Abstract
Free full text

Epimorphin Functions as a Key Morphoregulator for Mammary Epithelial Cells
Abstract
Hepatocyte growth factor (HGF) and EGF have been reported to promote branching morphogenesis of mammary epithelial cells. We now show that it is epimorphin that is primarily responsible for this phenomenon. In vivo, epimorphin was detected in the stromal compartment but not in lumenal epithelial cells of the mammary gland; in culture, however, a subpopulation of mammary epithelial cells produced significant amounts of epimorphin. When epimorphin-expressing epithelial cell clones were cultured in collagen gels they displayed branching morphogenesis in the presence of HGF, EGF, keratinocyte growth factor, or fibroblast growth factor, a process that was inhibited by anti-epimorphin but not anti-HGF antibodies. The branch length, however, was roughly proportional to the ability of the factors to induce growth. Accordingly, epimorphin-negative epithelial cells simply grew in a cluster in response to the growth factors and failed to branch. When recombinant epimorphin was added to these collagen gels, epimorphin-negative cells underwent branching morphogenesis. The mode of action of epimorphin on morphogenesis of the gland, however, was dependent on how it was presented to the mammary cells. If epimorphin was overexpressed in epimorphin-negative epithelial cells under regulation of an inducible promoter or was allowed to coat the surface of each epithelial cell in a nonpolar fashion, the cells formed globular, alveoli-like structures with a large central lumen instead of branching ducts. This process was enhanced also by addition of HGF, EGF, or other growth factors and was inhibited by epimorphin antibodies. These results suggest that epimorphin is the primary morphogen in the mammary gland but that growth factors are necessary to achieve the appropriate cell numbers for the resulting morphogenesis to be visualized.
Dynamic and reciprocal communication between epithelial and stromal compartments is a critical component of epithelial morphogenesis (Grobstein, 1953; Spooner and Wessells, 1970; Kratochwil, 1983; Saxsen and Sariola, 1987; Sakakura, 1991). Recombination experiments of stromal and epithelial tissues from different origin have indicated that the stroma can dictate both the growth of epithelia and the resulting morphological pattern. For instance, salivary epithelium that was combined with mammary stroma developed a mammary-like ductal tree system, and mammary epithelium combined with salivary mesenchyme developed a salivary gland-like pattern (Sakakura et al., 1976). Attempts to understand the underlying molecular mechanism have identified morphoregulatory molecules that are preferentially expressed by the stroma. These include cell surface molecules such as nerve growth factor receptor and the ganglioside GD-3 (Sariola et al., 1988, 1991), extracellular matrix (ECM)1 molecules such as nidogen/entactin and tenascin (Ekblom et al., 1994; Young et al., 1994) and growth factors such as hepatocyte growth factor (HGF)/scatter factor (Montesano et al., 1991b ; Soriano et al., 1995), neu differentiation factor/heregulin (HRG) (Yang et al., 1995), and keratinocyte growth factor (KGF)/FGF-7 (Simonet et al., 1995). Recently HGF has emerged as an ubiquitous and central regulator of epithelial morphogenesis in various tissues, including the mammary gland (Brinkmann et al., 1995). Synthesis of HGF by the stroma of the mammary gland is developmentally regulated during ductal and alveolar branching morphogenesis in puberty and pregnancy, whereas HGF receptor c-met is expressed by the epithelium throughout development (Yang et al., 1995). EGF has been shown also to be a potent morphogen in the mammary gland (Tonelli and Sorof, 1980; Coleman et al., 1988). In contrast to HGF, EGF and its receptor are expressed by both epithelial and stromal cells and also participate in regulation of both ductal and alveolar morphogenesis (Snedeker et al., 1991).
Epimorphin was isolated as a stromal protein mediating morphogenesis of embryonic skin and lung (Hirai et al., 1993). Epimorphin is a member of a gene family that comprises SED5, Pep12, Sso1p, Sso2p, and syntaxins 1A (HPC-1), 1B, and 3-5 (Pelham, 1993). Members of this family were shown to be involved in vesicle trafficking between Golgi, endoplasmatic reticulum, and plasma membrane and lack a signal peptide for secretion. Nevertheless, some antibodies to syntaxin 1A react with living cultured cells (Inoue et al., 1992) and syntaxin 1B functions as a glutamic acid receptor at the cell surface in addition to its intracellular function (Smirnova et al., 1993a ,b). Analogous to syntaxin 1A and B, epimorphin is localized both in the cytoplasm and at the extracellular surface of the plasma membrane (Hirai, 1994; Butt et al., 1996). Some isoforms of epimorphin might be anchored in the plasma membrane by a putative membrane-spanning region, the expression of which is modulated by alternative splicing (Bennett et al., 1993; Hirai, 1993). Other forms of this molecule are generated by intermolecular interactions within the coiled-coil domains of epimorphin giving rise to dimers and tetramers (Yoshida et al., 1992; Hirai, 1994). The latter are preferentially expressed on the cell surface, whereas the epimorphin monomer is most abundant in the cytoplasm. The existence of both intracellular and extracellular forms of epimorphin raises the question of whether epimorphin affects epithelial morphogenesis by modulating the secretion of stromal morphregulatory molecules, or through interaction of extracellular epimorphin with epithelial cells.
To understand the role of extracellular epimorphin in morphogenesis of the mammary gland in the context of a microenvironment containing growth factors and stromal collagen, we analyzed its effect on morphological differentiation of mammary epithelial cells embedded in collagen gels. We show here that it is epimorphin that acts as a key morphoregulatory molecule for mammary epithelial cells, and that HGF, EGF, and other growth factors assist in epimorphin-dependent morphogenesis, approximately proportional to their growth-stimulating activity.
Materials and Methods
Cells
The functionally normal and nontumorigenic mouse mammary epithelial cell lines CID-9 (Schmidhauser et al., 1990), SCp2 (Desprez et al., 1993) and EpH4 (a gift from Dr. Reichmann, Institute Suisse de Recherches, Switzerland; Reichmann et al., 1989; Brinkmann et al., 1995) were maintained in DME/F12 supplemented with 5% heat-inactivated FCS, 5 μg/ml insulin (Sigma Chemical Co., St. Louis, MO) and 50 μg/ml gentamicin (Life Technologies, Ltd., Paisley, Scotland; growth medium). For generation of SCp2 and EpH4 cell lines overexpressing extracellular epimorphin under control of a tetracycline-regulated promoter, the signal peptide sequence of interleukin-2 (ATGTACAGCATGCAGCTCGCATCCTGTGTCACATTGACACTTGTGCTCCTTGTCAACAGCGCTCCC) was connected to the NH2 terminus of a cDNA coding for full length epimorphin isoform I (Hirai et al., 1992) and cloned into the HindIII restriction site of the eukaryotic expression vector pTeT-splice (Life Technologies, Ltd.). Cells (5 × 105) were transfected with 5 μg of the above construct, 5 μg of pTet.tTAK vector (Life Technologies, Ltd.) coding for the tTAK protein, which suppresses transcription of the transgene in the presence of tetracycline, and 0.5 μg of pSV40neo containing a neomycin resistance cassette (Schmidhauser et al., 1992) in 1 ml of Opti-MEM (Life Technologies, Ltd.) using Lipofectin (Life Technologies, Ltd.), according to the manufacturer's instructions. After selection of neomycin resistant clones in the continuous presence of tetracycline, the expression of epimorphin was analyzed by Western blotting, in the presence and absence of 5 μg/ml tetracycline. PTSEd and PTSEe, which expressed epimorphin transgene after removal of tetracycline from the culture medium, and PTSEa and PTSEb, which did not express epimorphin with and without tetracycline, were isolated from SCp2 cells; ETSEII, which inducibly expressed exogenous epimorphin, was isolated from the epimorphin-negative population of EpH4 cells.
Generation of Recombinant Epimorphin
Recombinant full-length epimorphin (H123) is identical to endogenous epimorphin lacking membrane-spanning region and represents all isoforms (see Fig. Fig.33 A). Recombinantly generated epimorphin fragments H2 and H13 represent the cellular recognition domain of epimorphin (amino acids 104–187) and a fusion of NH2- (amino acids 1–103) and COOH- (amino acids 188–264) terminal coiled-coil domains of epimorphin, respectively. They were tagged with six histidine residues, expressed in Escherichia coli and purified over Ni columns in the presence of urea as described (Oka and Hirai, 1996). Urea was necessary, since all recombinant products precipitated immediately upon removal of urea under the neutral pH. For use in cell culture, recombinant epimorphin was dialyzed against 1.5 mM HCl and filtered under sterile conditions.

Preparation of recombinant epimorphin. (A) Schematic diagram of epimorphin isoforms and the recombinant epimorphin fragments used in this study. There are three epimorphin isoforms produced by alternative splicing. Isoforms I and II (34-kD products) have a putative membrane-spanning region at the COOH terminus, whereas isoform III (31-kD products) has not. The recombinant epimorphin fragment H123 represents the epimorphin sequence shared by all the isoforms. H13 is a fusion peptide of the NH2- and COOH-terminal coiled-coil domains of H123, and H2 is a peptide of only the cellular recognition domain inserted between the coiled-coil domains. *, NH2-terminally tagged histidine residues. (B) Coomassie brilliant blue–stained epimorphin fragments in SDS-PAGE gel.
Antibodies
Affinity-purified antibodies to epimorphin were prepared as follows: rabbit antiserum against untagged recombinant epimorphin (Hirai, 1994) was mixed with an equal volume of PBS, pH 7.4, precipitated with 40% ammonium sulfate, dissolved in PBS, and dialyzed against PBS. Subsequently, antibodies were adsorbed to nitrocellulose membranes (Millipore Corp., So. San Francisco, CA) precoated with 1 mg/ml histidine-tagged epimorphin by incubation for 4 h at ambient temperature. After several washes with PBS, antibodies bound to recombinant epimorphin were eluted with 0.25 M glycine-HCl, pH 2.7. Affinity-purified anti-epimorphin antibody was immediately neutralized with 1 M phosphate buffer, pH 8.0, precipitated with 60% ammonium sulfate and dissolved in DME/F12 (Life Technologies, Ltd.). Epimorphin antibodies were then dialyzed against DME/F12 and filtered under sterile conditions. Purified rabbit IgG (DAKO Corp., Glostrup, Denmark) were dialyzed against DME/F12, sterile filtered, and used as the control.
Mouse anti-vimentin, mouse anti–α-smooth muscle actin, FITC-conjugated sheep anti–mouse IgG, and rhodamine-conjugated goat anti–rabbit IgG antibodies were purchased from Sigma Chemical Co. FITC-conjugated goat anti–rat IgG antibodies were from Caltag Labs (So. San Francisco, CA). HRP-conjugated donkey anti–rabbit Ig antibodies were from Amersham Corp. (Buckinghamshire, U.K.). Function blocking anti-HGF antibodies were from Sigma Chemical Co. Rat monoclonal antibody against E-cadherin, ECCD2 (Shirayoshi et al., 1986), was a generous gift from Dr. Takeichi (Kyoto University, Japan).
Cell Attachment and Proliferation Assays
Cell attachment assays were carried out as previously described (Oka and Hirai, 1996), with minor modifications. In brief, each well of 24-well plates (nontreated for cell culture; Falcon, Becton Dickinson Labware, Franklin Lakes, NJ), was coated with 5 μg/cm2 of recombinant epimorphin (H123), epimorphin fragments H2 or H13 in 1.5 mM HCl, and subsequently air dried. Cells (2 × 104) were plated in DME/F12 medium containing 4 mg/ ml of BSA onto epimorphin-coated substrata and incubated at 37°C. Anti-epimorphin antibodies and control antibodies, at a total concentration of 20 μg/ml, were added to medium. After an 8-h incubation, plates were tapped sharply and washed twice with PBS. Cells bound to the plates were then detached after trypsinization and counted. Wells uncoated and coated with 5 μg/cm2 type I collagen (Cellagen™ ; ICN, Irvine, CA) in 1.5 mM HCl were used as negative and positive controls. All experiments were conducted in duplicate and repeated three times.
For proliferation assays, cells (1 × 105) were seeded into each well of 12-well plates coated with epimorphin fragments, as described above. After 8 h of incubation, replicate cultures of attached cells were washed, detached, and counted (N0). Cells attached in similar numbers regardless of whether wells were precoated with H123, H2, or collagen type I. Growth medium was added to remaining wells and incubated for an additional 2 d. After such time, cell number was determined (N2) and growth ratio (N2/ N0) was calculated. The proliferation assay was also carried out in the presence of 50 ng/ml recombinant HGF (Becton Dickinson, Bedford, MA) or 50 ng/ml EGF (Sigma Chemical Co.). The proliferation assays were performed in duplicate and repeated three times.
To measure the effects of HGF, EGF, KGF, and FGF on proliferation of cell clusters in collagen gels (see three-dimensional cultures, below), the diameters of >50 cell clusters cultured for 8 d with one of these growth factors (50 ng/ml) were measured and compared to those of control.
Preparation of Cell Clusters
The cell clusters were prepared as follows: agarose was heated in PBS (final 2%) and 250 μl of the solution was added to each well of 24-well plates. After the agarose gelled, 1.5 ml of growth medium was added to each well and incubated for 30 min at 37°C in CO2 incubator. This medium was then discarded and 5 × 104 cells, suspended in 500 μl of growth medium containing 1,000 U of desoxyribonuclease I (DNase I; Sigma Chemical Co.), were seeded on top of the agarose gel and incubated with gentle rotation (100 rpm) for 24 h at 37°C. After incubation, cells formed smoothly rounded and well packed clusters of 150 to 250 μm diam. The remaining single cells were removed by centrifugation for 30 s at 200 rpm. To obtain clusters of primary mammary cells, a cell suspension of glands from 12 to 15 d pregnant mice was prepared as follows: the glands were minced with a sharp razor blade and incubated with 0.2% trypsin (Life Technologies, Ltd.) and 0.2% collagenase I (Life Technologies, Ltd.) in DME/F12 with agitation (100 rpm) for 30 min at 37°C, followed by centrifugation at 1,000 rpm for 3 min. After discarding the fat-containing supernatant, cells in the pellet were resuspended in growth medium supplemented with 1,000 U of DNase I and incubated for 2 min. Cells were then washed three times with growth medium.
Three-Dimensional Cultures
For three-dimensional cell cultures, cell clusters were embedded in type I collagen gels. The embedding process into collagen gels was similar to that described for single cells by Montesano et al. (1991a). Acid-soluble collagen (7.5 vol of a 0.5% solution; Cellagen™ AC-5; ICN) was mixed gently on ice with 1 vol of 1.5 mM HCl (blank solution) and 1 vol of 10× DME/ F12, followed by mixing with 0.5 vol of alkaline solution to neutralize the pH. After adding 1:20 vol of FCS, 250 μl of the collagen solution was poured into each well of a 24-well dish, and the dish was incubated at 37°C to allow gelation of the basal collagen layer. The cell clusters were suspended in growth medium at a concentration of 2,500 to 4,000 clusters/ml, and 10 μl of the suspension (25–40 clusters) was mixed with 250 μl of ice-cold collagen solution, poured onto the basal collagen layer, and placed immediately at 37°C. To prepare collagen substrate containing recombinant epimorphin or control peptides, one vol of a 1 mg/ml stock solution of H123 or its fragments (H2 or H13) in 1.5 mM HCl was used instead of the blank solution. To prepare cell clusters in which recombinant epimorphin were present around each cell, cells were precultured on H123- or H2-coated plates for 4 d, as described in Cell Attachment and Proliferation Assay before making clusters (see Fig. Fig.77 B). After gelation of collagen, 500 μl of growth medium was added to each well. Medium was exchanged every 5 d. HGF, EGF, KGF (Sigma Chemical Co.), or bFGF (Sigma Chemical Co.) were added to replicate wells at a concentration of 50 ng/ml. Blocking antibodies to epimorphin, HGF (Sigma Chemical Co.) or control antibodies were added to replicate wells at a concentration of 100 μg/ml.
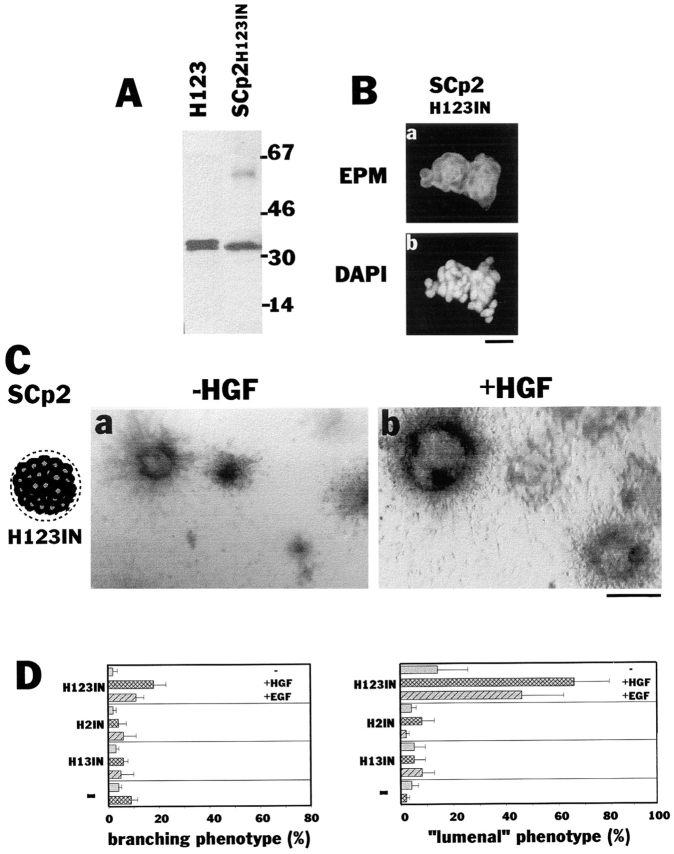
Nonpolar presence of recombinant epimorphin around each cell of the cluster leads to “lumen” formation. (A) Immunoblot detection of recombinant epimorphin in the clusters of SCp2 cells. (Right lane) Clusters of SCp2 cells precultured on H123 for 4 d (SCp2H123IN). (Left lane) Purified H123 (5 ng). (B) Detection of recombinant epimorphin (H123) in the cell clusters. Sections of the clusters were stained with anti-epimorphin antibodies (a) and with DAPI (b). (C) Appearance of the clusters of H123-containing SCp2 cells (SCp2 H123IN) in collagen gels, cultured for 8 d in the absence (a) or presence (b) of 50 ng/ml HGF. (D) Quantification of the branching and “lumenal” phenotype of the clusters of SCp2. Clusters of cells precultured on H123, H2, or H13 are shown as H123IN, H2IN, or H13IN, respectively. Note that H123 but not H2 and H13 fragments in the cluster induced “lumen” formation. EGF as well as HGF dramatically enhanced the effect of H123. Bars: (B) 120 μm; (C) 200 μm.
Analysis of the Morphogenic Phenotypes of Cell Clusters
The phenotype of cell clusters embedded in collagen gels was determined after cultivation for 8 d. A cluster was defined as a “branching” phenotype if it had at least three independent extending cell processes that were longer than the diameter of the cell cluster. A cluster was defined as a “lumenal” phenotype if it had a clearly visible lumen occupying >50% of the volume of cluster. There were few clusters that had the double phenotype; these were scored in each category. The quantification of the phenotypic appearance was carried out by counting the percentage of a particular phenotype from at least 50 randomly selected clusters in each experiment. The experiments were repeated at least three times.
Western Blotting and Immunohistochemistry
Western blotting for detection of epimorphin was carried out as described previously (Hirai, 1994). In brief, cells were lysed with Laemmli sample buffer (Laemmli, 1970), and proteins were separated on 4 to 20% SDS– polyacrylamide gradient gels and subsequently blotted onto polyvinyllidene difluoride membranes (Immobilon P; Millipore Corp.). Membranes were then washed with TBS (50 mM Tris, pH 7.5, 100 mM NaCl), and treated successively with 5% skim milk in TBS (STBS) for 1 h, 1:150 diluted anti-epimorphin antibodies in STBS for 1 h and 1/1,000 diluted HRP- labeled anti–rabbit antibodies in STBS for an additional hour, with excessive washing with TBS in each interval. Labeled epimorphin was visualized with enhanced chemiluminescence (ECL) reagent (Amersham Corp.) according to the manufacturer's instructions.
Immunohistochemistry was carried out as described previously (Oka and Hirai, 1996). In brief, 10-μm cryosections of tissues or cell clusters were treated successively with STBS, first antibody solution in STBS and FITC-, rhodamine-, or HRP-labeled second antibody solution in STBS, with excessive washing with TBS in each interval. To visualize HRP- labeled epimorphin, sections were treated with 0.69 mM 3,3′-diaminobenzidine (Sigma Chemical Co.) and 0.3 mM H2O2 dissolved in TBS. Sections were counterstained with 4,6-diamidino-2-phenyl-indole (DAPI; Sigma Chemical Co.) or hematoxylin (Sigma Chemical Co.). Antibodies to epimorphin, vimentin, α-smooth muscle actin, or E-cadherin (ECCD-2) were diluted to 1:100, 1:100, 1:300, or 1:300, respectively, and used for primary antibody treatment. The FITC-, rhodamine-, or HRP-labeled secondary antibodies to rabbit, mouse, and rat Ig were diluted to 1:150.
Results
Localization of Epimorphin in the Mouse Mammary Gland and in Mouse Mammary Cell Lines
In fetal skin, lung, and submandibular gland, epimorphin expression is restricted to the stromal tissue compartment (Hirai et al., 1992; Kadoya et al., 1995). In the postnatal mammary gland, epimorphin was detected between fibroblasts and adipocytes and around ducts and alveoli in virgin, pregnant, and lactating mice (Fig. (Fig.11 A). The pattern of immunostaining suggested that epimorphin was not expressed by lumenal epithelial cells, but by fibroblasts and possibly by myoepithelial cells. Indeed, when isolated cells from the mammary gland were examined for epimorphin expression, only fibroblasts and some myoepithelial cells, but not lumenal epithelial cells, were stained for epimorphin, as determined by double labeling of cells with antibodies to epimorphin and E-cadherin, α-smooth muscle actin, and vimentin, markers for, respectively, lumenal epithelial cells (Daniel et al., 1995), myoepithelial cells (Deugnier et al., 1995), and fibroblasts (Dulbecco et al., 1983) (Fig. (Fig.11 B). To ascertain whether epimorphin was present extracellularly at the surface of mammary cells, anti-epimorphin antibodies were added to clusters of living primary mammary cells for 2 h on ice, washed several times with growth medium, and embedded for cryosections. As a negative (intracellular) control for this assay, anti- vimentin antibodies and FITC-labeled anti–mouse Ig were mixed with anti-epimorphin antibodies and rhodamine- labeled anti–rabbit Ig, respectively. Labeling of the living cells with anti-epimorphin antibodies demonstrated that epimorphin was present also extracellularly (Fig. (Fig.11 B, d). We then examined expression of epimorphin in three mouse mammary epithelial cell lines, CID-9, SCp2, and EpH4, that have been used previously to study tissue-specific gene expression and morphogenesis of mammary epithelial cells in culture (Schmidhauser et al., 1990; Desprez et al., 1993; Brinkmann et al., 1995). In CID-9 cells, which consist of both epithelial and “fibroblastic” cells (Schmidhauser et al., 1990; Desprez et al., 1993), all vimentin-expressing cells were epimorphin positive (Fig. (Fig.2,2, a–c), in agreement with our observations with primary cells isolated from the mammary gland (Fig. (Fig.11 B). However, a minority of vimentin-negative cells also expressed epimorphin. This was also the case for SCp2, a clonal epithelial cell line derived from CID-9 (Desprez et al., 1993) and especially for EpH4, an independent cell line isolated by Reichmann et al. (1989). Although neither cell line expressed vimentin, 60–70% of EpH4 and ~5% of SCp2 cells expressed epimorphin (Fig. (Fig.2,2, d–i). Epimorphin-expressing SCp2 and EpH4 cells were cytokeratin and E-cadherin positive but did not express α-smooth muscle actin (not shown), indicating that some lumenal epithelial cells do turn on epimorphin gene expression in culture.

Expression of epimorphin in the mammary gland. (A) Localization of epimorphin in the sections of glands from virgin (a and b), mid-pregnant (c), and lactating (d) CD-1 mice. The area boxed in black in a is shown at higher magnification in b. (B) Detection of epimorphin-producing cell types. Frozen sections of mammary glands from mid-pregnant mice were immunostained with anti-epimorphin antibodies and rhodamine-labeled secondary antibodies (red). Sections were also stained for E-cadherin (a), α-smooth muscle actin (b), or vimentin (c) using their specific antibodies and FITC-labeled secondary antibodies (green). Nuclei were visualized with DAPI (blue). (d) Living cells were treated with a mixture of anti-epimorphin and anti-vimentin antibodies, and labeled antibodies were visualized with rhodamine- (for epimorphin) and FITC- (for vimentin) labeled secondary antibodies. Note that both anti-epimorphin and vimentin antibodies stained sections (c), but only the former labeled the living cells, indicating epimorphin is localized also at the cell surface (d). Bars, 120 μm.
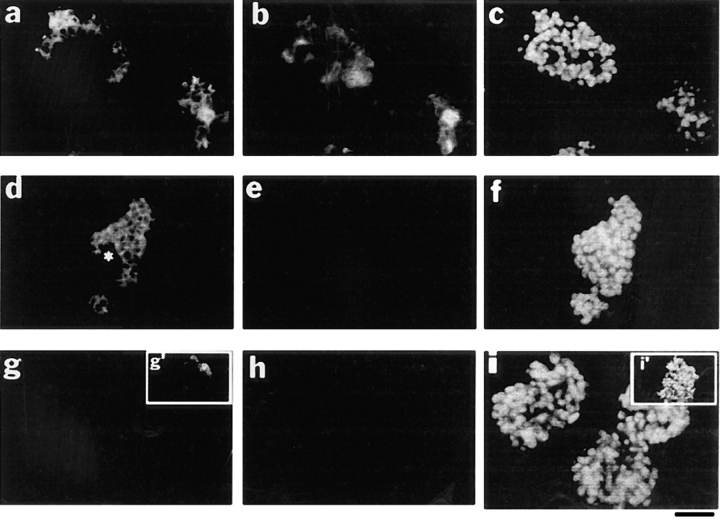
Expression of epimorphin in epithelial model cells. Sections of cell clusters of CID-9 (a–c), EpH4 (d–f), and SCp2 (g–i) were stained simultaneously for epimorphin (a, d, and g), vimentin (b, e, and h), and DAPI (c, f, and i). *, epimorphin-negative subpopulation of EpH4. Note that 30–40% of CID-9 cells and 60–70% of EpH4 cells expressed epimorphin. Almost all SCp2 cells were negative for epimorphin but a minor subpopulation (~5%) expressed epimorphin (g′ and i′). Bar, 120 μm.
Effect of Epimorphin on Attachment and Proliferation of Mammary Epithelial Cells
Given that mammary lumenal epithelial cells in vivo do not express epimorphin but are surrounded by epimorphin-positive cell populations, one could postulate the existence of epimorphin receptors (not yet characterized) if epimorphin were to act directly on epithelium. We asked whether mammary epithelial cells in culture can interact with epimorphin. Since SCp2 cells were largely devoid of endogenous epimorphin, as are mammary epithelial cells in vivo (Fig. (Fig.2,2, g–i), these cells were tested for their ability to bind to purified recombinant epimorphin (Fig. (Fig.3).3). About 80% of plated SCp2 cells attached to substratum-bound recombinant epimorphin within 8 h (Fig. (Fig.4,4, A and B). Attachment of cells to epimorphin was as efficient as their attachment to type I collagen. As was shown previously for endothelial cells (Oka and Hirai, 1996), cells attached to the H2 cell-binding domain of epimorphin and to full-length epimorphin H123 but not to the coiled-coil domain H13 (Fig. (Fig.44 B). Binding of cells to epimorphin-coated plates could be inhibited with anti-epimorphin antibodies in a dose-dependent fashion, whereas binding to type I collagen could not. These observations suggest that SCp2 cells can interact with extracellularly presented epimorphin.
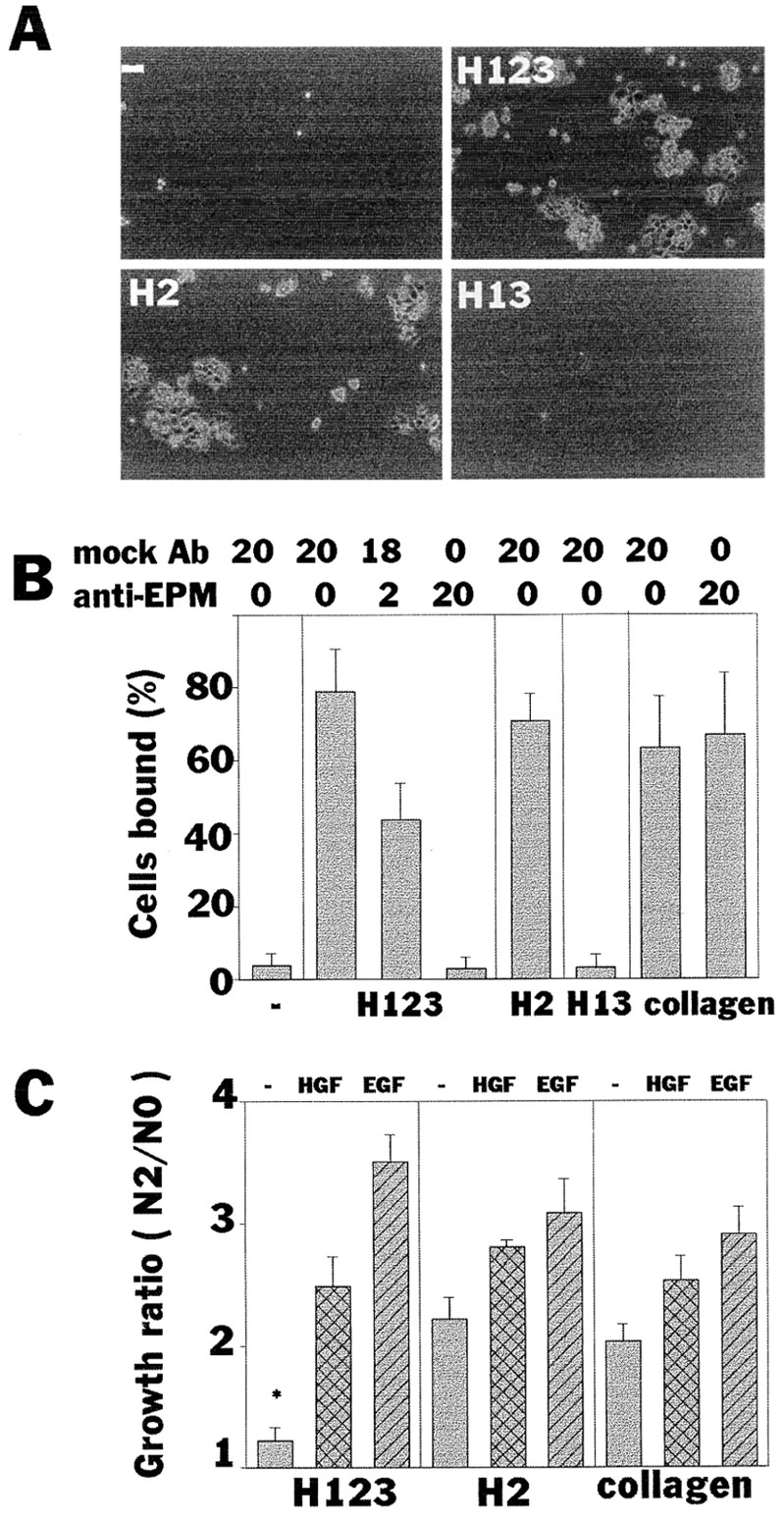
Attachment and proliferation of an epithelial cell line on recombinant epimorphin. (A and B) Attachment of SCp2 cells to substrate-coated epimorphin fragments. Cells bound to each epimorphin fragment in 8 h were photographed (A) and quantified (B). Uncoated (−) and collagen type I–coated wells were used as negative and positive controls, respectively. Medium contained mock antibodies and anti-epimorphin antibodies at a total concentration of 20 μg/ml as indicated. P values between − and H123 (mock Ab20), and H123 (mock Ab20) and H123 (anti-EPM20) were <0.0001. (C) The growth ratios (cell number in 2 d plus 8 h divided by cell numbers 8 h after plating) of SCp2 cells cultured on type I collagen, H123, and H2 fragments. HGF and EGF were tested at 50 ng/ml. Significant inhibition of cell growth was observed in cells cultured on H123 in the absence of additional growth factors. *, P values versus H2 (−) and collagen (−) were <0.001.
When cells were maintained in the presence of serum-containing medium, substratum-bound epimorphin H123, but not its cell-binding H2 domain alone, slightly inhibited proliferation of SCp2 cells (Fig. (Fig.44 C), as was seen previously in endothelial cells (Oka and Hirai, 1996). When HGF or EGF were added to the culture medium, cells overcame the inhibitory effects of epimorphin and continued to grow (Fig. (Fig.44 C).
Effect of Epimorphin on Branching Morphogenesis of Mammary Epithelial Cells
To analyze the effect of epimorphin on morphogenesis of mammary epithelial cells, clustered SCp2 cells were embedded in collagen gels containing recombinant epimorphin. Cell clusters rather than individual cells were used in this assay because of epimorphin's growth inhibitory effect (Fig. (Fig.44 C).
As shown in Fig. Fig.5,5, A and B, ~20% of cell clusters of SCp2 cells exhibited branching morphogenesis in the presence of epimorphin (H123), compared to ~5% in the absence of epimorphin. HGF, in the absence of epimorphin, accelerated growth but had no effect on morphogenesis of SCp2 cells (Fig. (Fig.55 A, d, and B). However, the percentage of cell clusters undergoing visible branching morphogenesis was markedly increased to 80% when cells were stimulated by both epimorphin and HGF. The H2 cell-binding domain of epimorphin alone was insufficient to induce branching morphogenesis (Fig. (Fig.55 B). We asked whether or not HGF was necessary or whether another growth factor could replace its branching function. EGF, KGF, and FGF also promoted growth of SCp2 cell, and morphogenesis was stimulated only when epimorphin was also present (Fig. (Fig.5,5, C and D). The branch length was roughly proportional to the ability of the factor to induce growth (Fig. (Fig.5,5, C and D). Anti-HGF function-blocking antibodies did not prevent branching if HGF was replaced by EGF and if epimorphin was present. These observations demonstrate that epimorphin positions the cells to undergo branching morphogenesis in response to several growth factors.
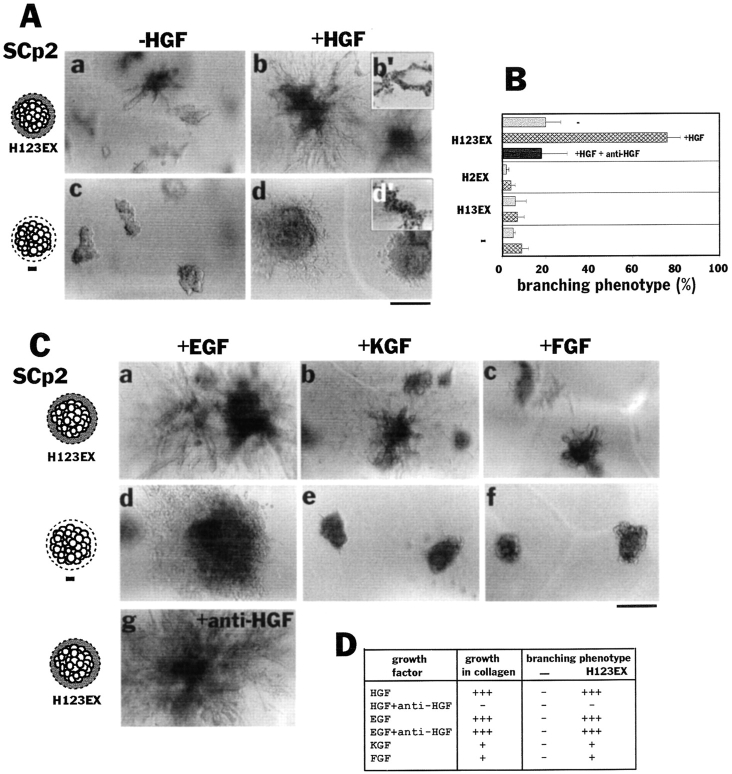
Effect of recombinant epimorphin and growth factors on branching morphogenesis of SCp2 cells. (A) Morphology of SCp2 cell clusters cultured for 8 d in collagen gels mixed with (a and b) or without (c and d) recombinant epimorphin H123. Presence of H123 in substrate and outside the clusters is indicated as H123EX. HGF (50 ng/ml) was added to the medium in b and d. b′ and d′ are sections of b and d stained with hematoxylin, respectively. (B) Quantification of the branching phenotype of SCp2 cells depicted in A. Cell clusters were surrounded by H123, H2, or H13 in collagen (indicated as H123EX, H2EX, or H13EX, respectively). Note that H123, but not H2 and H13 fragments induced branching morphogenesis in collagen gels. HGF dramatically enhanced the effect of H123 and functional blocking antibodies to HGF (100 μg/ml) completely neutralized HGF. (C) Effect of other growth factors on morphology of SCp2 cell clusters. EGF (a, d, and g), KGF (b and e), and FGF (c and f) can also induce branching morphogenesis in the presence (a–c and g) but not in the absence (a–c) of epimorphin H123 (H123EX). All of the factors were added to medium at 50 ng/ml. Note that EGF induced epimorphin-dependent branching morphogenesis even in the presence of function-blocking anti-HGF antibodies. (D) Summary of above data. For growth in collagen, when the average diameter of >50 clusters was 1–2× the control (size of cluster without the factor addition) and P value versus control was <0.1, it was denoted +; 2–3× the control is ++, and 3× or larger, +++. Branching was scored as described in Materials and Methods. Bars: (A and C) 200 μm.
To prove that epimorphin was indeed essential for branching morphogenesis of SCp2 cells, we isolated D6 and I6 cell lines, two clonal derivatives of the epimorphin-positive subpopulation of SCp2 cells. These cells expressed both epimorphin monomers comprised of 31 (isoform III) and 34 kD (isoform I and II; Hirai, 1993) and epimorphin dimers and tetramers comprised of ~70 and 150 kD (Hirai, 1994), respectively (Fig. (Fig.66 A). When preclustered, these cells expressed epimorphin surprisingly only at the periphery of cell aggregates (Fig. (Fig.66 B), reminiscent of the epimorphin staining pattern in the mammary gland around ducts and alveoli (Fig. (Fig.11 A). Consistent with what was observed in the mixed populations such as CID-9, this cell type displayed branching morphogenesis in the presence of growth factors without exogenously added epimorphin. However, the extent of the branching could be increased further by addition of epimorphin to the collagen gels (Fig. (Fig.66 C). The branching phenotype of D6 and I6 cells in the presence of HGF was blocked by anti-epimorphin antibodies (Fig. (Fig.6,6, C and D). On the other hand, cell clusters isolated from the epimorphin-negative subpopulations could not branch in the absence of exogenous epimorphin (Fig. (Fig.66 D). We therefore conclude that epimorphin is essential for branching morphogenesis of mammary epithelial cells in culture.
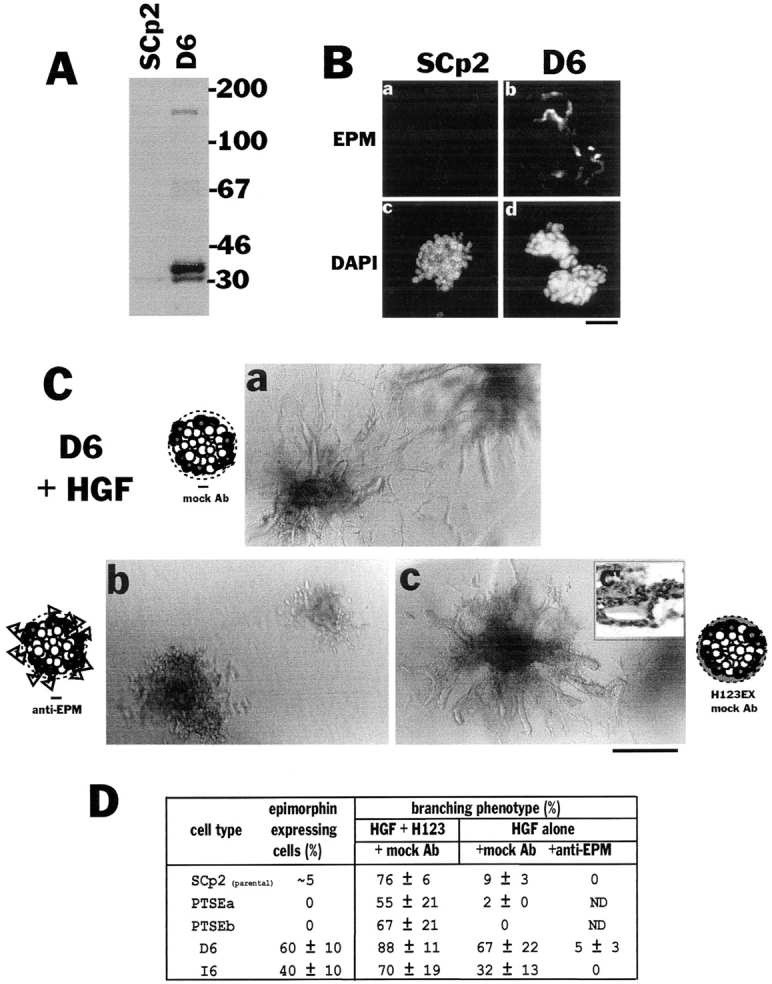
Branching morphogenesis of clonal derivatives of SCp2 cells. (A) The level of endogenous epimorphin in parental SCp2 and an epimorphin-positive clonal population (D6). Note that only very faint bands of endogenous epimorphin were detected in the SCp2 cells, as would be expected from the immunofluorescence studies (only ~5% express epimorphin). (B) Detection of endogenous epimorphin in the clusters of SCp2 and D6 cells. The sections of SCp2 (a and c) and D6 (b and d) cells were stained for epimorphin (a and b) and DAPI (c and d). The expression of epimorphin was still heterogenous in D6 cells and only the peripheral portion of the clusters were epimorphin-positive. (C) Appearance of cell clusters of D6 cultured for 8 d in collagen gels with (c) or without (a and b) additional recombinant epimorphin H123, in the presence of 50 ng/ml HGF. Mock antibodies (a and c) or anti-epimorphin antibodies (b) were added to medium at 100 μg/ ml. c′ is a section of c stained with hematoxilin. Note that D6 demonstrated branching morphogenesis in collagen, but exogenous epimorphin (H123EX) enhanced, and anti-epimorphin antibodies perturbed the phenotypic appearance. (D) Effect of H123 and anti-epimorphin antibodies on the appearance of branching phenotype of several subclones derived from SCp2. About 60% of D6 and 40% of I6 cells were epimorphin positive, whereas PTSEa and PTSEb (refer to Fig. Fig.8)8) were epimorphin negative. The values indicate mean ± SD of three separate experiments. Bars: (B) 120 μm; (C) 200 μm.
Pericellular Epimorphin Leads to “Lumen” Formation Instead of Branching Morphogenesis
In the experiments described above, cells were in contact with epimorphin only at the periphery of cell clusters even in the case of epimorphin-positive D6 and I6 cells, where the center of the cluster was negative. This suggests that polarized presentation of epimorphin at the basal cell surface, where epithelial cells interact with the ECM, might be important for establishment of ductal branching. To find out what will happen if epimorphin was presented to the cells in a nonpolar fashion, SCp2 cells were cultured on epimorphin-coated plates for 4 d, trypsinized briefly, and subsequently embedded into collagen gels after preclustering. This treatment allowed all cells to be coated with the otherwise insoluble epimorphin from the tissue culture dish, since trypsin treatment does not remove all the recombinant epimorphin from the cells (Fig. (Fig.77 A). After preclustering, recombinant epimorphin localized to both the periphery and around each cell of the clusters (Fig. (Fig.77 B). Once placed in collagen gels, ~20% of SCp2 cell clusters developed a “lumenal” phenotype characterized by large globular structures that were devoid of branching ducts (Fig. (Fig.77 C). As was the case with the branching phenotype, the lumenal phenotype was dramatically enhanced in the presence of HGF or EGF (Fig. (Fig.77 D; as well as KGF and FGF, and it was roughly proportional to the growth factor's ability to induce cell proliferation in collagen gels; not shown). Thus when epimorphin is presented in a nonpolar fashion around all the epithelial cell surface as a coating, it induces lumen formation in place of branching morphogenesis. Growth factors again simply enhance this phenotype.
To further confirm this finding, a cDNA coding for epimorphin connected to a signal peptide for secretion was transfected into SCp2 cells, under the control of a tetracyline-repressible promoter (Shockett et al., 1995). When tetracycline was removed from the cell culture medium, epimorphin, modified with N-linked glycosylation, was expressed uniformly on all the cell surfaces of the transfectants PTSEd and PTSEe (Fig. (Fig.8,8, A and B). Induction of epimorphin on a tissue culture substratum resulted in inhibition of cell growth (Fig. (Fig.88 C) as observed also with exogenous epimorphin (Fig. (Fig.44 C). When cell clusters were embedded into collagen gels and cultured in the presence of HGF, induction of the epimorphin transgene led to formation of large globular structures and little or no ductal branching (Fig. (Fig.99 A), similar to the results obtained in the preceding set of experiments (Fig. (Fig.7).7). Expression of epimorphin transgene in ETSEII cells, which were derived from an epimorphin-negative subpopulation of another mammary cell line EpH4, also led to lumen formation (Fig. (Fig.99 B). Again, HGF could be replaced by EGF (not shown), and anti-epimorphin antibodies could block this type of morphogenesis as well (Fig. (Fig.9,9, A and B). These data indicate that epimorphin, when expressed homogeneously on the entire cell surface, induces formation of large spherical structures, whereas polarized presentation of epimorphin results in formation of branching ducts. Thus epimorphin dictates the mode of morphogenesis of mammary epithelia depending on its localization, and growth factors such as HGF and EGF assist in this process by stimulation of cell proliferation.
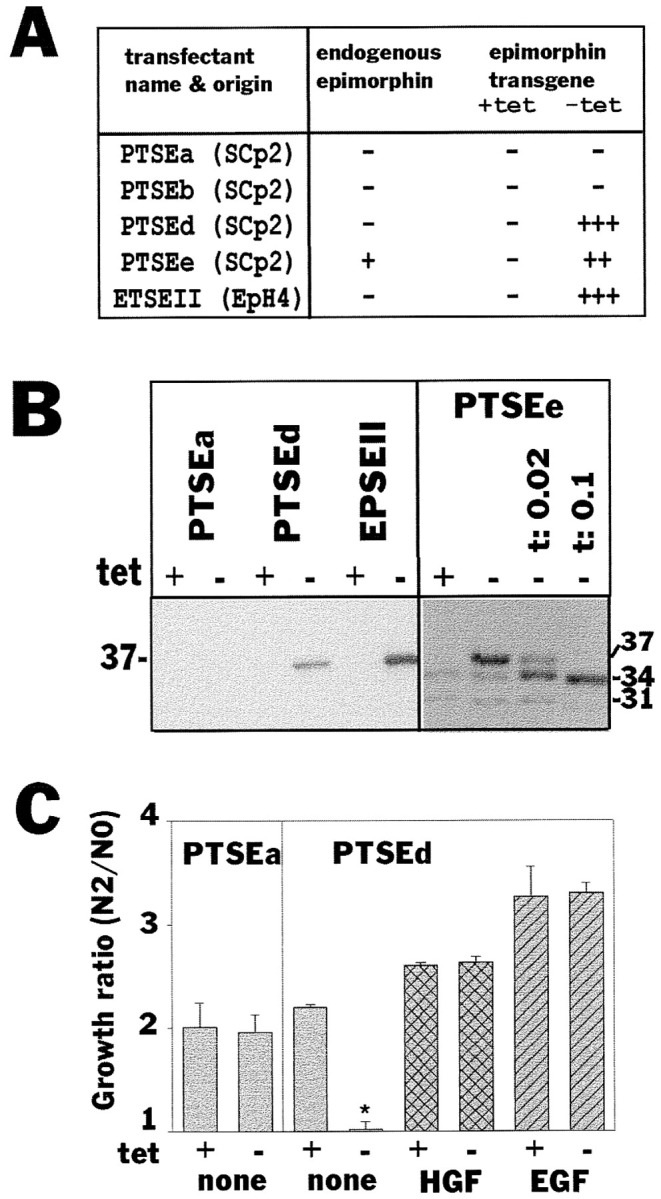
Epimorphin expression and cellular growth in the transfectants. (A) Characterization of transfectant clones isolated and used in this study: PTSEd, PTSEe (from SCp2), and ETSEII (from EpH4) expressed epimorphin transgene after removal of tetracycline. PTSEa and PTSEb cells were isolated from SCp2 as controls. Note that ETSEII was from an epimorphin-negative subpopulation of EpH4 cells. (B) Immunoblot analysis of epimorphin in the transfectants. The molecular mass of introduced extracellular epimorphin was 37 kD, which was reduced to that of endogenous epimorphin isoform I (34 kD) when medium contained 0.02 and 0.1 μg/ml tunicamycin. (C) The growth ratios of PTSEa and PTSEd cultured with or without tetracycline for 2 d. Media containing 50 ng/ml HGF and EGF were also tested. Note that the growth of transfected cells was severely suppressed when epimorphin transgene was induced (*).
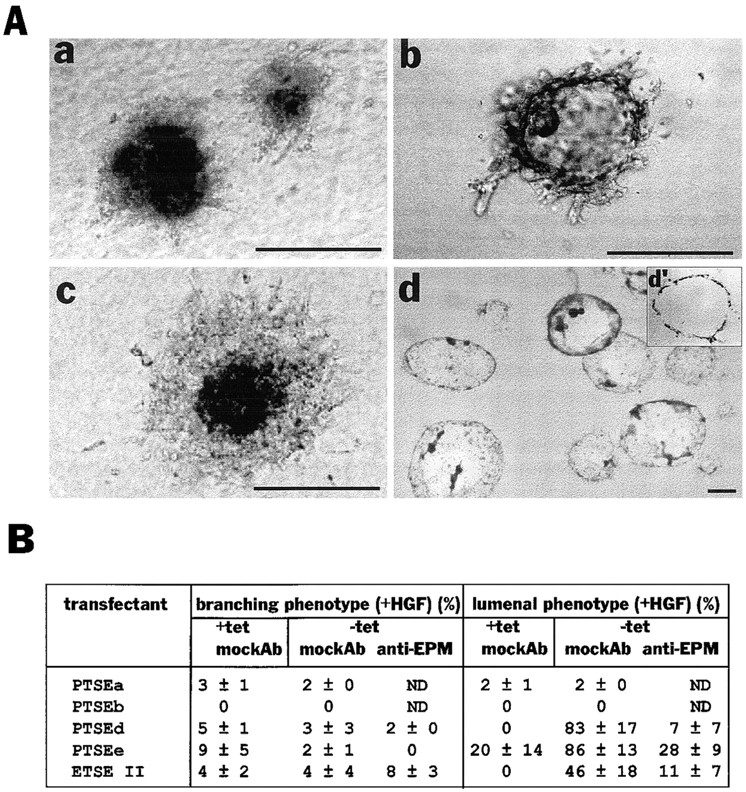
Epimorphin production by transfectants in clonal cultures leads to lumen formation. (A) The appearances of clusters of PTSEd cultured in the presence (a) or absence (b–d) of 5 μg/ml tetracycline, either for 8 d (a–c) or 14 d (d). Anti-epimorphin antibodies (100 μg/ml) were added to medium in c. d′ is a section of d stained with hematoxylin. Medium contained 50 ng/ml HGF in each case. The appearance in c was often slightly different from a, probably due to nonhomogeneous blocking of epimorphin transgene by the antibodies, leading to some focal, polar presentation of epimorphin outside the cluster. This is in turn would allow some fine branching from the cluster mass (which nevertheless did not resemble a branching phenotype). (B) Quantification of the appearance of branching and lumenal phenotype of the transfectants (The values indicate mean ± SD of three separate experiments). The concentration of the anti-epimorphin or mock antibodies added to medium was 100 μg/ml. Bars, 200 μm.
Discussion
The importance of epimorphin in control of morphogenesis of mammary epithelia is supported by the following observations: first, morphogenesis of epimorphin-negative epithelial cells was induced only by addition of epimorphin but not by growth factors alone. Second, epimorphin could induce different patterns of morphological differentiation, depending on the way it was presented to the cells. Third, morphogenesis of epimorphin-expressing epithelial cells was completely blocked by anti-epimorphin antibodies, even in the presence of growth factors. And fourth, as long as a growth factor could elicit growth from cells, it could augment the morphogenesis, but it did not matter which growth factor was used. Indeed, cells branched very well in the presence of function blocking antibodies to HGF, if epimorphin and another growth factor such as EGF were present.
Epimorphin was present in the mammary gland of virgin, pregnant, and lactating mice within the mesenchyme and around ducts and alveoli. Isolation of mammary cells revealed that epimorphin was produced not only by mesenchymal cells, but also by some myoepithelial cells, whereas lumenal epithelial cells were epimorphin negative. These data extend the previous observations made on mesenchymes prepared from skin, lung, and submandibular tissues, where epimorphin is also synthesized primarily by mesenchymal cells. The mouse mammary epithelial cell lines, SCp2 and EpH4, used in our studies are lumenal epithelial cells since they can produce milk proteins under appropriate culture conditions (Reichmann et al., 1989; Desprez et al., 1993); they also express cytokeratins and E-cadherin but not the myoepithelial marker α-smooth muscle actin or the mesenchymal marker vimentin (not shown). We found that EpH4 cells and a small subpopulation of SCp2 cells express epimorphin, suggesting that epimorphin expression in lumenal epithelial cells in vivo is suppressed by the cellular microenvironment in the mammary gland. In further support of our finding that epimorphin is required for morphogenesis, clonal subpopulations of SCp2 cells that expressed epimorphin branched upon exposure to growth factors, whereas epimorphin-negative cell clones did not. This finding also provides an explanation for the perplexing data where HGF induced branching morphogenesis in some, but not all, of the mammary cell models examined (Brinkmann et al., 1995; Soriano et al., 1995). Indeed, we found that EpH4 cells, which were shown previously to branch when HGF was added (Brinkmann et al., 1995), were heterogeneous and contained epimorphin-expressing cells that could signal to epimorphin-negative cells to undergo growth factor–induced morphogenesis in the absence of exogenously added epimorphin. Accordingly, when epimorphin-negative subclones were isolated they required exogenous epimorphin for branching morphogenesis. Interestingly, a study using primary lumenal epithelial and myoepithelial cells from mouse and human mammary gland concluded that HGF promoted branching morphogenesis only in myoepithelial cells (shown here to contain epimorphin-positive cells) but not lumenal epithelial cells which, as demonstrated here, are epimorphin negative in vivo (Niranjan et al., 1995). Likewise, branching and alveolar morphogenesis in primary organoid cultures that contain lumenal epithelial cells, myoepithelial cells and perhaps some fibroblasts, are efficiently induced by growth factors (Yang and Nandi, 1983; Darcy et al., 1995). Thus, all studies presented in the literature are compatible with our conclusion that epimorphin is indispensable for branching morphogenesis of mammary epithelia.
In previous studies that analyzed morphogenesis of mammary epithelia, proliferative influences were not distinguished from morphogenic effects. We found that HGF, EGF, KGF, and FGF promoted growth but not morphogenesis of mammary epithelia in the absence of epimorphin. Epimorphin, on the other hand, had some inhibitory influence on cell growth. Consequently, it was difficult to visualize stimulation of morphogenesis by epimorphin in the absence of growth factors. While epimorphin appears to be present throughout the mammary gland development, growth factor expression appears to be highest during intense morphogenetic phases in vivo (Yang et al., 1995). Thus, the coordinate expression of epimorphin and growth factors is likely to dictate morphogenesis in the mammary gland and possibly other organs, with one signaling component (growth factors) triggering growth and another (epimorphin) triggering morphogenesis. The nature of the latter is determined by the localization and distribution of epimorphin.
When epimorphin was presented at the periphery of epithelial clusters (at the border where epithelial cells contact collagen in culture, and stroma in vivo), it induced ductal branching; when epimorphin was accessible to the entire cell surface, a different type of morphogenesis was observed. Here, epimorphin induced formation of large globular structures, which were surrounded by a single layer of epithelial cells. Such “lumenal” structures, in conjugation with ducts, were reported previously for EpH4 cells upon cultivation in collagen in the presence of EGF (Oft et al., 1996), although no explanation for the diverse morphology was offered at that time. We propose that epimorphin is capable of giving directional information: when presented only to the periphery of cell clusters, it would lead to focal lumen formation and branching as a gradient is established, whereas exposure to the entire cell surface would induce nondirectional radial growth and development of spheres with a lumen.
The spheres that developed as a result of exposure to epimorphin initially resembled mammary alveoli. However, with prolonged time in culture, the volume of spheres dramatically increased, and structures began to resemble large fluid-filled cysts, as are seen during gross cystic disease and pathological conditions of the mammary gland. It is possible that during pathogenesis of the mammary gland, alterations in ECM and disturbance of epithelial polarity lead to accessibility of epimorphin to other than basal cell surfaces, or that epithelial cells begin to express epimorphin themselves (as do EpH4 and other mammary cells in culture). While these results do not prove whether epimorphin is involved in alveolar development during pregnancy and lactation or cyst formation during pathological conditions (or both), epimorphin appears to shape epithelial morphogenesis by providing directional information.
The molecular mechanism by which epimorphin promotes ductal branching and lumen formation remains to be established. For endothelial cells, which undergo changes in cellular architecture upon exposure to epimorphin in three-dimensional collagen culture, it was found that epimorphin augmented expression of angiogenic factors interleukin-8 and GM-CSF (Oka and Hirai, 1996). In the same study, the ECM-degrading proteinase urokinase-type plaminogen activator was upregulated by epimorphin. It is unlikely that in mammary cells epimorphin would increase expression of growth factors which in turn could stimulate branching in an autocrine or paracrine manner, since proliferation was reduced in the presence of epimorphin. However, we found upregulation of gelatinase A (not shown) when cells were maintained on an epimorphin substratum. Gelatinase A may focally proteolyze ECM molecules secreted by mammary epithelial cells and thereby alter cell–substratum interactions or might initiate proteolytic cascades that could result in degradation of type I collagen.
Epimorphin receptors have not yet been identified; however, it was discovered that a 19–amino acid motif (NL-peptide) within the H2 cell-binding domain of epimorphin mediates binding of cells to epimorphin (Koshida and Hirai, 1997). Furthermore, the NL-peptide perturbed morphogenesis of embryonic lung as efficiently as function-blocking antibodies to epimorphin. The identification of the NL-peptide that functions in cell recognition also lends further support to the notion that extracellular and not cytoplasmic epimorphin is the morphoregulatory form of epimorphin. It remains unclear whether epimorphin interacts with epithelial cells directly through binding to specific cell surface receptors or indirectly through binding of other cell-associated molecules. One possibility is that epimorphin binds to ECM molecules that are secreted by epithelial and mesenchymal cells such as laminins or type IV collagen. In this context it is noteworthy that epimorphin can be found in preparations of Engelbreth-Holm-Swarm tumor matrix (Matrigel; Collaborative Biomedical Products, Waldham, MA; Hirai et al., 1993), which consists mainly of basement membrane components laminin, entactin/nidogen, type IV collagen, and heparan sulfate proteoglycan (Kleinman et al., 1982). Interactions of epimorphin with ECM molecules could alter cellular function by altering signaling through ECM receptors. Alternatively, epimorphin itself may be involved in establishment of polarity, formation of an organized basement membrane, or cell–cell junctional complexes. These possibilities are under investigation.
Acknowledgments
The authors thank Drs. E.R. Reichmann and M. Takeichi for EpH4 cells and ECCD-2 antibody, respectively. We are also grateful to Dr. R. Schwarz for his computer analysis and B. Johansen for his administrative support.
This work was supported by the Office of Health and Environmental Research of the U.S. Department of Energy (contract DE-AC03-76SF00098) with additional support from the National Institutes of Health (contract CA57621) and the Science and Technology Agency of Japan.
Abbreviations used in this paper
ECM | extracellular matrix |
HGF and KGF | hepatocyte and keratinocyte growth factor |
Footnotes
Address all correspondence to Yohei Hirai, Lawrence Berkeley National Laboratory, Life Science Division, One Cyclotron Road, MS 83-101, Berkeley, CA 94720. Tel.: (510) 486-4368. Fax: (510) 486-5586. E-mail: vog.lbl@iarihy
A. Lochter's present address is Center for Clinical and Basic Research, 222 Ballerup Byvej, DK-2750, Ballerup, Denmark.
References
- Bennett MK, Garcia-Arraras JE, Elferink LA, Peterson K, Fleming AM, Hazuka CD, Sheller RH. The syntaxin family of vesicular transport receptors. Cell. 1993;74:863–873. [Abstract] [Google Scholar]
- Brinkmann V, Foroutan H, Sachs M, Weidner KM, Birchmeier W. Hepatocyte growth factor/scatter factor induces a variety of tissue-specific morphogenic programs in epithelial cells. J Cell Biol. 1995;131:1573–1586. [Europe PMC free article] [Abstract] [Google Scholar]
- Butt KI, Manabe M, Yaguchi H, Tsuboi R, Ogawa H. Immunolocalization of epimorphin in skin. J Dermatol Sci. 1996;13:193–201. [Abstract] [Google Scholar]
- Coleman S, Silberstein GB, Daniel CW. Ductal morphogenesis in the mouse mammary gland: evidence supporting a role for epidermal growth factor. Dev Biol. 1988;127:304–315. [Abstract] [Google Scholar]
- Daniel CW, Strickland P, Friedmann Y. Expression and functional role of E- and P-cadherins in mouse mammary ductal morphogenesis and growth. Dev Biol. 1995;169:511–519. [Abstract] [Google Scholar]
- Darcy KM, Shoemaker SF, Lee PH, Vaughan MM, Black JD, Ip MM. Prolactin and epidermal growth factor regulation of the proliferation, morphogenesis, and functional differentiation of normal rat mammary epithelial cells in three dimensional primary culture. J Cell Physiol. 1995;163:346–364. [Abstract] [Google Scholar]
- Deugnier M-A, Moiseyeva EP, Thiery JP, Glukhova M. Myoepithelial cell differentiation in the developing mammary gland: progressive acquisition of smooth muscle phenotype. Dev Dyn. 1995;204:107–117. [Abstract] [Google Scholar]
- Desprez P-Y, Roskelley C, Campisi J, Bissell MJ. Isolation of functional cell lines from a mouse mammary epithelial cell strain: the importance of basement membrane and cell-cell interaction. Mol Cell Differ. 1993;1:99–110. [Google Scholar]
- Dulbecco R, Unger M, Armstrong B, Bowman M, Syka P. Epithelial cell types and their evolution in rat mammary gland determined by immunological markers. Proc Natl Acad Sci USA. 1983;80:1033–1037. [Europe PMC free article] [Abstract] [Google Scholar]
- Ekblom P, Ekblom M, Fecker L, Klein G, Zhang HY, Kadoya Y, Chu ML, Mayer U, Timpl R. Role of mesenchymal nidogen for epithelial morphogenesis in vitro. Development. 1994;120:2003–2014. [Abstract] [Google Scholar]
- Grobstein C. Morphogenic interaction between embryonic mouse tissues separated by a membrane filter. Nature. 1953;172:869–871. [Abstract] [Google Scholar]
- Hirai Y. Molecular cloning of human epimorphin: identification of isoforms and their unique properties. Biochem Biophys Res Commun. 1993;191:1332–1337. [Abstract] [Google Scholar]
- Hirai Y. Sodium-dodecyl-sulfate-resistant complex formation of epimorphin monomers and interaction of the 150KDa complex with the cell surface. Eur J Biochem. 1994;225:1133–1139. [Abstract] [Google Scholar]
- Hirai Y, Nakagawa S, Takeichi M. Reexamination of the properties of epimorphin and its possible roles. Cell. 1993;73:426–427. [Abstract] [Google Scholar]
- Hirai Y, Takebe K, Takashina M, Kobayashi S, Takeichi M. Epimorphin: a mesenchymal protein essential for epithelial morphogenesis. Cell. 1992;69:471–481. [Abstract] [Google Scholar]
- Inoue A, Obata K, Akagawa K. Cloning and sequence analysis of cDNA for a neuronal cell membrane antigen, HPC-1. J Biol Chem. 1992;267:10613–10619. [Abstract] [Google Scholar]
- Kadoya Y, Kadoya K, Durbeej M, Holmvall K, Sorokin L, Ekblom P. Antibodies against E3 of laminin and integrin α6 subunit perturb branching epithelial morphogenesis of submandibular gland, but by different modes. J Cell Biol. 1995;129:521–534. [Europe PMC free article] [Abstract] [Google Scholar]
- Kleinman HK, McGarvey ML, Liotta LA, Robey PG, Tryggvason K, Martin GR. Isolation and characterization of type IV procollagen, laminin, and heparan sulfate proteoglycan from the EHS sarcoma. Biochemistry. 1982;21:6188–6193. [Abstract] [Google Scholar]
- Koshida S, Hirai Y. Identification of cellular recognition sequence of epimorphin and critical role of cell/epimorphin interaction in lung branching morphogenesis. Biochem Biophys Res Commun. 1997;234:522–525. [Abstract] [Google Scholar]
- Kratochwil, K. 1983. Embryonic induction. In Cell Interactions and Development. Molecular mechanisms. K.M. Yamada, editor. John Wiley and Sons, New York. 100–122.
- Laemmli UK. Cleavage of structural proteins during the assembly of the head of bacteriophage T4. Nature. 1970;227:680–685. [Abstract] [Google Scholar]
- Montesano R, Schaller G, Orci L. Induction of epithelial tubular morphogenesis in vitro by fibroblast-derived soluble factors. Cell. 1991a;66:697–711. [Abstract] [Google Scholar]
- Montesano R, Matsumoto K, Nakamura T, Orci L. Identification of a fibroblast-derived epithelial morphogen as hepatocyte growth factor. Cell. 1991b;67:901–908. [Abstract] [Google Scholar]
- Niranjan B, Buluwela L, Yant J, Perusinghe N, Atherton A, Phippard D, Dale T, Gusterson B, Kamalati T. HGF/SF: a potent cytokine for mammary growth, morphogenesis and development. Development. 1995;121:2897–2908. [Abstract] [Google Scholar]
- Oka Y, Hirai Y. Inductive influences of epimorphin on endothelial cells in vitro. Exp Cell Res. 1996;222:189–198. [Abstract] [Google Scholar]
- Oft M, Peli J, Rudaz C, Schwarz H, Beug H, Reichmann E. TGF-β1 and Ha-Ras collaborate in modulating the phenotypic plasticity and invasiveness of epithelial tumor cells. Genes Dev. 1996;10:2462–2477. [Abstract] [Google Scholar]
- Pelham HR. Is epimorphin involved in vesicular transport? . Cell. 1993;73:425–426. [Abstract] [Google Scholar]
- Reichmann E, Ball R, Groner B, Friis RR. New mammary epithelial and fibroblastic cell clones in coculture form structures competent to differentiate functionally. J Cell Biol. 1989;108:1127–1138. [Europe PMC free article] [Abstract] [Google Scholar]
- Sakakura T. New aspects of stroma-parenchyma relations in mammary gland differentiation. Int Rev Cytol. 1991;125:165–202. [Abstract] [Google Scholar]
- Sakakura T, Nishizuka Y, Dawe CJ. Mesenchymal-dependent morphogenesis and epithelium-specific cytodifferentiation in mouse mammary gland. Science. 1976;194:1439–1441. [Abstract] [Google Scholar]
- Sariola H, Aufderheide E, Bernhard H, Henke-Fahle S, Dippold W, Ekblom P. Antibodies to cell surface ganglioside GD3 perturb inductive epithelial-mesenchymal interactions. Cell. 1988;54:235–245. [Abstract] [Google Scholar]
- Sariola H, Saarma M, Sainio K, Arumae U, Palgi J, Uaahtokovia A, Thesleff I, Karavanov A. Dependence of kidney morphogenesis on the expression of nerve growth factor receptor. Science. 1991;254:571–573. [Abstract] [Google Scholar]
- Saxen L, Sariola H. Early organogenesis of the kidney. Pediatr Nephrol. 1987;1:385–392. [Abstract] [Google Scholar]
- Schmidhauser C, Bissell MJ, Myers CA, Casperson GF. Extracellular matrix and hormones transcriptionally regulate bovine β-casein 5′ sequences in stably transfected mouse mammary cells. Proc Natl Acad Sci USA. 1990;87:9118–9122. [Europe PMC free article] [Abstract] [Google Scholar]
- Schmidhauser C, Casperson GF, Myers CA, Sanzo KT, Bolten S, Bissell MJ. A novel transcriptional enhancer is involved in the prolactin- and extracellular matrix-dependent regulation of β-casein gene expression. Mol Biol Cell. 1992;3:699–709. [Europe PMC free article] [Abstract] [Google Scholar]
- Shirayoshi Y, Nose A, Iwasaki K, Takeichi M. N-linked oligosaccharides are not involved in the function of a cell-cell binding glycoprotein E-cadherin. Cell Struct Funct. 1986;11:245–252. [Abstract] [Google Scholar]
- Shockett P, Difilippantonio M, Hellman N, Schatz DG. A modified tetracycline-regulated system provides autoregulatory, inducible gene expression in cultured cells and transgenic mice. Proc Natl Acad Sci USA. 1995;92:6522–6526. [Europe PMC free article] [Abstract] [Google Scholar]
- Simonet WS, DeRose ML, Bucay N, Nguyan HQ, Wert SE, Zhou L, Ulich TR, Thomason A, Danilenko DM, Whitsett JA. Pulmonary malformation in transgenic mice expressing human keratinocyte growth factor in the lung. Proc Natl Acad Sci USA. 1995;92:12461–12465. [Europe PMC free article] [Abstract] [Google Scholar]
- Smirnova T, Stinnakre J, Mallet J. Characterization of a presynaptic glutamate receptor. Science. 1993a;262:430–433. [Abstract] [Google Scholar]
- Smirnova T, Laroche S, Errington ML, Hicks AA, Bliss TVP, Mallet J. Transsynaptic expression of presynaptic glutamate receptor during hippocampal long-term potentiation. Science. 1993b;262:433–435. [Abstract] [Google Scholar]
- Snedeker SM, Brown CF, DiAugustine RP. Expression and functional properties of transforming growth factor α and epidermal growth factor during mouse mammary gland ductal morphogenesis. Proc Natl Acad Sci USA. 1991;88:276–280. [Europe PMC free article] [Abstract] [Google Scholar]
- Soriano JV, Pepper MS, Nakamura T, Orci L, Montesano R. Hepatocyte growth factor stimulates extensive development of branching duct-like structures by cloned mammary gland epithelial cells. J Cell Sci. 1995;108:413–430. [Abstract] [Google Scholar]
- Spooner BS, Wessells NK. Mammalian lung development: interactions in primordium formation and bronchial morphogenesis. J Exp Zool. 1970;175:445–454. [Abstract] [Google Scholar]
- Tonelli QJ, Sorof S. Epidermal growth factor requirement for development of cultured mammary glands. Nature. 1980;285:250–252. [Abstract] [Google Scholar]
- Yang J, Nandi S. Growth of cultured cells using collagen as substrate. Int Rev Cytol. 1983;81:249–286. [Abstract] [Google Scholar]
- Yang Y, Spitzer E, Meyer D, Sachs M, Niemann C, Hartmann G, Weidner KM, Birchmeier C, Birchmeier W. Sequential requirement of hepatocyte growth factor and neuregulin in the morphogenesis and differentiation of the mammary gland. J Cell Biol. 1995;131:215–226. [Europe PMC free article] [Abstract] [Google Scholar]
- Yoshida A, Oho C, Omori A, Kuwahara R, Ito T, Takahashi M. HPC-1 is associated with synaptotagmin and ω-conotoxin receptor. J Biol Chem. 1992;267:24925–24928. [Abstract] [Google Scholar]
- Young SL, Chang LY, Erickson HP. Tenascin-C in rat lung: distribution ontogeny and role in branching morphogenesis. Dev Biol. 1994;161:615–625. [Abstract] [Google Scholar]
- Zarnegar R, Michalopoulos G. Purification and biological characterization of human hepatopoietin A, a polypeptide growth factor for hepatocytes. Cancer Res. 1989;49:3314–3320. [Abstract] [Google Scholar]
Articles from The Journal of Cell Biology are provided here courtesy of The Rockefeller University Press
Full text links
Read article at publisher's site: https://doi.org/10.1083/jcb.140.1.159
Read article for free, from open access legal sources, via Unpaywall:
https://rupress.org/jcb/article-pdf/140/1/159/1273409/16471.pdf
Citations & impact
Impact metrics
Citations of article over time
Alternative metrics
Smart citations by scite.ai
Explore citation contexts and check if this article has been
supported or disputed.
https://scite.ai/reports/10.1083/jcb.140.1.159
Article citations
Extracellular epimorphin impairs expression and processing of profilaggrin in HaCaT keratinocytes.
Cytotechnology, 75(2):123-133, 15 Dec 2022
Cited by: 1 article | PMID: 36969570 | PMCID: PMC10030722
STX2 Promotes Trophoblast Growth, Migration, and Invasion Through Activation of the PI3K-AKT Pathway in Preeclampsia.
Front Cell Dev Biol, 9:615973, 06 Jul 2021
Cited by: 11 articles | PMID: 34295885 | PMCID: PMC8292021
Local accumulation of extracellular matrix regulates global morphogenetic patterning in the developing mammary gland.
Curr Biol, 31(9):1903-1917.e6, 10 Mar 2021
Cited by: 20 articles | PMID: 33705716 | PMCID: PMC8119325
3D culture models for studying branching morphogenesis in the mammary gland and mammalian lung.
Biomaterials, 198:135-145, 23 Aug 2018
Cited by: 14 articles | PMID: 30174198 | PMCID: PMC6387650
Review Free full text in Europe PMC
Membrane-tethered syntaxin-4 locally abrogates E-cadherin function and activates Smad signals, contributing to asymmetric mammary epithelial morphogenesis.
J Cell Biochem, 119(9):7525-7539, 16 May 2018
Cited by: 0 articles | PMID: 29767852
Go to all (100) article citations
Other citations
Wikipedia
Similar Articles
To arrive at the top five similar articles we use a word-weighted algorithm to compare words from the Title and Abstract of each citation.
Hepatocyte growth factor stimulates extensive development of branching duct-like structures by cloned mammary gland epithelial cells.
J Cell Sci, 108 ( Pt 2):413-430, 01 Feb 1995
Cited by: 129 articles | PMID: 7768990
The interplay of matrix metalloproteinases, morphogens and growth factors is necessary for branching of mammary epithelial cells.
Development, 128(16):3117-3131, 01 Aug 2001
Cited by: 238 articles | PMID: 11688561 | PMCID: PMC2785713
Roles of hepatocyte growth factor/scatter factor and transforming growth factor-beta1 in mammary gland ductal morphogenesis.
J Mammary Gland Biol Neoplasia, 3(2):133-150, 01 Apr 1998
Cited by: 39 articles | PMID: 10819523
Review
Epimorphin mediates mammary luminal morphogenesis through control of C/EBPbeta.
J Cell Biol, 153(4):785-794, 01 May 2001
Cited by: 48 articles | PMID: 11352939 | PMCID: PMC2192384
Funding
Funders who supported this work.
NCI NIH HHS (2)
Grant ID: CA57621
Grant ID: R01 CA057621