Abstract
Free full text

ESAM supports neutrophil extravasation, activation of Rho, and VEGF-induced vascular permeability
Abstract
Endothelial cell–selective adhesion molecule (ESAM) is specifically expressed at endothelial tight junctions and on platelets. To test whether ESAM is involved in leukocyte extravasation, we have generated mice carrying a disrupted ESAM gene and analyzed them in three different inflammation models. We found that recruitment of lymphocytes into inflamed skin was unaffected by the gene disruption. However, the migration of neutrophils into chemically inflamed peritoneum was inhibited by 70% at 2 h after stimulation, recovering at later time points. Analyzing neutrophil extravasation directly by intravital microscopy in the cremaster muscle revealed that leukocyte extravasation was reduced (50%) in ESAM−/− mice without affecting leukocyte rolling and adhesion. Depletion of >98% of circulating platelets did not abolish the ESAM deficiency–related inhibitory effect on neutrophil extravasation, indicating that it is only ESAM at endothelial tight junctions that is relevant for the extravasation process. Knocking down ESAM expression in endothelial cells resulted in reduced levels of activated Rho, a GTPase implicated in the destabilization of tight junctions. Indeed, vascular permeability stimulated by vascular endothelial growth factor was reduced in ESAM−/− mice. Collectively, ESAM at endothelial tight junctions participates in the migration of neutrophils through the vessel wall, possibly by influencing endothelial cell contacts.
Extravasating leukocytes have to migrate through the blood vessel wall, a process called diapedesis. Several endothelial cell contact proteins, such as platelet endothelial cell adhesion molecule-1 (PECAM-1), members of the junctional adhesion molecule (JAM) family (JAM-A, JAM-B, and JAM-C), CD99, and ICAM-2, have been described to support leukocyte diapedesis (1–4), although the detailed mechanisms by which they mediate this process are not yet understood.
The endothelial cell–selective adhesion molecule (ESAM), although related to the JAM family members, differs considerably in structure (5), cytosolic binding partners (6, 7), and tissue distribution. In contrast to the JAMs, expression of ESAM is strictly limited to endothelial cells and platelets and cannot be found on leukocytes or epithelia (8). On platelets ESAM is only expressed upon activation, whereas on endothelial cells it is strictly restricted to tight junctions as was demonstrated by immunogold labeling (8).
Recently, ESAM-deficient mice were described to have defects in tumor angiogenesis, although embryonal angiogenesis was unaffected (9). Because of its localization at endothelial tight junctions, we decided to investigate a potential role of ESAM in leukocyte extravasation. Here we show that migration of neutrophils into inflamed peritoneum is delayed in ESAM-deficient mice, whereas the entry of lymphocytes into inflamed skin was unaffected. Intravital microscopy revealed that it was the migration of leukocytes through the vessel wall, the diapedesis process, which was impaired by the lack of ESAM. We could exclude ESAM on platelets as being relevant for leukocyte extravasation and found that endothelial ESAM supports the activation of Rho. Because activated Rho supports the opening of tight junctions and because the increase of vascular endothelial growth factor (VEGF)-stimulated vascular permeability was attenuated by the lack of ESAM, our results point toward a role of ESAM in the opening of endothelial cell contacts.
RESULTS AND DISCUSSION
Generation of ESAM-deficient mice
The targeting construct to disrupt the ESAM gene was created such that the coding sequences of exons 1 and 2 were replaced by a neomycin expression cassette leading to the deletion of the start codon (Fig. 1 A). Southern blot analysis of DNA of the generated mice with an external probe as well as PCR genotyping revealed the expected results (Fig. 1, B and C). As determined in immunoblots of lung and heart tissue, heterozygous ESAM+/− mice showed reduced levels of ESAM protein expression, whereas the ESAM protein was undetectable with polyclonal antibodies in tissues of ESAM−/− mice (Fig. 1 D).
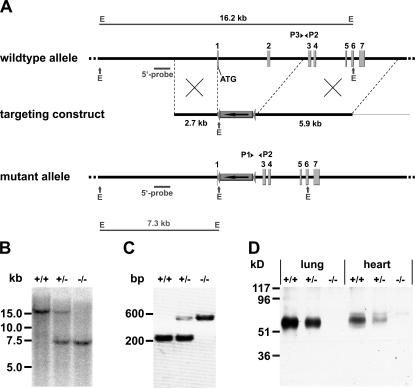
Targeted disruption of the ESAM gene in mice. (A) Targeting strategy. A map of the relevant genomic region containing the first ATG-containing and the following six exons of ESAM (top), the targeting construct (middle), and the mutated locus after recombination (bottom) are shown. P1, P2, and P3 represent oligonucleotides used for PCR screening. The “external” probe used for Southern screening is depicted as a small bar. The 16.2- and 7.3-kb Southern fragments obtained for the WT or the mutated allele upon EcoRV (E) digestion, respectively, are depicted at the top and the bottom. (B) Detection of WT and targeted alleles by Southern blot analysis. Genomic DNA of mice of the F1 generation was digested with EcoRV. (C) PCR genotyping using primers P1, P2, and P3 resulted in 225- or 538-bp products for the mutant or WT allele, respectively. (D) Western blot of SDS-urea extracts of lung or heart tissue (as indicated) of adult mice of the three different genotypes with polyclonal antibody against mouse ESAM.
Heterozygous as well as homozygous mice backcrossed into the C57BL/6 background were viable and apparently normal. Mutant mice had a 10% reduction in weight in agreement with Ishida et al. (9). Absolute and differential leukocyte counts (determined for leukocytes stained for CD4, CD8, CD19, Gr-1, and F4-80 antigen) were normal.
ESAM is required for the recruitment of neutrophils, but not of lymphocytes, into inflamed tissue
We have recently reported that polyclonal antibodies against ESAM did not block the migration of lymphocytes into inflamed skin in a delayed-type hypersensitivity model (3). However, these antibodies may not block functional epitopes of ESAM. To clarify the role of ESAM in lymphocyte extravasation, we compared ESAM−/− and ESAM+/+ animals in the delayed-type hypersensitivity model. As shown in Fig. 2 A, dinitrofluorobenzene (DNFB)-elicited emigration of T cells at 15 and 5 h after injection of radioactively labeled T cells was similar between ESAM+/+ and ESAM−/− animals. No significant T cell emigration was detectable at 2 h after T cell inoculation (not depicted). Thus, ESAM is not required for the accumulation of lymphocytes into inflamed skin. Neither the recruitment of CD4+ nor CD8+ T cells was affected, as was analyzed by immunohistochemistry (not depicted).
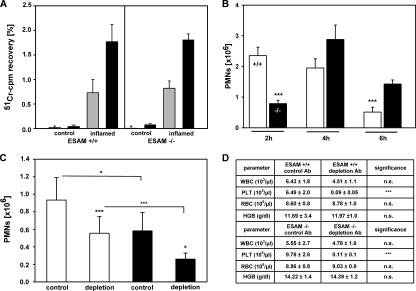
Role of ESAM in neutrophil, but not lymphocyte, extravasation. (A) T cells from the lymph nodes of DNFB-sensitized donor mice were labeled with 51Cr and injected into the tail vein of sensitized and challenged ESAM+/+ or ESAM−/− mice (as indicated). After 5 (gray bars) and 15 h (black bars), radioactivity accumulated in the noninflamed (control) or DNFB-challenged ear (inflamed) was calculated as percent of total injected radioactivity. (B) WT (+/+) or ESAM-deficient (−/−) mice were i.p. challenged with thioglycollate, and peritoneal neutrophils were counted 2, 4, or 6 h later. The data represent the mean ± SD of four or more mice in each group, and the depicted experiment represents one of three independent experiments with similar results. (C) ESAM+/+ (white bars) and ESAM−/− mice (black bars) were either injected i.v. with control antibodies (control) or with mAb against the platelet antigen GP1bα (depletion) to deplete platelets from the circulation. 24 h later, peripheral blood samples were analyzed for white and red blood cell and platelet counts (D) immediately followed by i.p. stimulation of mice with thioglcollate. 2 h later, peritoneal neutrophils were counted. The data in B and C represent the mean ± SD of four or more mice in each group, and the depicted experiment represents one of three independent experiments with similar results. *, P < 0.05; ***, P < 0.001.
Using the thioglycollate-induced peritonitis model, we tested whether neutrophil accumulation in the peritoneum would be affected by the lack of ESAM. Comparing the number of neutrophils accumulating at 2, 4, and 6 h after thioglycollate injection in ESAM−/− and ESAM+/+ mice revealed a strong reduction by 70% (±4.5%) of neutrophil emigration at 2 h (Fig. 2 B). At later time points leukocyte accumulation in ESAM−/− mice recovered, resulting in even more neutrophils at the 6-h time point in ESAM−/− mice than in ESAM+/+ mice. Similar results as shown here for mice backcrossed with C57BL/6 mice for four generations were also obtained with mice backcrossed for eight generations (not depicted). We conclude that the lack of ESAM impairs neutrophil extravasation, leading to a delay of the extravasation process shifting the peak of neutrophil accumulation to a later time point.
We wanted to test whether ESAM would also be dispensable for the extravasation of lymphocytes in a tissue and at a time point where and when neutrophils do require ESAM. Because neutrophils usually enter inflamed sites earlier than lymphocytes, we created an artificial situation and stimulated mice i.p. with IL-1β as well as with the chemokine CCL19 (MIP-3β). As shown in Fig. S1, available at http://www.jem.org/cgi/content/full/jem.20060565/DC1, neutrophils and CD4+ lymphocytes were recruited at the 2-h time point; however, only the number of recruited neutrophils, but not the number of artificially recruited, CCL19-responsive lymphocytes was reduced in ESAM−/− mice.
Because PECAM-1 is also known to mediate extravasation of neutrophils, but not of lymphocytes (1), we tested whether the contribution of PECAM-1 to this process would be dependent or independent of ESAM. As shown in Fig. S2, available at http://www.jem.org/cgi/content/full/jem.20060565/DC1, blocking PECAM-1 with antibodies clearly reduced neutrophil recruitment in WT C57BL/6 mice and in ESAM−/− mice to the same extent, indicating that the role of PECAM-1 in neutrophil extravasation is independent of ESAM.
ESAM on platelets is not involved in the contribution of platelets to neutrophil extravasation
Platelets represent the only other cell type besides endothelial cells that express ESAM. Because ESAM has been reported to mediate homotypic cell binding (5, 8) and because platelets participate in leukocyte extravasation as mediators of leukocyte–endothelial interactions, it seemed plausible that ESAM on platelets would be involved in leukocyte extravasation. To clarify this, we tested the effect of platelet depletion on the accumulation of neutrophils in the inflamed peritoneum of ESAM+/+ and ESAM−/− mice. As shown in Fig. 2 D, antibodies against GP1bα very efficiently reduced the number of platelets by 98% (±1%), whereas peripheral white blood cell counts were not significantly reduced. Platelet depletion inhibited neutrophil recruitment into inflamed peritoneum of ESAM+/+ mice by 41% (±20%; Fig. 2 C), clearly demonstrating the important role of platelets in neutrophil extravasation at this early time point. Interestingly, the same extent of inhibition was caused by the deficiency of ESAM. However, this reduced level of neutrophil recruitment was further reduced by 55% (±13%) if platelets were depleted in ESAM−/− mice (Fig. 2 C). Thus, platelets contribute to the process of neutrophil extravasation to a similar extent in ESAM+/+ mice as in ESAM−/− mice, demonstrating that ESAM is not required for the participation of platelets in this process. We conclude that it is not the lack of ESAM on platelets, but the lack of ESAM at endothelial tight junctions that is responsible for the reduction of neutrophil extravasation.
ESAM mediates leukocyte extravasation in vivo at the level of transmigration
To determine whether ESAM is involved in the diapedesis process rather than in the docking and adherence of neutrophils to the inner surface of the blood vessel wall, we visualized leukocyte extravasation in the cremaster muscle. When mice were stimulated intrascrotally with TNF-α for 4 h before microscopical analysis, we found a reduction of extravasated leukocytes by almost 50% in ESAM−/− mice when compared with ESAM+/+ mice (Fig. 3, D and E). In contrast, no significant difference between the two genotypes was observed in the number of adherent or rolling cells (Fig. 3, B, C, and E). Similar results were found if mice were stimulated for 4 h with IL-1β. The number of extravasated leukocytes was reduced by 45% in ESAM−/− mice compared with ESAM+/+ mice, and no significant difference was found in the number of rolling and adherent cells (Fig. 3 E). To rule out that the difference in extravasation could be caused by differences in leukocyte rolling or adhesion at an earlier time point during the 4-h period of cytokine stimulation, we stimulated mice for only 2 h with IL-1β before the analysis. Leukocyte extravasation was just starting at this time point, whereas leukocyte rolling and adherence was already well detectable and again was not different between the two genotypes (not depicted). We conclude that the absence of ESAM affects leukocyte extravasation on the level of transendothelial migration.
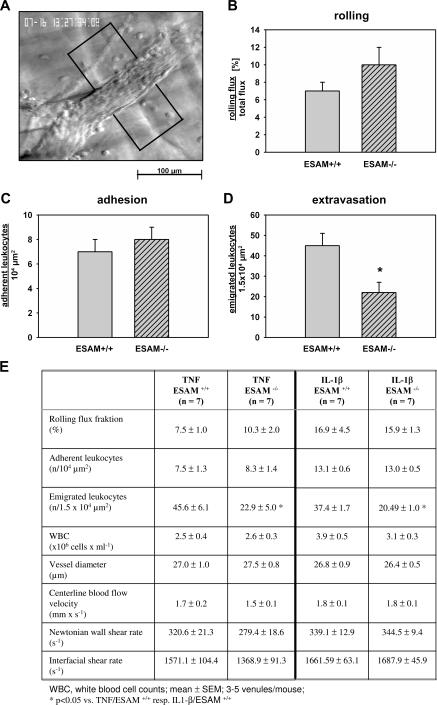
Intravital microscopy of TNF-α–stimulated cremaster muscle: neutrophil extravasation is reduced in ESAM−/− mice. (A) Reflected light oblique transillumination microscopic image of a venule of the mouse cremaster muscle, demarcations on each side of the venule determine the areas in which extravasated leukocytes were counted. (B) Rolling flux fraction. (C) Number of adhering leukocytes per 104 μm2 of venule surface area. (D) Numbers of extravasated leukocytes in cremasteric venules of TNF-α–treated ESAM+/+ and ESAM−/− mice per 1.5 × 104 μm2 tissue area. The measurements were performed 4 h after intrascrotal TNF-α injection. n = 7 for each group; *, P < 0.05 versus ESAM+/+. (E) Quantitation of the intravital microscopy results.
80% of extravasated cells upon TNF-α stimulation were positive for the granulocyte antigen Gr-1 as was determined by immunohistochemistry (not shown).
ESAM is involved in mechanisms regulating vascular permeability
To test whether the lack of ESAM would affect the leakiness of blood vessels for molecules, we injected mice i.v. with the dye Evan's blue and stimulated the peritoneum 15 min later for either 2 or 4 h with thioglycollate or for controls with PBS. As shown in Fig. 4 A, the leakiness for plasma protein–adsorbed Evan's blue was similar between ESAM+/+ and ESAM−/− mice in the absence of inflammation. Stimulation with thioglycollate increased the entry of Evan's blue into inflamed peritoneum. However, similar to our results for the extravasation of neutrophils (Fig. 2 B), less dye had leaked out into the peritoneum in ESAM−/− mice than in ESAM+/+ mice at 2 h, whereas at 4 h, exit of dye molecules had “recovered” in ESAM-deficient mice (Fig. 4 A). Thus, ESAM deficiency retards the increase of leakiness upon inflammatory stimulation in a similar way as it retards the extravasation of neutrophils. To analyze a potential role of ESAM in the regulation of endothelial permeability independent from leukocyte extravasation, we again injected ESAM+/+ and ESAM−/− mice i.v. with Evan's blue and stimulated them intradermally with VEGF165. Injected skin areas were excised from killed mice 15 and 30 min later, and the dye was extracted with formamide. As shown in Fig. 4 B, VEGF-stimulated vascular permeability increased less strongly in ESAM−/− mice than in ESAM+/+ mice, suggesting that ESAM is indeed involved in signaling mechanisms that trigger the opening of endothelial cell contacts.
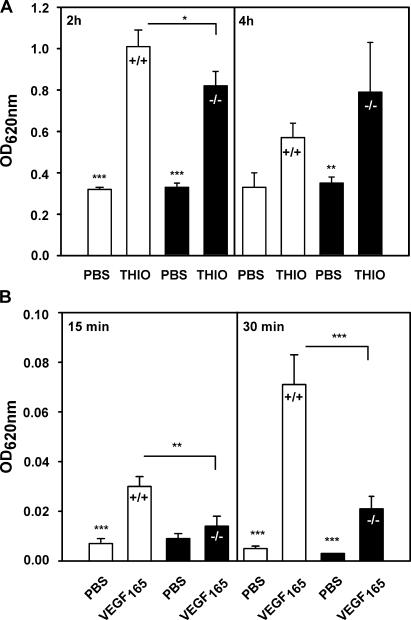
ESAM deficiency attenuates the increase of vascular permeability stimulated by inflammatory mediators as well as by VEGF. ESAM+/+ (white bars) and ESAM−/− mice (black bars) were i.v. injected with Evan's blue. (A) 15 min later, mice were i.p. injected with PBS or thioglycollate (as indicated) and either at 2 or 4 h after injection, the dye that had leaked into the peritoneum was quantified. (B) 10 min later, Evan's blue–injected mice were intradermally injected with VEGF and killed after an additional 15 or 30 min (as indicated), and the dye was extracted from skin samples and quantified. *, P < 0.05; **, P < 0.01; ***, P < 0.001.
The expression level and subcellular distribution pattern of other endothelial cell contact proteins, such as VE-cadherin, PECAM-1, and JAM-A, were unchanged in ESAM−/− mice as analyzed by quantitative immunoblot analysis of kidney extracts (not depicted) and immunofluorescence analysis of cryosections of brain, lung, and kidney, respectively (not depicted).
ESAM supports activation of the small GTPase Rho
Because small GTPases such as Rho and Rac1 have been reported to affect the stability of endothelial cell contacts, we tested whether the reduction of ESAM expression levels in cultured endothelial cells by RNAi would affect the activation levels of these GTPases. Testing six different pairs of small interfering RNA (siRNA) oligonucleotides resulted in the identification of one pair that reduced the expression level of ESAM to 5% within 36 h of transfection of the siRNAs into mouse bEnd.3 endothelioma cells (Fig. 5, top). We found that this inhibition reduced the levels of activated Rho by 77% (±7%; three experiments quantitated) in these cells, whereas no effects were seen on the levels of activated Rac1, Cdc42, or Rap1 (Fig. 5). Thus, the presence of ESAM supports signaling mechanisms that lead to the activation of Rho. Because activated Rho can lead to the destabilization of tight junctions (10, 11) and transendothelial migration of monocytes and neutrophils have been reported to require active Rho and Rho kinase (12, 13), the influence of ESAM on the activation of Rho could be involved in the mechanism by which ESAM supports neutrophil extravasation.
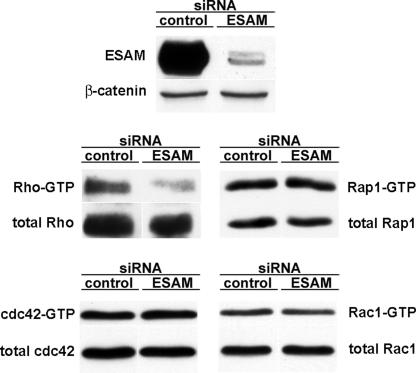
Knocking down ESAM expression in endothelial cells by siRNA interferes with activation of Rho, but not of Rac1, Cdc42, and Rap1. The mouse endothelioma cell line bEnd.3 was transfected either with control siRNAs (control) or with siRNAs directed against ESAM (ESAM). 36 h later, cell lysates were immunoblotted for ESAM and as a negative control for β-catenin (top). Activation levels of various GTPases (as indicated on the side) were analyzed by pull-down experiments with effector proteins followed by immunoblotting for the respective GTPase.
Concluding remarks
We have shown that ESAM is involved in the extravasation of neutrophils at an early step of the inflammatory process, whereas lymphocytes extravasate independently of ESAM. Although the detailed mechanism is unknown, several results reported here may lead the way toward understanding how ESAM functions. First, we found that ESAM is involved in the transmigration step, but not in the capturing and firm adhesion of neutrophils on the endothelial cell surface. Second, we could exclude a contribution of platelet-expressed ESAM and defined the endothelial tight junction–associated ESAM as the relevant molecule in this process. Third, we found that the expression of ESAM supports the activation of the GTPAse Rho. This opens the intriguing possibility that ESAM may be involved in Rho-mediated signaling mechanisms that trigger the opening of endothelial cell contacts. It will be important to elucidate how ESAM is stimulated in such a process. Direct binding of neutrophils to immobilized recombinant ESAM or to ESAM-transfected cells could not be demonstrated (not depicted). Alternatively, ESAM could be involved in a signaling pathway where it could serve as a transmitter of signals from other endothelial receptors, which would trigger the destabilization of endothelial contacts. Fourth, the lack of ESAM does not only attenuate neutrophil extravasation, but also the increase in VEGF-triggered permeability. This demonstrates that ESAM is relevant for the control of endothelial cell contacts beyond the process of leukocyte extravasation. Fifth, ESAM is involved in neutrophil extravasation mechanisms independent of the type of stimulating cytokine (TNF-α or IL-1β). This is in contrast to PECAM-1 and ICAM-2 because disrupting their genes in the C57BL/6 mouse strain affects neutrophil extravasation only if IL-1β, but not if TNF-α, was used for stimulation (4, 14). Sixth, besides PECAM-1, ESAM is the second molecule at endothelial cell contacts that selectively affects neutrophil, but not lymphocyte, extravasation. This hints toward interesting differences between lymphocytes and neutrophils with respect to their way of overcoming the endothelial barrier.
Collectively, our results establish ESAM as a new membrane protein at endothelial junctions that is involved in neutrophil extravasation and point toward a role of ESAM in signaling mechanisms that trigger the opening of endothelial cell contacts.
MATERIALS AND METHODS
Antibodies.
The following antibodies were used: mAb RB6-8C5 against Ly-6G and Ly-6C (Gr-1); FITC-conjugated monoclonal anti-CD4 and anti-CD8 antibodies (all from BD Biosciences); rat mAb RB40.34 against mouse P-selectin (15) and Mel14 against L-selectin (no. HB-132; American Type Culture Collection); 1G5.1 (IgG2b) and 5D2.6 (IgG2a), both against mouse PECAM-1 (unpublished data); isotype-matched negative control antibodies; and a polyclonal rabbit antibody against mouse ESAM-Fc (8).
Peritonitis assay.
For each assay, five 8–12-wk-old ESAM−/− mice and five sex-matched ESAM+/+ mice were used. Thioglycollate-induced peritonitis assays (induced by 1 ml of 3% thioglycollate broth) were essentially performed as described previously (16), except that the peritoneal lavage was performed with 20 ml PBS containing 3 mM EDTA, the total cell counts were determined with a cell counter (CASY; Schärfe-System), and the percentage of neutrophils was determined by flow cytometry (FACSCalibur; BD Biosciences) using the mAb RB6-8C5. Neutrophil numbers in peritoneum increased 50-fold in 2 h over mock-stimulated mice. For kinetic studies, new animals were used for each time point. Alternatively to thioglycollate, 10 ng IL-1β (Abazyme) was injected i.p. with 1 ml PBS. For antibody inhibition, 50 μg/mouse was injected i.v. 15 min before administering IL-1β.
For lymphocyte recruitment into inflamed peritoneum, mice were injected i.p. with 10 ng IL-1β and 4 μg CCL19 (R&D Systems). Lymphocytes were determined by flow cytometry using antibodies against CD4. Numbers of CD4+ T cells in the peritoneum were fourfold increased compared with mock-stimulated mice.
Platelet depletion experiments.
Platelet depletion was performed as described previously (17). For each assay, five 8–12-wk-old ESAM−/− and five sex-matched ESAM+/+ mice were used. Mice were injected i.v. with 80 μg rat mAb (in 200 μl PBS) against mouse GpIbα (CD42b) or with the same amount of isotype-matched control rat IgG, both obtained from Emfret Analytics. 24 h later, blood was collected from the retro-orbital plexus (under brief ether anesthesia) using heparinized microcapillaries (50 μl) treated with 0.1 mol/L sodium citrate. Blood was immediately collected in a tube containing 10% (vol/vol) 0.1 mol/L sodium citrate and then diluted with cold PBS. Platelets and white blood cells were counted with an automated cell counter (KX-21) from Sysmex. Shortly after bleeding, mice were challenged i.p. with thioglycollate, and neutrophil recruitment was determined as described above.
Blood vessel permeability assays.
The permeability of blood vessels was analyzed essentially as described previously (18). Evan's blue leaking into the peritoneal cavity was measured after peritoneal challenge with either 1 ml 3% thioglycollate broth or 1 ml PBS. For each assay, five 7–10-wk-old ESAM−/− or ESAM+/+ mice were used.
Modified Miles assay for VEGF165 (PeproTech)-stimulated vascular permeability in the skin was described previously (19). For each assay, three 8–12-wk-old ESAM−/− and three sex-matched ESAM+/+ mice were used. In brief, Evan's blue dye (Sigma-Aldrich) was injected into the tail vein (100 μl of a 1% solution in PBS), and 10 min later, 50 μl PBS or mouse VEGF165 (100 ng in 50 μl PBS) was intradermally injected into the shaved back skin. 15 or 30 min later, skin areas were excised and extracted with formamide for 5 d and the dye was measured at 620 nm. To exclude that the increase of Evan's blue simply reflected the increase in blood flow, we measured the quantity of hemoglobin in the tissue by noninvasive white light spectroscopy using an O2C spectroscopy device (LEA Medizintechnik). The quantity of hemoglobin in the VEGF-injected skin only increased by ~20%.
Intravital microscopy.
Surgical preparation of cremaster muscles and intravital microscopy were essentially done as described previously (20). Inflammatory stimulation was achieved by intrascrotal injection of 500 ng of recombinant murine TNF-α or 50 ng of recombinant murine IL-1β (R&D Systems) diluted in 0.3 ml PBS, administered to either ESAM+/+ or ESAM−/− mice 4 or 2 h (the latter only for IL-1β) before microscopic observation (n = 7 for each group). In each animal, three to five single unbranched postcapillary venules with diameters of 17.5 to 35 μm were analyzed. During a 15-min observation period, leukocyte rolling, adhesion, and extravasation were assessed by reflected light oblique transillumination microscopy. Videotaped images were evaluated off-line using CAPIMAGE software (Zeintl). Leukocyte rolling flux fraction is defined as the flux of rolling leukocytes in percent of total leukocyte flux. The total number of adherent leukocytes was determined for each venule segment (100 μm) and is expressed per 104 μm2 of venule surface area. Emigrated cells were counted in an area reaching out 75 μm to each side of a vessel over a distance of 100-μm vessel length and are presented per 1.5 × 104 μm2 tissue area. Centerline blood flow velocity was measured by using intra-arterial–administered microspheres (0.96 μm; FluoSpheres; Invitrogen). The Newtonian wall shear rate [s−1] was estimated as 8 x [V b /d], where V b is the mean blood flow velocity and d is the diameter of the vessel. Mean blood velocity, V b, was approximated by multiplying the centerline blood velocity by 0.625. The interfacial shear rate is the slope of the velocity profile at the interface of the endothelial surface layer and the vessel lumen, and it was calculated as 4.9 × 8 x [V b /d], where 4.9 is a mean empirical correction factor (21). Statistical analysis was done with the Mann-Whitney rank sum test, and p-values of <0.05 were considered significant.
All animal experiments were performed in accordance with the local animal protection legislation (Bezirksregierung Muenster).
Online supplemental material.
Fig. S1 demonstrates that the lack of ESAM impairs neutrophil, but not lymphocyte recruitment, into IL-1β/CCL19-stimulated peritoneum. Fig. S2 shows that antibodies against PECAM-1 block neutrophil recruitment independent of ESAM. Figs. S1 and S2 as well as supplemental Material and methods are available at http://www.jem.org/cgi/content/full/jem.20060565/DC1.
Acknowledgments
We thank Melissa Cudmore for help with the immunoblots depicted in Fig. 1 and Tanja Möller for excellent technical assistance.
This work was partially funded by the Deutsche Forschungsgemeinschaft (SFB492), the European Community (NoE MAIN 502935), and funds from the Max-Planck-Society.
The authors have no conflicting financial interests.
References
Articles from The Journal of Experimental Medicine are provided here courtesy of The Rockefeller University Press
Full text links
Read article at publisher's site: https://doi.org/10.1084/jem.20060565
Read article for free, from open access legal sources, via Unpaywall:
https://rupress.org/jem/article-pdf/203/7/1671/1157978/1671.pdf
Citations & impact
Impact metrics
Citations of article over time
Alternative metrics

Discover the attention surrounding your research
https://www.altmetric.com/details/102673893
Article citations
Ubiquitination of VE-cadherin regulates inflammation-induced vascular permeability in vivo.
EMBO Rep, 25(9):4013-4032, 07 Aug 2024
Cited by: 0 articles | PMID: 39112792 | PMCID: PMC11387630
Conditions that promote transcellular neutrophil migration in vivo.
Sci Rep, 14(1):14471, 24 Jun 2024
Cited by: 0 articles | PMID: 38914623 | PMCID: PMC11196655
The Basic Requirement of Tight Junction Proteins in Blood-Brain Barrier Function and Their Role in Pathologies.
Int J Mol Sci, 25(11):5601, 21 May 2024
Cited by: 1 article | PMID: 38891789 | PMCID: PMC11172262
Review Free full text in Europe PMC
Therapy-induced modulation of tumor vasculature and oxygenation in a murine glioblastoma model quantified by deep learning-based feature extraction.
Sci Rep, 14(1):2034, 23 Jan 2024
Cited by: 1 article | PMID: 38263339 | PMCID: PMC10805754
Factors Influencing Venous Remodeling in the Development of Varicose Veins of the Lower Limbs.
Int J Mol Sci, 25(3):1560, 26 Jan 2024
Cited by: 1 article | PMID: 38338837 | PMCID: PMC10855638
Review Free full text in Europe PMC
Go to all (145) article citations
Data
Data behind the article
This data has been text mined from the article, or deposited into data resources.
BioStudies: supplemental material and supporting data
Similar Articles
To arrive at the top five similar articles we use a word-weighted algorithm to compare words from the Title and Abstract of each citation.
Leukocyte transmigration in inflamed liver: A role for endothelial cell-selective adhesion molecule.
J Hepatol, 50(4):755-765, 07 Feb 2009
Cited by: 21 articles | PMID: 19231013
Endothelial cell-selective adhesion molecule regulates albuminuria in diabetic nephropathy.
Microvasc Res, 77(3):348-355, 24 Jan 2009
Cited by: 27 articles | PMID: 19323980
Endothelial cell-selective adhesion molecule in diabetic nephropathy.
Eur J Clin Invest, 42(11):1227-1234, 08 Sep 2012
Cited by: 10 articles | PMID: 22957687
Rho GTPases and leukocyte adhesion receptor expression and function in endothelial cells.
Circ Res, 98(6):757-767, 01 Mar 2006
Cited by: 99 articles | PMID: 16574916
Review