Abstract
Free full text
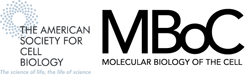
The Mitochondrial Fission Protein hFis1 Requires the Endoplasmic Reticulum Gateway to Induce Apoptosis
Abstract
Mitochondrial fission ensures organelle inheritance during cell division and participates in apoptosis. The fission protein hFis1 triggers caspase-dependent cell death, by causing the release of cytochrome c from mitochondria. Here we show that mitochondrial fission induced by hFis1 is genetically distinct from apoptosis. In cells lacking the multidomain proapoptotic Bcl-2 family members Bax and Bak (DKO), hFis1 caused mitochondrial fragmentation but not organelle dysfunction and apoptosis. Similarly, a mutant in the intermembrane region of hFis1-induced fission but not cell death, further dissociating mitochondrial fragmentation from apoptosis induction. Selective correction of the endoplasmic reticulum (ER) defect of DKO cells restored killing by hFis1, indicating that death by hFis1 relies on the ER gateway of apoptosis. Consistently, hFis1 did not directly activate BAX and BAK, but induced Ca2+-dependent mitochondrial dysfunction. Thus, hFis1 is a bifunctional protein that independently regulates mitochondrial fragmentation and ER-mediated apoptosis.
INTRODUCTION
Mitochondria are crucial organelles in life and death of eukaryotic cells. They participate in numerous metabolic reactions, provide most of the energy required for endoergonic processes, and play a key role in Ca2+ signaling, apoptosis, and aging (Rizzuto et al., 2000; Ferri and Kroemer, 2001; Dufour and Larsson, 2004). This functional versatility is matched by their structural plasticity. Mitochondria are dynamic organelles that continuously undergo fusion and fission (Okamoto and Shaw, 2005). Mounting evidence indicates that the equilibrium between fusion and fission is crucial for several key cellular processes and that mitochondrial shape is finely regulated in response to intracellular needs and extracellular signals (Westermann, 2002; Bossy-Wetzel et al., 2003).
Fusion is required to maintain mtDNA integrity and therefore mitochondrial function (Ono et al., 2001). In higher metazoan, mitochondrial fusion is driven by two GTPases of the outer membrane, Mitofusin (Mfn) 1 and 2 (Santel and Fuller, 2001; Legros et al., 2002; Chen et al., 2003; Santel et al., 2003). Mfns appear to play distinct roles in fusion, as suggested by their different rates of GTP hydrolysis and substantiated by the different morphology of mitochondria in single Mfn knockout fibroblasts (Chen et al., 2003; Ishihara et al., 2004). Consistent with this, Opa1, a GTPase of the inner membrane, requires Mfn1 but not Mfn2 to promote mitochondrial fusion (Cipolat et al., 2004). Disruption of fusion results in cellular dysfunction. Mfn-null and Opa1-RNAi cells display reduced respiration, loss of mitochondrial membrane potential, and inhibition of cell growth (Chen et al., 2005). In addition, proteins involved in mitochondrial fusion can protect cells from apoptosis, as shown by gain and loss of function approaches (Lee et al., 2004; Sugioka et al., 2004).
Mitochondrial fission in mammals involves at least two proteins: dynamin-related protein 1 (Drp1) and Fis1. Drp1 is a cytosolic GTPase, which has been proposed to couple GTP hydrolysis to membrane constriction and fission (Smirnova et al., 2001). Fis1 is an outer membrane protein evenly distributed on the surface of mitochondria (James et al., 2003). Its N-terminal domain is exposed to the cytoplasm and forms a tetratricopeptide (TPR)-like fold (Suzuki et al., 2003). The C-terminus of Fis1 harbors a predicted transmembrane domain and a short stretch of amino acids facing the intermembrane space (IMS). Fis1 is thought to recruit Drp1 to punctuate structures on mitochondria. It is therefore considered the limiting factor in the fission reaction (Stojanovski et al., 2004).
Pro- and anti-apoptotic members of the Bcl-2 family regulate the mitochondrial participation in the apoptotic cascade (Danial and Korsmeyer, 2004). Activation of the mitochondrial pathway results in the release of apoptogenic factors, such as cytochrome c, in the cytosol, where they are required to fully activate effector caspases. This release is accompanied by fragmentation and remodeling of the internal structure of mitochondria (Frank et al., 2001; Scorrano et al., 2002). Both Drp1 and Fis1 have been implicated in these pathways. Their silencing confers resistance to some apoptotic stimuli (Lee et al., 2004). Drp1 participates in cristae remodeling elicited by the BH3-only proapoptotic Bcl-2 family member Bik (Germain et al., 2005). Finally, fragmentation of mitochondria induced by Fis1 is followed by release of cytochrome c, caspase activation and cell death (James et al., 2003). However, it is unclear whether fission always results in increased susceptibility to death. For example, mitochondrial fragmentation can also prevent apoptosis by blocking Ca2+ waves required for amplification of some apoptotic signals (Szabadkai et al., 2004) or by directly cross-talking with members of the Bcl-2 family. In Saccharomyces cerevisiae the Drp1 orthologue dnm1p also seems to favor apoptosis. This prodeath activity of dnm1p is blocked by the antiapoptotic proteins Bcl-2 and Bcl-xL and by the yeast Fis1 orthologue, fis1p (Fannjiang et al., 2004). Thus, at least in yeast, mitochondrial shaping proteins and Bcl-2 family members display some functional redundancy. Moreover, foci corresponding to sites of mitochondrial fission are enriched in Drp1, Mfn2, and the multidomain proapoptotic Bax (Karbowski et al., 2002). Overexpression of Mfn2, as well as silencing of hFis1, interferes with translocation of Bax to mitochondria (Lee et al., 2004; Neuspiel et al., 2005), and the profusion Mfn2 physically interacts with Bcl-XL and the Caenorhabditis elegans orthologue CED-9 (Delivani et al., 2006).
Several central questions remain open. First, it is unclear whether apoptosis and mitochondrial fission are related processes or whether one can occur independently from the other. Second, it is even less clear whether fragmentation occurs before mitochondrial permeabilization (Arnoult et al., 2005). Last, it is unknown how promotion of fission results in caspase-dependent death. We therefore undertook a genetic analysis exploring mitochondrial fission and killing by hFis1 in cells deficient for the multidomain proapoptotics BAX and BAK.
MATERIALS AND METHODS
Molecular Biology
Full-length hFis1 and Δ1-32 hFis1 were subcloned into the XbaI and EcoRI sites of pCI. A myc-tag sequence was inserted by PCR at the 5′ end of the sequence. The K138R mutant of Fis1 was generated by site-specific mutagenesis. All constructs were confirmed by sequencing. YFP-hFis1 was previously described (James et al., 2003).
Reagents, Cell Culture, and Transfection
Mouse embryonic fibroblasts (MEFs) were cultured as described before (Scorrano et al., 2003). Transfection was performed using Transfectin Lipid Reagent (Bio-Rad, Richmond, CA) following the manufacturer's instructions. Cyclosporine H was generously provided by P. Bernardi (University of Padova). All chemicals, unless specified, were from Sigma (St. Louis, MO).
Real-Time Imaging of Mitochondrial Membrane Potential
MEFs, 1 × 105, plated on 24-mm round glass coverslips were transfected and after 24 h were loaded with 20 nM tetramethyl rhodamine methyl ester (TMRM) dissolved in Hanks' balanced salt solution (HBSS) supplemented with 1.5 μM cyclosporine H (a P-glycoprotein inhibitor) or cyclosporine A for 30 min at 37°C. Cells were then placed on the stage of an Olympus IMT-2 inverted microscope (Melville, NY) equipped with a CellR imaging system. After identification of GFP-positive cells, sequential images of TMRM fluorescence were acquired every 60 s using exposure times of 30 ms with a 40×, 1.4 NA Plan Apo objective (Olympus). Cells were excited using a 525/20 BP excitation filter, and emitted light was acquired using a 570/LP filter. Images were stored and analysis of TMRM fluorescence over mitochondrial regions of interest was performed as described in Scorrano et al. (2003).
Confocal Imaging and Morphometric Analysis
MEFs, 4 × 105, plated on 13-mm-diameter glass coverslips (VWR Scientific Products, West Chester, PA) and transfected as indicated in the figure legends were incubated after 24 h in HBSS supplemented with 10 mM HEPES. For confocal images of mitochondrial network, red-channel images were acquired using the detector assembly of a Nikon Eclipse E600FN microscope (Melville, NY) equipped with a Bio-Rad Radiance 2100 Confocal Laser Scanning system. Morphometric analysis was performed as described (Cipolat et al., 2004). Cells were scored with fragmented or coalesced mitochondria when more than 50% of the objects in the image (i.e., mitochondria) displayed aberrant morphology (either fragmented or coalesced). For confocal imaging of mitochondrial movement, mtRFP fluorescence was imaged using a Nikon Eclipse TE300 inverted microscope equipped with a spinning-disk Perkin Elmer-Cetus Ultraview LCI confocal system (Norwalk, CT) and a Orca ER 12-bit CCD camera (Hamamatsu, Bridgewater, NJ).
Polyethylene Glycol Fusion Assay
For polyethylene glycol (PEG) fusion assay, 5 × 105 MEFs were cotransfected with mtYFP or with mtRFP and β-Gal or HA-Fis1. After 24 h cells labeled with different fluorescent proteins were coplated in a 1:1 ratio onto 13-mm round coverslips. Fusion was induced after 24 h by a 60-s treatment with a 50% (wt/vol) solution of PEG1500 in PBS, followed by extensive washes in DMEM supplemented with 10% FCS. To inhibit de novo synthesis of fluorescent proteins, 30 min before PEG treatment cells were incubated with the protein synthesis inhibitor cycloheximide (20 μg/ml), which was subsequently kept in all solutions and tissue culture media until cells were fixed for 30 min with ice-cold 3.7% (vol/vol) formaldehyde in PBS. After two washes with PBS, coverslips were mounted on slides using Anti-Fade Reagent (Molecular Probes, Eugene, OR). Confocal images were acquired and fusion was calculated exactly as described in Cipolat et al. (2004).
Immunofluorescence
MEFs, 6 × 105, plated on 13-mm-diameter glass coverslips (VWR Scientific Products) were transfected as indicated in the figure legends, and after 24 h immunofluorescence was performed as described in Griffiths et al. (1999). Briefly, cells were fixed in 0.25% (wt/vol) paraformaldehyde, incubated overnight with anti Bak (Ab1, Calbiochem, La Jolla, CA), washed, and then incubated with TRITC-conjugated isotype matched antibody. Alternatively, cells were fixed in 4% (wt/vol) paraformaldehyde, incubated overnight with anti-Bax N20 (Santa Cruz Biotechnology, Santa Cruz, CA), and anti-cytochrome c (BD Biosciences, San Jose, CA) washed and then incubated with TRITC- and FITC-conjugated isotype-matched antibodies.
Electron Microscopy
MEFs (5 × 106 cells) transfected with β-Gal or HA-hFis1 were fixed in a 2.5% (vol/vol) solution of glutaraldehyde in PBS for 30 min. Conventional electron microscopy was then performed as described in Scorrano et al. (2002).
Analysis of Cell Death
MEFs, 6 × 104, of the indicated genotype grown in 12-well plates were cotransfected with pEGFP and the indicated vector. At the indicated times, cells were collected and stained with Annexin-V–Alexa568 according to the manufacturer's protocol. Apoptosis was measured by flow cytometry (FACSCalibur, BD Biosciences) as the percentage of annexin-V–positive events in the GFP-positive population.
Subcellular Fractionation
MEFs transfected as indicated were harvested and resuspended in isolation buffer (IB, 0.2 M sucrose, 10 mM Tris-MOPS, pH 7.4, 0.5 mM EGTA-Tris), and mitochondria were isolated by standard differential centrifugation. Light membranes, which included endoplasmic reticulum (ER) and peroxisomes, were obtained by centrifugation of the postmitochondrial supernatant at 100,000 × g for 30 min. Protein concentration was determined by BCA assay (Pierce, Rockford, IL).
Recombinant Protein Production and Purification
Full-length hFIS1 was cloned in pET15b (Novagen, Madison, WI) and the His-tagged hFIS1 (r-HisFIS1) was expressed in Escherichia coli (BL21DE3). After induction with IPTG, bacteria were lysed, and monomeric FIS1 was recovered in the soluble bacterial fraction and purified by chromatography on nickel–nitriloacetic acid–agarose followed by Q-Sepharose resin. The protein was stored in 25 mM Tris/HCl, 100 mM NaCl, 0.2 mM dithiothreitol, and 30% (vol/vol) glycerol, pH 7.5, at −80°C. p7/p15 recombinant BID was produced, purified, and cleaved with caspase 8 as described in Scorrano et al. (2002).
In Vitro Assays and Cross-Linking
Purified mitochondria (0.5 mg/ml) were incubated at 37°C with p7/p15 BID (3.5 ng) or with r-HisFIS1 (125 ng) in experimental buffer (EB, 125 mM KCl, 10 mM Tris-Mops, pH 7.4, 10 μM EGTA-Tris, 1 mM Pi, 5 mM glutamate, and 2.5 mM malate) in the presence of cytosolic extract (0.5 mg/ml). After 30 min, mitochondria were centrifuged at 8000 × g for 5 min. For BAX activation and insertion, mitochondria were resuspended in 0.1 M Na2CO3, pH 11.5, incubated on ice for 30 min, and spun at 100,000 × g for 30 min. Proteins in pellet and supernatant were then separated by SDS-PAGE. BAK oligomerization was assessed as previously described (Scorrano et al., 2002).
Immunoblotting
Protein extracts were separated by SDS-PAGE (NuPAGE, Invitrogen, Carlsbad, CA), transferred onto PVDF membranes (Millipore, Bedford, MA) and probed using the following antibodies: rabbit polyclonal anti-BAK (1:1000 Upstate Biotechnology, Lake Placid, NY), anti-BAX (1:1000, Upstate Biotechnology), anti-hFis1, anti-MnSOD (1:1000, StressGen Biotechnologies, San Diego, CA), anti LDH (1:1000, Fitzgerald Industries International, Chelmsford, MA), anti-Tom20 (1:1000, Santa Cruz), or anti-actin (1:3000, Chemicon International, Temecula, CA). Horseradish peroxidase–conjugated isotype-matched secondary antibodies (Amersham Pharmacia Biotechnology, Piscataway, NJ) were used followed by detection by chemiluminescence (Amersham).
Cell Sorting
Transfected MEFs, 100 × 106, were analyzed by light forward and side scatter and for YFP fluorescence through a 530-nm bandpass filter as they traversed the beam of an argon ion laser (488 nm, 100 mW) of a FACSAria (Becton Dickinson Biosciences, San Jose, CA). Untransfected control MEF cells were used to set the background fluorescence. Sorted cells were checked for viability by Trypan Blue exclusion.
Respiratory Assays
Intact or digitonin (0.01%, wt/vol) permeabilized MEFs (5 × 106 cells) transfected with either β-Gal or HA-hFis1 were incubated in 0.5 ml of HBSS or EB, respectively. Cellular oxygen consumption was measured using a Clarke-type oxygen electrode (Hansatech Instruments, King's Lynn, Norfolk, United Kingdom).
Calcium Retention Capacity
After sorting, YFP-positive MEFs (5 × 106 cells) were incubated in 1 ml of EB supplemented with digitonin (0.01% wt/vol) for 5 min. After permeabilization of the plasma membrane, 2,5-di-(tert-butyl)-1,4 benzohydroquinone (tBuQH, 100 μM) was added before measurement to inhibit sarcoplasmic ER Ca2+ ATPase (SERCA) pumps. The calcium retention capacity (CRC) of mitochondria was assessed using the fluorescent Ca2+ indicator Calcium Green-5N (1 μM, Molecular Probes, Eugene, OR) in a Perkin Elmer-Cetus Life Sciences LS50B spectrofluorimeter (Boston, MA) as described previously (Fontaine et al., 1998).
In Gel ATPase Activity
Mitochondria were isolated as described previously and lysed for 10 min in 1 mM aminocaproic acid, 100 mM Bis-Tris, pH 7.0, 2% (wt/vol) n-dodecylmaltoside at 4°C. After centrifuging for 30 min at 20,000 × g, protein concentration of the solubilized supernatant was measured by Bradford assay, and equal amounts of protein were separated by Blue Native Gel Electrophoresis as described in Schagger, (1995). After running the first dimension, ATPase activity was assessed directly in the gel by incubating it for 2 h in a solution containing 35 mM Tris, 270 mM glycine, 14 mM MgSO4, 0.2% Pb(NO3)2, and 8 mM ATP, pH 7.8 (Nijtmans et al., 2002).
RESULTS
hFis1 Independently Regulates Mitochondrial Fission and Cell Death
To determine which domains of hFis1 regulate fission and death, we generated a set of mutants in crucial regions of the molecule (Figure 1A). We deleted the first α-helix of the TPR motif (hFis1Δ1-32), required for hFis1-induced fragmentation and perinuclear clustering of mitochondria (James et al., 2003; Yu et al., 2005) and introduced a point mutation in the short stretch of amino acids protruding in the IMS (hFis1K148R). After transfection of MEFs, levels of these mutants were comparable, as judged by immunoblotting (Supplementary Figure 1). Expression of hFis1 in MEFs led to fragmented, punctiform organelles, whereas hFis1Δ1-32 promoted coalescence of mitochondria in larger, round structures clustering around the nucleus (Figure 1, B and quantification in C). Mutation of charged amino acids of the IMS stretch of hFis1 to neutral ones resulted in mistargeting of the protein to other intracellular membranes, including ER (unpublished data), consistent with what was observed when the C-terminal of the molecule was tagged (Yoon et al., 2003). However, a conservative substitution of K148 to more positive R did not affect mitochondrial localization of hFis1 (Supplementary Figure 1). This mutant retained the ability to fragment mitochondria, because fission was undistinguishable from that induced by wild-type (wt) hFis1 (Figure 1, B and quantification in C).
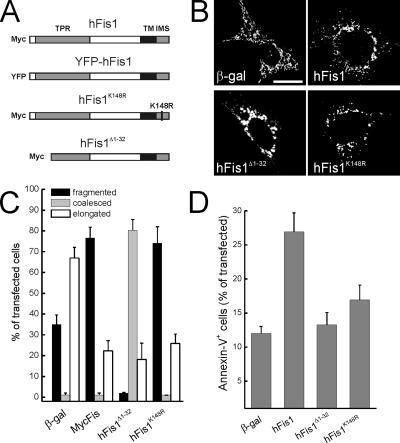
Mutational analysis of hFis1 domains required for mitochondrial fission and apoptosis. (A) Cartoon of constructs used in this study. (B) Representative images of mitochondrial morphology in wt MEFs cotransfected with the indicated plasmid and mtRFP. Forty-eight hours after transfection fluorescence of mtRFP was visualized by confocal microscopy. Bar 15, μm. (C) Morphometric analysis of mitochondrial shape. Experiments were carried exactly as in A. Data represent mean ± SE of five different experiments. (D) Deletion of the first α-helix and K148R point mutation abolish hFis1-induced apoptosis. wt MEFs were cotransfected with GFP and the indicated plasmids, and after 48 h apoptosis was determined as the percentage of GFP-positive, Annexin-V–positive cells by flow cytometry. Data represent mean ± SE of six independent experiments.
The mitochondrial fragmentation in MEFs expressing hFis1 could be in principle caused not only by a stimulation of the fission process, but also by inhibition of fusion. We therefore measured whether hFis1 modified mitochondrial fusion rates using an established PEG fusion assay (Cipolat et al., 2004). Mitochondria expressing hFis1 or hFis1K148R, albeit fragmented, fully retained their ability to fuse (Figure 2, A and quantification in B). Accordingly, mitochondrial movement, which is required to promote encounters among organelles that are going to fuse, was unaltered by expression of hFis1 (Supplementary Figure 2). On the other hand, hFis1Δ1-32 impaired mitochondrial fusion, suggesting a dominant negative effect of this mutant (Figure 2, A and B). Taken together these results suggest that hFis1 causes fragmentation of mitochondria, without impairing fusion of the organelle. The N-terminus of hFis1 is required to mediate mitochondrial fission: positive amino acids of the IMS are crucial for proper targeting and increasing the positivity of this stretch does not interfere with the profission effect of hFis1. On the other hand, deletion of the first α-helix of the TPR motif results in mitochondrial coalescence that is accompanied by an impairment of fusion.
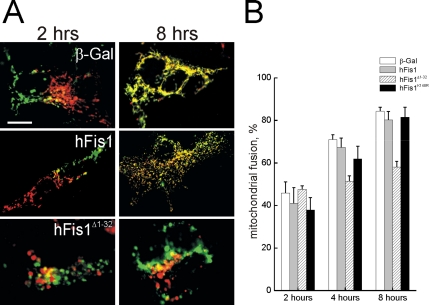
hFis1 does not alter mitochondrial fusion. (A) Representative images of heteropolykaryons after PEG-induced cell fusion. MEFs cotransfected with mtYFP or mtRFP and the indicated plasmids were coplated on glass coverslips, fused, and fixed at the indicated times. Confocal images of representative polykaryons are shown. Bar, 20 μm. (B) Quantification of the effects of wt and mutants of hFis1 on the mitochondrial PEG fusion assay. Experiments were carried out as in A, and cells were fixed at the indicated times. Mitochondrial fusion was evaluated as described in Materials and Methods from 30 randomly selected polykaryons. Data represent mean ± SE of three different experiments.
Because excessive mitochondrial fission is lethal for mammalian cells (James et al., 2003; Yu et al., 2005), we examined whether these hFis1 mutants differentially regulated cell death. Full-length, but not hFis1Δ1-32 promoted apoptosis of MEFs (Figure 1D; Yu et al., 2005). Surprisingly, the hFis1K148R mutant was also unable to induce cell death (Figure 1D). This suggests that the C-terminus of hFis1 not only regulates mitochondrial localization, but is also crucial for induction of apoptosis, as even conservative amino acid substitution abrogates the proapoptotic effect of the molecule. Moreover, these experiments show that fission is not sufficient to induce cell death, at least in the case of hFis1, whose mutants retaining the ability to fragment mitochondria fail to induce apoptosis.
Expression of hFis1 results in the release of apoptogenic factors from the IMS (James et al., 2003), albeit the underlying molecular mechanism is not clear. We therefore turned to a genetic model of Bax, Bak−/− (DKO) MEFs to verify whether these multidomain proapoptotic Bcl-2 family members were required. hFis1 promoted mitochondrial fission also in the absence of Bax and Bak (Figure 3, A and quantification in B). On the other hand, DKO cells remained viable after hFis1 expression (Figure 3C), even after 3 d (unpublished data). These results further dissociate fission and apoptosis induced by hFis1. We investigated interdependence between fission and death using staurosporine (STS), which activates Drp1-dependent fission early in the course of apoptosis (Frank et al., 2001). STS induced mitochondrial fragmentation in both wt and DKO MEFs, but as expected failed to trigger death in DKO cells (Supplementary Figure 3). Silencing of hFis1 interfered only partially with fission-induced by STS (unpublished data), suggesting that mechanisms independent from hFis1 are recruited by this broad range kinase inhibitor to fragment mitochondria. Taken together, these results suggest two separate pathways operate downstream of hFis1: one that mediates mitochondrial fission and requires an intact N-terminus, and a second that triggers cell death, depends on crucial amino acids of the IMS region, and requires BAX and BAK. Moreover, fission induced by intrinsic stimuli occurs independently of multidomain proapoptotics and therefore is genetically positioned upstream of mitochondrial permeabilization.
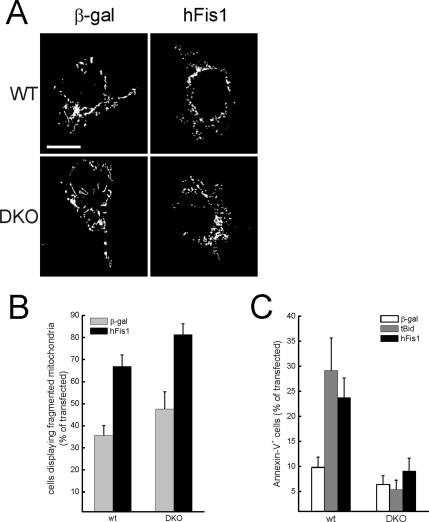
hFis1 triggers mitochondrial fission but not apoptosis in DKO MEFs. (A) Representative images of mitochondrial morphology in wt and DKO cells. wt and DKO MEFs were cotransfected with the indicated plasmid and mtRFP. Experiments were carried out exactly as in Figure 1. (B) Morphometric analysis of mitochondrial shape was carried out exactly as in Figure 1. Data represent mean ± SE of five different experiments. (C) hFis1 does not trigger cell death in DKO cells. MEFs of the indicated genotype were cotransfected with GFP and the indicated plasmids. Apoptosis was determined as in Figure 1. Data represent mean ± SE of six different experiments.
Bax and Bak Are Required for Mitochondrial Dysfunction by hFis1
Apoptosis is characterized by impairment of mitochondrial function, including loss of membrane potential (Δψm). We analyzed the relationship between mitochondrial dysfunction and fragmentation induced by hFis1. Real-time imaging of wt MEFs loaded with the potentiometric dye TMRM showed equivalent accumulation of the dye in mock-transfected cells and in cells expressing wt and hFisK148R (Figure 4A), a result confirmed by a cytofluorimetric analysis of TMRM uptake (unpublished data). Oligomycin, a F1F0 ATPase inhibitor, unveils latent mitochondrial dysfunction, by inducing depolarization of damaged organelles that maintain their membrane potential by reversal of the ATPase (Irwin et al., 2003). As expected, Δψm was maintained in control transfected wt MEFs upon treatment with oligomycin, whereas mitochondria expressing wt hFis1 but not hFisK148R underwent depolarization (Figure 4, A and quantification in B). On the other hand, hFis1 did not cause loss of Δψm in response to oligomycin in DKO cells (Figure 4, C and quantification in D).
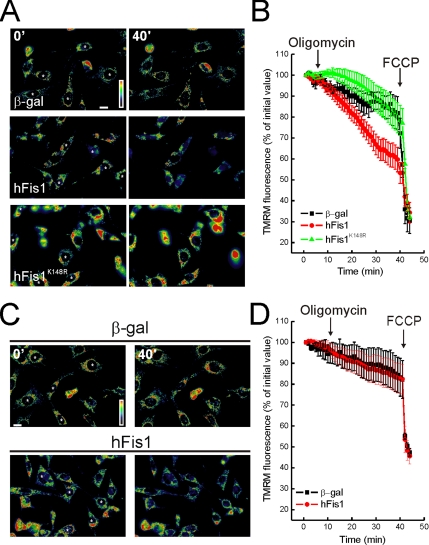
DKO cells are resistant to mitochondrial dysfunction induced by hFis1. (A and C) Pseudocolor-coded, representative images of TMRM fluorescence intensity in wt (A) and DKO (C) cells at 5 (5′) and 40 min (40′) of the acquisition sequence. MEFs cotransfected with GFP and the indicated plasmids (asterisks) after 24 h were loaded with TMRM and imaged as described. Oligomycin (2.5 μg/ml) was added at min 3 of the sequence. (B and D) Quantification of the TMRM fluorescence changes over mitochondrial regions in wt (B) and DKO (D) MEFs. Experiments were carried out as A and C, respectively. Quantification procedure is described in the Materials and Methods. Where indicated (arrows), oligomycin (2.5 μg/ml) and FCCP (4 μM) were added.
To further dissect the effect of hFis1 on mitochondrial function, we turned to measurements of oxygen consumption in intact and digitonin-permeabilized cells. hFis1 did not affect basal or uncoupled respiration of intact wt MEFs (Figure 5A). These results were confirmed in cells permeabilized with digitonin whose mitochondria were energized using substrates for different respiratory chain complexes (Figure 5B). Thus, transient expression of hFis1 does not impair mitochondrial respiration, irrespective of the substrate used to feed the respiratory chain. We next addressed the function of mitochondrial ATPase. A specific in-gel analysis showed that ATPase activity of mitochondria expressing hFis1 was equivalent to that of mock-transfected ones (Figure 5C). Accordingly, cellular ATP content after expression of hFis1 remained unchanged for up to 3 d (3.0 ± 0.5 μM in mock vs. 2.7 ± 0.22 in hFis1-transfected cells at 24 h; 3.7 ± 0.2 vs. 3.9 ± 0.7 at 48 h; 2.8 ± 0.3 vs. 2.9 ± 0.5 at 72 h; n = 3 ± SE). These data indicate 1) that fragmented mitochondria are not necessarily dysfunctional, 2) that apoptosis by hFis1 correlates with induction of organelle dysfunction, and 3) that mitochondrial dysfunction by hFis1 is not caused by an impairment of mitochondrial respiration nor ATPase activity, but requires BAX and BAK.

Mitochondrial respiration, ultrastructure, and ATPase activity in cells expressing hFis1. (A) Oxygen consumption in control and hFis1-expressing intact wt MEFs. Forty-eight hours after transfection, cells were harvested, and 108 cells were incubated in HBSS into an oxygen electrode chamber. Where indicated (arrows), the uncoupler FCCP (2 μM) and the complex IV inhibitor NaN3 (10 mM) were added. (B) Oxygen consumption in control and hFis1-expressing permeabilized MEFs. Experiments were as in A, except that the cells (108) were incubated in 0.5 ml EB containing 0.01% (wt/vol) digitonin. Where indicated (arrows), glutamate plus malate (G/M, 5/2.5 mM) or succinate (Succ, 5 mM in the presence of 2 μM Rotenone, an inhibitor of complex I), ADP (100 μM), oligomycin (2.5 μg/ml), FCCP (60 nM), and NaN3 (1 mM) were added. (C) In-gel activity assay of F1-ATPase. Mitochondria from hFis1-transfected and untransfected cells were isolated and solubilized in n-dodecylmaltoside. Solubilized samples were then separated by BN-PAGE as described in experimental procedures. The Blue Native gel was then histochemically stained for ATP hydrolysis activity. Mitochondria isolated from human hearth samples were loaded as a control. (D) Representative ultrastructure of mitochondria in hFis1-expressing cells. Cells transfected with hFis1 were fixed, and standard electron microsopy images were acquired as described. Bar, 100 nm.
The ER Gateway Regulates Apoptosis by hFis1
We measured whether dysfunction was accompanied by cytochrome c release. Cells expressing hFis1 displayed a markedly diffuse cytochrome c immunostaining pattern, a picture similar to that observed in MEFs expressing BAX (Figure 6A). We therefore verified whether hFis1 triggered activation of BAX and BAK. We first used an in vitro assay using purified organelles and recombinant proteins. Incubation of isolated mitochondria with purified cytosol and recombinant active truncated BID (tBID) resulted in the insertion of BAX in the mitochondrial membranes (Figure 6B) and in oligomerization of BAK (Figure 6C). Recombinant HisFIS1 was conversely unable to induce both BAK oligomerization and BAX insertion (Figure 6, B and C). tBID but not hFis1 induced BAX and BAK activation also in situ, as shown by immunostaining with antibodies that specifically recognize the activated forms of these proteins (Figure 6, C and D).
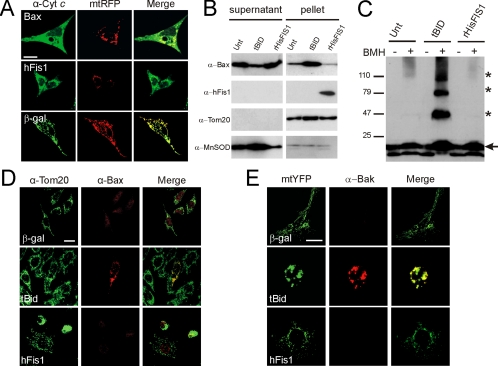
hFis1 does not activate BAX and BAK. (A) hFis1 induces release of cytochrome c. wt MEFs were cotransfected with mtRFP and the indicated plasmid. After 24 h, cells were fixed and immunostained for cytochrome c, and confocal images of mtRFP and cytochrome c were acquired. Images are representative of 80 different cells in three independent experiments. Bar, 15 μm. (B) hFis1 does not trigger BAX insertion into mitochondrial membranes. Purified mitochondria (50 μg) were incubated with p7/p15 BID or with r-HisFis1. Mitochondria were then treated with 0.1 M Na2CO3, and alkali-resistant (pellet) and -sensitive fractions (supernatant) were separated by centrifugation. Proteins were separated by SDS-PAGE and immunoblotted using the indicated antibodies. (C) hFis1 does not induce BAK oligomerization. Purified mitochondria (50 μg) were incubated as in A, and proteins were cross-linked where indicated by incubating with bismaleimidohexane (BMH, 10 mM) for 15 min. Samples were separated by SDS-PAGE and immunoblotted with an anti-BAK antibody. Asterisk, BAK multimers; arrow, BAK monomer. (D) hFis1 does not trigger BAX activation in vivo. MEFs were transfected with indicated plasmids, and 48 h after transfection cells were stained for Tom20 and for activated BAX using a BAX-NT antibody and counterstained with FITC- and TRITC-conjugated isotype-matched secondary antibodies. (E) hFis1 does not trigger BAK activation in vivo. wt MEFs were cotransfected with the indicated plasmid and mtYFP. After 48 h cells were fixed and immunostained with a monoclonal anti-BAK antibody and counterstained with a TRITC-conjugated secondary antibody. Bar, 15 μM.
The lack of direct activation of BAX and/or BAK by hFis1 prompted a more detailed evaluation of its killing mechanism. DKO cells also display lower ER steady state Ca2+ concentration ([Ca2+]ER), a gateway for Ca2+-dependent apoptotic stimuli (Scorrano et al., 2003). Selective correction of the ER and the mitochondrial defect of DKO cells by expression of SERCA or by mitochondrially targeted Bax (mtBAX) enabled us to test which pathway was recruited by hFis1 (Scorrano et al., 2003). As expected, apoptosis by tBID totally depended on the correction of the mitochondrial pathway. On the other hand, hFis1 induced apoptosis only in the DKO-SERCA–corrected MEFs (Figure 7A). In line with this, apoptosis by tBID was not affected when we depleted ER Ca2+ stores by culturing wt MEFs in low Ca2+ (Pinton et al., 2000), and this maneuver fully prevented death by hFis1 (Figure 7B). Finally, mitochondrial dysfunction by hFis1 in DKO cells depended on ER Ca2+ levels, as shown by correction of the ER defect by SERCA (Figure 8, A and quantification in B). Taken together, these experiments show that the apoptotic program elicited by hFis1 is controlled by the ER death gateway requiring adequate [Ca2+]ER.
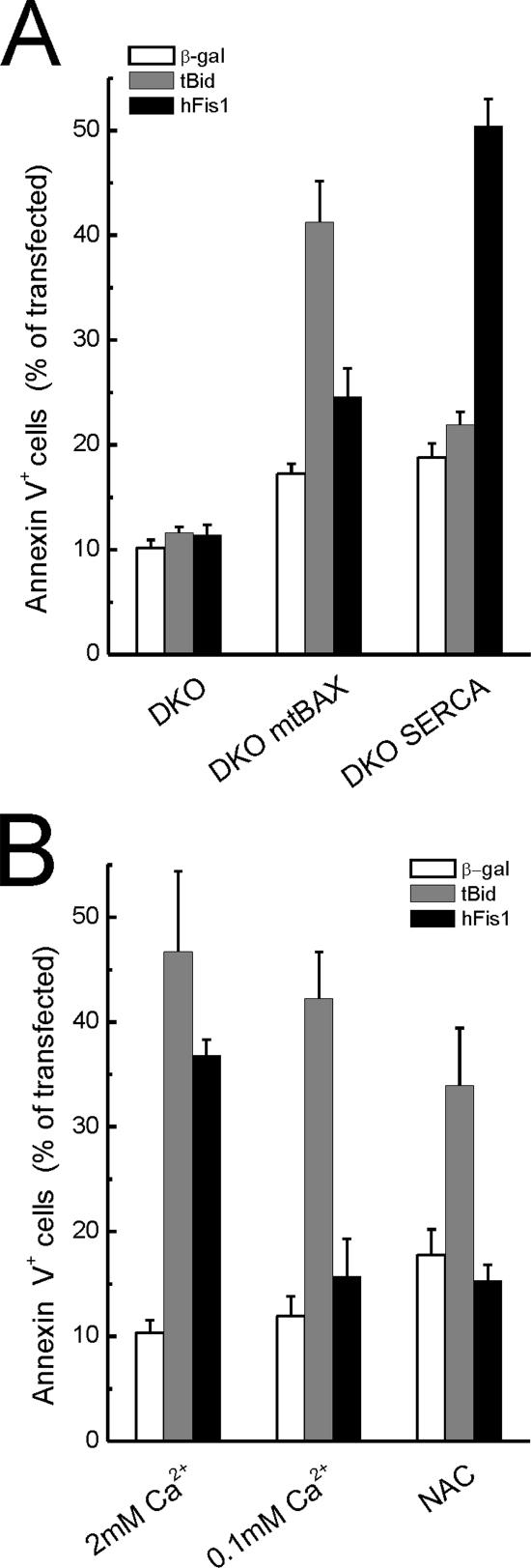
The BAX, BAK ER gateway controls death by hFis1. (A) hFis- induced apoptosis is restored in DKO-SERCA but not in DKO-mtBAX MEFs. MEFs of the indicated genotype were cotransfected with GFP and the indicated plasmids. Apoptosis was determined as the percentage of GFP-positive, Annexin-V–positive cells by flow cytometry. Data represent mean ± SE of six different experiments. (B) hFis1 induced cell death is inhibited by low extracellular [Ca2+] and by NAC. wt MEFs were transfected as in A, and after 4 h NAC (2.5 mM) was added to the media. Apoptosis was monitored 48 h after transfection. Where indicated (0.1 mM Ca2+), wt MEFs were incubated in KRB supplemented with EGTA for 3 h and then maintained in complete DMEM containing 0.1 mM Ca2 before transfection.
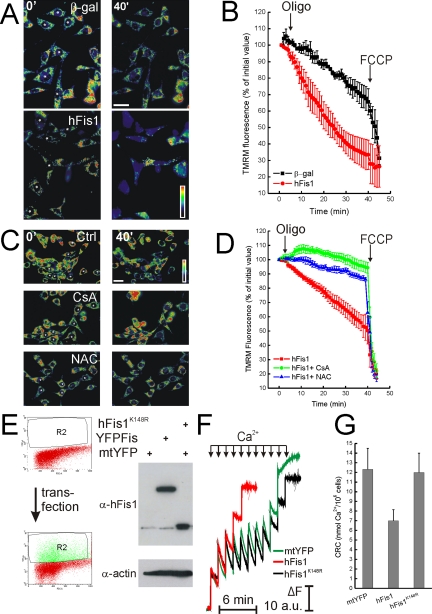
Mitochondrial dysfunction by hFis1 is mediated by permeability transition. (A and B) hFis1 induces mitochondrial dysfunction in DKO-SERCA MEFs. DKO-SERCA MEFs cotransfected with GFP, and the indicated plasmid (asterisks in A) were loaded with TMRM, and fluorescence imaging was performed as described in Figure 3. Data represent mean ± SE of three independent experiments. (C and D) Mitochondrial dysfunction induced by hFis1 is inhibited by CsA and NAC. wt MEFs cotransfected with GFP and Myc-hFis1 (asterisks in C) were loaded with TMRM in the presence of 2.5 mM NAC or 1.5 μM CsA when indicated. TMRM fluorescence over mitochondrial regions was imaged, stored, and analyzed as described in Figure 3. Where indicated (arrows in B and D), oligomycin (2.5 μg/ml) and FCCP (4 μM) were added. Data represent mean ± SE of five different experiments. (E) Cell sorting of YFP-expressing wt MEF. Twenty-four hours after transfection with YFP-hFis1 (YFPFis), mtYFP or mtYFP plus hFis1K148R (FisK148R), wt MEFs (108) were washed, harvested, and sorted as described in the Materials and Methods. Dot plot histograms of YFP fluorescence in control (top panel) and transfected (bottom panel) cells are shown. R2 indicates sorted population. Sorted cells were lysed in RIPA buffer, and equal amounts of protein (40 μg) were separated by SDS-PAGE and immunoblotted with the indicated antibodies. (F) Representative traces of mitochondrial calcium retention capacity (CRC). Sorted (5 × 106) cells were permeabilized with digitonin (0.001% wt/vol) in experimental buffer, and Ca2+ uptake was measured after the fluorescence changes of the Ca2+ indicator Ca-Green. Where indicated (arrows), 5 μM Ca2+ were added. Final volume, 1 ml, pH 7.4, 37°C. (G) Quantitative analysis of CRC of mitochondria from digitoninpermeabilized cells. CRC of mitochondria of sorted wt MEFs transfected with the indicated plasmids was measured exactly as in F. Data represent mean ± SE of five different experiments.
Death by hFis1 Involves Mitochondrial Permeability Transition and Production of Reactive Oxygen Species
Apoptotic stimuli passing trough the ER gateway can impinge on the mitochondrial permeability transition pore (PTP), a Ca2+-dependent inner mitochondrial membrane channel whose dysregulated opening participates in death after selected stimuli (Bernardi, 1999). We wanted to test whether mitochondrial dysfunction induced by hFis1 could be ascribed to dysregulation of PTP. To this end, we tested the effect of cyclosporine A (CsA), an immunosuppressive drug that is also a well-characterized inhibitor of the PTP (Bernardi, 1999). CsA prevented depolarization induced by oligomycin in MEFs expressing hFis1 (Figure 8, C and quantification in D). Prevention of production of reactive oxygen species (ROS) can block opening of the PTP by a variety of inducers, including Ca2+ (Kowaltowski et al., 1996). The glutathione precursor N-acetylcysteine (NAC) prevents PTP opening (Reid et al., 2005) and inhibits apoptosis by several agents (Hockenbery et al., 1993; Zamzami et al., 1995). NAC blocked apoptosis (Figure 7B) and mitochondrial dysfunction (Figure 8, C and D) induced by hFis1 in wt MEFs, further substantiating a role for the PTP in this.
We next decided to measure CRC, an indicator of PTP open probability, in mitochondria expressing different levels of hFis1. After transfection of wt MEFs with a YFP-tagged version of hFis1 (that induced death as efficiently as wt hFis1; unpublished data), cells were sorted. Expression of high levels of hFis1 was confirmed by specific immunoblotting of sorted samples (Figure 8E). Increased hFis1 resulted in markedly reduced mitochondrial CRC in these digitonin-permeabilized, sorted cells (Figure 8, F and quantification in G). Conversely, hFis1K148R did not reduce CRC (Figure 8, F and quantification in D). These results show that hFis1 regulates PTP. Moreover, fission does not necessarily correlate with increased PTP opening probability.
Transient openings of the PTP have been associated with mitochondrial remodeling, which ensures complete release of cytochrome c. We therefore verified whether mitochondria of cells expressing hFis1 displayed an altered ultrastructure. Electron microscopy showed that in ~40% of the mitochondria the expression of hFis1 resulted in a more positive curvature of the cristae and in a widening of the narrow tubular junction (Figure 5).
DISCUSSION
Changes in mitochondrial shape have been recently identified as a common subroutine of apoptosis. Genetic disruption of profusion mitochondria-shaping proteins impairs function of the organelle, whereas excess fission results in cytochrome c release and apoptosis. An emerging view predicts a linear relation between fragmentation of mitochondria and their permeabilization. Whether and how fission necessarily results in permeabilization of mitochondria is however largely unknown. A current model implies that components of the mitochondrial fission machinery can cross-talk with members of the Bcl-2 family to regulate egress of cytochrome c from mitochondria. Multidomain proapoptotic members seem likely candidate to participate in the release of proapoptotic factors after fragmentation of the organelles, but their role in this process remains unclear. Here we genetically dissected the mechanism of death by the profission protein hFis1 and found that fragmentation of mitochondria and apoptosis can occur independently.
hFis1 Does Not Require BAX and BAK To Fragment Mitochondria
Proapoptotic multidomain Bcl-2 family members are multifunctional gatekeepers of organelles that participate in the amplification of apoptotic signals (Scorrano and Korsmeyer, 2003). It has been suggested that they participate in apoptotic fragmentation of mitochondria, based on the colocalization of BAX with sites of fission and on the ability of overexpressed BAX to promote mitochondrial shape changes (Frank et al., 2001; Karbowski et al., 2002). hFis1, a component of the mitochondrial fission machinery, and the intrinsic death stimulus staurosporine, equally fragment mitochondria in wt and Bax, Bak doubly deficient cells. Fragmentation by hFis1 was not associated with a decrease in mitochondrial fusion or with a decreased or disordered movement of the fragmented organelles. Thus, both BAX and BAK are dispensable for fission induced by hFis1 or by a widely used intrinsic apoptotic stimulus. In accordance with this, expression of Bcl-XL blocked death, but not fission induced by hFis1 (James et al., 2003). Moreover, because DKO cells do not undergo mitochondrial permeabilization, our results contribute to place fission temporally in the course of apoptosis. At least in MEFs, fragmentation occurs before the release of mitochondrial IMS proteins, such as DP/TIMM8a, which has been reported to trigger apoptotic translocation of Drp1 to mitochondria (Arnoult et al., 2005). On the other hand, we cannot exclude that mitochondrial fission by staurosporine in DKO cells is the morphological epiphenomenon of complete inhibition of fusion occurring in the course of apoptosis (Karbowski et al., 2004). This possibility was reinforced by silencing of hFis1 expression that only partially inhibited fission induced by staurosporine. In any case, this would imply that during cell death by staurosporine fusion is inhibited independently from BAX and BAK as well as from the activation of the postmitochondrial program of apoptosis.
DKO cells display multiple defects, including a reduction in the steady state ER Ca2+ levels (Scorrano et al., 2003). DKO cells proved therefore informative to address the role of Ca2+ in controlling mitochondrial shape. DKO cells displayed normal steady state morphology and underwent equivalent fission when hFis1 was expressed. This implies that adequate [Ca2+]ER, crucial for several mitochondrial functions, is not required to control morphology of the organelle. Likely, dynamic changes in cytosolic and/or mitochondrial Ca2+ can conversely modulate mitochondrial shape by yet unidentified mechanisms, such as regulation of kinase/phosphatase couples (Yi et al., 2004).
The ER Gateway Controls Apoptosis by hFis1
The resistance of DKO cells to death induced by hFis1 suggested that hFis1 would directly or indirectly activate these multidomain proapoptotics to provide an efflux pathway for cytochrome c. A set of in vitro experiments using purified organelles and recombinant proteins excluded a direct effect of hFis1 on BAX and BAK activation, as well as on cytochrome c release (J.-C. Martinou, unpublished results). This did not depend on additional factors missing in this reconstituted assay, because activation of multidomain proapoptotics was also excluded in situ. These surprising results suggest that in the case of hFis1 induced fission, insertion and oligomerization of BAX at sites of fragmentation is not favored as in other paradigms of apoptosis (Frank et al., 2001).
Selective correction of ER versus mitochondrial defect of DKO cells has been used to define the gateway through which a given apoptotic stimulus passes (Scorrano et al., 2003). hFis1 induced death in SERCA-, but not in mtBAX-corrected DKO MEFs. Further evidences showed that hFis1 required adequate ER Ca2+ to kill, placing it genetically downstream of the ER gateway of apoptosis. Our results provide a molecular explanation for the previous finding that death by hFis1 is blocked by Bcl-XL (James et al., 2003), which also regulates release of Ca2+ from the ER (Li et al., 2002). The small reduction in capacitative Ca2+ entry (CCE) promoted by hFis1 (Frieden et al., 2004) can therefore be viewed as a compensatory mechanism to protect cells with high levels of this protein, because CCE dictates Ca2+ refilling of the ER (Jaconi et al., 1997). In line with this, direct measurement of free [Ca2+]ER using targeted aequorin showed that expression of hFis1 reduced in rate and extent of ER refilling by ~40% (unpublished data). Whether other conditions of excessive fragmentation kill via the ER gateway still needs to be elucidated, but this remains a viable possibility that extends ER-mitochondria cross-talk to a previously unexpected process.
Latent Mitochondrial Dysfunction Precedes Death by hFis1
Mitochondria lacking essential components of mitochondrial fusion display multiple functional defects, including greatly reduced respiratory rates (Chen et al., 2005). This holds true also in yeast, where most deletion mutants of genes involved in mitochondrial fusion are petite, as a consequence of their respiratory incompetence (Dimmer et al., 2002). Moreover, the importance of profusion proteins for cellular physiology is confirmed by their ablation in the mouse, which results in early embryonic lethality (Chen et al., 2003). We therefore reasoned that increasing mitochondrial fission by expressing hFis1 could have similar results. On the other hand, hFis1 did not impair mitochondrial fusion nor did it affect a variety of bioenergetic parameters, including total ATP content, mitochondrial respiration, or membrane potential. This raised the question of the mechanism of killing by hFis1, which apparently did not involve a gross destabilization of mitochondrial function.
A detailed analysis of mitochondrial membrane potential in situ in cells expressing hFis1 showed conversely a latent organelle dysfunction, unveiled only after treatment with oligomycin. This drug is expected to induce slight hyperpolarization, because the inhibition of the F1F0 ATPase blocks diffusion of protons back into the matrix of phosphorylating mitochondria. On the other hand, oligomycin induced depolarization of wt mitochondria expressing hFis1, which most probably maintain their membrane potential by reversal of the ATPase. Such a depolarization was not observed in hFis1-transfected DKO cells and was restored by genetic correction of DKO with SERCA. A more detailed analysis of respiration of intact and digitonin-permeabilized cells ruled out gross impairment of the respiratory chain. Moreover, assembly of the ATPase and total cellular ATP content were not affected by high levels of hFis1. When hFis1 is overexpressed, basic bioenergetics appears therefore to be conserved. On the other hand, hFis1 reduces the mitochondrial threshold to stresses, as highlighted by the oligomycin-induced depolarization. It should be noted that similar experiments have been performed in HeLa cells, where oligomycin had apparently no effect (Frieden et al., 2004). One explanation can be found in the short experimental window used by Demaurex and colleagues that precluded highlighting of the slow depolarization induced by oligomycin (Gugliucci et al., 2002; Irwin et al., 2003). Latent mitochondrial dysfunction unveiled by oligomycin characterizes cells from mouse models of collagen VI deficiency (Irwin et al., 2003), as well as prostate cancer cells where accumulation of arachidonic acid was induced by pharmacological inhibition of its metabolism (Gugliucci et al., 2002). In both these models, dysfunction was prevented by the PTP inhibitor CsA, suggesting a role for this inner mitochondrial membrane channel.
An IMS Mutant of hFis1 Dissociates Fission from PTP Opening
CsA as well as NAC, inhibitors of Ca2+-induced PTP opening, blocked depolarization induced by oligomycin in mitochondria expressing hFis1. Furthermore, CRC of mitochondria expressing high levels of hFis1 was reduced by ~50% compared with control organelles, indicating that hFis1 does not require additional cytosolic components to induce mitochondrial dysfunction. The mechanism by which hFis1 reduces the threshold for PTP opening remains to be investigated. Recently, it has been proposed that mitochondrial fission is required for the generation of ROS in a model of hyperglycemia (Yu et al., 2006), suggesting a cross-talk between the fission machinery and the mechanisms involved in the production of ROS. It is tempting to speculate that high levels of hFis1 can trigger production or favor accumulation of ROS that in turn lower the Ca2+ threshold of the PTP (Chernyak and Bernardi, 1996).
Mitochondrial dysfunction and death induced by hFis1 was genetically distinct from mitochondrial fission. A conservative single amino acid mutant in the short IMS stretch of hFis1 completely abolished mitochondrial dysfunction in situ and restored CRC to levels found in control mitochondria. These results have important implications. First, they point to a crucial role for a previously overlooked domain of the molecule. Second, they show that mitochondrial dysfunction is not caused solely by fission, because this K148R mutant causes efficient fragmentation. Finally, they imply that the hFis1 mutant lacking the first α-helix induces PTP-dependent mitochondrial dysfunction and death via its intact IMS stretch (Yu et al., 2005). In this view, the IMS stretch could somehow communicate with the inner mitochondrial membrane to elicit opening of the PTP and perhaps remodelling of the cristae, a process accompanied by transient opening of the this channel (Scorrano et al., 2002; Germain et al., 2005). On this line, we found that ~40% of mitochondria overexpressing hFis1 displayed a morphology reminiscent of cristae remodelling. Opening of the PTP has been reported in cells expressing the α1-deleted mutant of hFis1 and associated with the appearance of “swollen” mitochondria as opposed to the small, fragmented ones caused by expression of wt hFis1 (Yu et al., 2005). Interestingly, we found that this mutant interferes with mitochondrial fusion, raising the possibility that the coalesced appearance of mitochondria expressing α1-deleted hFis1 is caused also by an inhibition of fusion. It remains to be addressed whether this inhibition is a consequence of the depolarization caused by the deleted hFis1 mutant (Yu et al., 2005).
In conclusion, our genetic dissection illustrates that hFis1 kills via the ER gateway, impinging on Ca2+-dependent, PTP-mediated mitochondrial dysfunction.
ACKNOWLEDGMENTS
We thank A. Cabrelle (Venetian Institute of Molecular Medicine Flow Cytometry Facility) for the skillful FACS analysis and C. Frezza for help with figures. L.S. is an Assistant Telethon Scientist of the Dulbecco-Telethon Institute. This research was supported by Telethon Italy; Associazione Italiana Ricerca sul Cancro, Italy; Compagnia di San Paolo; and Human Frontier Science Program Organization. E.A. was a recipient of a fellowship from the Société Académique de Genève and of the Swiss National Science Foundation (subsidy 3100A0-109419/1).
Abbreviations used:
CRC | Ca2+-retaining capacity of mitochondria |
CsA | cyclosporine A |
DKO, Bax−/−, Bak−/− | |
DRP1 | dynamin-related protein 1 |
ER | endoplasmic reticulum |
IMS | intermembrane space |
mtBAX | mitochondrially targeted BAX |
mtRFP | mitochondrially targeted dsRED |
NAC | N-acetylcysteine |
PTP | permeability transition pore |
ROS | reactive oxygen species |
SERCA | sarcoplasmic endoplasmic reticulum Ca2+ ATPase |
TMRM | tetramethyl rhodamine methyl ester. |
Footnotes
The online version of this article contains supplemental material at MBC Online (http://www.molbiolcell.org).
This article was published online ahead of print in MBC in Press (http://www.molbiolcell.org/cgi/doi/10.1091/mbc.E06-05-0377) on August 16, 2006.
REFERENCES
- Arnoult D., Rismanchi N., Grodet A., Roberts R. G., Seeburg D. P., Estaquier J., Sheng M., Blackstone C. Bax/Bak-dependent release of DDP/TIMM8a promotes Drp1-mediated mitochondrial fission and mitoptosis during programmed cell death. Curr. Biol. 2005;15:2112–2118. [Abstract] [Google Scholar]
- Bernardi P. Mitochondrial transport of cations: channels, exchangers and permeability transition. Physiol. Rev. 1999;79:1127–1155. [Abstract] [Google Scholar]
- Bossy-Wetzel E., Barsoum M. J., Godzik A., Schwarzenbacher R., Lipton S. A. Mitochondrial fission in apoptosis, neurodegeneration and aging. Curr. Opin. Cell Biol. 2003;15:706–716. [Abstract] [Google Scholar]
- Chen H., Chomyn A., Chan D. C. Disruption of fusion results in mitochondrial heterogeneity and dysfunction. J. Biol. Chem. 2005;280:26185–26192. [Abstract] [Google Scholar]
- Chen H., Detmer S. A., Ewald A. J., Griffin E. E., Fraser S. E., Chan D. C. Mitofusins Mfn1 and Mfn2 coordinately regulate mitochondrial fusion and are essential for embryonic development. J. Cell Biol. 2003;160:189–200. [Europe PMC free article] [Abstract] [Google Scholar]
- Chernyak B. V., Bernardi P. The mitochondrial permeability transition pore is modulated by oxidative agents through both pyridine nucleotides and glutathione at two separate sites. Eur. J. Biochem. 1996;238:623–630. [Abstract] [Google Scholar]
- Cipolat S., de Brito O. M., Dal Zilio B., Scorrano L. OPA1 requires mitofusin 1 to promote mitochondrial fusion. Proc. Natl. Acad. Sci. USA. 2004;101:15927–15932. [Europe PMC free article] [Abstract] [Google Scholar]
- Danial N. N., Korsmeyer S. J. Cell death: critical control points. Cell. 2004;116:205–219. [Abstract] [Google Scholar]
- Delivani P., Adrain C., Taylor R. C., Duriez P. J., Martin S. J. Role for CED-9 and Egl-1 as regulators of mitochondrial fission and fusion dynamics. Mol. Cell. 2006;21:761–773. [Abstract] [Google Scholar]
- Dimmer K. S., Fritz S., Fuchs F., Messerschmitt M., Weinbach N., Neupert W., Westermann B. Genetic basis of mitochondrial function and morphology in Saccharomyces cerevisiae. Mol. Biol. Cell. 2002;13:847–853. [Europe PMC free article] [Abstract] [Google Scholar]
- Dufour E., Larsson N. G. Understanding aging: revealing order out of chaos. Biochim. Biophys. Acta. 2004;1658:122–132. [Abstract] [Google Scholar]
- Fannjiang Y., Cheng W. C., Lee S. J., Qi B., Pevsner J., McCaffery J. M., Hill R. B., Basanez G., Hardwick J. M. Mitochondrial fission proteins regulate programmed cell death in yeast. Genes Dev. 2004;18:2785–2797. [Europe PMC free article] [Abstract] [Google Scholar]
- Ferri K. F., Kroemer G. Organelle-specific initiation of cell death pathways. Nat. Cell Biol. 2001;3:E255–E263. [Abstract] [Google Scholar]
- Fontaine E., Eriksson O., Ichas F., Bernardi P. Regulation of the permeability transition pore in skeletal muscle mitochondria. Modulation by electron flow through the respiratory chain complex I. J. Biol. Chem. 1998;273:12662–12668. [Abstract] [Google Scholar]
- Frank S., Gaume B., Bergmann-Leitner E. S., Leitner W. W., Robert E. G., Catez F., Smith C. L., Youle R. J. The role of dynamin-related protein 1, a mediator of mitochondrial fission, in apoptosis. Dev. Cell. 2001;1:515–525. [Abstract] [Google Scholar]
- Frieden M., James D., Castelbou C., Danckaert A., Martinou J. C., Demaurex N. Ca2+ homeostasis during mitochondrial fragmentation and perinuclear clustering induced by hFis1. J. Biol. Chem. 2004;279:22704–22714. [Abstract] [Google Scholar]
- Germain M., Mathai J. P., McBride H. M., Shore G. C. Endoplasmic reticulum BIK initiates DRP1-regulated remodelling of mitochondrial cristae during apoptosis. EMBO J. 2005;24:1546–1556. [Europe PMC free article] [Abstract] [Google Scholar]
- Griffiths G. J., Dubrez L., Morgan C. P., Jones N. A., Whitehouse J., Corfe B. M., Dive C., Hickman J. A. Cell damage-induced conformational changes of the pro-apoptotic protein Bak in vivo precede the onset of apoptosis. J. Cell Biol. 1999;144:903–914. [Europe PMC free article] [Abstract] [Google Scholar]
- Gugliucci A., Ranzato L., Scorrano L., Colonna R., Petronilli V., Cusan C., Prato M., Mancini M., Pagano F., Bernardi P. Mitochondria are direct targets of the lipoxygenase inhibitor M.K886. A strategy for cell killing by combined treatment with Mk886 and cyclooxygenase inhibitors. J. Biol. Chem. 2002;277:31789–31795. [Abstract] [Google Scholar]
- Hockenbery D. M., Oltvai Z. N., Yin X. M., Milliman C. L., Korsmeyer S. J. Bcl-2 functions in an antioxidant pathway to prevent apoptosis. Cell. 1993;75:241–251. [Abstract] [Google Scholar]
- Irwin W. A. Mitochondrial dysfunction and apoptosis in myopathic mice with collagen VI deficiency. Nat. Genet. 2003;35:367–371. [Abstract] [Google Scholar]
- Ishihara N., Eura Y., Mihara K. Mitofusin 1 and 2 play distinct roles in mitochondrial fusion reactions via GTPase activity. J. Cell Sci. 2004;117:6535–6546. [Abstract] [Google Scholar]
- Jaconi M., Pyle J., Bortolon R., Ou J., Clapham D. Calcium release and influx colocalize to the endoplasmic reticulum. Curr. Biol. 1997;7:599–602. [Abstract] [Google Scholar]
- James D. I., Parone P. A., Mattenberger Y., Martinou J. C. hFis1, a novel component of the mammalian mitochondrial fission machinery. J. Biol. Chem. 2003;278:36373–36379. [Abstract] [Google Scholar]
- Karbowski M., Arnoult D., Chen H., Chan D. C., Smith C. L., Youle R. J. Quantitation of mitochondrial dynamics by photolabeling of individual organelles shows that mitochondrial fusion is blocked during the Bax activation phase of apoptosis. J. Cell Biol. 2004;164:493–499. [Europe PMC free article] [Abstract] [Google Scholar]
- Karbowski M., Lee Y. J., Gaume B., Jeong S. Y., Frank S., Nechushtan A., Santel A., Fuller M., Smith C. L., Youle R. J. Spatial and temporal association of Bax with mitochondrial fission sites, Drp1, and Mfn2 during apoptosis. J. Cell Biol. 2002;159:931–938. [Europe PMC free article] [Abstract] [Google Scholar]
- Kowaltowski A. J., Castilho R. F., Grijalba M. T., Bechara E. J., Vercesi A. E. Effect of inorganic phosphate concentration on the nature of inner mitochondrial membrane alterations mediated by Ca2+ions. A proposed model for phosphate-stimulated lipid peroxidation. J. Biol. Chem. 1996;271:2929–2934. [Abstract] [Google Scholar]
- Lee Y. J., Jeong S. Y., Karbowski M., Smith C. L., Youle R. J. Roles of the mammalian mitochondrial fission and fusion mediators Fis1, Drp1, and Opa1 in apoptosis. Mol. Biol. Cell. 2004;15:5001–5011. [Europe PMC free article] [Abstract] [Google Scholar]
- Legros F., Lombes A., Frachon P., Rojo M. Mitochondrial fusion in human cells is efficient, requires the inner membrane potential, and is mediated by mitofusins. Mol. Biol. Cell. 2002;13:4343–4354. [Europe PMC free article] [Abstract] [Google Scholar]
- Li C., Fox C. J., Master S. R., Bindokas V. P., Chodosh L. A., Thompson C. B. Bcl-XL affects Ca2+ homeostasis by altering expression of inositol 1,4,5-trisphosphate receptors. Proc. Natl. Acad. Sci. USA. 2002;99:9830–9835. [Europe PMC free article] [Abstract] [Google Scholar]
- Neuspiel M., Zunino R., Gangaraju S., Rippstein P., McBride H. M. Activated Mfn2 signals mitochondrial fusion, interferes with Bax activation and reduces susceptibility to radical induced depolarization. J. Biol. Chem. 2005 [Google Scholar]
- Nijtmans L. G., Henderson N. S., Holt I. J. Blue native electrophoresis to study mitochondrial and other protein complexes. Methods. 2002;26:327–334. [Abstract] [Google Scholar]
- Okamoto K., Shaw J. M. Mitochondrial morphology and dynamics in yeast and multicellular eukaryotes. Annu. Rev. Genet. 2005;39:503–536. [Abstract] [Google Scholar]
- Ono T., Isobe K., Nakada K., Hayashi J. I. Human cells are protected from mitochondrial dysfunction by complementation of DNA products in fused mitochondria. Nat. Genet. 2001;28:272–275. [Abstract] [Google Scholar]
- Pinton P., Ferrari D., Magalhaes P., Schulze-Osthoff K., Di V. F., Pozzan T., Rizzuto R. Reduced loading of intracellular Ca2+ stores and downregulation of capacitative Ca2+influx in Bcl-2-overexpressing cells. J. Cell Biol. 2000;148:857–862. [Europe PMC free article] [Abstract] [Google Scholar]
- Reid A. B., Kurten R. C., McCullough S. S., Brock R. W., Hinson J. A. Mechanisms of acetaminophen-induced hepatotoxicity: role of oxidative stress and mitochondrial permeability transition in freshly isolated mouse hepatocytes. J. Pharmacol. Exp. Ther. 2005;312:509–516. [Abstract] [Google Scholar]
- Rizzuto R., Bernardi P., Pozzan T. Mitochondria as all-round players of the calcium game. J. Physiol. 2000;529(Pt1):37–47. [Abstract] [Google Scholar]
- Santel A., Frank S., Gaume B., Herrler M., Youle R. J., Fuller M. T. Mitofusin-1 protein is a generally expressed mediator of mitochondrial fusion in mammalian cells. J. Cell Sci. 2003;116:2763–2774. [Abstract] [Google Scholar]
- Santel A., Fuller M. T. Control of mitochondrial morphology by a human mitofusin. J. Cell Sci. 2001;114:867–874. [Abstract] [Google Scholar]
- Schagger H. Native electrophoresis for isolation of mitochondrial oxidative phosphorylation protein complexes. Methods Enzymol. 1995;260:190–202. [Abstract] [Google Scholar]
- Scorrano L., Ashiya M., Buttle K., Weiler S., Oakes S. A., Mannella C.A., Korsmeyer S. J. A distinct pathway remodels mitochondrial cristae and mobilizes cytochrome c during apoptosis. Dev. Cell. 2002;2:55–67. [Abstract] [Google Scholar]
- Scorrano L., Korsmeyer S. J. Mechanisms of cytochrome c release by proapoptotic BCL-2 family members. Biochem. Biophys. Res. Commun. 2003;304:437–444. [Abstract] [Google Scholar]
- Scorrano L., Oakes S. A., Opferman J. T., Cheng E. H., Sorcinelli M. D., Pozzan T., Korsmeyer S. J. BAX and BAK regulation of endoplasmic reticulum Ca2+: a control point for apoptosis. Science. 2003;300:135–139. [Abstract] [Google Scholar]
- Smirnova E., Griparic L., Shurland D. L., van der Bliek A. M. Dynamin-related protein Drp1 is required for mitochondrial division in mammalian cells. Mol. Biol. Cell. 2001;12:2245–2256. [Europe PMC free article] [Abstract] [Google Scholar]
- Stojanovski D., Koutsopoulos O. S., Okamoto K., Ryan M. T. Levels of human Fis1 at the mitochondrial outer membrane regulate mitochondrial morphology. J. Cell Sci. 2004;117:1201–1210. [Abstract] [Google Scholar]
- Sugioka R., Shimizu S., Tsujimoto Y. Fzo1, a protein involved in mitochondrial fusion, inhibits apoptosis. J. Biol. Chem. 2004;279:52726–52734. [Abstract] [Google Scholar]
- Suzuki M., Jeong S. Y., Karbowski M., Youle R. J., Tjandra N. The solution structure of human mitochondria fission protein Fis1 reveals a novel TPR-like helix bundle. J. Mol. Biol. 2003;334:445–458. [Abstract] [Google Scholar]
- Szabadkai G., Simoni A. M., Chami M., Wieckowski M. R., Youle R. J., Rizzuto R. Drp-1-dependent division of the mitochondrial network blocks intraorganellar Ca2+waves and protects against Ca2+-mediated apoptosis. Mol. Cell. 2004;16:59–68. [Abstract] [Google Scholar]
- Westermann B. Merging mitochondria matters: cellular role and molecular machinery of mitochondrialfusion. EMBO Rep. 2002;3:527–531. [Europe PMC free article] [Abstract] [Google Scholar]
- Yi M., Weaver D., Hajnoczky G. Control of mitochondrial motility and distribution by the calcium signal: a homeostatic circuit. J. Cell Biol. 2004;167:661–672. [Europe PMC free article] [Abstract] [Google Scholar]
- Yoon Y., Krueger E. W., Oswald B. J., McNiven M. A. The mitochondrial protein hFis1 regulates mitochondrial fission in mammalian cells through an interaction with the dynamin-like protein DLP1. Mol. Cell. Biol. 2003;23:5409–5420. [Europe PMC free article] [Abstract] [Google Scholar]
- Yu T., Fox R. J., Burwell L. S., Yoon Y. Regulation of mitochondrial fission and apoptosis by the mitochondrial outer membrane protein hFis1. J. Cell Sci. 2005;118:4141–4151. [Abstract] [Google Scholar]
- Yu T., Robotham J. L., Yoon Y. Increased production of reactive oxygen species in hyperglycemic conditions requires dynamic change of mitochondrial morphology. Proc. Natl. Acad. Sci. USA. 2006;103:2653–2658. [Europe PMC free article] [Abstract] [Google Scholar]
- Zamzami N., Marchetti P., Castedo M., Decaudin D., Macho A., Hirsch T., Susin S. A., Petit P. X., Mignotte B., Kroemer G. Sequential reduction of mitochondrial transmembrane potential and generation of reactive oxygen species in early programmed cell death. J. Exp. Med. 1995;182:367–377. [Europe PMC free article] [Abstract] [Google Scholar]
Articles from Molecular Biology of the Cell are provided here courtesy of American Society for Cell Biology
Full text links
Read article at publisher's site: https://doi.org/10.1091/mbc.e06-05-0377
Read article for free, from open access legal sources, via Unpaywall:
https://europepmc.org/articles/pmc1635393?pdf=render
Citations & impact
Impact metrics
Citations of article over time
Alternative metrics

Discover the attention surrounding your research
https://www.altmetric.com/details/102672166
Article citations
A preliminary study of the miRNA restitution effect on CNV-induced miRNA downregulation in CAKUT.
BMC Genomics, 25(1):218, 27 Feb 2024
Cited by: 1 article | PMID: 38413914 | PMCID: PMC10900603
Role of Mitochondrial Dynamics in Heart Diseases.
Genes (Basel), 14(10):1876, 26 Sep 2023
Cited by: 8 articles | PMID: 37895224 | PMCID: PMC10606177
Review Free full text in Europe PMC
Human Fis1 directly interacts with Drp1 in an evolutionarily conserved manner to promote mitochondrial fission.
J Biol Chem, 299(12):105380, 20 Oct 2023
Cited by: 3 articles | PMID: 37866629 | PMCID: PMC10694664
PEX11β and FIS1 cooperate in peroxisome division independently of mitochondrial fission factor.
J Cell Sci, 135(13):jcs259924, 08 Jul 2022
Cited by: 9 articles | PMID: 35678336 | PMCID: PMC9377713
Mitochondria-Associated Membrane Scaffolding with Endoplasmic Reticulum: A Dynamic Pathway of Developmental Disease.
Front Mol Biosci, 9:908721, 14 Jun 2022
Cited by: 1 article | PMID: 35775081 | PMCID: PMC9237565
Go to all (90) article citations
Other citations
Wikipedia
Data
Data behind the article
This data has been text mined from the article, or deposited into data resources.
BioStudies: supplemental material and supporting data
Similar Articles
To arrive at the top five similar articles we use a word-weighted algorithm to compare words from the Title and Abstract of each citation.
Inhibiting the mitochondrial fission machinery does not prevent Bax/Bak-dependent apoptosis.
Mol Cell Biol, 26(20):7397-7408, 01 Oct 2006
Cited by: 165 articles | PMID: 17015472 | PMCID: PMC1636857
The mitochondrial outer membrane protein hFis1 regulates mitochondrial morphology and fission through self-interaction.
Exp Cell Res, 314(19):3494-3507, 25 Sep 2008
Cited by: 29 articles | PMID: 18845145 | PMCID: PMC2605612
Regulation of mitochondrial fission and apoptosis by the mitochondrial outer membrane protein hFis1.
J Cell Sci, 118(pt 18):4141-4151, 23 Aug 2005
Cited by: 129 articles | PMID: 16118244
Regulation of endoplasmic reticulum Ca2+ dynamics by proapoptotic BCL-2 family members.
Biochem Pharmacol, 66(8):1335-1340, 01 Oct 2003
Cited by: 74 articles | PMID: 14555206
Review
Funding
Funders who supported this work.
Swiss National Science Foundation (1)
Grant ID: 109419
Telethon (1)
MITOCHONDRIAL APOPTOTIC PATHWAYS AND MITOCHONDRIAL REMODELLING IN DOMINANT OPTIC ATROPHY
Prof Luca Scorrano, Fondazione per la Ricerca Biomedica Avanzata Onlus - Istituto Veneto di Medicina Molecolare (VIMM), Centro di Ricerca della Fondazione per la Ricerca Biomedica Avanzata
Grant ID: TCP02016