Abstract
Free full text

Type 1 pilus-mediated bacterial invasion of bladder epithelial cells
Abstract
Most strains of uropathogenic Escherichia coli (UPEC) encode filamentous adhesive organelles called type 1 pili. We have determined that the type 1 pilus adhesin, FimH, mediates not only bacterial adherence, but also invasion of human bladder epithelial cells. In contrast, adherence mediated by another pilus adhesin, PapG, did not initiate bacterial internalization. FimH-mediated invasion required localized host actin reorganization, phosphoinositide 3-kinase (PI 3-kinase) activation and host protein tyrosine phosphorylation, but not activation of Src-family tyrosine kinases. Phosphorylation of focal adhesin kinase (FAK) at Tyr397 and the formation of complexes between FAK and PI 3-kinase and between α-actinin and vinculin were found to correlate with type 1 pilus-mediated bacterial invasion. Inhibitors that prevented bacterial invasion also blocked the formation of these complexes. Our results demonstrate that UPEC strains are not strictly extracellular pathogens and that the type 1 pilus adhesin FimH can directly trigger host cell signaling cascades that lead to bacterial internalization.
Introduction
Urinary tract infections (UTIs) account for an estimated 6–7 × 106 out-patient hospital visits per year and are associated with considerable morbidity (Barnett and Stevens, 1997; Hooton and Stamm, 1997). Escherichia coli is the etiological agent in >80% of all UTIs. The vast majority of E.coli UTI isolates encode 1–2 µm long filamentous surface-adhesive organelles called type 1 pili (Langermann et al., 1997). These organelles are composite fibers consisting of a 7 nm thick helical rod made up of repeating FimA subunits joined to a 3 nm wide distal tip fibrillum structure containing two adaptor proteins, FimF and FimG, and the adhesin, FimH (Russell and Orndorff, 1992; Jones et al., 1995). FimH binds mannose-containing glycoprotein receptors and can mediate bacterial attachment to a variety of different host cell types (Tewari et al., 1993; Baorto et al., 1997; Malaviya and Abraham, 1998; Mulvey et al., 1998). Interactions between FimH and receptors expressed on the lumenal surface of the bladder epithelium appear to be critical to the ability of many uropathogenic E.coli (UPEC) strains to colonize the bladder and cause disease (Connell et al., 1996; Langermann et al., 1997; Thankavel et al., 1997; Mulvey et al., 1998).
Subsequent to attachment, it is generally considered that UPEC strains exist as extracellular pathogens within the urinary tract. More than a decade ago, however, transmission electron microscopy (TEM) studies of infected rat and mouse bladders indicated that bladder epithelial cells could internalize UPEC in vivo (Fukushi et al., 1979; McTaggart et al., 1990). Bacteria were observed within membrane-bound vacuoles and free within the cytoplasm of the superficial epithelial cells that line the lumenal surface of the bladder. It was proposed that the bladder epithelial cells internalized bacteria as part of an innate host defense mechanism. A more recent study, however, suggested that bacterial internalization by bladder epithelial cells could benefit the pathogens (Mulvey et al., 1998). Using a murine cystitis model, it was shown that a subpopulation of type 1-piliated E.coli inoculated into the bladder could invade the epithelium and that these bacteria appeared to have a distinct survival advantage over their extracellular counterparts.
In general, invasion of host cells by pathogenic bacteria can allow pathogens to evade host defenses and facilitate their dissemination both within and across cellular barriers (Sansonetti, 1991; Falkow et al., 1992; Menard et al., 1996). Invasion of bladder epithelial cells by UPEC may allow these bacteria to avoid the bulk flow of urine through the bladder and may give them access to a more nutrient-rich environment. Intracellular uropathogens may also be better able to avoid the diverse array of antimicrobial substances and immune cells present within the urine and the bladder tissue. In addition, studies using murine cystitis models have suggested that intracellular UPEC strains can persist within mouse bladders virtually undeterred in the face of antibiotic treatments that effectively reduce bacterial titers within the urine (Mulvey et al., 1998, 2000; Hvidberg et al., 2000). Recently, we have found that UPEC strains can persist at steady-state levels for multiple days within host bladder epithelial cells, but the bacteria also have the capacity to proliferate to high levels intracellularly (M.A.Mulvey, J.D.Schilling and S.J.Hultgren, in preparation). These data argue that the ability of UPEC to invade bladder epithelial cells has a critical role in the pathogenesis of UTIs.
A mechanism by which UPEC can invade bladder epithelial cells has not been described. Scanning EM (SEM) studies of infected mouse bladders have revealed that type 1-piliated E.coli strains, but not isogenic mutants lacking the FimH adhesin, are able to induce localized changes in the host cell surface at points of contact with murine bladder epithelial cells (Mulvey et al., 1998). In addition, high-resolution EM has suggested that mouse bladder epithelial cells can envelop adherent bacteria, seemingly through contacts with the adhesive, FimH-containing tips of type 1 pili. These observations suggested that type 1 pilus-mediated attachment might have a role in the invasion of bladder cells by UPEC.
Various studies have demonstrated that bacterial attachment can lead to the activation of host signal transduction cascades, sometimes inducing dramatic rearrangements of the eukaryotic cytoskeleton that can result in the internalization of adherent bacteria (Finlay and Falkow, 1997). Following attachment, some invasive bacteria can introduce effector molecules into their target host cells, triggering intense ruffling of the host cell membrane that results in bacterial uptake (Finlay and Cossart, 1997). In other cases, the host cell membrane can zipper around and envelope adherent bacteria in response to direct, sequential interactions between host cell membrane receptors and specific bacterial adhesins, which are sometimes referred to as invasins. The invasin protein encoded by Yersinia pseudotuberculosis (Isberg et al., 1987) and internalin A expressed by Listeria monocytogenes (Mengaud et al., 1996) are two prototypic invasins. Here, we present data indicating that the type 1 pilus adhesin FimH can function as an invasin, directly mediating bacterial invasion of bladder epithelial cells by inducing localized rearrangements of the host cell cytoskeleton. We also show that FimH-mediated bacterial invasion requires the activation of distinct host cell signaling events.
Results
Type 1 pili mediate invasion into bladder epithelial cells
The ability of type 1-piliated E.coli strains to invade cultured human bladder epithelial cells (5637 cells) was examined by EM techniques. SEM of 5637 cells fixed 1 h after infection with a type 1-piliated clinical cystitis isolate, NU14, revealed that the host cell membrane could zipper around attached bacteria (Figure 1A). Like NU14, the recombinant laboratory K-12 strain AAEC185/pSH2 (type 1+) also adhered to 5637 cells and could be enveloped similarly by the host cell membrane (data not shown). The envelopment of NU14 and AAEC185/pSH2 by 5637 cells in vitro was morphologically similar to the envelopment of type 1-piliated E.coli by murine bladder cells in in vivo experiments (Mulvey et al., 1998). The uptake of NU14 and AAEC185/pSH2 by 5637 cells was confirmed by TEM studies (Figure 1B and C). Internalized bacteria were found predominantly within membrane-bound vacuoles. In contrast to NU14 and AAEC185/pSH2, the fimH– isogenic mutants, NU14-1 and AAEC185/pUT2002 (type 1+, FimH–) did not bind to, and were not enveloped by, 5637 cells as determined by EM and adherence assays (Figure 2C; data not shown).
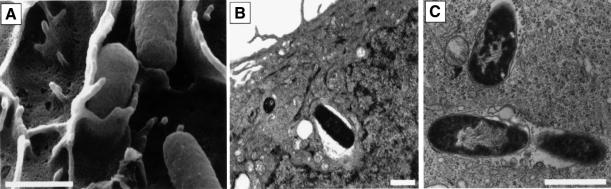
Fig. 1. Invasion of bladder epithelial cells by type 1-piliated E.coli. 5637 bladder cells were examined by (A) SEM or (B and C) TEM 1–2 h after infection with NU14. Adherent bacteria were often observed being enveloped by bladder epithelial cells by SEM and could be detected within membrane-bound vacuoles by TEM. The scale bars indicate 1 µm.
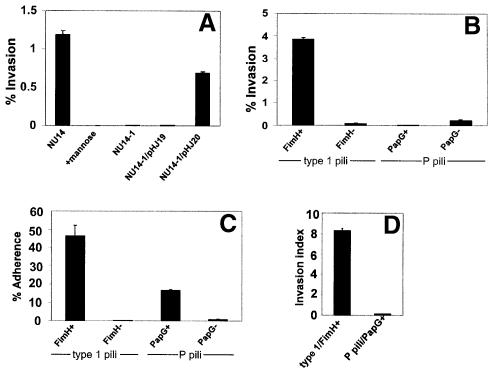
Fig. 2. Type 1 pili mediate bacterial invasion of bladder cells. (A) Gentamicin protection assays indicate that NU14 can invade 5637 cells, while the isogenic fimH– mutant, NU14-1, was non-invasive. Addition of 2.5% d-mannose to the cell culture medium inhibited NU14 invasion. The plasmid pHJ20 (encoding FimH), but not pHJ19 (encoding FimH in the incorrect orientation), complements the invasion defect of NU14-1. (B) The non-invasive K-12 strain AAEC185 (type 1–), when transformed with the plasmid pSH2 (type 1+, FimH+), could also invade 5637 cells. AAEC185 strains transformed with pUT2002 (type 1+, FimH–) or pPIL110-35 (P pili+, with or without the PapG adhesin) were non-invasive. (C) Adherence assays indicate that AAEC185 expressing either wild-type type 1 pili (fimH+) or wild-type P pili (papG+) are able to adhere well to 5637 cells (46.2 ± 5.9% versus 16.5 ± 0.6%, respectively) in comparison with strains expressing type 1 or P pili lacking the FimH and PapG adhesins, respectively. (D) Calculated invasion indices (c.f.u. invaded/c.f.u. adherent) demonstrate that AAEC185 type 1 pili (FimH+) are ~98% more efficient at mediating bacterial invasion than bacteria expressing P pili (PapG+). All data are representative of three or more independent assays, each performed in triplicate. Between experiments, the invasion frequencies of the type 1-piliated E.coli strains varied from 0.5 to 10%. This variability appears to be related to the passage number and status of the host cells and to the degree of bacterial piliation on the day of the experiment.
The role of type 1 pili, and specifically the FimH adhesin, in mediating bacterial internalization by 5637 cells was investigated further using standard gentamicin protection assays (Elsinghorst, 1994; see Materials and methods). NU14 was found to invade 5637 cells via a mannose-inhibitable mechanism, while the isogenic fimH-negative mutant of NU14, NU14-1, was non-invasive (Figure 2A). Expression of fimH in trans within NU14-1 from plasmid pHJ20 restored the invasive phenotype. In contrast, the plasmid pHJ19, having fimH cloned in the incorrect orientation, was unable to complement the invasion defect of NU14-1. Several other type 1-piliated clinical E.coli cystitis isolates and the recombinant K-12 strain AAEC185/pSH2 (type 1+) were also tested for their ability to invade 5637 bladder cells. Like NU14, all type 1-piliated E.coli strains tested were able to invade 5637 cells (Figure 2B and data not shown). In contrast, the fimH-negative mutant AAEC185/pUT2002 (type 1+, fimH–) was non-invasive, as was the parent strain AAEC185, which has a complete deletion in the type 1 pilus gene cluster (Figure 2B and data not shown) (Blomfield, 1991). Invasion of 5637 cells by NU14 and other type 1-piliated strains occurred at levels that are comparable to the internalization frequencies of established invasive pathogens such as Listeria and Shigella (Braun et al., 1998; Mecsas et al., 1998). NU14 and AAEC185/pSH2 were also able to invade effectively other cultured human bladder epithelial cells, including T24, RT4 and J82 cells (data not shown).
To test whether or not the observed internalization of type 1-piliated bacteria was the result of simply adhering to the host cells, we examined the ability of E.coli expressing P pili to adhere to and invade 5637 cells. P pili are encoded by the Pap (pyelonephritis-associated pili) gene cluster and mediate bacterial adhesion to Galα(1–4)Gal-containing host receptors through the P pilus-associated adhesin PapG (Leffler and Svanborg-Eden, 1980). AAEC185 was transformed with pPIL110-35 plasmids encoding the Pap gene cluster with or without papG (Stromberg et al., 1990). As shown in Figure 2B, AAEC185/pPIL110-35 (PapG+) was non-invasive even though the strain is able to adhere well to 5637 cells (Figure 2C). The papG– isogenic mutant was non-adherent and non-invasive. Comparison of the invasion indices (no. of c.f.u. invaded/no. of c.f.u. adhered) for type 1-piliated versus P-piliated AAEC185 indicated that adherence to 5637 cells in the absence of the FimH adhesin is not sufficient to trigger bacterial internalization into bladder epithelial cells (Figure 2D).
FimH is sufficient to mediate bacterial internalization
The ability of FimH to mediate directly internalization into bladder epithelial cells was tested using adhesin-coated polystyrene latex beads. Similar amounts of purified FimC–FimH chaperone–adhesin complexes, FimC alone or bovine serum albumin (BSA) were covalently attached to latex beads (~1.17 µm in diameter). FimH, when bound to the FimC chaperone, can be purified easily in a native state that is able to bind mannose-containing host receptors (Choudhury et al., 1999). The ability of the protein-coated beads to be internalized by 5637 cells was examined by SEM and TEM. Following a 1 h incubation with FimCH-coated beads, SEM revealed numerous beads just beneath the bladder cell surface and in the process of internalization (Figure 3A and B). TEM indicated that the bladder cell membrane could ‘zipper’ around and eventually internalize attached FimCH-coated beads (Figure 3D–G). This zippering process appeared to be morphologically identical to that leading to the internalization of type 1-piliated E.coli (see Figure 1A; Mulvey et al., 1998). In contrast, BSA-coated beads and FimC-coated beads rarely adhered to the 5637 bladder cells and did not induce any apparent changes in the host cell membrane (Figure 3C and data not shown).
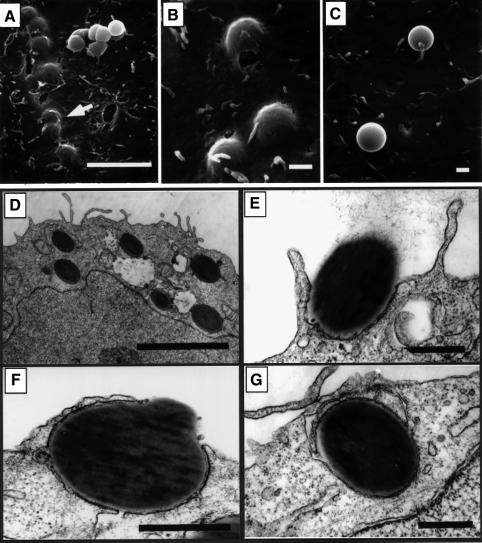
Fig. 3. Internalization of FimCH-coated beads. 5637 cells were incubated with FimCH- or BSA-coated beads for 30–60 min and then processed for SEM (A–C) or TEM (D–G). (A and B) FimCH-coated beads were internalized efficiently by 5637 cells, while the few adherent BSA-coated beads (C) did not induce any appreciable alterations in the 5637 cell membrane. (D–G) The host cell membrane appears to zipper around and eventually envelope FimCH-coated beads during the internalization process. Scale bars represent 5.0 µm (A and E), 0.5 µm (B–D) and 1.0 µm (F and G).
The ability of FimCH-coated beads to be internalized by 5637 cells was quantified using an immunofluorescence-based assay (see Materials and methods). As shown in Figure 4, significant numbers of adherent FimCH-coated beads were internalized into 5637 cells within 60 min after addition to the cell culture wells. In contrast, the few BSA- and FimC-coated beads that occasionally adhered to 5637 cells were rarely internalized (Figure 4A). Like type 1-piliated bacteria, internalization of the FimCH-coated beads was inhibited by soluble d-mannose. However, d-mannose had no effect on the number of BSA- or FimC-coated beads that were internalized (Figure 4A). These results indicate that the type 1 pilus adhesin, FimH, cannot only mediate adherence to host cells, but is also sufficient to trigger internalization into human bladder cells in the absence of other bacterial factors.

Fig. 4. FimH is necessary and seemingly sufficient to mediate internalization. (A) 5637 cells, grown on sterile coverslips, were incubated with equal amounts of FimCH-, FimC- or BSA-coated beads ±2.5% d-mannose for 30–60 min. FimCH-coated beads were internalized in a d-mannose-inhibitable manner. In contrast, few control FimC- or BSA-coated beads were internalized and their uptake was not inhibited by d-mannose. Numbers of intracellular beads were determined as described in Materials and methods. Data are presented as an invasion index (total number of internalized beads divided by the total number of host cell-associated beads) and are representative of at least four independent assays. (B and C) Representative images of the internalization assay demonstrating the internalization of FimCH-coated beads into 5637 cells. (C) Fluorescent images (TRITC filter setting) were superimposed onto the corresponding phase contrast images such that extracellular beads appear red (arrow). The scale bar represents 5 µm.
FimH-mediated invasion is dependent upon host actin polymerization
The envelopment of type 1-piliated bacteria and FimCH-coated beads by 5637 cells appeared to involve substantial host cytoskeletal alterations, probably requiring actin rearrangements at sites of FimH-mediated contact with host cells. The role of actin in the invasion process was investigated using a specific inhibitor of F-actin polymerization, cytochalasin D. 5637 bladder cells were incubated with increasing concentrations of cytochalasin D for 30 min prior to infection with either NU14 or AAEC185/pSH2 (type 1+, fimH+). Cytochalasin D dramatically reduced FimH-mediated invasion (>99% inhibition; Figure 5A) but had no effect on bacterial adherence to the bladder cells (data not shown). Removal of the drug prior to infection made the host cells susceptible once again to type 1 pilus-mediated bacterial invasion, indicating that the effects of cytochalasin D were reversible (Figure 5A). The treatment of 5637 cells with cytochalasin D also inhibited the internalization of FimCH-coated beads (~90% reduction; Figure 5B).
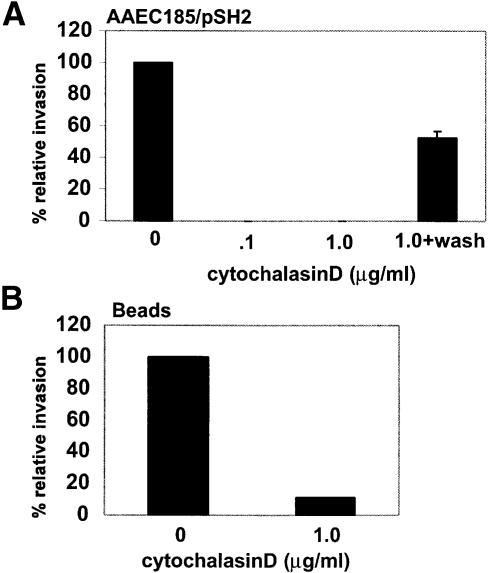
Fig. 5. Host cell actin polymerization plays an important role in the invasion process. (A) 5637 cells were pre-treated with cytochalasin D for 30 min prior to infection with AAEC185/pSH2. Gentamicin protection assays demonstrate that cytochalasin D (1 µg/ml) can completely abolish invasion. The effects of cytochalasin D are reversible if the host cells are washed with PBS prior to infection in fresh medium without drug. Similar results were obtained using NU14. (B) Cytochalasin D also inhibits the internalization of FimCH-coated beads.
The effects of FimH-mediated adherence on the host cell actin cytoskeletal network were visualized using 5637 cells that were infected with green fluorescent protein (GFP)-expressing AAEC185/pSH2 or NU14. Following infection, 5637 cells were stained with Texas red-labeled phalloidin, a toxin that specifically binds to host F-actin. Confocal microscopy revealed that type 1-piliated bacteria (green) induced localized rearrangement of actin (red) at points of bacterial attachment (arrows, Figure 6B). Such localized alterations of the actin cytoskeleton were not observed in uninfected cells (Figure 6A). Like type 1-piliated E.coli, FimCH-coated beads, but not control BSA-coated beads, also induced localized changes of the actin cytoskeleton (data not shown). No large-scale, global changes, such as the formation of large actin stress fibers, were induced by FimH-mediated bacterial or bead attachment. In addition, type 1-piliated bacteria lacking the FimH adhesin did not bind 5637 cells and failed to induce any cytoskeletal changes (data not shown). These data suggest that FimH-mediated attachment is sufficient to induce localized actin polymerization.
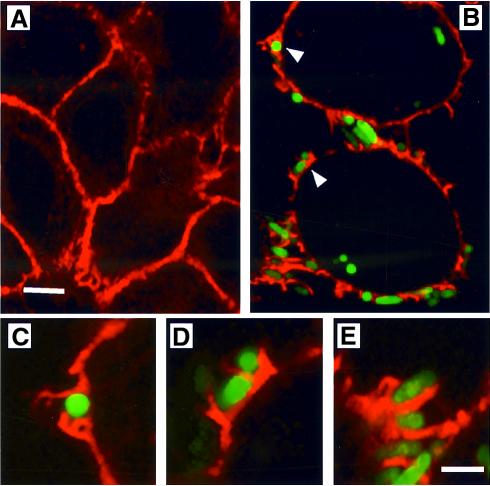
Fig. 6. Actin rearrangements leading to internalization of type 1-piliated E.coli. (A) Texas red–phalloidin staining of uninfected 5637 cells highlighting cortical actin (red). (B) Localized actin polymerization is induced at points of contact (arrows) with AAEC185/pSH2/pcomGFP (type 1+, GFP+). (C and D) Images from (B) (arrows) and (E) are enlarged to show detail. The scale bar represents 2 µm.
Invasion requires host protein tyrosine phosphorylation and PI 3-kinase activation
Modification of the host actin cytoskeletal network can be influenced directly by the induction of specific host signaling events, including protein tyrosine phosphorylation and the activation of phosphoinositide 3-kinase (PI 3-kinase) (Guinebault et al., 1995; Hartwig et al., 1995; Carpenter and Cantley, 1996; Parsons, 1996). The involvement of host protein tyrosine phosphorylation in FimH-mediated bacterial invasion was tested using a potent general tyrosine kinase inhibitor, genistein, and a specific inhibitor of Src-family tyrosine kinases, PP1 (Gerwien et al., 1999). Treatment with genistein greatly inhibited the ability of both NU14 (Figure 7A) and AAEC185/pSH2 (data not shown) to invade 5637 bladder cells in a concentration-dependent fashion. In contrast, the inhibition of Src-family tyrosine kinases using PP1 had no effect on the ability of type 1-piliated E.coli to bind to and subsequently invade 5637 cells (Figure 7B). These data indicate that tyrosine kinases, other than members of the Src-family of kinases, are involved in FimH-mediated bacterial invasion.

Fig. 7. Inhibition of host protein tyrosine phosphorylation and PI 3-kinase activation diminishes invasion. (A) Gentamicin protection assays show that genistein prevents FimH-mediated bacterial internalization into 5637 cells in a concentration-dependent manner (>90% inhibition at 250 µM). (B) PP1, a Src-family tyrosine kinase inhibitor, had no effect on the internalization process. 5637 cells were treated with PP1 at concentrations that are known to inhibit Src-family kinase activity effectively (Gerwein et al., 1999). (C and D) Wortmannin and LY294002, two compounds that inhibit PI 3-kinase activity, can block FimH-mediated bacterial invasion of 5637 cells (~90% inhibition at 100 nM and 50 µM, respectively). (E) Internalization of FimCH-coated beads into 5637 cells is also inhibited by wortmannin (~80% inhibition at 100 nM).
The role of PI 3-kinase in FimH-mediated bacterial invasion was investigated using two structurally unrelated compounds, wortmannin and LY294002, both of which inhibit PI 3-kinase activity (Vlahos et al., 1994). Treatment of 5637 cells with either 100 nM wortmannin or 50 µM LY294002 reduced FimH-mediated invasion of 5637 cells by ~90% when compared with the control cells treated with solvent (dimethylsulfoxide; DMSO) alone (Figure 7C and D). Wortmannin also greatly inhibited the internalization of FimCH-coated beads by 5637 cells (Figure 7E). Neither wortmannin nor LY294002 had any effect on FimH-mediated bacterial adherence (data not shown).
Induced formation of signaling and cytoskeletal complexes
Previous work has shown that the p85α subunit of PI 3-kinase can interact with activated protein tyrosine kinases (Carpenter et al., 1990), including focal adhesin kinase (FAK) (Chen and Guan, 1994; Parsons, 1996). These interactions can lead eventually to local changes in the host actin cytoskeleton. Interactions between PI 3-kinase and FAK are facilitated by the phosphorylation of FAK at Tyr397 (Chen and Guan, 1996; Chen et al., 1996). Kinetic studies using a phospho-specific polyclonal anti-FAK antibody demonstrated that FAK becomes phosphorylated at Y397 within 5637 bladder cells shortly after infection with AAEC185/pSH2 (type 1+) (Figure 8A). Phosphorylation of FAK at Y397 correlated with the transient formation of complexes between FAK and PI 3-kinase, seen within 15 min after infection with AAEC185/pSH2 (Figure 8B). Induced phosphorylation of FAK at Y397 and the formation of complexes between FAK and PI 3-kinase were not detected in host cells after infection with the FimH– mutant AAEC185/pUT2002 (Figure 8A and B). Inhibitors that block FimH-mediated bacterial invasion (cytochalasin D, genistein, wortmannin and LY294002; Figure 7) were also found to inhibit the formation of complexes between FAK and PI 3-kinase (Figure 8C). The Src-family kinase inhibitor, PP1, which had no effect on FimH-mediated bacterial invasion, also had no effect on complex formation. These results indicate that phosphorylation of FAK at Y397, and the subsequent formation of complexes between FAK and PI 3-kinase, may be critical events in the signal transduction cascades that result in localized host actin cytoskeletal rearrangements and internalization of type 1-piliated E.coli.
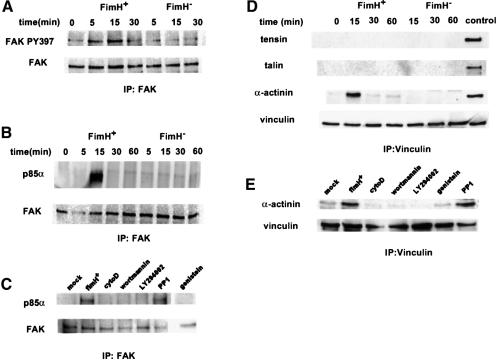
Fig. 8. Type 1-piliated E.coli induce complex formation between FAK and PI 3-kinase and between vinculin and α-actinin. (A) Shortly after infection of 5637 cells with AAEC185/pSH2 (FimH+), blots probed with a phospho-specific anti-FAK antibody show that FAK becomes phosphorylated at Y397. Infection with AAEC185/pUT2002 (FimH–) did not induce phosphorylation of FAK Y397. (B) PI 3-kinase (the p85α subunit) co-precipitates with FAK within 15 min after infection with AAEC185/pSH2, but not after infection with AAEC185/pUT2002. (D) α-actinin, but not tensin or talin, transiently co-precipitates with vinculin beginning within 15 min after infection with AAEC185/pSH2, but not after infection with AAEC185/pUT2002. Control lysates containing tensin, talin and α-actinin were used to ensure that the different antibodies were functional in these assays. (C and E) Inhibitors of type 1 pilus-mediated bacterial invasion block complex formation between FAK and PI 3-kinase and between vinculin and α-actinin. The Src-family kinase inhibitor, PP1, had no effect on complex formation.
PI 3-kinase and FAK can potentially modulate, directly or indirectly, a number of other molecules, including vinculin, tensin, talin and α-actinin, which can function in the reorganization and stabilization of the host actin cytoskeletal network (Parsons, 1996; Dekker and Segal, 2000). Earlier work has shown that α-actinin can function as a link between cross-linked actin filaments (Kuhlman et al., 1992) and vinculin (Kroemker et al., 1994), and it has been proposed that these interactions may serve to stabilize the cytoskeletal network. Co-immunoprecipitation experiments demonstrated that infection of 5637 cells with AAEC185/pSH2, but not with AAEC185/pUT2002, could stimulate the formation of transient complexes between vinculin and α-actinin (Figure 8D). However, infection with AAEC185/pSH2 did not appear to induce complex formation between α-actinin and either tensin or talin. Compounds that inhibit FimH-mediated bacterial invasion and the formation of complexes between FAK and PI 3-kinase (Figures 7 and and8C)8C) also inhibited complex formation between vinculin and α-actinin, while PP1 had no effect (Figure 8E). These correlative data indicate that the formation of transient complexes between vinculin and α-actinin, like the formation of FAK–PI 3-kinase complexes, may be critical events leading to the internalization of type 1-piliated bacteria.
Discussion
Emerging data indicate that the ability of type 1-piliated UPEC strains to invade bladder epithelial cells is a key event in the establishment and persistence of a UTI (Mulvey et al., 1998; M.A.Mulvey, J.D.Schilling, S.Langermann and S.J.Hultgren, in preparation). Here we have described the ability of the type 1 pilus-associated adhesin, FimH, to mediate the invasion of bladder epithelial cells by E.coli. FimH was necessary and sufficient to induce internalization of both bacteria and protein-coated latex beads, suggesting that FimH can function like an archetypal invasin. SEM and TEM studies indicated that FimH-mediated invasion proceeded via a zipper-like mechanism involving localized alterations of the host cell membrane. Invasion required reorganization of the actin cytoskeleton and was inhibited by the soluble FimH receptor analog, d-mannose. Non-specific adherence to bladder epithelial cells, or adherence mediated by the P pilus adhesin, PapG, did not result in bead or bacteria internalization. These data strongly implicate the type 1 pilus FimH adhesin as the critical factor involved in the uptake of UPEC by bladder epithelial cells. It has been reported that other adhesive pili may have accessory functions in the internalization of some bacterial pathogens (Weinberg et al., 1997; Comolli et al., 1999; Dibb-Fuller et al., 1999; Griffiss et al., 1999). However, this is the first demonstration that a pilus-associated adhesin, namely FimH, can directly mediate bacterial invasion of host cells.
To gain entry into host cells, most invasive bacterial pathogens exploit pre-existing host signal transduction cascades (Finlay et al., 1991; Falkow et al., 1992; Bliska et al., 1993; Finlay and Falkow, 1997). In many instances, signaling pathways involving tyrosine phosphorylation of host proteins appear to have key roles in the internalization of bacterial pathogens (Bliska et al., 1993; Palmer et al., 1997). A role for tyrosine phosphorylation in FimH-mediated bacterial invasion of bladder epithelial cells was indicated by our observation that invasion could be blocked by a general inhibitor of protein tyrosine kinases, genistein. One host protein potentially affected by genistein is FAK. This tyrosine kinase is an important modulator of host cytoskeletal components and has been shown to be involved in the invasion of non-phagocytic cells by Y.pseudotuberculosis (Alrutz and Isberg, 1998). Following infection of 5637 bladder epithelial cells by type 1-piliated E.coli, we have found that FAK is transiently phosphorylated at Y397, the major autophosphorylation site of FAK. Phosphorylation of FAK at Y397 creates a binding site for other proteins, including Src and PI 3-kinase (Cobb et al., 1994; Chen and Guan, 1996; Chen et al., 1996).
Both Src and PI 3-kinase have been implicated in the regulation of events involved in the alteration of the actin cytoskeleton (Hartwig et al., 1995; Carpenter and Cantley, 1996) and roles for both kinases in bacterial invasion of host cells have been reported (Ireton et al., 1996, 1999; Alrutz and Isberg, 1998; Dumenil et al., 1998; Evans et al., 1998;Schulte et al., 1998). We have found, however, that Src kinases do not appear to be involved in FimH-mediated E.coli invasion of 5637 bladder epithelial cells since PP1, an inhibitor of Src-family tyrosine kinases, had no effect on this process. In contrast, two structurally unrelated PI 3-kinase inhibitors, wortmannin and LY294002, significantly reduced the uptake of type 1-piliated E.coli by 5637 cells without affecting bacterial binding to the host cells. These data indicate that PI 3-kinase activity, but not the activity of Src-family kinases, can modulate FimH-mediated invasion.
PI 3-kinases catalyze the generation of the 3-phosphoinositides PI(3)P, PI(3,4)P2 and PI(3,4,5)P3 (PIP3) (Leevers et al., 1999). These lipids are important second messengers that can affect host cytoskeletal changes by binding to and altering the cellular localization, conformation and activity of a number of different eukaryotic proteins, including structural proteins, kinases, phospholipases, nucleotide exchange factors and adaptor proteins (Fukami et al., 1994; Vanhaesebroeck and Waterfield, 1999; Dekker and Segal, 2000). In addition, PI(3,4)P2 and PIP3 generated by PI 3-kinases can directly alter the actin cytoskeleton by uncapping barbed actin filaments (Hartwig et al., 1995).
Interactions with tyrosine-phosphorylated peptides and proteins, including FAK, can apparently stimulate PI 3-kinase activity (Shoelson et al., 1993). In response to infection by type 1-piliated, FimH+ E.coli, transient complexes between PI 3-kinase and FAK were detected within 5637 cells. The appearance of these complexes after infection paralleled the induced phosphorylation of FAK at Y397, suggesting that FAK phosphorylation was linked to PI 3-kinase–FAK complex formation in this situation. Complex formation between PI 3-kinase and FAK was blocked by inhibitors that prevented FimH-mediated bacterial invasion, arguing that this complex is an important signaling intermediate during the invasion process. It is conceivable that interactions between Y397-phosphorylated FAK and PI 3-kinase serve to boost PI 3-kinase activity, stimulating the generation of 3-phosphoinositide second messengers that could modulate the actin cytoskeletal rearrangements involved in FimH-mediated bacterial invasion.
The recruitment of cytoskeletal components, such as vinculin, α-actinin, tensin and talin, may help stabilize the actin rearrangements induced during bacterial invasion mediated by FimH. Concurrent with the induced phosphorylation of FAK and the formation of FAK–PI 3-kinase complexes following the infection of bladder epithelial cells with type 1-piliated E.coli, we detected transient interactions between vinculin and α-actinin. However, vinculin interactions with talin or tensin, two proteins that can link vinculin with cell membrane receptors such as integrin (Gilmore et al., 1992), were not detected. Inhibitor studies suggest that complexes between vinculin and α-actinin form downstream of protein tyrosine kinase and PI 3-kinase activation, but independently of Src-family kinase activities. These data suggest a model in which interactions between actin, vinculin, α-actinin and possibly other host components modulate and stabilize localized cytoskeletal changes that can lead to the envelopment and internalization of E.coli subsequent to FimH-mediated bacterial attachment. This work demonstrates that interactions between type 1-piliated UPEC strains and host bladder epithelial cells are more dynamic than previously thought, and may provide insight into the development of more efficacious therapies for the treatment and prevention of UTIs.
Materials and methods
Bacteria and cell lines
The E.coli cystitis isolate NU14 and the isogenic fimH– mutant NU14-1 have been described (Langermann et al., 1997). The plasmids pHJ19 or pHJ20 (Jones et al., 1995) were transformed into NU14-1 by electroporation. AAEC185, having a complete deletion of the type 1 pilus gene cluster (Blomfield et al., 1991), was transformed with pSH2 (type 1 gene cluster), pUT2002 (type 1+, fimH–) (Orndorff and Falkow, 1984; Minion et al., 1986), or with pPIL110-35 plasmids encoding the Pap gene cluster with or without papG (Stromberg et al., 1990). pcomGFP, which is comprised of the GFPmut3 gene in pcom100 (Cormack et al., 1996) and which constitutively expresses GFP, was kindly provided by J.St Geme. To induce expression of type 1 pili, bacterial strains were grown in 20 ml of static Luria–Bertani (LB) broth with appropriate antibiotics at 37°C for 48 h prior to use in experiments. Type 1 pilus expression was confirmed by mannose-sensitive agglutination of 1% baker’s yeast or of guinea pig erythrocytes (A640 ~1.9) (Colorado Serum Company) suspended in phosphate-buffered saline (PBS). P-pilus expression was induced by growing bacteria on tryptic soy agar plates (TSA) + 15 µg/ml tetracycline 24 h prior to use.
The human bladder epithelial cell lines 5637 (ATCC HTB-9) and J82 (ATCC HTB-1) were grown in RPMI 1640 (Sigma, St Louis, MO) supplemented with 10% fetal bovine serum (FBS; Sigma), 2.0 g/l sodium bicarbonate (Sigma) and 0.3 g/l l-glutamine. T24 cells (ATCC HTB-4) were grown in McCoy’s medium supplemented with 10% FBS and 20 mM l-glutamine. RT4 cells (ATCC HTB-2) were grown in RPMI 1640:Dulbecco’s modified Eagle’s medium (DMEM) (1:1) supplemented with 5% FBS. All cell lines were grown at 37°C with 5% CO2 and utilized between passages 10 and 24.
Adherence and gentamicin protection (invasion) assays
Adherence and invasion assays were performed essentially as described (Elsinghorst, 1994). Briefly, host epithelial cells were seeded into 24-well plates and grown to confluence. Just before infection, the cell culture medium in each well was replaced with 960 µl of fresh, pre-warmed medium. In three sets of triplicate wells, bladder cells were infected with a multiplicity of infection (m.o.i.) of 5–10 bacteria per host cell (20 µl of bacteria diluted in LB broth, A600 ~0.5). Bacterial contact with host cells was expedited by centrifugation of plates at 600 g for 5 min. After 2 h of incubation at 37°C, one set of triplicate wells was lysed by the addition of 20 µl of 5% Triton X-100. Bacteria present in these lysates, representing the total number of bacteria present both intra- and extracellularly, were titered. For adherence assays, a second set of wells was washed five times in PBS (containing Mg2+/Ca2+), lysed in 0.1% Triton X-100 in ddH2O, and then plated on LB-agar plates. Adherence frequencies were calculated as the number of bacteria recovered after PBS washes divided by the total number of bacteria present in each well. To determine invasion frequencies, after the initial 2 h incubation, an additional set of wells was washed twice with PBS (with Mg2+/Ca2+) and then incubated for another 2 h in medium containing 100 µg/ml membrane-impermeable bactericidal antibiotic gentamicin (Sigma) to kill any extracellular bacteria. Cells were then washed three times with PBS, lysed in 1 ml of 0.1% Triton X-100 in ddH2O, and plated on LB-agar plates. Invasion frequencies were calculated as the number of bacteria surviving incubation with gentamicin divided by the total number of bacteria present just before addition of gentamicin.
To test the effects of specific inhibitors on bacterial invasion, genistein (100 mM stock in DMSO) was added 15 min prior to addition of bacteria, while LY294002 (100 mM stock) and wortmannin (0.1 mM stock in DMSO) were added 10 min before infection. Cytochalasin D (0.1 or 1 µg/ml) was added 30 min prior to infection. As a control, DMSO was added to cells to a final concentration of 0.1%. None of the inhibitors or DMSO alone had any effect on bacterial attachment or host cell viability during the course of the experiments (data not shown).
Immunoprecipitations
Subconfluent monolayers of 5637 cells, grown in six-well plates, were infected with 100 µl of a bacterial suspension in PBS (OD600 ~1.0). Plates were centrifuged for 5 min at 600 g and then incubated at 37°C for 0, 5, 15, 30 and 60 min. Bacteria and inhibitors were added directly to the conditioned medium in which the host cells had been grown. At the end of each time point, cells were washed three times with ice-cold PBS, lysed with 1% NP-40 lysis buffer [20 mM Tris pH 8.0, 137 mM NaCl, 1% NP-40, 10% glycerol and 1× complete protease inhibitor cocktail (Boehringer Mannheim, Germany)] and then centrifuged at 12 000 g. Cleared lysates were adjusted for equal protein content using the BCA reagent system (Pierce) and immunoprecipitated overnight at 4°C with anti-FAK antiserum (1:100 rabbit polyclonal; Santa Cruz Biotech. Inc.) or anti-vinculin antiserum (2 µg/ml, clone V284 Upstate Biotechnology Inc.). Immune complexes were captured with 50 µl of 50% protein A–Sepharose for 1 h at 4°C, washed three times with ice-cold lysis buffer and then boiled for 8 min in SDS sample buffer. Proteins were resolved by SDS–PAGE using 7.5% polyacrylamide gels, transferred to nitrocellulose and immunoblotted with anti-PI 3-kinase antiserum (1 µg/ml rabbit polyclonal; UBI), rabbit phospho-specific anti-FAKPY397 (0.5 µg/ml; Biosource Inc.), anti-α-actinin (2 µg/ml, clone AT6/172; UBI), anti-tensin (2 µg/ml, clone 5B9; UBI) or anti-talin (4 µg/ml; UBI). Proteins were visualized with the Super Signal WestDura enhanced chemiluminescence system (Pierce) and analyzed using the Fluor-S MultiImager system equipped with Quantity One analysis software (Bio-Rad). In some cases, blots were stripped with IgG elution buffer (Pierce) according to the manufacturer’s instructions and re-probed with the appropriate antisera to demonstrate that similar amounts of proteins were present in each lane.
Protein-coated bead preparation and internalization assays
Carboxylate-modified non-fluorescent beads (1.17 µm diameter; IDC Microspheres, Portland, OR) were incubated with ~250 µg of purified FimCH complex, FimC or BSA (Sigma) as described (Braun et al., 1998). The presence of FimC in the FimCH complex is solely to stabilize the FimH adhesin in a mannose-binding-competent conformation (Choudhury et al., 1999). Equal numbers of FimC-, FimCH- and BSA-coated beads (±2.5% d-mannose) were added to 5637 cells grown on 12 mm sterile glass coverslips in 24-well plates. Plates were then centrifuged for 5 min at 600 g and incubated for 30–60 min at 37°C. Cells were then washed three times with PBS ± 2.5% d-mannose and fixed for 20 min at room temperature in fresh 2.5% paraformaldehyde in PBS.
To stain extracellular beads, cells were blocked with RPMI/10% FBS for 10 min at room temperature, washed with RPMI and then incubated for 1 h at room temperature with a 1:500 dilution of either mouse anti-FimCH antiserum (MedImmune Inc., Gaithersburg, MD) or rabbit anti-BSA antiserum (Sigma) in RPMI/1% FBS. Cells were washed in PBS and incubated with either anti-mouse IgG–Cy3 or anti-rabbit IgG–Cy3 conjugate (1:500) (Amersham) for 45 min at room temperature. After additional washes, coverslips were mounted in anti-fade mounting medium (kind gift of R.Rosqvist, Umea, Sweden). Samples were examined with an Olympus BX60 fluorescent microscope using an 80× objective. Images were captured using IP LabSpectrum software. Random fields of cells were viewed in phase contrast and fluorescent modes and the images were superimposed using Adobe Photoshop. Beads that were inaccessible to the staining procedure were scored as intracellular. Data are presented as the number of beads internalized divided by the number of adherent beads. Data shown are representative of at least four separate assays.
Examination of the actin cytoskeleton
5637 cells, seeded onto 12 mm sterile glass coverslips, were infected with GFP-expressing bacteria or incubated with FimCH-, FimC- or BSA-coated beads as described above. After 30–60 min, cells were washed, fixed with 2.5% paraformaldehyde/PBS and blocked with RPMI/10% FBS. Cells were then permeabilized using 0.2% Triton X-100 in RPMI/1% FBS and incubated for 20 min at room temperature with Texas red-labeled phalloidin (Molecular Probes, Eugene, OR). FimCH-coated beads were stained with mouse anti-FimCH antiserum followed by anti-mouse IgG–Cy3 conjugate (Amersham) prior to host cell permeabilization. Samples were examined using a Bio-Rad MRC 1024 dual laser scanning confocal microscope system equipped with a Zeiss Axioplan fluorescent microscope, and images were analyzed using the LaserSharp (Bio-Rad) and Confocal Assistant software packages.
Scanning and transmission EM
For SEM, 5637 cells grown on glass coverslips and infected with type 1-piliated bacteria or incubated with beads for 30–60 min were washed and fixed in 2% glutaraldehyde in NHC buffer (100 mM NaCl, 30 mM HEPES pH 7.4 and 2 mM CaCl2). Samples were then post-fixed with 1% OsO4/NHC, rinsed, dehydrated in ascending concentrations of ethyl alcohol, critical point dried from liquid CO2, coated with ~150 Å gold and examined using a Hitachi S-4500 FEG scanning electron microscope at 15 kV accelerating voltage.
For TEM, 5637 cells grown in 10 mm tissue culture dishes were incubated with E.coli or FimCH- or BSA-coated beads, washed, fixed in 1% glutaraldehyde in 0.1 M cacodylate buffer and post-fixed with 1.25% OsO4. Samples were stained en bloc for 30 min at room temperature with 4% aqueous uranyl acetate and then dehydrated in increasing concentrations of ethanol. Cells were embedded in gelatin capsules containing Poly/Bed 812 (Electron Microscopy Sciences, Fort Washington, PA) overnight at 60°C, thin sectioned (1 µm) using a Reichert-Jung Ultra Cut device and then post-stained in 4% uranyl acetate and lead citrate. Samples were viewed on a Zeiss 902 electron microscope.
Acknowledgements
We would like to thank M.Veith and M.Levy for their help with scanning and transmission EM; H.Teng and R.Rosqvist for assistance with the confocal microscopy; S.Langermann and MedImmune Inc. for providing anti-FimH antibodies; and S.Normark, H.Wolf-Watz, J.Vogel, D.Russell and members of the Hultgren laboratory for helpful discussions and suggestions. This work was supported by NIH grants 5R37AI2954910 and R01DK51406. M.A.M was supported by a Lucille P.Markey Special Emphasis Pathway in Human Pathobiology postdoctoral fellowship and by NIH fellowship AI09787.
References
- Alrutz M.A. and Isberg,R.R. (1998) Involvement of focal adhesion kinase in invasin mediated uptake. Proc. Natl Acad. Sci. USA, 95, 13658–13663. [Europe PMC free article] [Abstract] [Google Scholar]
- Baorto D.M., Gao,Z., Malaviya,R., Dustin,M.L., van der Merwe,A., Lublin,D.M. and Abraham,S.N. (1997) Survival of FimH-expressing enterobacteria in macrophages relies on glycolipid traffic. Nature, 389, 636–639. [Abstract] [Google Scholar]
- Barnett B.J. and Stephens,D.S. (1997) Urinary tract infection: an overview. Am. J. Med. Sci., 314, 245–249. [Abstract] [Google Scholar]
- Bliska J.B., Galan,J.E. and Falkow,S. (1993) Signal transduction in the mammalian cell during bacterial attachment and entry. Cell, 73, 903–920. [Abstract] [Google Scholar]
- Blomfield I.C., McClain,M.S. and Eisenstein,B.I. (1991) Type 1 fimbriae mutants of Escherichia coli K12: characterization of recognized afimbriate strains and construction of new fim deletion mutants. Mol. Microbiol., 5, 1439–1445. [Abstract] [Google Scholar]
- Braun L., Ohayon,H. and Cossart,P. (1998) The InlB protein of Listeria monocytogenes is sufficient to promote entry into mammalian cells. Mol. Microbiol., 27, 1077–1087. [Abstract] [Google Scholar]
- Carpenter C.L. and Cantley,L.C. (1996) Phosphoinositide kinases. Curr. Opin. Cell Biol., 8, 153–158. [Abstract] [Google Scholar]
- Carpenter C.L., Duckworth B.C., Auger,K.R., Cohen,B., Schaffhausen,B.S. and Cantley,L.C. (1990) Purification and characterization of phosphoinositide 3-kinase from rat liver. J. Biol. Chem., 265, 19704–19711. [Abstract] [Google Scholar]
- Chen H.-C. and Guan,J.-L. (1994) Association of focal adhesion kinase with its potential substrate phosphatidylinositol 3-kinase. Proc. Natl Acad. Sci. USA, 91, 10148–10152. [Europe PMC free article] [Abstract] [Google Scholar]
- Chen H.-C. and Guan J.-L. (1996) The association of focal adhesion kinase with a 200-kDa protein that is tyrosine phosphorylated in response to platelet-derived growth factor. Eur. J. Biochem., 235, 495–500. [Abstract] [Google Scholar]
- Chen H.-C., Appeddu,P.A., Isoda,H. and Guan,J.-L. (1996) Phosphorylation of tyrosine 397 in focal adhesion kinase is required for binding phosphatidylinositol 3-kinase. J. Biol. Chem., 271, 26329–26334. [Abstract] [Google Scholar]
- Choudhury D., Thompson,A., Stojanoff V., Langermann,S., Pinkner,J., Hultgren,S.J. and Knight,S.D. (1999) X-ray structure of the FimC–FimH chaperone–adhesin complex from uropathogenic Escherichia coli. Science, 285, 1061–1066. [Abstract] [Google Scholar]
- Cobb B.S., Schaller,M.D., Leu,T.H. and Parsons,J.T. (1994) Stable association of pp60src and pp59fyn with focal adhesion-associated protein tyrosine kinase, pp125FAK. Mol. Cell. Biol., 14, 147–155. [Europe PMC free article] [Abstract] [Google Scholar]
- Comolli J.C., Waite,L.L., Mostov,K.E. and Engel,J.N. (1999) Pili binding to asialo-GM1 on epithelial cells can mediate cytotoxicity or bacterial internalization by Pseudomonas aeruginosa. Infect. Immun., 67, 3207–3214. [Europe PMC free article] [Abstract] [Google Scholar]
- Connell H., Agace,W., Klemm,P., Schembri,M., Marild,S. and Svanborg,C. (1996) Type 1 fimbrial expression enhances Escherichia coli virulence for the urinary tract. Proc. Natl Acad. Sci. USA, 93, 9827–9832. [Europe PMC free article] [Abstract] [Google Scholar]
- Cormack B.P., Valdivia,R.H. and Falkow,S. (1996) FACS-optimized mutants of the green fluorescent protein (GFP). Gene, 173, 33–38. [Abstract] [Google Scholar]
- Dekker L.V. and Segal,A.W. (2000) Signals to move cells. Science, 287, 982–985. [Abstract] [Google Scholar]
- Dibb-Fuller M.P., Allen-Vercoe,E., Thorns,C.J. and Woodward,M.J. (1999) Fimbriae- and flagella-mediated association with and invasion of cultured epithelial cells by Salmonella enteritidis. Microbiology, 145, 1023–1031. [Abstract] [Google Scholar]
- Dumenil G., Olivo,J.C., Pellegrini,S., Fellous,M., Sansonetti,P.J. and Nhieu,G.T. (1998) Interferon α inhibits a Src-mediated pathway necessary for Shigella-induced cytoskeletal rearrangements in epithelial cells. J. Cell Biol., 143, 1003–1012. [Europe PMC free article] [Abstract] [Google Scholar]
- Elsinghorst E.A. (1994) Measurement of invasion by gentamicin resistance. Methods Enzymol., 236, 405–420. [Abstract] [Google Scholar]
- Evans D.J., Frank,D., Finck-Barbancon,V., Wu,C. and Fleiszig,S. (1998) Pseudomonas aeruginosa invasion and cytotoxicity are independent events, both of which involve protein tyrosine kinase activity. Infect. Immun., 66, 1453–1459. [Europe PMC free article] [Abstract] [Google Scholar]
- Falkow S., Isberg,R.R. and Portnoy,D.A. (1992) The interaction of bacteria with mammalian cells. Annu. Rev. Cell Biol., 8, 333–363. [Abstract] [Google Scholar]
- Finlay B.B. and Cossart,P. (1997) Exploitation of mammalian host cell function by bacterial pathogens. Science, 276, 718–725. [Abstract] [Google Scholar]
- Finlay B.B. and Falkow,S. (1997) Common themes in microbial pathogenicity revisited. Microbiol. Mol. Biol. Rev., 61, 136–169. [Europe PMC free article] [Abstract] [Google Scholar]
- Finlay B.B, Ruschkowski,S. and Dedhar,S. (1991) Cytoskeletal rearrangements accompanying Salmonella entry into epithelial cells. J. Cell Sci., 99, 283–296. [Abstract] [Google Scholar]
- Fukami K., Endo,T., Imamura,M. and Takenawa,T. (1994) α-actinin and vinculin are PIP2-binding proteins involved in signaling by tyrosine kinase. J. Biol. Chem., 269, 1518–1522. [Abstract] [Google Scholar]
- Fukushi Y., Orikasa,S. and Kagayama,M. (1979) An electron microscopic study of the interaction between vesical epithelium and E.coli. Invest. Urol., 17, 61–68. [Abstract] [Google Scholar]
- Gerwien J., Nielsen,M., Labuda,T., Nissen,M.H., Svejgaard,A., Geisler,C., Ropke,C. and Odum,N. (1999) Cutting edge: TCR stimulation by antibody and bacterial superantigen induces Stat3 activation in human T cells. J. Immunol., 163, 1742–1745. [Abstract] [Google Scholar]
- Gilmore A.P., Jackson,P., Waites,G.T. and Critchley,D.R. (1992) Further characterization of the talin-binding site in the cytoskeletal protein vinculin. J. Cell Sci., 103, 719–731. [Abstract] [Google Scholar]
- Griffiss J.M., Lammel,C.J., Wang,J., Dekker,N.P. and Brooks,G.F. (1999) Neisseria gonorrhoeae coordinately uses pili and Opa to activate HEC-1-B microvilli, which causes engulfment of the gonococci. Infect. Immun., 67, 3469–3480. [Europe PMC free article] [Abstract] [Google Scholar]
- Guinebault C., Payrastre,B., Racaud-Sultan,C., Mazarguil,H., Breton,M., Mauco,G., Plantavid,M. and Chap,H. (1995) Integrin-dependent translocation of phosphoinositide 3-kinase to the cytoskeleton of thrombin-activated platelets involves specific interactions of p85 α with actin filaments and focal adhesion kinase. J. Cell Biol., 129, 831–842. [Europe PMC free article] [Abstract] [Google Scholar]
- Hartwig J.H., Bokoch,G.M., Carpenter,C.L., Janmey,P.A., Taylor,L.A., Toker,A. and Stossel,T.P. (1995) Thrombin receptor ligation and activated Rac uncap actin filament barbed ends through phosphoinositide synthesis in permeabilized human platelets. Cell, 82, 643–653. [Abstract] [Google Scholar]
- Hooton T.M. and Stamm,W.E. (1997) Diagnosis and treatment of uncomplicated urinary tract infection. Infect. Dis. Clin. North Am., 11, 551–581. [Abstract] [Google Scholar]
- Hvidberg H., Struve,C., Krogfelt,K.A., Christensen,N., Rasmussen,S.N. and Frimodt-Moller,N. (2000) Development of a long-term ascending urinary tract infection mouse model for antibiotic treatment studies. Antimicrob. Agents Chemother., 44, 156–163. [Europe PMC free article] [Abstract] [Google Scholar]
- Ireton K., Payrastre,B., Chap,H., Ogawa,W., Sakaue,H., Kasuga,M. and Cossart,P. (1996) A role for phosphoinositide 3-kinase in bacterial invasion. Science, 274, 780–784. [Abstract] [Google Scholar]
- Ireton K., Payrastre,B. and Cossart,P. (1999) The Listeria monocytogenes protein InlB is an agonist of mammalian phosphoinositide 3-kinase. J. Biol. Chem., 274, 17025–17032. [Abstract] [Google Scholar]
- Isberg R.R., Voorhis,D.L. and Falkow,S. (1987) Identification of invasin, a protein that allows enteric bacteria to penetrate cultured mammalian cells. Cell, 50, 769–778. [Abstract] [Google Scholar]
- Jones C.H., Pinkner,J.S., Roth,R., Heuser,J., Nicholes,A.V., Abraham,S.N. and Hultgren,S.J. (1995) FimH adhesin of type 1 pili is assembled into a fibrillar tip structure in the Enterobacteriaceae. Proc. Natl Acad. Sci. USA, 92, 2081–2085. [Europe PMC free article] [Abstract] [Google Scholar]
- Kroemker M., Rudiger,A.H., Jockusch,B.M. and Rudiger,M. (1994) Intramolecular interactions in vinculin control α-actinin binding to the vinculin head. FEBS Lett., 355, 259–262. [Abstract] [Google Scholar]
- Kuhlman P.A., Hemmings,L. and Critchley,D.R. (1992) The identification and characterization of an actin binding site in α-actinin by mutagenesis. FEBS Lett., 304, 201–206. [Abstract] [Google Scholar]
- Langermann S. et al. (1997) Prevention of mucosal Escherichia coli infection by FimH-adhesin-based systemic vaccination. Science, 276, 607–611. [Abstract] [Google Scholar]
- Leevers S.J., Vanhaesebroeck,B. and Waterfield,M.D. (1999) Signalling through phosphoinositide 3-kinases: the lipids take centre stage. Curr. Opin. Cell Biol., 11, 219–225. [Abstract] [Google Scholar]
- Leffler H. and Svanborg-Eden,C. (1980) Chemical identification of a glycosphingolipid receptor for Escherichia coli attaching to human urinary tract epithelial cells and agglutinating human erythrocytes. FEMS Microbiol. Lett., 8, 127–134. [Google Scholar]
- Malaviya R. and Abraham,S.N. (1998) Clinical implications of mast cell–bacteria interactions. J. Mol. Med., 76, 617–623. [Abstract] [Google Scholar]
- McTaggart L.A., Rigby,R.C. and Elliot,T.S.J. (1990) The pathogenesis of urinary tract infections associated with Escherichia coli, Staphylococcus saprophyticus and S.epidermidis. J. Med. Microbiol., 32, 135–141. [Abstract] [Google Scholar]
- Mecsas J., Raupach,B. and Falkow,S. (1998) The Yersinia Yops inhibit invasion of Listeria, Shigella and Edwardsiella but not Salmonella into epithelial cells. Mol. Microbiol., 28, 1269–1281. [Abstract] [Google Scholar]
- Menard R., Dehio,C. and Sansonetti,P.J. (1996) Bacterial entry into epithelial cells: the paradigm of Shigella. Trends Microbiol., 4, 220–226. [Abstract] [Google Scholar]
- Mengaud J., Ohayon,H., Gounon,P., Mege,R.-M. and Cossart,P. (1996) E-cadherin is the receptor for internalin, a surface protein required for entry of L.monocytogenes into epithelial cells. Cell, 84, 923–932. [Abstract] [Google Scholar]
- Minion F.C., Abraham,S.N., Beachy,E.H. and Goguen,J.D. (1986) The genetic determinant of adhesive function in type 1 fimbriae of Escherichia coli is distinct from the gene encoding the fimbrial subunit. J. Bacteriol., 165, 1033–1036. [Europe PMC free article] [Abstract] [Google Scholar]
- Mulvey M.A., Lopez-Boado,Y.S., Wilson,C.L., Roth,R., Parks,W.C., Heuser,J. and Hultgren,S.J. (1998) Induction and evasion of host defenses by type 1-piliated uropathogenic Escherichia coli.Science, 282, 1494–1497. [Abstract] [Google Scholar]
- Mulvey M.A., Schilling,J.D., Martinez,J.J. and Hultgren,S.J. (2000) Bad bugs and beleaguered bladders: interplay between uropathogenic E.coli and innate host defenses. Proc. Natl Acad. Sci. USA, in press. [Europe PMC free article] [Abstract] [Google Scholar]
- Orndorff P.E. and Falkow,S. (1984) Identification and characterization of a gene product that regulates type 1 piliation in Escherichia coli.J. Bacteriol., 160, 61–66. [Europe PMC free article] [Abstract] [Google Scholar]
- Palmer L.M., Reilly,T.J., Utsalo,S.J. and Donnenberg,M.S. (1997) Internalization of Escherichia coli by human renal epithelial cells is associated with tyrosine phosphorylation of specific host cell proteins. Infect. Immun., 65, 2570–2575. [Europe PMC free article] [Abstract] [Google Scholar]
- Parsons J.T. (1996) Integrin-mediated signaling: regulation by protein tyrosine kinases and small GTP-binding proteins. Curr. Opin. Cell Biol., 8, 146–152. [Abstract] [Google Scholar]
- Russell P.W. and Orndorff,P.E. (1992) Lesions in two Escherichia coli type 1 genes alter pilus number and length without affecting receptor binding. J. Bacteriol., 174, 5923–5935. [Europe PMC free article] [Abstract] [Google Scholar]
- Sansonetti P.J. (1991) Genetic and molecular basis of epithelial cell invasion by Shigella species. Rev. Infect. Dis., 13, 285–292. [Abstract] [Google Scholar]
- Schulte R., Zumbihl,R., Kampik,D., Fauconnier,A. and Autenrieth,I.B. (1998) Wortmannin blocks Yersinia invasin-triggered internalization, but not interleukin production by epithelial cells. Med. Microbiol. Immunol., 187, 53–60. [Abstract] [Google Scholar]
- Shoelson S.E., Sivaraja,M., Williams,K.P., Hu,P., Schlessinger,J. and Weiss,M.A. (1993) Specific phosphopeptide binding regulates a conformational change in the PI 3-kinase SH2 domain associated with enzyme activation. EMBO J., 12, 795–802. [Europe PMC free article] [Abstract] [Google Scholar]
- Stromberg N., Marklund,B.I., Lund,B., Ilver,D., Hamers,A., Gaastra,W., Karlsson,K.A. and Normark,S. (1990) Host-specificity of uropathogenic Escherichia coli depends on differences in binding specificity to Galα1–4Gal-containing isoreceptors. EMBO J., 9, 2001–2010. [Europe PMC free article] [Abstract] [Google Scholar]
- Tewari R., MacGregor,J.I., Ikeda,T., Little,J.R., Hultgren,S.J. and Abraham,S.N. (1993) Neutrophil activation by nascent FimH subunits of type 1 fimbriae purified from the periplasm of Escherichia coli. J. Biol. Chem., 268, 3009–3015. [Abstract] [Google Scholar]
- Thankavel K., Madison,B., Ikeda,T., Malaviya,R., Shah,A.H., Arumugan,P.M. and Abraham,S.N. (1997) Localization of a domain in the fimH adhesin of Escherichia coli type 1 fimbriae capable of receptor recognition and use of a domain-specific antibody to confer protection against experimental urinary tract infection. J. Clin. Invest., 100, 1123–1136. [Europe PMC free article] [Abstract] [Google Scholar]
- Vanhaesebroeck B. and Waterfield,M.D. (1999) Signaling by distinct classes of phosphoinositide 3-kinases. Exp. Cell Res., 253, 239–254. [Abstract] [Google Scholar]
- Vlahos C.J., Matter,W.F., Hui,K.Y. and Brown,R.F. (1994) A specific inhibitor of phosphatidylinositol 3-kinase, 2-(4-morpholinyl)-8-phenyl-4H-1-benzopyran-4-one (LY294002). J. Biol. Chem., 269, 5241–5248. [Abstract] [Google Scholar]
- Weinberg A., Belton,C.M., Park,Y. and Lamont,R.J. (1997) Role of fimbriae in Porphyromonas gingivalis invasion of gingival epithelial cells. Infect. Immun., 65, 313–316. [Europe PMC free article] [Abstract] [Google Scholar]
Articles from The EMBO Journal are provided here courtesy of Nature Publishing Group
Full text links
Read article at publisher's site: https://doi.org/10.1093/emboj/19.12.2803
Read article for free, from open access legal sources, via Unpaywall:
https://europepmc.org/articles/pmc203355?pdf=render
Citations & impact
Impact metrics
Citations of article over time
Alternative metrics
Article citations
Towards Understanding Tumour Colonisation by Probiotic Bacterium E. coli Nissle 1917.
Cancers (Basel), 16(17):2971, 26 Aug 2024
Cited by: 0 articles | PMID: 39272829 | PMCID: PMC11394440
Review Free full text in Europe PMC
Uropathogenic Escherichia coli Subverts Host Autophagic Defenses by Stalling Preautophagosomal Structures to Escape Lysosome Exocytosis.
J Infect Dis, 230(3):e548-e558, 01 Sep 2024
Cited by: 1 article | PMID: 38330453 | PMCID: PMC11420784
Diabetes compromises tight junction protein claudin 14 in the urinary bladder.
Cell Tissue Res, 398(1):27-33, 20 Aug 2024
Cited by: 0 articles | PMID: 39162877 | PMCID: PMC11424655
A New "Non-Traditional" Antibacterial Drug Fluorothiazinone-Clinical Research in Patients with Complicated Urinary Tract Infections.
Antibiotics (Basel), 13(6):476, 22 May 2024
Cited by: 0 articles | PMID: 38927143
Gentamicin loaded niosomes against intracellular uropathogenic Escherichia coli strains.
Sci Rep, 14(1):10196, 03 May 2024
Cited by: 0 articles | PMID: 38702355
Go to all (449) article citations
Data
Similar Articles
To arrive at the top five similar articles we use a word-weighted algorithm to compare words from the Title and Abstract of each citation.
Requirement of Rho-family GTPases in the invasion of Type 1-piliated uropathogenic Escherichia coli.
Cell Microbiol, 4(1):19-28, 01 Jan 2002
Cited by: 94 articles | PMID: 11856170
Integrin-mediated host cell invasion by type 1-piliated uropathogenic Escherichia coli.
PLoS Pathog, 3(7):e100, 01 Jul 2007
Cited by: 177 articles | PMID: 17630833 | PMCID: PMC1914067
Human Urine Decreases Function and Expression of Type 1 Pili in Uropathogenic Escherichia coli.
mBio, 6(4):e00820, 30 Jun 2015
Cited by: 41 articles | PMID: 26126855 | PMCID: PMC4488945
Covert operations of uropathogenic Escherichia coli within the urinary tract.
Traffic, 6(1):18-31, 01 Jan 2005
Cited by: 86 articles | PMID: 15569242 | PMCID: PMC2523259
Review Free full text in Europe PMC
Funding
Funders who supported this work.
NIAID NIH HHS (3)
Grant ID: F32 AI009787
Grant ID: 5R37AI2954910
Grant ID: AI09787
NIDDK NIH HHS (2)
Grant ID: R01DK51406
Grant ID: R01 DK051406