.57 | ||
nH | 1.04±0.14 | 1.17±0.12 |
Nicotine is a selective agonist on nematode AChRs that activates the N-subtype of nicotinic acetylcholine receptor. Figure 5c shows the concentration–response relationships for SENS and LEV-R for nicotine. The pEC50 for nicotine in SENS was 2.50±0.11 and the pEC50 in LEV-R was 2.44±0.12. This difference was not statistically significant and corresponds to a resistance ratio of 1.2. This ratio contrasts with much bigger SENS : LEVR-R ratios of levamisole and pyrantel. We can explain these observations if there is a reduction in the number of functional L-subtype of receptors associated with resistance without a change in the N-subtype of receptors. The observations also allow comparison of the concentration–response relationships of SENS and LEV-R isolates to distinguish agonists that are selective for L- or N-subtypes.
We then tested the effect of methyridine, Figure 5D, and again compared the effects of methyridine in SENS and LEV-R. Table 1 shows the constants of the parameters that were used to describe the concentration–response relationships. Methyridine is much less potent than levamisole with a pEC50 of 3.15±0.05 in SENS and 2.95±0.06 in LEV-R (P=0.008, Table 1) with a resistance ratio of only 1.6. This ratio is much less than that obtained for levamisole (4.4) and pyrantel (4.16), but close to that obtained for nicotine (1.2), suggesting that methyridine is more selective for the N-subtype than the L-subtype. The relative selectivity for the L-subtype may be quantified by using the equation:

where ρL is the relative selectivity for the L-subtype of receptor with a value of 1 for an agonist that is as selective as levamisole for the L-subtype; and that decreases to 0 for an agonist that has no action on the L-subtype. Λa is the agonist anthelmintic resistance ratio and ΛL is the resistance ratio for levamisole. ρL for levamisole was 1.0; for pyrantel it was 0.93; for methyridine it was 0.17; and for nicotine it was 0.06.
Discussion
AChR subtypes in nematodes
The nicotinic acetylcholine ion channel is composed of five subunits (five α-subunits or a combination of at least two α-subunits combined with other β-subunits) that form a ring around the ion-channel pore. The ligand-binding site includes a contribution from an α-subunit and an adjacent β-subunit (Corringer et al., 1998; Sharples & Wonnacott, 2001). In vertebrate muscle α-, β-, δ-, -subunits form the ion channels with a stoichiometry of α2, β, δ,
at the end plate. In neuronal receptors of mammals, the α- and β-subunits present are: α2–7, α9, α10 and β2–4. The ligand-binding sites (Corringer et al., 1998) of AChRs are composed of six amino-acid loops (A, B and C from the α-subunit and D, E and F from the adjacent β-subunit). Since AChRs have two or more α-subunits, there are two or more ligand-binding sites on each receptor, and these may not be equivalent: the ligand-binding sites formed by the loops, ABC and DEF, will vary with the particular α- and β-subunits that are adjacent on the ion-channel receptor. Consequently, selective agonists and competitive antagonists are expected to distinguish between receptor subtypes and between nonequivalent sites on an individual AChRs.
In nematodes, the potential for different AChR-binding sites is numerically much greater. Mongan et al. (1998) have described the presence of 20 distinct α-subunits and seven β-subunits of the AChR channel in the nematode C. elegans. Five of the α-subunits, including UNC-38 (but not UNC-63), contain a distinct motif (Tyr-x-x-Cys-Cys) in loop C rather than the normally conserved Tyr-x-Cys-Cys (as in the UNC-63) motif. The distinctive Tyr-x-x-Cys-Cys motifs of part of the ligand-binding sites suggests that the pharmacology associated with the UNC-38-containing AChRs will be different from that of the UNC-63 AChRs. The presence of 20 distinct α-subunits is the largest known number of α-subunit genes in a single species and suggests a large potential for pharmacological subtypes of AChR present in C. elegans and in nematode parasites. In support of the presence of multiple subtypes of AChR subtypes in nematode parasites, we have observed the presence of different biophysical subtypes in O. dentatum muscle using single-channel recordings to observe the properties of the receptor channel. In addition, we have shown that there are at least two main pharmacological subtypes in A. suum: the L-subtype is more sensitive to levamisole and the N-subtype is more sensitive to nicotine (Robertson et al., 2002). The presence of two pharmacological subtypes on body wall muscle of C. elegans has also been illustrated by Richmond & Jorgesen (1999) using a combination of voltage-clamp and genetic mutants: UNC-38 (α-subunit) and UNC-29 (β-subunit) mutants resulted in the elimination of muscle responses to levamisole, but not to nicotine. These experiments illustrate that the L-subtype includes UNC-38 and UNC-29 subunits. The N-subtype might include the UNC-63 subunit that has the conventional Tyr-x-Cys-Cys motif in loop C and be selective for nicotine rather than levamisole. It is also possible that other subunits remain to be detected in nematode muscle.
Levamisole resistance
In SENS and LEV-R larval migration assay studies, we have shown in levamisole resistance that there is a change in the EC50 for levamisole, but little change with nicotine as the agonist. This observation could be explained by a reduced number (or sensitivity) of the L-subtype of nAChR without a change in the N-subtype. The observation illustrates that resistance to levamisole may be due to a change in the sensitivity of a single pharmacological subtype of AChR and is not a generalized change in all ACh receptors or muscle contraction mechanisms.
One potential therapeutic approach that is predicted to overcome this type of levamisole resistance would be to use cholinergic agents to treat levamisole resistance that have selective agonist effects on the N-subtypes of AChR. Methyridine has a selective agonist effect on the N-subtype and Figure 5 suggests that methyridine would be useful for the development of treatments for levamisole resistance.
Methyridine mode of action
Methyridine was shown (Broome & Greenhalgh, 1961) to be effective therapeutically against a sample of three species of nematode parasite infection: Nematospiroides dubius; Nippostrongylus mursi and Heterakis spumosa; to be effective against immature (larval stage) nematode infections; and to be effective when given subcutaneously as well as orally. Broome & Greenhalgh (1961) showed that following subcutaneous administration, methyridine appeared in the blood and intestine, particularly the large intestine and suggested that methyridine was excreted along the length of the intestine. The wide distribution of methyridine in the blood following subcutaneous absorption and the therapeutic ratio of ~10 (LD50/ED50), which is not very large, suggests that the relevant nematode AChRs are some 10 times more sensitive than the most sensitive mammalian host AChRs. Vertebrate receptor AChRs that have been described to be affected by high concentrations of methyridine (Eyre, 1970) are in the phrenic nerve diaphragm (1 mM methyridine), in the biventer muscle preparation (1 mM), in the peroneal nerve-digital extensor muscle, and affecting respiration and carotid blood pressure (200 mg kg−1).
Methyridine was also observed to have a rapid paralyzing effect on intact nematode parasites in vitro, demonstrating that methyridine passes through the cuticle to get to the neuromuscular system. The effect of methyridine (10 μg ml−1=73 μM) on A. suum segments (Broome, 1961) was complete paralysis within 2 min. Interestingly, the effects were observed rapidly after applying methyridine to the preparation, and not reversed by the addition of acetylcholine and decamethonium. Thus, the work of Broome & Greenhalgh (1961) and Broome (1961) suggested that methyridine is a selective cholinergic agonist in nematodes. These observations are consistent with the electrophysiological observations made in this paper. We have extended these findings and described the selective effect of methyridine on the N-subtype of nematodes AChRs. Methyridine does not have an agonist effect on all nematode AChRs: no effect of methyridine up to a concentration of 1 mM is observed when the ACR-16 subunit from C. elegans is expressed as a homoligomer in Xenopus oocytes (Raymond et al., 2000); and in this paper we report a selective effect on the N-subtype of AChR.
Conclusion
We have observed electrophysiological effects of methyridine demonstrating an agonist action on ionotropic acetylcholine receptors present on the muscle of A. suum. We suggest that methyridine has selective effects on the N-subtype of nematode receptor and that larval migration studies reveal that the levamisole-resistant isolates are associated with a loss of functional L-subtypes but not N-subtypes. Methyridine may be useful for the development of new approaches for dealing with levamisole resistance.
Abbreviations
ANOVA | analysis of variance |
AChR | acetylcholine-gated ion-channel receptor |
DMSO | dimethylsulfoxide |
dr | the dose ratio |
EC50 | concentration of agonist producing 50% of the maximum response |
HEPES | 4-(2-hydroxyethyl)-1-piperazineethanesulfonic acid |
LEV-R | levamisole-resistant isolate of Oesophagostomum dentatum |
L-subtype | the levamisole-sensitive (preferring) AChR of nematode parasites |
N-subtype | the nicotine-sensitive (preferring) AChR of nematode parasites |
N | value equivalent to the slope of the Schild plot |
nH | the Hill coefficient |
pA2 | the concentration of the antagonist producing a dose ratio of 2 |
pEC50 | the negative logarithm of the concentration of agonist producing 50% of the maximum response |
pKB | the negative logarithm of the dissociation constant of the antagonist |
Rmin | the % response at zero agonist concentration |
Rmax | the maximum tissue response |
s.e. | standard error of the mean |
SENS | levamisole-sensitive isolate of Oesophagostomum dentatum |
Xa | concentration of agonist |
Xb | concentration of antagonist |
ρL | the relative selectivity for the L-subtype of receptor |
Λa | the agonist anthelmintic resistance ratio |
ΛL | the resistance ratio for levamisole |
References
- BROOME A.W.J. Studies on the mode of action of methyridine. Br. J. Pharmacol. 1961;17:327–338. [Europe PMC free article] [Abstract] [Google Scholar]
- BROOME A.W.J., GREENHALGH N. Anthelmintic activity of methyridine against experimental nematode infections in mice. Br. J. Pharmacol. 1961;17:321–326. [Europe PMC free article] [Abstract] [Google Scholar]
- BROWNING C. Experimental chemotherapy in trypanosome infections. Br. Med. J. 1907;2:1405–1409. [Google Scholar]
- COLQUHOUN L., HOLDEN-DYE L., WALKER R.J. The pharmacology of cholinoceptors on the somatic muscle cells of the parasitic nematode Ascaris suum. J. Exp. Biol. 1991;158:509–530. [Abstract] [Google Scholar]
- CORRINGER P.J., BERTRAND S., BOHLER S., EDELSTEIN S.J., CHANGEUX J.P., BERTRAND D. Critical elements determining diversity in agonist binding and desensitization of neuronal nicotinic acetylcholine receptors. J. Neurosci. 1998;18:648–657. [Abstract] [Google Scholar]
- EHRLICH P., SHIGA K. Farbentherapeutische Versuche bei Trypanosomenerkrankung. Berlin klin Wochenschrift xli. 1904;20:329–362. [Google Scholar]
- EYRE P. Some pharmacodynamic effects of the nematodes: methyridine, tetramisole and pyrantel. J. Pharm. Pharmacol. 1970;22:26–36. [Abstract] [Google Scholar]
- HARROW I.D., GRATION K.A.F. Mode of action of the anthelmintics morantel, pyrantel and levamisole on muscle-cell membrane of the nematode Ascaris suum. Pest. Sci. 1985;16:662–672. [Google Scholar]
- LEW M.J., ANGUS J.A. Analysis of competitive agonist–antagonist interactions by nonlinear regression. Trends Pharmacol. Sci. 1995;16:328–337. [Abstract] [Google Scholar]
- MONGAN N.P., BAYLIS H.A., ADCOCK C., SMITH G.R., SANSOM M.S., SATTELLE D.B. An extensive and diverse gene family of nicotinic acetylcholine receptor alpha subunits in Caenorhabditis elegans. Receptors Channels. 1998;6:213–228. [Abstract] [Google Scholar]
- RAYMOND V., MONGAN N.P., SATTELLE D.B. Anthelmintic actions on homomer-forming nicotinic acetylcholine receptor subunits: chicken alpha7 and ACR-16 from the nematode Caenorhabditis elegans. Neuroscience. 2000;101:785–791. [Abstract] [Google Scholar]
- RICHMOND J.E., JORGENSEN E.M. One GABA and two acetylcholine receptor functions at the C. elegans neuromuscular junction. Nat. Neurosci. 1999;19:196–199. [Europe PMC free article] [Abstract] [Google Scholar]
- ROBERTSON A.P., BJORN H.E., MARTIN R.J. Resistance to levamisole resolved at the single-channel level. FASEB J. 1999;13:749–760. [Abstract] [Google Scholar]
- ROBERTSON A.P., BJORN H.E., MARTIN R.J. Pyrantel resistance alters nematode nicotinic acetylcholine receptor single-channel properties. Eur. J. Pharmacol. 2000;394:1–8. [Abstract] [Google Scholar]
- ROBERTSON A.P., CLARK C.L., BURNS T.A., THOMPSON D.P., GEARY T.G., TRAILOVIC S.M., MARTIN R.J. Paraherquamide and 2-deoxy-paraherquamide distinguish cholinergic receptor subtypes in Ascaris muscle. J. Pharmacol. Exp.Ther. 2002;302:853–860. [Abstract] [Google Scholar]
- SHARPLES C.G.V., WONNACOTT S. Neuronal nicotinic receptors. Tocris Rev. 2001;19:1–12. [Google Scholar]
Articles from British Journal of Pharmacology are provided here courtesy of The British Pharmacological Society
Full text links
Read article at publisher's site: https://doi.org/10.1038/sj.bjp.0705528
Read article for free, from open access legal sources, via Unpaywall:
https://europepmc.org/articles/pmc1574116?pdf=render
Citations & impact
Impact metrics
Citations of article over time
Smart citations by scite.ai
Explore citation contexts and check if this article has been
supported or disputed.
https://scite.ai/reports/10.1038/sj.bjp.0705528
Article citations
Calcineurin-Dependent Homeostatic Response of C. elegans Muscle Cells upon Prolonged Activation of Acetylcholine Receptors.
Cells, 12(17):2201, 03 Sep 2023
Cited by: 2 articles | PMID: 37681933 | PMCID: PMC10486475
Effect of a Single and Triple Dose of Levamisole on Hematological Parameters in Controlled Inflammation Model.
Animals (Basel), 12(16):2110, 17 Aug 2022
Cited by: 1 article | PMID: 36009703 | PMCID: PMC9404755
An extracellular scaffolding complex confers unusual rectification upon an ionotropic acetylcholine receptor in C. elegans.
Proc Natl Acad Sci U S A, 119(29):e2113545119, 12 Jul 2022
Cited by: 3 articles | PMID: 35858330 | PMCID: PMC9304021
Effective drug combination for Caenorhabditis elegans nematodes discovered by output-driven feedback system control technique.
Sci Adv, 3(10):eaao1254, 04 Oct 2017
Cited by: 12 articles | PMID: 28983514 | PMCID: PMC5627981
Tribendimidine: mode of action and nAChR subtype selectivity in Ascaris and Oesophagostomum.
PLoS Negl Trop Dis, 9(2):e0003495, 13 Feb 2015
Cited by: 14 articles | PMID: 25679515 | PMCID: PMC4334517
Go to all (23) article citations
Data
Data behind the article
This data has been text mined from the article, or deposited into data resources.
BioStudies: supplemental material and supporting data
Similar Articles
To arrive at the top five similar articles we use a word-weighted algorithm to compare words from the Title and Abstract of each citation.
Oxantel is an N-type (methyridine and nicotine) agonist not an L-type (levamisole and pyrantel) agonist: classification of cholinergic anthelmintics in Ascaris.
Int J Parasitol, 34(9):1083-1090, 01 Aug 2004
Cited by: 33 articles | PMID: 15313135
Paraherquamide and 2-deoxy-paraherquamide distinguish cholinergic receptor subtypes in Ascaris muscle.
J Pharmacol Exp Ther, 302(3):853-860, 01 Sep 2002
Cited by: 50 articles | PMID: 12183640
Pharmacology of N-, L-, and B-subtypes of nematode nAChR resolved at the single-channel level in Ascaris suum.
FASEB J, 20(14):2606-2608, 20 Oct 2006
Cited by: 44 articles | PMID: 17056760
Electrophysiology of Ascaris muscle and anti-nematodal drug action.
Parasitology, 113 Suppl:S137-56, 01 Jan 1996
Cited by: 18 articles | PMID: 9051932
Review
Funding
Funders who supported this work.
NIAID NIH HHS (1)
Grant ID: R01 AI047194
PHS HHS (1)
Grant ID: R01 A14794