Abstract
Free full text

Brain-derived neurotrophic factor rapidly enhances phosphorylation of the postsynaptic N-methyl-d-aspartate receptor subunit
1
Abstract
Although neurotrophins have traditionally been regarded as neuronal survival factors, recent work has suggested a role for these factors in synaptic plasticity. In particular, brain-derived neurotrophic factor (BDNF) rapidly enhances synaptic transmission in hippocampal neurons through trkB receptor stimulation and postsynaptic phosphorylation mechanisms. Activation of trkB also modulates hippocampal long-term potentiation, in which postsynaptic N-methyl-d-aspartate glutamate receptors play a key role. However, the final common pathway through which BDNF increases postsynaptic responsiveness is unknown. We now report that BDNF, within 5 min of exposure, elicits a dose-dependent increase in phosphorylation of the N-methyl-d-aspartate receptor subunit 1. This acute effect occurred in hippocampal synaptoneurosomes, which contain pre- and postsynaptic elements, and in isolated hippocampal postsynaptic densities. Nerve growth factor, in contrast, caused no enhancement of phosphorylation. These results suggest a potential mechanism for trophin-induced potentiation of synaptic transmission.
Neurotrophins play important roles in the survival and differentiation of specific neuronal populations during development and adulthood (1–3). Four members of the mammalian neurotrophin gene family have been identified: nerve growth factor (NGF), brain-derived neurotrophic factor (BDNF), neurotrophin-3 (NT-3), and NT-4/5 (4, 5). The neurotrophins bind to receptor tyrosine kinases of the trk protooncogene family with high affinity. trkA is a high-affinity receptor for NGF (6, 7), trkB for BDNF and NT-4/5 (8, 9) as well as NT-3 (10), and trkC for NT-3 (11).
In addition to classical trophic activities, we and others have shown that neurotrophins acutely modulate synaptic transmission (12–19) and long-term potentiation (20). Experiments with dissociated cultures of hippocampal neurons indicate that BDNF and NT-4/5 rapidly and selectively potentiate excitatory synaptic currents (17–19), and this trkB-mediated synaptic enhancement involves phosphorylation-dependent modulation of postsynaptic responsiveness (18). The exact mechanisms by which trkB activation modulates synaptic transmission remain to be established.
We recently found that trkB is a functionally active, intrinsic component of the adult rat postsynaptic density (PSD) isolated from cerebral cortex and hippocampus (21). The PSD is a proteinaceous disc-shaped subcellular organelle apposed to the inner surface of the postsynaptic membrane of chemical synapses (22). Neurotransmitter receptors, protein kinases, and ion channel proteins are anchored to the PSD (22), suggesting that the PSD participates in signal transduction and even receptor regulation. In fact, our recent studies revealed that N-methyl-d-aspartate (NMDA) receptors can be phosphorylated by intrinsic PSD kinases (23, 24). Phosphorylation of NMDA receptors is known to enhance receptor binding, channel conductance, and synaptic transmission (25–27). Because trkB activation enhances synaptic activity via postsynaptic phosphorylation mechanisms (18, 19), and trkB is found in the PSD (21), increased synaptic transmission may be due to neurotrophin-mediated phosphorylation of receptors in the PSD. In this study, we examined the effects of BDNF on phosphorylation of the NMDA receptor subunit 1 (NR1) in both adult hippocampal synaptoneurosomes and PSDs, to determine whether neurotrophins can elicit phosphorylation of synaptic NMDA receptors.
MATERIALS AND METHODS
Animals and Preparation of Synaptoneurosomal and PSD Fractions.
Adult Sprague–Dawley rat tissue was used. Animals were anesthetized with CO2 and decapitated. Tissues were then removed within 3 min and used immediately for synaptoneurosomal preparation or stored at −80°C until use for PSD preparation.
Procedures used to prepare synaptoneurosomal fractions were modified from those reported by Hollingsworth et al. (28). Briefly, fresh rat hippocampi were gently homogenized (five strokes at 100 rpm) with a Teflon/glass homogenizer in 7 vol (wt/vol) of a Hepes buffer [20 mM Hepes (pH 7.5) containing 110 mM NaCl/5.3 mM KCl/1.0 mM MgSO4/1.8 mM CaCl2/25 mM glucose/68.3 mM sucrose/10 μg/ml aprotinin/10 μg/ml leupeptin/20 μg/ml soybean trypsin inhibitor/1 mM phenylmethylsulfonyl fluoride (PMSF)]. The homogenate was filtered by gravity through a triple layer of 80-mm nylon netting, followed by pressure filtration through a Millipore 10-mm filter loaded in Swinnex holders (Millipore). The filtrate was centrifuged at 1,000 × g for 15 min. The pellets were resuspended in 30 vol of the Hepes buffer and recentrifuged. The synaptoneurosomes were resuspended in the Hepes buffer to yield a protein concentration of 3 mg/ml.
PSD fractions of adult rat hippocampus were prepared from frozen tissues as described (29).
BDNF-Mediated NR1 Phosphorylation Assays.
Synaptoneurosomal fractions. Samples (1 mg each in a final volume of 500 μl) were preincubated at 37°C for 1 hr with 0.5 mM [32P]orthophosphate (monosodium phosphate, 0.5 mCi/ml; 1 Ci = 37 GBq) in a shaking water bath. The mixtures were then incubated at 37°C for 5 min with vehicle (1 mM BSA in PBS) or BDNF (10, 50, 100, or 250 ng/ml). NGF (100 ng/ml) was used in parallel to determine specificity. PBS, vehicle, and 100 ng/ml BDNF were used to define the time course of BDNF effects (5, 10, or 15 min). The incubation was terminated by placing tubes on ice, followed by centrifugation in a microcentrifuge for 5 min. The pellets were boiled in 1% SDS (5 min), followed by the addition of 9 vol of solubilization buffer, and processed for immunoprecipitation as described below.
PSD fractions.
Samples (250 μg each in a final volume of 100 μl) were suspended in a buffer consisting of 50 mM Hepes (pH 7.5), 0.2 mM EGTA, 2 mM CaCl2, 10 μg/ml leupeptin, 10 μg/ml aprotinin, 20 μg/ml soybean trypsin inhibitor, and 1 mM PMSF. PBS, vehicle, or BDNF (50, 100, 250, 500, or 1250 ng/ml) was added to each experimental group. In parallel, NGF (500 ng/ml) was used to determine specificity of neurotrophin response. The reaction mixtures were incubated for 5 min with 20 μM [γ-32P]ATP (≈0.05 mCi/ml) at 37°C to define dose-response relationships. To examine the time course of BDNF effects, BDNF (500 ng/ml) was incubated for 5, 10, or 15 min. After centrifugation, the pellets were solubilized with ice-cold solubilization buffer (see below) for immunoprecipitation.
Immunoprecipitation.
After phosphorylation and centrifugation, pellets were boiled in 100 μl of 1% SDS for 5 min and the reaction mixtures were brought to 0.1% SDS with a solubilization buffer [50 mM TrisHCl (pH 7.5) containing 150 mM NaCl/10 mM EDTA/2 mM EGTA/1% Triton X-100/1% 3-[(3-cholamidopropyl)dimethylammonio]-1-propanesulfonate (CHAPS)/0.5% Nonidet P-40/10 μg/ml leupeptin/10 μg/ml aprotinin/20 μg/ml soybean trypsin inhibitor/0.1% BSA/1 mM PMSF/50 mM NaF/0.5 mM microcystin-LR]. The mixtures were incubated for 2 hr with shaking at 4°C and were spun at 10,000 × g for 5 min. The supernatant was then incubated with anti-NR1 (1 μg/ml) polyclonal antibodies (Chemicon) overnight at 4°C. Protein A-Sepharose CL-4B (Pharmacia LKB) (100 μl per tube) was added and mixed for another hour at 4°C. After centrifugation (10,000 × g for 5 min at 4°C), the Sepharose–antigen–antibody complexes were washed four times with a buffer [50 mM Tris
HCl (pH 8.1) containing 150 mM NaCl/1% Triton X-100/1% CHAPS/0.5% Nonidet P-40/0.1% SDS/1 mM PMSF). The immunoprecipitates were solubilized and boiled in a sample buffer (0.0625 M Tris
HCl, pH 6.8/2% SDS/5% 2-mercaptoethanol/10% glycerol/0.002% bromophenol blue) for 5 min. The samples were then subjected to SDS/PAGE for autoradiography and Western blot analysis.
SDS/PAGE, Autoradiography, and Western Blot Analysis.
All procedures were performed at room temperature. Vertical slab SDS/PAGE was performed using a continuous 3.5–15% acrylamide gradient. Electrophoresis was carried out at 125 V for 20 hr. Proteins were electrophoretically transferred to Immobilon-P membrane (Millipore) (30) at 25 V for 20 hr. The transfer buffer consisted of 25 mM TrisHCl and 200 mM glycine (pH 8.3–8.6). The resultant membrane was initially analyzed by autoradiography. Then, it was blocked with 5% fat-free milk in 0.1% TBS-T (20 mM Tris
HCl, pH 7.4/0.1% Tween 20) for 1 hr at room temperature, incubated with antibodies (0.5 μg/ml for anti-NR1) for another hour, followed by incubation with horseradish peroxidase-conjugated anti-rabbit IgG (Amersham) (1:5,000) for 45 min. Products were analyzed using the enhanced chemiluminescence Western blotting detection system supplied by Amersham.
Quantitation.
The intensity of bands on autoradiographs and immunoblots was quantitated with a scanning densitometer (CliniScan, Helena Laboratories). The NR1 phosphorylation levels per unit protein were obtained by normalizing arbitrary densitometric data of autoradiographs per unit immunoblot value. NR1 phosphorylation per unit protein in the vehicle control was assigned a value of 100%. All experiments were performed three times. Representative blots and autoradiographs are shown in the figures.
RESULTS
Effects of BDNF on Synaptoneurosomal NR1 Phosphorylation.
The isolated synaptoneurosome contained a sealed postsynaptic cytosolic compartment, based on electron microscopic examination of the preparations (not shown), reproducing the findings by the original authors (28). To examine whether BDNF is capable of enhancing hippocampal NR1 phosphorylation, we examined phosphorylation of synaptoneurosomal proteins in the presence of the trophin, followed by immunoprecipitation using anti-NR1 antibodies. The results of BDNF dose-dependent responses are presented in Fig. Fig.1.1. Western blot analyses (Fig. (Fig.11A) identified the bands in the autoradiographs (Fig. (Fig.11B) as NR1 (110 kDa). The densitometric scanning data are expressed as autoradiographic values per immunoblot protein, representing NR1 phosphorylation per unit protein (Fig. (Fig.11C). The effects of BDNF on NR1 phosphorylation were dose-dependent, with a significant 50% increase at 10 ng/ml and a maximal, 3-fold enhancement at 100 ng/ml (Fig. (Fig.11 B and C). Several proteins, including trkB, were coimmunoprecipitated with NR1 (Fig. (Fig.11B) and may be involved in NR1-related signaling cascades. The major coimmunoprecipitated phosphorylated protein, with a molecular weight of 51 kDa, similar to that of the Ig used for immunoprecipitation, may be the major PSD protein (mPSDp). Vehicle or NGF (100 ng/ml) caused no enhancement of NR1 phosphorylation, indicating specificity of the BDNF action.
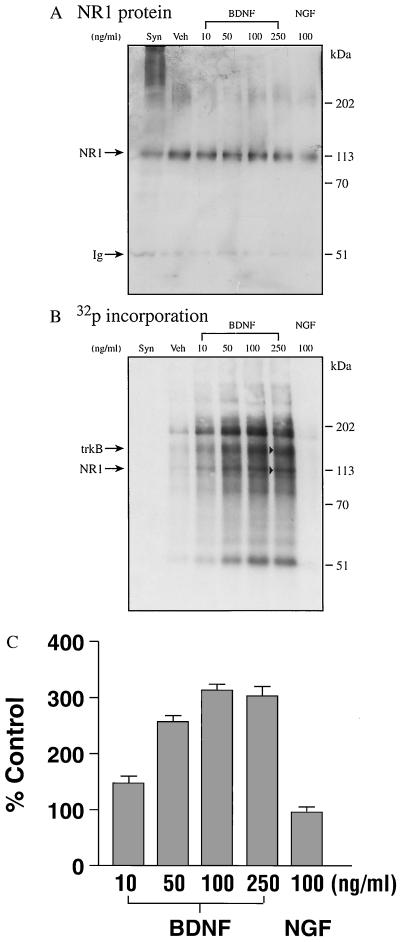
Enhancement of synaptoneurosomal NR1 phosphorylation by BDNF. Synaptoneurosomal proteins (1 mg each) were incubated in the presence of different concentrations of BDNF as described. NGF was used to define specificity of BDNF action. (A) Western blot. (B) Autoradiograph. (C) Normalized densitometric scanning values. The data shown in C are the mean ± SE of three separate determinations that varied less than 10% from each other. Immunoprecipitated NR1 (110 kDa) is indicated by arrows. Syn, synaptoneurosomal proteins that did not receive phosphorylation treatment; Veh, vehicle control consisting of 1 mM BSA in PBS; Ig, light or heavy chains of immunoglobin used in the immunoprecipitation.
To begin defining the acute effects of BDNF in mediating synaptic transmission, we studied the time course of BDNF effects on NR1 phosphorylation. Incubation with BDNF for 5 min elicited maximal NR1 phosphorylation (Fig. (Fig.22B). Incubation with BDNF for 15 min, however, had far less effect on phosphorylation, perhaps due to active dephosphorylation. The results obtained at 15 min were not attributable to proteolysis, since there were relatively much smaller changes in NR1 protein levels (Fig. (Fig.22A, lanes 3 and 11, from left) than in phosphorylation (Fig. (Fig.22B, lanes 3 and 11, from left). PBS, vehicle, or NGF alone did not enhance NR1 phosphorylation during the incubation periods examined.
Effects of BDNF on NR1 Phosphorylation in the PSD.
To determine whether BDNF potentiates NR1 phosphorylation through postsynaptic mechanisms, we examined phosphorylation of NR1 in the PSD. BDNF treatment (50–1,250 ng/ml) elicited a dose-dependent increase in NR1 phosphorylation (Fig. (Fig.33B), using equivalent concentrations of NR1 protein (Fig. (Fig.33A). BDNF treatment elicited a maximal, 7-fold increase in NR1 phosphorylation per unit of protein (Fig. (Fig.33C). The BDNF concentration (500 ng/ml) needed to achieve maximal effects in the PSD was 5-fold higher in the relatively inaccessible PSD preparation than in the synaptoneurosomal preparation. NR1 phosphorylation was not enhanced by vehicle or NGF (500 ng/ml), indicating the specificity of BDNF action (Fig. (Fig.3).3).
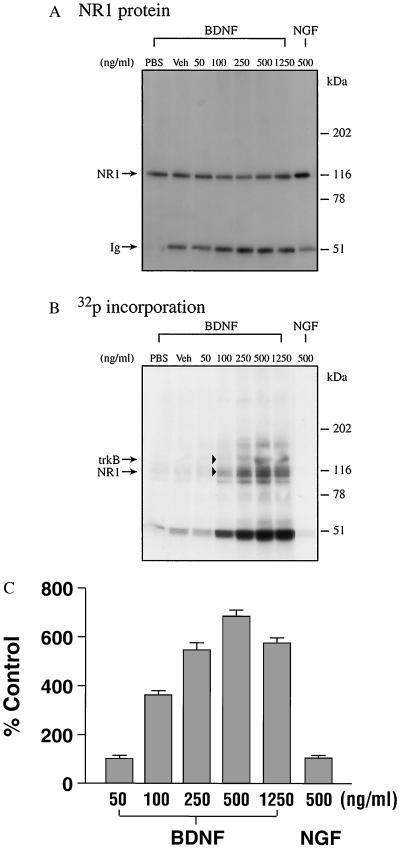
Potentiation of PSD NR1 phosphorylation by BDNF. PSD proteins (250 μg each) were incubated in the presence of various concentrations of BDNF. (A) Western blot. (B) Autoradiograph. (C) Normalized densitometric scanning values. The data shown in C are the mean ± SE of three separate determinations that varied less than 10% from each other.
To define acute effects of BDNF, we examined phosphorylation as a function of incubation time. The maximal effect on NR1 phosphorylation occurred at 5 min (Fig. (Fig.44B), paralleling that observed with synaptoneurosomes (Fig. (Fig.22B). Similarly, BDNF had far less effect on phosphorylation after 15 min of incubation. PBS, vehicle, or NGF caused no enhancement of NR1 phosphorylation regardless of incubation time (Fig. (Fig.44B).
DISCUSSION
Previous work from our laboratory and others has indicated that BDNF acutely enhances synaptic transmission in the hippocampus (14–19). These studies were designed to begin exploring underlying molecular mechanisms. Our results indicate that BDNF specifically enhances the phosphorylation of NR1 subunits of hippocampal NMDA receptors within 5 min of exposure. NR1 phosphorylation with enhanced conductance may thus constitute a critical mechanism mediating trophin stimulation of synaptic transmission.
We employed two different hippocampal preparations to investigate phosphorylation in vitro. We began with hippocampal synaptoneurosomes, which contain the entire pre- and postsynaptic apparatus (28), to most closely approximate physiological conditions, and to ensure that necessary synaptic components were present. BDNF specifically increased 32P incorporation into NR1 subunits 3-fold with a 5-min exposure. The rapidity of the phosphorylation response is entirely consistent with our previous observations that BDNF enhances synaptic transmission in hippocampal neurons within 2–3 min (18). Moreover, NGF had no detectable effect on synaptoneurosomal NR1 phosphorylation, paralleling its lack of effect on hippocampal synaptic transmission (16, 18, 19). Finally, we employed a range of trophin concentrations to determine whether phosphorylation required supranormal BDNF levels in this cell-free, in vitro model system. Significant enhancement of phosphorylation occurred at a concentration as low as 10 ng/ml, and a maximal, 3-fold increase was obtained at 100 ng/ml. However, any interpretation warrants caution, since the synaptoneurosomes undergo extensive preparative purification with potential compromise or loss of trkB receptors and signaling cascades. The relationship of present concentrations to those required in whole-cell culture remains open, necessitating further analysis. It should be noted that PSD preparations were prepared by detergent treatment of the synaptosomal fractions. It is apparent that some proteins may be modified in this purification procedure. Thus, the higher concentrations required to elicit a response with the isolated PSD fractions may be due to partial inactivation and/or loss of signaling element(s) involved in the trkB-mediated synaptic signaling cascade.
Our previous studies indicated that BDNF modulated synaptic transmission through postsynaptic actions involving trkB activation and phosphorylation mechanisms (18, 19). To determine whether BDNF can elicit NR1 phosphorylation specifically at the postsynaptic locus, we analyzed actions on the isolated PSD. The trophin evoked a maximal, 7-fold increase in NR1 phosphorylation in hippocampal PSDs. Maximal phosphorylation occurred within 5 min, and was actually decreased at 15 min of incubation, suggesting that the phosphorylation response is rapid and transient. The decrease after 15 min may reflect the participation of active, phosphatase-mediated, dephosphorylation mechanisms. In this connection, the coimmunoprecipitation of proteins with NR1 in both synaptoneurosomal and PSD preparations may represent molecules participating in the NR1 phosphorylation cascade.
We are presently investigating the possibility that these coimmunoprecipitated proteins include protein kinase C and CaM kinases. The latter two enzyme classes phosphorylate NR1 and associate noncovalently with NR1 in the PSD (23, 24), and may directly modulate NMDA receptor channel function (25). It is noteworthy that the coimmunoprecipitated proteins were qualitatively similar in the two preparations, suggesting that a common set of molecules regulate NR1 phosphorylation. This is consistent with the fact that there is at least a 200-fold purification of the PSD from synaptoneurosomes (K.W., P.-C.S., J.-L.X., unpublished results).
The precise sequence of events through which BDNF increases NR1 phosphorylation remains to be determined. BDNF is known to increase intracellular Ca2+, cAMP, and inositol triphosphate in the hippocampus (31, 32). We are presently investigating the potential role of these signals, as well as specific kinases and phosphatases, in BDNF-mediated NR1 phosphorylation.
Acknowledgments
We thank Ms. Betty Wheeler for excellent technical assistance and Dr. Geoff McAuliffe for performing electron microscopic examination of the synaptoneurosomal preparations. This research was supported by National Institutes of Health Grant HD 23315.
ABBREVIATIONS
PSD | postsynaptic density |
NMDA | N-methyl-d-aspartate |
NR1 | NMDA receptor subunit 1 |
BDNF | brain-derived neurotrophic factor |
NT-3 and -4/5 | neurotrophins-3 and -4/5 |
NGF | nerve growth factor |
References
Articles from Proceedings of the National Academy of Sciences of the United States of America are provided here courtesy of National Academy of Sciences
Full text links
Read article at publisher's site: https://doi.org/10.1073/pnas.94.15.8191
Read article for free, from open access legal sources, via Unpaywall:
https://europepmc.org/articles/pmc21579?pdf=render
Citations & impact
Impact metrics
Citations of article over time
Smart citations by scite.ai
Explore citation contexts and check if this article has been
supported or disputed.
https://scite.ai/reports/10.1073/pnas.94.15.8191
Article citations
Progressive reduction of nuclear receptor Nr4a1 mediates age-dependent cognitive decline.
Alzheimers Dement, 20(5):3504-3524, 11 Apr 2024
Cited by: 1 article | PMID: 38605605 | PMCID: PMC11095431
Brain-derived neuerotrophic factor and related mechanisms that mediate and influence progesterone-induced neuroprotection.
Front Endocrinol (Lausanne), 15:1286066, 26 Feb 2024
Cited by: 1 article | PMID: 38469139 | PMCID: PMC10925611
Review Free full text in Europe PMC
Investigating the Role of BDNF in Insomnia: Current Insights.
Nat Sci Sleep, 15:1045-1060, 07 Dec 2023
Cited by: 2 articles | PMID: 38090631 | PMCID: PMC10712264
Review Free full text in Europe PMC
Cellular mechanisms of contextual fear memory reconsolidation: Role of hippocampal SFKs, TrkB receptors and GluN2B-containing NMDA receptors.
Psychopharmacology (Berl), 241(1):61-73, 12 Sep 2023
Cited by: 0 articles | PMID: 37700085
Endocytosis is required for consolidation of pattern-separated memories in the perirhinal cortex.
Front Syst Neurosci, 17:1043664, 23 Feb 2023
Cited by: 1 article | PMID: 36911226 | PMCID: PMC9995888
Go to all (205) article citations
Similar Articles
To arrive at the top five similar articles we use a word-weighted algorithm to compare words from the Title and Abstract of each citation.
BDNF acutely increases tyrosine phosphorylation of the NMDA receptor subunit 2B in cortical and hippocampal postsynaptic densities.
Brain Res Mol Brain Res, 55(1):20-27, 01 Mar 1998
Cited by: 195 articles | PMID: 9645956
Brain-derived neurotrophic factor increases activity of NR2B-containing N-methyl-D-aspartate receptors in excised patches from hippocampal neurons.
J Neurosci Res, 62(3):357-362, 01 Nov 2000
Cited by: 80 articles | PMID: 11054804
Brain-derived neurotrophic factor acutely enhances tyrosine phosphorylation of the AMPA receptor subunit GluR1 via NMDA receptor-dependent mechanisms.
Brain Res Mol Brain Res, 130(1-2):178-186, 01 Nov 2004
Cited by: 43 articles | PMID: 15519688
Neurotoxicity of lead. Hypothetical molecular mechanisms of synaptic function disorders.
Neurol Neurochir Pol, 46(6):569-578, 01 Nov 2012
Cited by: 36 articles | PMID: 23319225
Review
Funding
Funders who supported this work.
NICHD NIH HHS (2)
Grant ID: P01 HD023315
Grant ID: HD 23315