Abstract
Free full text

The yin and yang of intestinal epithelial cells in controlling dendritic cell function
Abstract
Recent work suggests that dendritic cells (DCs) in mucosal tissues are “educated” by intestinal epithelial cells (IECs) to suppress inflammation and promote immunological tolerance. After attack by pathogenic microorganisms, however, “non-educated” DCs are recruited from nearby areas, such as the dome of Peyer's patches (PPs) and the blood, to initiate inflammation and the ensuing immune response to the invader. Differential epithelial cell (EC) responses to commensals and pathogens may control these two tolorogenic and immunogenic functions of DCs.
The immune complexity of the gut
The principal functions of the mucosal epithelial barrier are to digest and absorb food nutrients, as well as to protect the body from dangerous microorganisms (1, 2). Our body is not fully equipped to transform all ingested food into its “recyclable” constituents, and we need the help of beneficial microorganisms for the digestion of complex macromolecules. These microbes, known collectively as the intestinal flora, have the difficult task of cohabiting and controlling each others' growth. Beneficial microorganisms also partially protect against pathogens by competing for metabolites, producing antimicrobial peptides, occupying epithelial/mucous niches, and preventing host–pathogen interactions; thus, it is our interest to tolerate them.
At the same time, however, immunity to dangerous microorganisms has to be initiated. Three main models have been proposed to account for how the immune system discriminates between and responds appropriately to commensal and pathogenic microorganisms. First, immune cells could discriminate between the two via engagement of different pattern-recognition receptors (PRRs) (3, 4). Second, ECs could “sense” the presence of noninvasive (commensal) versus invasive (pathogenic) microorganisms and transmit this information to antigen-presenting cells (APCs), such as DCs. Finally, APCs could be programmed to perform different tasks according to their tissue of origin, such that DCs or macrophages that are resident in the gut mucosa and regularly encounter commensal bacteria are tolerogenic, whereas cells that are recruited to the gut in response to pathogenic insult activate the immune response.
There is evidence to support each of these possibilities, and it is likely that all three mechanisms are involved in controlling the immune homeostasis of the gut. As the differential recognition of commensals versus pathogens by immune cells remains to be shown, here we will focus on the last two hypotheses. We will discuss the evidence that the DCs of the gut mucosa are predisposed to be tolorogenic, and that this characteristic is the result of their “education” by gut ECs. Unlike immune cells, there is growing evidence that gut ECs can discriminate between pathogens and commensals.
Unique functions of mucosal DCs
In the intestinal mucosa, DCs are found in two locations, the PPs and lamina propria (LP). In PPs, two important tasks are performed by DCs: uptake of antigen after its transcytosis across the follicle-associated epithelium (FAE), which is mediated by immature DCs located largely in the subepithelial dome, and T and B cell activation by mature DCs, which are found in the interfollicular T cell areas (IFRs) (Fig. 1). T and B cells activated in the PPs are “imprinted” to home back to the gut due to the unique ability of PP-DCs to induce lymphocytes to up-regulate expression of the intestinal homing integrin α4β7 and the chemokine receptor CCR9(5–8) via a retinoic acid–dependent mechanism (8, 9). Furthermore, PP-DCs promote the differentiation of the B cells into immunoglobulin (Ig)A-secreting cells (8, 10).
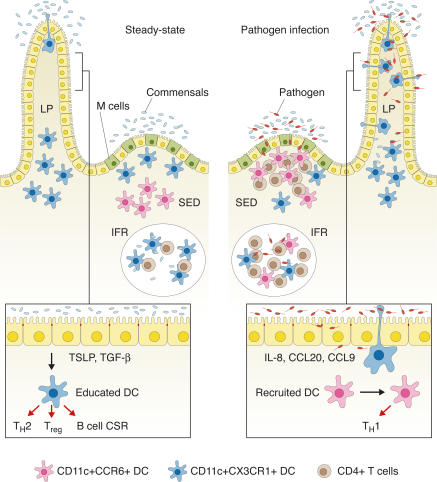
Gut mucosal immune cells involved in the “steady-state” and during infection. (Left) Steady-state. TSLP and possibly TGF-β released by ECs “condition” noninflammatory resident DCs (including the CX3CR1+ subtype), which extend processes into the intestinal lumen. These cells allow sampling and presentation of harmless commensal bacteria and may be involved in tolerance induction under homeostatic conditions or in innate defense in response to mucosal pathogens. These cells are generally noninflammatory and drive the development of Th2 or T reg cells and B cell class switch recombination (CSR). (Right) Pathogen infection. During infection, invasive pathogens can induce the release of inflammatory mediators, including IL-8 and CCL20. To initiate protective immunity, a pool of fully competent DCs able to respond quickly to bacteria is required. CCR6+ DCs in the PPs are a reservoir of non-educated DCs that can be rapidly recruited during infection.
Four different subsets of DCs have been described in mouse PPs (11, 12). All of them express the DC-specific cell marker CD11c but can be distinguished by their expression of the surface markers CD11b, CD8α, CCR6, and CX3CR1. These cell types have specialized functions: CD11b+CD8α−CCR6+ DCs activate primarily T helper (Th)2 T cells that produce interleukin (IL)-10, CD11b−CD8α+CCR6− DCs are localized in the T cell IFR and activate Th1-type responses, and CD11b−CD8α−CCR6+ DCs are located both in the subepithelial dome and in the IFR, and can also activate Th1-type responses (13). Recently, resident DCs expressing CX3CR1 (the receptor of CX3CL1, fraktalkine) have been described. They are scattered in the FAE but do not seem to participate in the induction of T cell responses (12). Two additional DC subsets are found in mesenteric lymph node and are characterized by the differential expression of CD4 and the lectin DEC-205 (14), but their functional significance remains to be elucidated. mesenteric lymph node DCs can also be distinguished by the expression of the integrin CD103, a marker of gut origin. CD103+ DCs have the unique ability to confer a CCR9+α4β7 gut-homing phenotype on CD8+ effector T cells (15) and Th cells (16), and to drive the development of CD4+CD25+Foxp3+ T cells via a retinoic acid–dependent mechanism (17, 18).
The mouse LP contains plasmacytoid DCs (pDCs) and two different subsets of myeloid (CD11c+CD11b+) DCs, which are distinguished by the expression of CD8α (19). Both human and mouse LP DCs respond to inflammatory stimuli but cannot initiate inflammatory reactions (19, 20). Myeloid LP DCs have been shown to take up luminal bacteria by extending dendrites between ECs, from the LP into the intestinal lumen (21). The extension of these processes relies on the expression of CX3CR1 (22) and in the small intestine depends on the presence of commensal bacteria, as antibiotic treatment markedly reduces the number of extensions (23). Further, whereas in the jejenum and proximal ileum DC processes are constitutively present, in the terminal ileum they are induced only after encounter with pathogens, such as Salmonella (23). This suggests that two different types of DCs can be found in the gut mucosa: those that are resident, which appear to be noninflammatory and tolerogenic, and those that are recruited after infection, which are likely involved in initiating inflammatory responses as discussed below.
IECs: regulators of the inflammatory response
The natural propensity of mucosal DCs to drive noninflammatory Th2-like immune responses does not seem to be an intrinsic property of all myeloid DCs, as CD11b+CD11c+ DCs isolated from PPs, but not from the spleen, drive the differentiation of Th2 T cells, even after exposure to strong Th1-inducing stimuli (24). Thus, the noninflammatory phenotype of mucosal DCs must be imparted by local differentiating factors. One source of such factors could be IECs, which are in close contact with LP DCs and have been shown to release molecules that influence the function of APC.
The cytokine thymic stromal lymphopoietin (TSLP) is one such molecule. Under steady-state conditions, TSLP is produced by the intestinal epithelium, and together with other EC-derived factors, it conditions DCs to induce noninflammatory Th2 responses by inhibiting the production of IL-12, a Th1-skewing cytokine (20). It has been proposed that steady-state concentrations of TSLP contribute to immune tolerance in the intestine by keeping gut DCs in a noninflammatory mode. In support of this idea, TSLP was not detected in the IECs of nearly 70% of Crohn's disease patients, suggesting that lack of TSLP-mediated control leads to gut inflammation (20). In addition, uncontrolled expression of TSLP production by keratinocytes or airway ECs is associated with Th2-driven pathologies, such as atopic dermatitis (25, 26) and allergic asthma (27, 28), suggesting that TSLP might also regulate immune homeostasis in other tissues. Furthermore, EC-derived TSLP is involved in amplifying Ig class switch by stimulating DCs to produce more B cell–activating factor (29).
Despite its role in promoting antibody- and Th2-associated immunity in the periphery, TSLP released by thymic ECs promotes the development of T regulatory (T reg) cells. TSLP is expressed by ECs of the Hassall's corpuscles and activates myeloid DCs in the thymic medulla. These activated DCs may provide strong survival and proliferation signals to self-reactive thymocytes, positively selecting them into the T reg cell lineage (30). TSLP-dependent T reg cell development may also take place in the gut. We found that human IEC-conditioned DCs are able to induce the generation of T reg cells from naive T cells via a TSLP-dependent mechanism (unpublished data). The ability of mucosal DCs to drive T reg cells does not seem to be restricted to conventional myeloid DCs, as Bilsborough et al. (31) have described a population of mucosally derived pDCs able to induce the differentiation of Th cells with regulatory functions. It would be interesting to evaluate whether these tolerogenic pDCs are also tissue conditioned.
TGF-β is another regulatory cytokine that has a direct effect on gut APCs. In response to local factors, gut LP mast cells and IECs release abundant latent TGF-β that binds to LP stroma (32) and undergoes activation by local proteases (33). Once activated, TGF-β modulates the activity of intestinal macrophages, which lose their ability to secret inflammatory cytokines in response to bacterial stimuli, but retain phagocytic functions (34). This is likely due to TGF-β–mediated alteration of NF-κB activation (35). TGF-β–dependent regulatory mechanisms have also been described in the lung, where alveolar ECs control the inflammatory state of alveolar macrophages under homeostatic conditions (36).
In addition to its suppressive effect on mucosa-associated macrophages, TGF-β is a potent inhibitor of DC activation. TGF-β–conditioned myeloid DCs appear be tolorogenic rather than immunogenic, as they do not respond to bacterial stimuli and can protect mice from lethal lipopolysaccharide-induced inflammation (37). And there is evidence that IEC-produced TGF-β may influence the function of mucosal DCs. EC-conditioned DCs produce more TGF-β, which could act in an autocrine fashion to suppress their activity (38). In agreement with this, blockade of TGF-β synthesis in EC-conditioned DCs results in increased proinflammatory cytokine production in response to ligands of the Toll-like receptors (TLRs).
Finally, prostaglandins (PGs), and in particular PGE-2, are also released by ECs and have been shown to modulate inflammation by influencing several levels of immune cell function (39). PGE-2 can inhibit the production of Th1 cytokines by T cells (40) and of IL-12 by DCs, thus driving the development of Th2 T cells (41).
Collectively, these observations suggest that EC-derived factors play a major role in controlling DC function and driving their tolerogenic phenotype, thus contributing to the homeostasis of the gut.
IECs as sensors of the external world
If mucosal APCs are programmed to deliver a tolerogenic signal to T cells, how is an accurate and prompt response mounted during pathogen attack? The discrimination between harmless and potentially harmful stimuli seems to be performed by the epithelium that first encounters luminal bacteria. Specifically, compartmentalization of PRRs to the basolateral membrane or into the cytosol of ECs may be a key mechanism to prevent responses to the abundant bacterial components in the gut lumen while preserving the ability to detect and mount responses against invasive pathogens that can cross the epithelium.
PRRs recognize conserved structures of bacteria and viruses and generally activate proinflammatory pathways. Two different families of PRRs are expressed in ECs: the TLRs and the nucleotide-binding oligomerization domain (Nod) molecules (Nod1, Nod2, and the recently described IPAF). Nod proteins are located intracellularly, whereas TLRs are typically expressed on the cell surface. Recently, however, it has been shown that TLR expression is regulated differently in the intestinal epithelium. Although still controversial, it has been reported that TLR2, TLR4, and TLR5, receptors for lipoprotein, lipopolysaccharide, and flagellin, respectively, are mostly localized intracellularly and at the basolateral membrane of IECs (42). In this way, only invasive pathogens, which are able to cross the cell monolayer, could activate an inflammatory response.
Nod proteins are similarly expressed in the intracellular compartment so they can only be activated by ligands that gain access to the cytosol. Pathogenic bacteria such as Salmonella typhimurium (43, 44) and Helicobacter pylori (45) that are equipped with type III or IV secretion systems can pump Nod ligands directly into the cytosol. Some invasive bacteria such as Listeria and Shigella evade the phagosome and are transmitted into the cytosolic compartment of adjacent cells, thereby making these microbes highly prone to Nod recognition. Consistent with the hypothesis that the altered location of PRRs in IECs allows them to distinguish between pathogenic bacteria and noninvasive commensals is the finding that TLR4 and TLR2 signaling from IECs, but not from DCs, is crucial for the extension of DC processes into the intestinal lumen upon S. typhimurium infection (21).
An alternative theory to explain the absence of inflammatory responses to commensal bacteria is that low amounts of TLR2 and TLR4 expressed on the apical surface of IECs could drive the development of so-called endotoxin tolerance, a process that confers nonresponsiveness to subsequent exposure to TLR ligands. In addition, commensals could aberrantly activate the NF-κB signaling pathway (46, 47). A prediction of this model is that PRR signaling could be cytoprotective. Indeed, TLR signaling by commensals is required to preserve the epithelial barrier (48), most likely via activation of NEMO (49) and PGE-2 production (50). It is also possible that engagement of TLRs on the apical membrane of ECs without simultaneous engagement of intracellular receptors prevents activation of the inflammatory response.
IEC activation drives the development of inflammatory responses
Differential recognition of pathogenic versus commensal bacteria by IEC results in two distinct outcomes (Fig. 1). In the absence of inflammation, resident immune cells are noninflammatory and participate in the maintenance of gut homeostasis. We found that “IEC-conditioned” DCs are blocked irreversibly in their ability to release IL-12 and to activate Th1 T cells, and cannot produce inflammatory mediators even after encounter with pathogenic bacteria such as Salmonella (51).
In contrast, when invasive or pathogenic bacteria can deliver their PRR ligands intracellularly they initiate an inflammatory response in IECs, which then release inflammatory cytokines and chemokines (51). This culminates in the recruitment of non-educated phagocytes, including DCs, which are absolutely required to initiate anti-Salmonella–specific T cell responses (12). CCL20 (52) or a still undefined chemokine could be involved in the recruitment of CCR6-expressing immature DCs from the PP. Salazar-Gonzalez et al. (12) showed that after bacterial infection, CCR6+ DCs are rapidly recruited within the FAE a few hours after oral Salmonella infection and are critical for the initiation of anti-Salmonella immune responses. CCR6+ DCs are different from local immature DCs, which express CX3CR1, but not CCR6, and are located in close proximity with M cells.
Collectively, these findings suggest that in the gut it is possible to distinguish between resident DCs, such as the CX3CR1+ cells that are sentinels of the intestinal lumen, and allow sampling and presentation of harmless commensal bacteria. These cells may be involved in tolerance induction under homeostatic conditions or in innate defense against mucosal pathogens, but are generally noninflammatory. To initiate protective immunity, a pool of fully competent DCs able to respond quickly and properly to bacteria is required. CCR6+ DCs in the PPs are a reservoir of non-educated DCs that can be rapidly recruited should an infection occur. The equivalent of CCR6+ cells in the LP still needs to be identified.
In conclusion, the homeostasis of the gut is maintained via a continuous control of DC function by EC- and presumably also stromal cell–derived factors. This interaction generates noninflammatory and tolerogenic DCs that promote the development of Th2 responses, T reg cells, and B cell class switch recombination. Altogether, these responses allow us to tolerate commensal bacteria and food antigens and keep inflammation at bay. Deregulation of these mechanisms can lead to chronic inflammation and immune-related gut disorders like inflammatory bowel disease and cancer.
Notes
I.D. Iliev, G. Matteoli, and M. Rescigno are at Department of Experimental Oncology, European Institute of Oncology, 20141 Milan, Italy.
References
Articles from The Journal of Experimental Medicine are provided here courtesy of The Rockefeller University Press
Full text links
Read article at publisher's site: https://doi.org/10.1084/jem.20062535
Read article for free, from open access legal sources, via Unpaywall:
https://rupress.org/jem/article-pdf/204/10/2253/1128933/jem_20062535.pdf
Citations & impact
Impact metrics
Citations of article over time
Smart citations by scite.ai
Explore citation contexts and check if this article has been
supported or disputed.
https://scite.ai/reports/10.1084/jem.20062535
Article citations
The impact on colostrum oxidative stress, cytokines, and immune cells composition after SARS-CoV-2 infection during pregnancy.
Front Immunol, 13:1031248, 15 Dec 2022
Cited by: 1 article | PMID: 36591280 | PMCID: PMC9798093
Immune Tolerance in the Oral Mucosa.
Int J Mol Sci, 22(22):12149, 10 Nov 2021
Cited by: 8 articles | PMID: 34830032 | PMCID: PMC8624028
Review Free full text in Europe PMC
Oral Versus Gastrointestinal Mucosal Immune Niches in Homeostasis and Allostasis.
Front Immunol, 12:705206, 05 Jul 2021
Cited by: 22 articles | PMID: 34290715 | PMCID: PMC8287884
Review Free full text in Europe PMC
Maternal Supplementation of Food Ingredient (Prebiotic) or Food Contaminant (Mycotoxin) Influences Mucosal Immune System in Piglets.
Nutrients, 12(7):E2115, 17 Jul 2020
Cited by: 2 articles | PMID: 32708852 | PMCID: PMC7400953
A Polysaccharide Isolated from Codonopsis pilosula with Immunomodulation Effects Both In Vitro and In Vivo.
Molecules, 24(20):E3632, 09 Oct 2019
Cited by: 20 articles | PMID: 31600890 | PMCID: PMC6832355
Go to all (61) article citations
Similar Articles
To arrive at the top five similar articles we use a word-weighted algorithm to compare words from the Title and Abstract of each citation.
Differential crosstalk between epithelial cells, dendritic cells and bacteria in a co-culture model.
Int J Food Microbiol, 131(1):40-51, 04 Mar 2009
Cited by: 38 articles | PMID: 19264370
How intestinal epithelial cells tolerise dendritic cells and its relevance to inflammatory bowel disease.
Gut, 58(9):1291-1299, 01 Sep 2009
Cited by: 27 articles | PMID: 19671557
Review
Uptake and presentation of orally administered antigens.
Vaccine, 23(15):1793-1796, 01 Mar 2005
Cited by: 15 articles | PMID: 15734043
Review
Phenotype and function of intestinal dendritic cells.
Semin Immunol, 17(4):284-294, 01 Aug 2005
Cited by: 61 articles | PMID: 15978836
Review