Abstract
Free full text

WIP is a chaperone for Wiskott–Aldrich syndrome protein (WASP)
Associated Data
Abstract
Wiskott–Aldrich syndrome protein (WASP) is in a complex with WASP-interacting protein (WIP). WASP levels, but not mRNA levels, were severely diminished in T cells from WIP−/− mice and were increased by introduction of WIP in these cells. The WASP binding domain of WIP was shown to protect WASP from degradation by calpain in vitro. Treatment with the proteasome inhibitors MG132 and bortezomib increased WASP levels in T cells from WIP−/− mice and in T and B lymphocytes from two WAS patients with missense mutations (R86H and T45M) that disrupt WIP binding. The calpain inhibitor calpeptin increased WASP levels in activated T and B cells from the WASP patients, but not in primary T cells from the patients or from WIP−/− mice. Despite its ability to increase WASP levels proteasome inhibition did not correct the impaired IL-2 gene expression and low F-actin content in T cells from the R86H WAS patient. These results demonstrate that WIP stabilizes WASP and suggest that it may also be important for its function.
WASP, the product of the gene mutated in Wiskott–Aldrich syndrome (WAS), is expressed only in hematopoietic cells and is the first identified member of a family of proteins that include N-WASP and Scar/WAVE (1–3). WASP plays a critical role in T cell activation and actin reorganization. WASP translocates to lipid rafts after T cell receptor (TCR) ligation and localizes at the immune synapse between T cells and antigen-presenting cells (4, 5). T cells from WAS patients and WASP−/− mice are deficient in their ability to increase their F-actin content, secrete IL-2, and proliferate after TCR ligation (6–8).
A complex of WASP/N-WASP and WASP-interacting protein (WIP) is detected in resting cells (9, 10). WIP is expressed at high levels in lymphoid tissues (11). NMR studies suggest a narrow but extended contact between WIP and N-WASP with amino acid residues 461–485 of WIP wrapping themselves around the EVH1 domain of N-WASP (12). WIP plays an important role in the recruitment of the WIP–WASP complex to ZAP-70 after TCR ligation (5). T cells from WIP knockout mice fail to proliferate, secrete IL-2, polarize, and extend protrusions after TCR ligation, are deficient in immune synapse formation, and have a disorganized actin cytoskeleton (13).
Most missense mutations in patients with WAS and X-linked thrombocytopenia are localized to the WIP binding EVH1 domain of WASP (12, 14). In fact, three of these mutations, R86H, Y107C, and A134T, which affect residues that are predicted by NMR studies to be contact points for WIP, were shown to disrupt WIP binding (15). Here we demonstrate that WIP stabilizes WASP from degradation by calpain and the proteasome.
Results
WASP Is Unstable in the Absence of WIP.
Expression of WASP in splenic T cells from WIP−/− mice was severely diminished (Fig. 1A). In contrast, expression of Vav1 and actin was unaffected (Fig. 1A). Scanning densitometry revealed that the level of WASP expression in WIP−/− T cells was 8.4 ± 0.9% of controls (n = 3). Diminished expression of WASP in WIP−/− T cells was confirmed by intracellular FACS analysis by using anti-WASP mAb (Fig. 1B). Northern blot analysis revealed similar levels of WASP mRNA in WIP−/− and WT T cells (Fig. 1C). WASP mRNA levels assessed by quantitative RT-PCR were similar in WT and WIP−/− T cells (mean WASP mRNA level in WIP−/− cells, normalized to GAPDH, 90 ± 8% of control T cells, n = 3; data not shown). Examination of B cells from WIP−/− mice revealed severely decreased WASP levels to 10.0 ± 3.0% of control (n = 3) (Fig. 1D). N-WASP level was similar in cultured lung fibroblasts from WIP−/− and WT controls (mean N-WASP level in WIP−/− fibroblasts, normalized to actin, 111 ± 3% of control fibroblasts) (Fig. 1E). WIP level was not affected by the absence of WASP (mean WIP level in WASP−/− T cells, normalized to actin level, was 94 ± 2% of control WT T cells, n = 3) (Fig. 1F). These results suggest that WIP stabilizes WASP.
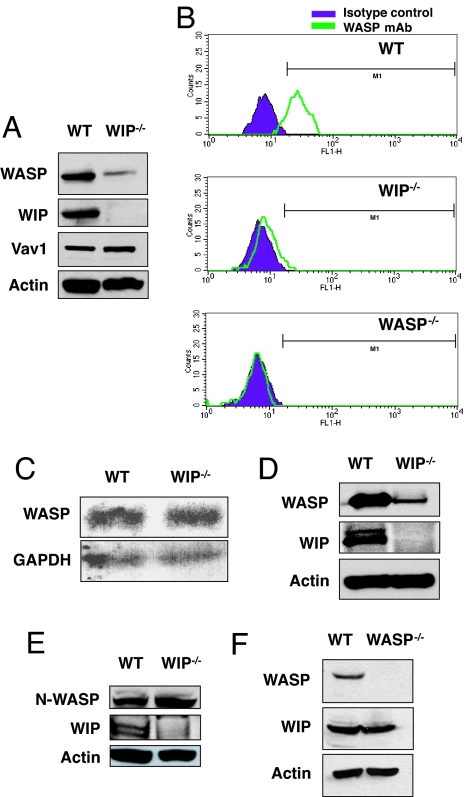
WASP is unstable in WIP−/− T cells. (A) WASP expression in T cells from WIP−/− and WT control mice. Vav and actin protein expression levels are shown as controls. (B) FACS analysis of intracellular WASP expression in WT, WIP−/−, and WASP−/− splenic T cells. Cells were stained with anti-WASP mAb 5A5 and isotype-matched mouse IgG2a as control. (C) Northern blot analysis of WASP mRNA in splenic T cells from WIP−/− mice and WT controls. (D) WASP expression in B cells from WIP−/− and WT controls. (E) N-WASP expression in fibroblasts from WIP−/− mice and WT controls. (F) WIP expression in T cells from WASP−/− mice and WT controls.
Overexpression of WIP Results in Increased WASP Levels.
Jurkat cells were stably transfected with myc-tagged constructs that encode C-terminal WIP (C-WIP, amino acids 401–503) or N-terminal WIP (N-WIP, amino acids 1–400), placed under the control of the doxycycline (DOX)-inducible promoter. Myc-tagged C-WIP but not myc-tagged N-WIP was confirmed to bind WASP (Fig. 2A). DOX treatment of C-WIP-transfected cells, but not of N-WIP-transfected cells, for 48 h resulted in an ≈3-fold increase in WASP level (Fig. 2B), with no change in WASP mRNA expression (data not shown). There was no detectable change in the level of endogenous WIP in Jurkat cells transfected with either C-WIP or N-WIP (data not shown). Overexpression of WIP–GFP in T293 cells caused no detectable increase in N-WASP levels (Fig. 2C). These results suggest that WIP stabilizes WASP, but not N-WASP, in cells.
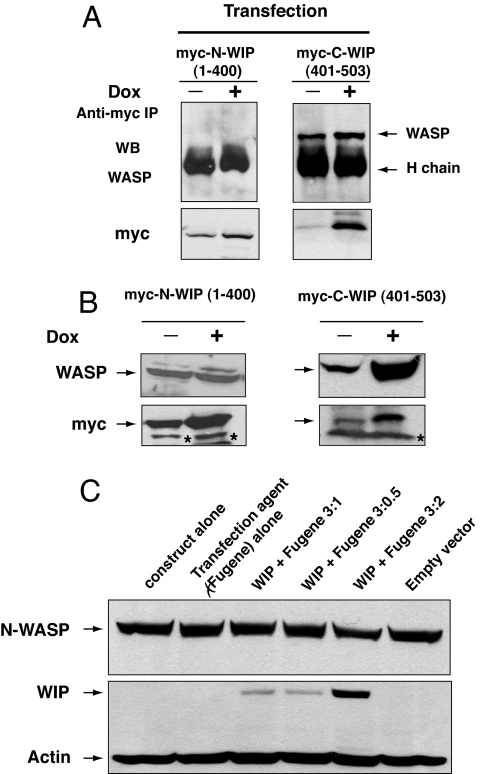
Overexpression of WIP in cells results in increased levels of WASP, but not N-WASP. (A) WASP binding to Myc-tagged N-WIP (1–400) and C-WIP (401–503) in Jurkat cells transfected with Myc-tagged C-WIP and N-WIP under the control of a DOX-inducible promoter. The presence of WASP in Myc immunoprecipitates was examined before and after DOX treatment. To achieve optimal resolution of Myc-N-WIP (≈50 kDa) and to avoid Myc-C-WIP (≈11 kDa) running off the gel, the gels were run for different time periods. (B) WASP expression in the same Jurkat cells. Representative results from one of four independent clones for each construct are shown. The asterisk denotes nonspecific bands. (C) Forced expression of WIP in T293 cells does not alter N-WASP levels.
Expression of WIP Increases WASP Levels in WIP−/− T Cells.
T cells were transfected by nucleofection with plasmids encoding for GFP, WIP–GFP, and WIPΔWASP–GFP, a deletion mutant that lacks amino acids 461–488, which are essential for WIP binding (12). Fig. 3A shows that probing of GFP immunoprecipitates from transfected cells revealed expression of GFP–WIP fusion proteins of the appropriate size as detected by anti-GFP antibodies. Fig. 3B shows that WASP was detected in GFP immunoprecipitates from WIP–GFP-transfected cells, but not from WIPΔWASP–GFP-transfected or GFP-transfected cells. Fig. 3C shows that expression of WIP–GFP, but not of WIPΔWASP–GFP or GFP, in WIP−/− T cells resulted in an increase in WASP levels as determined by FACS. The mean increase in WASP expression was 2.2 ± 0.3-fold in WIP–GFP-transfected cells and 0.9 ± 0.1-fold with WIPΔWASP–GFP transfection (n = 3, P < 0.05). These results confirm that WIP binding is essential for WASP stability.
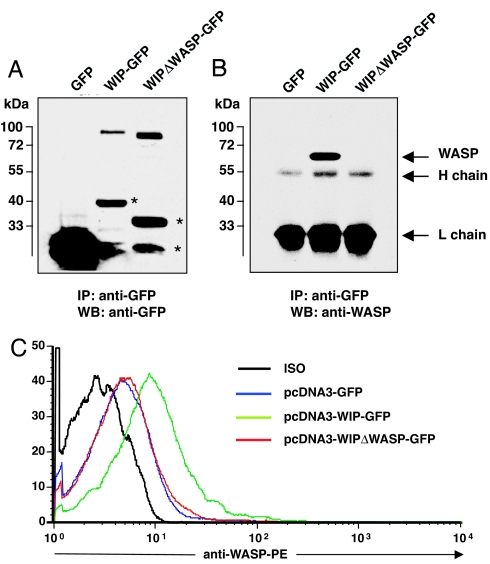
Forced expression of WIP in WIP−/− T cells increases WASP levels. (A) Expression of GFP proteins in mouse transfected T cells. The asterisks denote degradation products. (B) WASP binding to GFP proteins in transfected thymocytes. (C) FACS analysis of intracellular WASP expression in WIP−/− T cells expressing WIP–GFP, WIPΔWASP–GFP, and GFP alone.
The Majority of WASP and WIP Exist in a Complex in T Cells.
To determine how much of WASP in T cells is associated with WIP we examined the residual amount of WASP in T cell lysates immunodepleted of WIP by five sequential immunoprecipitations using WIP mAb. Fig. 4A shows that virtually no WIP could be detected in the T cell lysates after WIP immunodepletion. Scanning densitometry revealed that the amount of WASP that remained in lysates immunodepleted of WIP was 27% and 20%, respectively, in two independent experiments. Fig. 4B shows that virtually no WASP could be detected in the T cell lysates after WASP immunodepletion. Scanning densitometry revealed that the amount of WIP that remained in lysates immunodepleted of WASP was, respectively, 12% and 14% in two independent experiments. Thus, the majority of WASP and WIP are associated with each other in T cells.
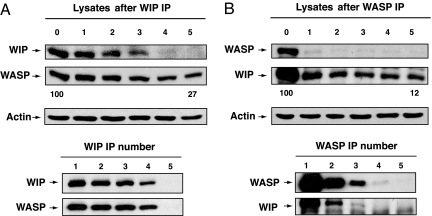
Effect of immunodepletion of WIP on WASP levels (A) and of WASP on WIP levels (B) in T cells. T cell lysates were immunoprecipitated sequentially five times with anti-WASP or anti-WIP mAbs, and the lysates and immunoprecipitates were run on gels and Western blotted and probed for WASP or WIP. The numbers 0–5 refer to the number of times sequential immunoprecipitation was performed. The numbers below the Western blot of the lysates refer to the percent residual WASP or WIP with 100 being the value in the lysates before immunodepletion.
WIP Protects WASP from Degradation by Calpain in Vitro.
WASP in platelet lysates is degraded by the calcium-dependent protease calpain (16). We first determined whether WASP is a direct substrate for calpain. In vitro transcribed and translated WASP was completely degraded by calpain I at 20 units/ml or more but not by caspase 3 [supporting information (SI) Fig. 7A]. GST-C-WIP (amino acids 401–503), which binds to WASP, but not GST-N-WIP (amino acids 1–108), which does not bind to WASP, inhibited the degradation of WASP by calpain I (SI Fig. 7B). This was not because of interference with calpain enzymatic activity, because GST-C-WIP did not inhibit the degradation of CrkL, a known substrate of calpain (17) (SI Fig. 7B). These results suggest that WIP masks calpain-sensitive sites in WASP.
Inhibitors of the Proteasome Increase WASP Levels in WIP−/− T Cells.
The finding that WASP is a substrate for calpain and the observation that N-WASP is subject to ubiquitination (18) prompted us to examine whether inhibitors of calpain and/or the proteasome increase WASP expression in WIP−/− T cells. FACS analysis revealed that preincubation for 6 h of WIP−/− T cells with MG132 increased the level of WASP, but not Vav1 protein (Fig. 5A). The mean fluorescence intensity for WASP staining in WIP−/− T cells treated with MG132 was 2.2 ± 0.2-fold that of untreated WIP−/− T cells (n = 3, P < 0.05). The level of WASP in WIP−/− T cells compared with that of untreated WT T cells rose from 0.10 ± 0.02 to 0.22 ± 0.03 after MG132 treatment as determined by mean fluorescence intensity (n = 3, P < 0.05). In contrast to MG132, calpeptin had no detectable effect on WASP levels in WIP−/− T cells and exerted no additional effect to that of MG132 when WIP−/− T cells were treated with both inhibitors. There was no detectable increase in the level of WASP in normal T cells treated with MG132 and/or calpeptin (Fig. 5A).
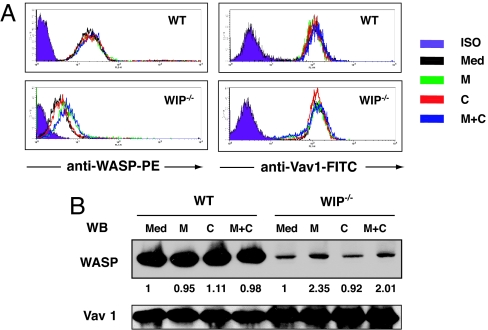
Inhibitors of calpain and the proteasome increase WASP levels in T cells from WIP−/− mice. T cells from WT and WIP−/− mice were incubated with medium containing DMSO vehicle (Med), MG132 (M), calpeptin (C), or both (M+C) for 6 h, then analyzed for WASP by flow cytometry by using mAb B-9 conjugated to phycoerythrin (A) or by Western blot using B-9 mAb (B). Expression of Vav1 was used as control. The numbers in B represent the ratio of WAS:Vav1 in inhibitor-treated cells versus untreated control cultured with medium alone.
Western blot analysis of T cell lysates confirmed the results obtained by FACS (Fig. 5B). Densitometric scanning revealed that the mean WASP levels in WIP−/− T cells treated with MG132 was 2.4 ± 0.2-fold that of untreated WIP−/− T cells (n = 3, P < 0.05). The level of WASP in WIP−/− T cells compared with that of untreated WT T cells rose from 0.09 ± 0.01 to 0.23 ± 0.04 after MG132 treatment (n = 3, P < 0.05). Calpeptin had no detectable effect on WASP levels in WIP−/− T cells. Increasing the concentrations of the inhibitors and/or increasing the incubation time beyond 6 h caused increased cell death and did not result in a detectable increase in WASP levels. These results suggest that degradation by the ubiquitin–proteasome pathway contributes to WASP degradation in the absence of WIP.
We confirmed the above results using additional proteasome and calpain inhibitors. Treatment of WIP−/− T cells with the proteasome inhibitor bortezomib caused an increase in WASP levels comparable to that observed with MG132 (SI Fig. 8A). This inhibitor caused negligible cell death after 6 h of treatment but resulted in >90% cell mortality by 24 h. In contrast, treatment of WIP−/− T cells with the calpain inhibitor CS peptide (27-aa peptide encoded by exon 1B of human calpastatin) caused no detectable increase in WASP levels (SI Fig. 8B). Both calpeptin and CS peptide were shown to substantially reduce calpain activity in T cell lysates (SI Fig. 8C).
Effect of Proteasome and Calpain Inhibitors on WASP Levels in Lymphocytes from WAS Patients with Missense Mutations in WASP That Disrupt WIP Binding.
The majority of WAS patients with missense mutations in WASP have point mutations in the WIP binding domain (19). One of these mutations, R86H, was shown to disrupt WIP binding and to be associated with severely diminished WASP levels with normal mRNA levels (15). Western blot analysis of lysates from freshly isolated T cells from a patient with the R86H mutation revealed that the WASP level in these T cells was 9% that of T cells from healthy controls (n = 2 independent experiments using T cells from two healthy donors as controls in each). Preincubation of freshly isolated T cells from this patient with MG132 increased WASP levels by a factor of 2.4-fold (mean of two experiments). This corresponded to a WASP level 22% that of untreated T cells from normal controls. There was no detectable effect of calpeptin on WASP levels in the primary T cells from this patient, with a mean increase of 0.85-fold in two experiments (Fig. 6A). The combination of MG132 and calpeptin caused a mean increase of 1.8-fold in WASP levels (n = 2) and was associated with increased cell death. Neither MG132 nor calpeptin caused a detectable increase in the level of WASP in normal T cells or of Vav1 in either normal or patient T cells.
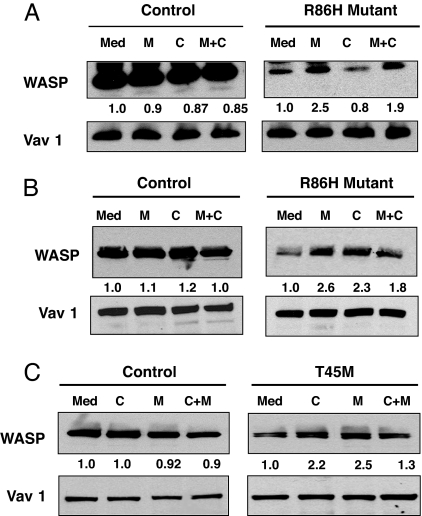
Effect of calpain and proteasome inhibitors on WASP levels in lymphocytes from WAS patients. Effect of inhibitors on WASP levels in purified peripheral blood T cells and PHA/IL-2 propagated T cells from a WAS patient with R86H mutation (A and B) and in EBV-transformed B cells from a WAS patient with T45M mutation (C).
T cell activation results in activation of calpain (20–24). We examined the effect of calpeptin on WASP levels in PHA- and IL-2-propagated T cell lines derived from the patient with the R86H mutation and two normal controls. The mean WASP content of two independent T cell lines from the patient was 12% that of T cells lines derived from the two normal donors. Preincubation with MG132 increased WASP levels in the patient's cell lines by 2.3 ± 0.4-fold (n = 3) to 28% that of untreated normal T cell lines. Calpeptin treatment of the patient's T cell lines caused a 2.1 ± 0.35-fold (n = 3) increase in WASP levels (Fig. 6B). The combination of MG132 and calpeptin caused a 1.8 ± 0.21-fold (n = 3) increase in WASP levels and was associated with increased cell death. This corresponded to a WASP level 25% that of untreated normal T cell lines. MG132 had no detectable effect on Vav1 levels in the patient's cell lines. MG132 and calpeptin had no detectable effect on WASP or Vav1 levels in normal T cell lines. These results suggest that both calpain and the proteasome participate in the degradation of the mutant WASP in activated T cells.
We examined the effect of calpeptin and MG132 on WASP levels using EBV-transformed B cell lines derived from a WAS patient with a T45M mutation and two healthy controls. The T45M mutation is predicted by NMR studies to be a contact point for WIP binding (12). The patient's peripheral blood mononuclear cells and B cell line expressed WASP at a level that ranged from 15% to 20% that of control but had normal WASP mRNA levels (25) (Fig. 6C and data not shown). Preincubation of the patient's B cell line with MG132 or calpeptin increased WASP levels by 2.3 ± 0.27-fold and 2.1 ± 0.22-fold, respectively (mean of three experiments), but had no detectable effect on Vav1 levels (Fig. 6C). The combination of MG132 and calpeptin caused a 1.4 ± 0.21-fold (n = 3) increase in WASP levels and was associated with increased cell death. Addition of both inhibitors resulted in diminished up-regulation of WASP levels compared with either inhibitor alone and was associated with increased cell death. MG132 and calpeptin had no detectable effect on WASP or Vav1 levels in normal B cell lines. These results suggest that both calpain and the proteasome participate in the degradation of the mutant WASP in activated B cells.
Effect of the Proteasome Inhibitor Bortezomib on Actin Content and IL-2 Expression by T Cells with R86H Mutation in WASP.
Primary T cells from patients with WAS have impaired IL-2 gene expression after stimulation by immobilized anti-CD3 mAb (26), and herpes virus saimiri-transformed T cell lines from these patients have a defect in polymerizing actin after TCR ligation (6). Because the proteasome inhibitor bortezomib was not toxic to murine T cells in the first 6 h, we examined its ability to correct the functional defect in primary T cells from the patient with the R86H mutation in WASP. Bortezomib caused negligible death of human T cells at 10 h but substantial death (>90%) at 24 h. Incubation of the patient's T cells with bortezomib for 10 h resulted in a 2.4 ± 0.5-fold increase in WASP level to 23% of the level in control T cells (n = 3), results similar to those obtained with MG132. However, bortezomib failed to ameliorate the impaired IL-2 gene expression in response to immobilized anti-CD3 mAb (SI Fig. 9A). T cells from the patient had decreased F-actin content, but, in contrast to what was reported for herpes virus saimiri-transformed T cell lines from WAS patients, they were able to polymerize actin after anti-CD3 stimulation. Bortezomib had no effect on the low F-actin content of these cells (SI Fig. 9B).
Discussion
Our results show that WIP deficiency or impaired WASP–WIP interaction results in WASP degradation by the proteasome and calpain. WASP levels were markedly diminished in T cells from WIP−/− mice and in lymphocytes of WAS patients with missense mutations in the EVH1 domain of WASP that disrupt WIP binding. The decrease occurred in the face of normal levels of WASP mRNA (Figs. 1A and and6).6). In contrast, WIP levels were normal in WASP-deficient T cells (Fig. 1F), indicating that WIP is necessary for the stabilization of WASP, but not vice versa. The finding that N-WASP levels were normal in WIP−/− fibroblasts (Fig. 1E) suggests that WIP may not be needed to stabilize N-WASP. This could be because of an intrinsic property of N-WASP or/and the expression in nonhematopoietic cells of WIP homologues, such as CR16 and WIRE/WICH (27–29).
Stabilization of WASP by WIP is likely the result of their direct interaction rather than an indirect effect of WIP on WASP degradation. First, overexpression of a WIP fragment that binds WASP, but not of a WIP fragment that does not bind WASP, increased WASP expression in Jurkat cells (Fig. 2). Second, expression of WIP, but not of a WIP deletion mutant that does bind WASP, resulted in an increase in WASP levels in WIP−/− T cells (Fig. 3). Third, a WIP fragment that binds WASP, but not a WIP fragment that does not bind WASP, protected WASP from degradation by calpain in vitro (SI Fig. 7). Finally, WASP levels are decreased in lymphocytes from patients with point mutations that destroy WIP binding (Fig. 6). We were unable to detect WASP fragments in WIP−/− T cells or in lymphocytes from WAS patients with the R86H and T45M mutations. This is possibly because of rapid degradation of these fragments.
Preincubation with the proteasome inhibitor MG132, but not with the calpain inhibitor calpeptin, partially restored WASP levels in primary T cells from WIP−/− mice and from a WAS patient with a missense mutation in the EVH1 domain of WASP, R86H, that disrupts WIP binding (Figs. 5 and and66A). Similar results were obtained in WIP−/− T cells with the proteasome inhibitor bortezomib and the calpain inhibitor CS peptide (SI Fig. 8). This suggests that WIP is a chaperone for WASP and that, in its absence, WASP can be degraded in primary T cells by the ubiquitin–proteasome pathway. The increase in WASP levels was specific because it was not observed in normal T cells and because Vav1 levels were unaffected. The failure to observe a detectable effect of MG132 on WASP levels in normal T cells may be explained by the observation that the majority of WASP is complexed with WIP in T cells (Fig. 4).
Our findings implicate calpain in WASP degradation in activated lymphocytes of patients with WASP mutations that disrupt WIP binding. In contrast to primary T cells, calpain inhibition increased WASP levels in activated T cells of the R86H patients and in EBV-transformed cells from another WAS patient with a missense mutation, T45M, that disrupts WIP binding (Fig. 6 B and C). Calpain is known to be activated after TCR and B cell receptor cross-linking (22, 30). Furthermore, calpain mRNA and expression levels are increased after activation of T and B cells (31).
WASP has seven potential calpain-sensitive sites based on the highly stringent criterion of P1 residue being Y, M, or R and P2 residue being a hydrophobic amino acid (32). Four of these sites are in the EVH1 domain. Using less stringent criteria, there are several additional potential calpain-sensitive sites in WASP. Our finding that the C-terminal WASP binding domain of WIP specifically protected WASP from degradation by calpain (SI Fig. 7) suggests that calpain attacks sites in the EVH1 domain of WASP and that WIP masks these sites. The minimal WIP peptide that binds WASP spans amino acids 461–485. We found that this peptide does not protect WASP from degradation by calpain in vitro (data not shown), suggesting that other regions of WIP contribute to its ability to protect WASP from degradation.
There are many similarities in the phenotype of WAS knockout mice and WIP knockout mice. They include defective proliferation and IL-2 secretion in response to immobilized anti-CD3 and defective immune synapse formation and F-actin reorganization (8, 13, 33). The finding that WASP levels are severely diminished in T cells from WIP−/− mice suggests that WASP deficiency contributes to the phenotype of these mice. However, WIP−/− T cells, but not WASP−/− T cells, have a disrupted subcortical actin cytoskeleton (13) and show severe defects in chemotaxis in response to stromal cell-derived factor 1α (34). Furthermore, the phenotype of WIP and WASP double deficient mice is markedly more severe than that of either WIP or WASP single deficient mice, with reduced numbers of thymocytes and peripheral CD4+ T cells and early development of colitis, pneumonitis, and iritis (our unpublished observations). These observations strongly suggest that WIP, which interacts independent of WASP with a number of proteins that include Nck, CrkL, Syk, actin, and profilin (5, 11, 35, 36), has distinct functions, which are not dependent on WASP.
We were unable to observe amelioration of the impaired IL-2 gene expression in response to immobilized anti-CD3 and of the decreased F-actin content of T cells with R86H mutation in WASP after incubation with bortezomib for 10 h, a time at which cell viability was intact (SI Fig. 9). It is possible that the WASP levels achieved may have not been sufficient to correct these defects. Alternatively, the ability of WASP to correct these defects may depended on its capacity to interact and function with WIP as a complex. A genetic approach in which a minimal WIP fragment that is sufficient to normalize WASP level is expressed in WIP−/− T cells is needed to distinguish between these possibilities.
Experimental Procedures
Cells, Mice, Reagents, and Antibodies.
Jurkat E6–1 T cells were obtained from American Type Culture Collection (Manassas, VA). Generation and characterization of WIP−/− and WASP−/− mice have been reported previously (8, 13). Mouse thymocytes were purified as described previously (13). Blood was obtained from WAS patients and controls with informed consent, and T cells were purified from peripheral blood mononuclear cells by using a human Magcellect Cell isolation kit (R & D Systems, Minneapolis, MN). T cell lines were derived by stimulation with PHA (10 μg/ml) for 3 days, and after 3 days the cells were expanded by addition of 100 units/ml human rIL-2 (R & D Systems). EBV-transformed B cell lines were established by using a standard protocol. Cells were cultured in RPMI medium 1640 with 10% FCS. WIP−/− fibroblasts derived from lung were cultured in Iscove's modified Dulbecco's medium with 10% FCS (13).
MG132, calpain I, calpeptin, caspase inhibitor Z-VAD-FMK, and human calpastatin exon 1B encoded peptide (CS peptide) were purchased from Calbiochem (San Diego, CA). Bortezomib (Velcade) was obtained from Millennium Pharmaceuticals (Cambridge, MA). FuGene transfection agent was from Roche (Indianapolis, IN). Generation of rabbit anti-WIP antiserum C14, anti-WASP antiserum K-374, and anti-WASP mAb 5A5 was described previously (5, 6, 37). Anti-WASP mAb B-9, anti-Vav, anti-CrkL mAb, and rabbit anti-NWASP antibody (H-100) were from Santa Cruz Biotechnology (Santa Cruz, CA). Anti-actin mAb was purchased from Chemicon (Temecula, CA). Anti-Vav1 was purchased from Abcam (Cambridge, MA). Anti-c-myc mAb 9E10 was from the Developmental Studies Hybridoma Bank at the University of Iowa (Iowa City, IA). Anti-WIP mAb 3D10 was raised against the N terminus of WIP. Calpain inhibition was quantified by using a calpain assay kit (Biovision, Mountain View, CA) as suggested by the manufacturer except that the cell lysates were incubated with the substrate overnight, protected from light.
Transfection of T Cells with WIP–GFP and GFP–WASP Constructs.
WT WIP and WIP Δ-461–488 were cloned in phase with GFP at the C terminus of WIP in the expression vector pCDNA3. Five micrograms of each construct was used to transfect 1 × 107 T cells by using a mouse T cell nucleofector kit and an Amaxa Biosystem nucleofector. The program X-01 was used, and optimal expression of the fusion proteins was observed 18 h after transfection.
For the analysis of WASP expression level, transfected cells were permeabilized and stained with mAb 5A5 or mAb B-9 by using goat anti-mouse phycoerythrin (BD Biosciences, San Jose, CA) as the second antibody. WASP fluorescence in gated GFP+ cells was analyzed by FACS. In parallel, 1 × 107 transfected cells were lysed in lysis buffer [50 mM Tris, pH 7.4/100 mM NaCl/1% Triton X-100/protease inhibitors (Complete, Roche)], immunoprecipitated with anti-GFP polyclonal antibody (BD Biosciences), Western blotted, and probed with anti-WASP mAb B-9. The blots were stripped and reprobed with anti-GFP mAb (BD Biosciences).
Stable Transfection and Inducible Expression of WIP.
Human N-terminal WIP (1–400) and C-terminal WIP (401–503) were cloned in frame into pTRE2hyg2-myc vector (Clontech, Mountain View, CA) and sequence-verified. A total of 10 μg of plasmids was transfected by electroporation into 1 × 107 Jurkat cell clones stably transfected with pTet-on vector (Clontech) by using a Bio-Rad Genepulser II set at 250 V and 975 μF. Cells were selected with 150 μg/ml hygromycin B (BD Biosciences) and 300 μg/ml G418 (Calbiochem) for 21 days. Selected cells were subjected to limiting dilution, each clone was cultured in the absence or presence of 2 μg/ml DOX (Clontech) for 48 h, and the cell lysates were analyzed by immunoblotting.
Cell Stimulation, Immunoprecipitation, Immunoblotting, and Northern Blotting.
Cells were lysed in ice-cold lysis buffer containing 1% Triton X-100, and lysates were clarified at 16,000 × g for 15 min at 4°C and precleared for 1 h with protein G-Sepharose (Amersham Pharmacia Biotech, Fairfield, CT). Immunoprecipitation was performed overnight at 4°C with the indicated antibody (4 μg) or antiserum (4 μl) preadsorbed onto protein G-Sepharose. Beads were washed five times with modified lysis buffer containing 0.2% Triton X-100. Bound proteins were eluted, run on SDS/PAGE 4–15% gradient gels, and analyzed by Western blotting with indicated antibodies followed by goat anti-mouse or anti-rabbit antibodies conjugated to HRP and ECL detection (PerkinElmer, Boston, MA) as previously described (5). Densitometry was performed by using NIH Image version 1.63 software. WASP:Vav1 ratios were calculated and normalized to the ratio of medium-treated cells, which was given a value of 1. Northern blotting and probing for murine WASP mRNA were performed as described previously (8).
Protein Preparation and in Vitro Calpain Cleavage.
Linearized human WASP cDNA (1 μg) was transcribed and translated in vitro with 50 μl of TNT-coupled reticulocyte lysate system (Promega, Madison, WI). GST-CrkL, GST-C-WIP (401–503), and GST protein were purified as described previously (5). In vitro translated WASP or GST-CrkL (≈25 ng) was preincubated with 40 ng of GST-WIP or GST alone for 1 h on ice. In vitro cleavage assay with calpain was performed by incubating WASP at the indicated concentrations of calpain I in reaction buffer containing 100 mM imidazole (pH 7.4), 10 mM 2-mercaptoethanol, and 5 mM CaCl2 with or without 100 μM calpeptin or 5 mM EDTA for 10 min at 30°C. Cleavage assay with caspase-3 was performed by incubating the same substrates at the indicated concentrations of caspase-3 in a reaction buffer containing 50 mM Hepes (pH 7.4), 100 mM NaCl, 0.1% CHAPS, 10% glycerol, and 10 mM DTT with or without 50 μM Z-DEVD-FMK for 30 min at 30°C.
Acknowledgments
This article is dedicated to the memory of our colleague Dr. Fred S. Rosen. We thank M. Byrne and C. Wilbur for technical help, the WAS patient for donating blood samples, and Drs. Michel Massaad and Severine Lebras for thoughtful comments. This work was supported by U.S. Public Health Service Grants HL59561, AI35714, and HD17427; U.S. Immunodeficiency Network Grant N01-AI-30070; and the March of Dimes. Y.S. is the recipient of an Immune Deficiency Foundation Fellowship.
Abbreviations
TCR | T cell receptor |
WAS | Wiskott–Aldrich syndrome |
WASP | WAS protein |
WIP | WASP-interacting protein |
DOX | doxycycline. |
Footnotes
The authors declare no conflict of interest.
This article contains supporting information online at www.pnas.org/cgi/content/full/0610275104/DC1.
References
Articles from Proceedings of the National Academy of Sciences of the United States of America are provided here courtesy of National Academy of Sciences
Full text links
Read article at publisher's site: https://doi.org/10.1073/pnas.0610275104
Read article for free, from open access legal sources, via Unpaywall:
https://www.pnas.org/doi/pdf/10.1073/pnas.0610275104
Citations & impact
Impact metrics
Citations of article over time
Article citations
High aggressiveness of papillary thyroid cancer: from clinical evidence to regulatory cellular networks.
Cell Death Discov, 10(1):378, 26 Aug 2024
Cited by: 0 articles | PMID: 39187514 | PMCID: PMC11347646
Review Free full text in Europe PMC
Wiskott-Aldrich syndrome protein interacts and inhibits diacylglycerol kinase alpha promoting IL-2 induction.
Front Immunol, 14:1043603, 17 Apr 2023
Cited by: 3 articles | PMID: 37138877 | PMCID: PMC10149931
Not too little, not too much: the impact of mutation types in Wiskott-Aldrich syndrome and RAC2 patients.
Clin Exp Immunol, 212(2):137-146, 01 Apr 2023
Cited by: 7 articles | PMID: 36617178
Review
Dendritic cells in inborn errors of immunity.
Front Immunol, 14:1080129, 23 Jan 2023
Cited by: 1 article | PMID: 36756122 | PMCID: PMC9899832
Review Free full text in Europe PMC
Primary Immunodeficiencies Associated With Early-Onset Inflammatory Bowel Disease in Southeast and East Asia.
Front Immunol, 12:786538, 13 Jan 2022
Cited by: 4 articles | PMID: 35095863 | PMCID: PMC8792847
Review Free full text in Europe PMC
Go to all (100) article citations
Data
Data behind the article
This data has been text mined from the article, or deposited into data resources.
BioStudies: supplemental material and supporting data
Similar Articles
To arrive at the top five similar articles we use a word-weighted algorithm to compare words from the Title and Abstract of each citation.
A peptide derived from the Wiskott-Aldrich syndrome (WAS) protein-interacting protein (WIP) restores WAS protein level and actin cytoskeleton reorganization in lymphocytes from patients with WAS mutations that disrupt WIP binding.
J Allergy Clin Immunol, 127(4):998-1005.e1-2, 03 Mar 2011
Cited by: 16 articles | PMID: 21376381 | PMCID: PMC3077682
T-cell receptor ligation causes Wiskott-Aldrich syndrome protein degradation and F-actin assembly downregulation.
J Allergy Clin Immunol, 132(3):648-655.e1, 16 May 2013
Cited by: 24 articles | PMID: 23684068
WIP, a protein associated with wiskott-aldrich syndrome protein, induces actin polymerization and redistribution in lymphoid cells.
Proc Natl Acad Sci U S A, 94(26):14671-14676, 01 Dec 1997
Cited by: 234 articles | PMID: 9405671 | PMCID: PMC25088
WASP-WIP complex in the molecular pathogenesis of Wiskott-Aldrich syndrome.
Pediatr Int, 58(1):4-7, 05 Dec 2015
Cited by: 8 articles | PMID: 26331277
Review
Funding
Funders who supported this work.
NHLBI NIH HHS (2)
Grant ID: P01 HL059561
Grant ID: HL 59561
NIAID NIH HHS (4)
Grant ID: N01 AI 30070
Grant ID: N01AI30070
Grant ID: AI 35714
Grant ID: P01 AI035714
NICHD NIH HHS (2)
Grant ID: HD 17427
Grant ID: R37 HD017427