Abstract
Free full text

Intracellular Ca2+ and Ca2+/Calmodulin-Dependent Kinase II Mediate Acute Potentiation of Neurotransmitter Release by Neurotrophin-3
Abstract
Neurotrophins have been shown to acutely modulate synaptic transmission in a variety of systems, but the underlying signaling mechanisms remain unclear. Here we provide evidence for an unusual mechanism that mediates synaptic potentiation at the neuromuscular junction (NMJ) induced by neurotrophin-3 (NT3), using Xenopus nerve–muscle co-culture. Unlike brain-derived neurotrophic factor (BDNF), which requires Ca2+ influx for its acute effect, NT3 rapidly enhances spontaneous transmitter release at the developing NMJ even when Ca2+ influx is completely blocked, suggesting that the NT3 effect is independent of extracellular Ca2+. Depletion of intracellular Ca2+ stores, or blockade of inositol 1, 4, 5-trisphosphate (IP3) or ryanodine receptors, prevents the NT3-induced synaptic potentiation. Blockade of IP3 receptors can not prevent BDNF-induced potentiation, suggesting that BDNF and NT3 use different mechanisms to potentiate transmitter release. Inhibition of Ca2+/calmodulin-dependent kinase II (CaMKII) completely blocks the acute effect of NT3. Furthermore, the NT3-induced potentiation requires a continuous activation of CaMKII, because application of the CaMKII inhibitor KN62 reverses the previously established NT3 effect. Thus, NT3 potentiates neurotransmitter secretion by stimulating Ca2+ release from intracellular stores through IP3 and/or ryanodine receptors, leading to an activation of CaMKII.
Introduction
Nerve growth factor, brain-derived neurotrophic factor (BDNF), neurotrophin-3 (NT3), and NT4 belong to the neurotrophin family of signaling proteins essential for the survival and differentiation of different populations of neurons. Recent studies have revealed an important but previously unrecognized role of neurotrophins in synapse transmission and plasticity (for reviews see Lu and Figurov 1997; McAllister et al. 1999). In the central nervous system (CNS), the synaptic actions of neurotrophins have been studied mainly in the visual cortex and hippocampus. For example, BDNF and NT4, which activate TrkB receptor, but not NT3, which primarily activates TrkC receptor, have long-term modulatory effects on the formation of ocular dominance columns in the visual cortex (Cabelli et al. 1995, Cabelli et al. 1997; Riddle et al. 1995). In layer 4 and 6 of the visual cortex, BDNF and NT3 oppose each other in regulating the dendritic growth of pyramidal neurons (McAllister et al. 1995, McAllister et al. 1997). In the hippocampus, BDNF has been shown to acutely regulate synaptic plasticity such as long-term potentiation (LTP) (Korte et al. 1995; Figurov et al. 1996; Patterson et al. 1996). In contrast, several recent reports have demonstrated that NT3 is not involved in the modulation of hippocampal LTP (Figurov et al. 1996; Kokaia et al. 1998; Ma et al. 1999). These studies suggest that the signaling mechanisms for BDNF and NT3 may be quite different.
Due to the complexity of CNS synapses, the mechanisms underlying the synaptic actions of neurotrophins are difficult to study. The neuromuscular junction (NMJ) offers a simple and easily accessible model to study the role and the mechanisms of neurotrophins in synaptic development and function in great detail. Two modes of neurotrophic regulation have been identified using the Xenopus nerve–muscle co-cultures: acute potentiation of neurotransmitter release and long-term regulation of synapse maturation. In the long-term mode, the spontaneous synaptic currents (SSCs) and impulse–evoked synaptic currents exhibit more mature properties after a prolonged treatment with NT3, and to a lesser extent, with BDNF (Wang et al. 1995; Liou and Fu 1997; Liou et al. 1997). The neurotrophins induce an increase in the expression of synaptic vesicle proteins, and in the number of synaptic varicosities in the presynaptic site (Wang et al. 1995), as well as changes in the acetylcholine (ACh) receptors in the postsynaptic site (Wang and Poo 1997; Gonzalez et al. 1999). In the acute mode, application of BDNF or NT3 rapidly enhances synaptic transmission at the NMJ (Lohof et al. 1993). The acute effect of neurotrophins is due strictly to an enhancement of transmitter release probability in the presynaptic site (Lohof et al. 1993; Stoop and Poo 1995). The SSC frequency is markedly increased, whereas the quantal sizes are not affected. The expression of NT3, but not BDNF or NT4, in the postsynaptic muscle cells is activity-dependent (Xie et al. 1997). Further, the secretion of NT4 in muscle cells seems to be induced by repetitive stimulation of presynaptic neurons (Wang and Poo 1997). These results suggest that neurotrophins may serve as target-derived, retrograde messengers that acutely modulate transmitter release at the developing neuromuscular synapses (Xie et al. 1997).
A critical and yet unresolved question is: what are the intracellular signaling mechanisms that mediate such rapid synaptic effects of neurotrophins? In the hippocampus, BDNF-induced enhancement of high frequency transmission at CA1 synapses appears to be mediated through the activation of mitogen-associated protein kinase and phosphatidylinositol-3 kinase pathways, but not phospholipase C-γ pathway (Gottschalk et al. 1999). The acute modulation of synaptic transmission by BDNF at NMJ appears to require Ca2+ influx into the presynaptic terminals, but signaling events downstream of Ca2+ influx are not known (Stoop and Poo 1996). Do neurotrophins share similar mechanisms in modulating synapses in the CNS and at the NMJ? Do BDNF and NT3 use the same signaling pathway to potentiate the neuromuscular synapses? In this report, we address the role of the nerve terminal Ca2+ in the acute regulation of neurotransmitter release at the NMJ by NT3. Specifically, we focus on the intracellular Ca2+ stores and the presynaptic Ca2+/calmodulin-dependent kinase II (CaMKII). A number of recent studies have suggested the involvement of intracellular Ca2+ stores in synaptic transmission (for review see Berridge 1998). Although extensive studies have revealed diverse effects of CaMKII in postsynaptic functions (Chapman et al. 1995), the only clearly defined presynaptic effects of CaMKII is to regulate the availability of readily releasable synaptic vesicles at the nerve terminals (Llinas et al. 1985; Greengard et al. 1993). We have now provided evidence that the acute potentiation of transmitter release by NT3 depends on a rise of Ca2+ concentrations ([Ca2+]i) in the presynaptic terminals. Surprisingly, the increase in [Ca2+]i was due to Ca2+ released from intracellular stores, but not to Ca2+ influx from extracellular sources. Furthermore, the continuous activation of CaMKII, which is triggered by the increase in [Ca2+]i, appears to be required for the effect of NT3. These results may help understand how neurotrophins acutely modulate neurotransmitter release.
Materials and Methods
Embryo Injection
Specific peptide inhibitor for CaMKII (Ishida et al. 1995) (500 μM; Calbiochem) was mixed with rhodamine-dextran (10 μg/μl, mol wt 10,000) at 1:1 ratio. Approximately 6–12 nl of the solution was injected into one blastomere of embryos at the 2- to 4-cell stage by a Picospritzer. The final concentration of the peptide within an injected blastomere was ~12.5 μM. 1 d after injection, the neural tube and the associated myotomal tissues were dissected and used to prepare nerve–muscle cultures. Cells containing CaMKII-pep were identified by rhodamine fluorescence.
Culture Preparation
Xenopus nerve–muscle cultures were prepared according to the procedure described previously (Lu et al. 1992). In brief, the neural tube and the associated myotomal tissue of Xenopus embryos at stage 20 to 22 were dissociated in Ca2+-Mg2+–free saline supplemented with EDTA (58.2 mM NaCl, 0.7 mM KCl, 0.3 mM EDTA, pH 7.4) for 15–20 min. The cells were grown on glass coverslips for 24 h at room temperature (20–22°C). The culture medium consisted (vol/vol) of 50% Leibovitz L-15 medium (Sigma), 1% FCS (Life Technologies), and 49% Ringer's solution (115 mM NaCl, 2 mM CaCl2, 2.5 mM KCl, 10 mM Hepes, pH 7.6). NT3 (2–5 × 10−9 M; kindly provided by Regeneron Pharmaceuticals, Inc.) and various inhibitors were applied directly to the culture media at the time of recording.
Electrophysiology
Synaptic currents were recorded at room temperature in culture medium from myocytes innervated by spinal motoneurons using whole cell, voltage-clamp recording techniques (Lu et al. 1992). The solution inside the recording pipette contained: 150 mM KCl, 1 mM NaCl, 1 mM MgCl2, and 10 mM Hepes buffer, pH 7.2. Membrane currents in all recordings were monitored by a patch clamp amplifier (EPC-7), with a current signal filter at 3 kHz. The membrane potentials of the muscle cells were generally in the range of −55–−75 mV and were voltage clamped at −70 mV after measuring the membrane potentials. For experiments performed in the absence of external Ca2+, the culture medium was replaced with a Ca2+-free extracellular solution containing 115 mM NaCl, 2 mM MgCl2, 10 mM Hepes, 3 mM EGTA, and 0.1% BSA. All data were stored on a videotape recorder for later playback on a storage oscilloscope (Tektronix TDS 420) and a chart recorder (Gould EasyGraf 240), or analysis using the SCAN program. To quantitatively measure the changes in neurotransmitter release, a time course of SSC frequency was first constructed on a minute-to-minute basis. The SSC frequencies in a 10-min period right before drug application were averaged as control. The changes in SSC frequency were measured by averaging a 10-min period recording starting from the highest number after drug application.
Results
Ca2+ Influx Is Not Required for NT3-induced Synaptic Potentiation
We recorded synaptic activities at the neuromuscular synapses in 1-d-old Xenopus nerve–muscle cultures using whole cell, voltage-clamp recording techniques. The SSCs are induced by spontaneous secretion of individual ACh-containing synaptic vesicles from motor nerve terminals independent of action potentials, since they are not affected in the presence of tetrodotoxin (Song et al. 1997). As shown before (Lohof et al. 1993; Stoop and Poo 1995, Stoop and Poo 1996), acute application of NT3 (50 ng/ml) to the synapses in the presence of extracellular Ca2+ dramatically enhanced spontaneous transmitter release, as reflected by a rapid increase in the frequency of SSCs (Fig. 1 A). A previous report showed that in the same type of cultures, BDNF enhances synaptic transmission by facilitating Ca2+ influx into the presynaptic terminals (Stoop and Poo 1996). We thus tested whether the acute NT3 effect uses a similar mechanism. The culture medium was substituted with Ca2+-free extracellular solution after several washes with the same solution. Surprisingly, application of NT3 still elicited an increase in the frequency of SSCs under the zero external Ca2+ condition (Fig. 1 B and Fig. 2). The time courses of the NT3-induced increase in SSC frequency in both Ca2+-free and Ca2+-containing media were very similar, although the basal level of SSC frequency in Ca2+-free media before NT3 application was slightly lower (Fig. 2A and Fig. B). Quantitative analysis indicated that NT3 only increased the frequency, without affecting the amplitude or decay time of SSCs (data not shown), suggesting that NT3 facilitates presynaptic transmitter release in the absence of extracellular Ca2+. To further examine the role of membrane Ca2+ channels, we blocked Ca2+ influx by the general Ca2+ channel blocker Cd2+ (0.4 mM). NT3 was still capable of elevating SSC frequency in the presence of Cd2+ (Fig. 2 B). Thus, NT3-induced potentiation of transmitter release does not depend on Ca2+ influx from extracellular sources. Our further analyses were thus performed mostly in Ca2+-free medium.
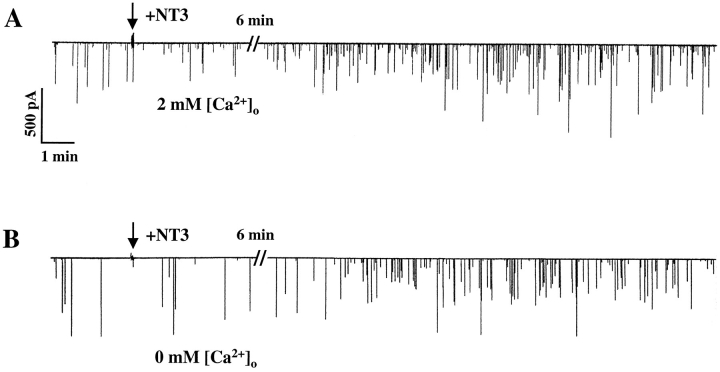
Acute potentiation of transmitter release at NMJ by NT3 is independent of extracellular Ca2+. (A) A sample recording showing that application of NT3 (50 ng/ml) rapidly increases the frequency of SSCs in the normal culture medium. (B) Application of NT3 also rapidly increases the frequency of SSCs in the Ca2+-free medium.
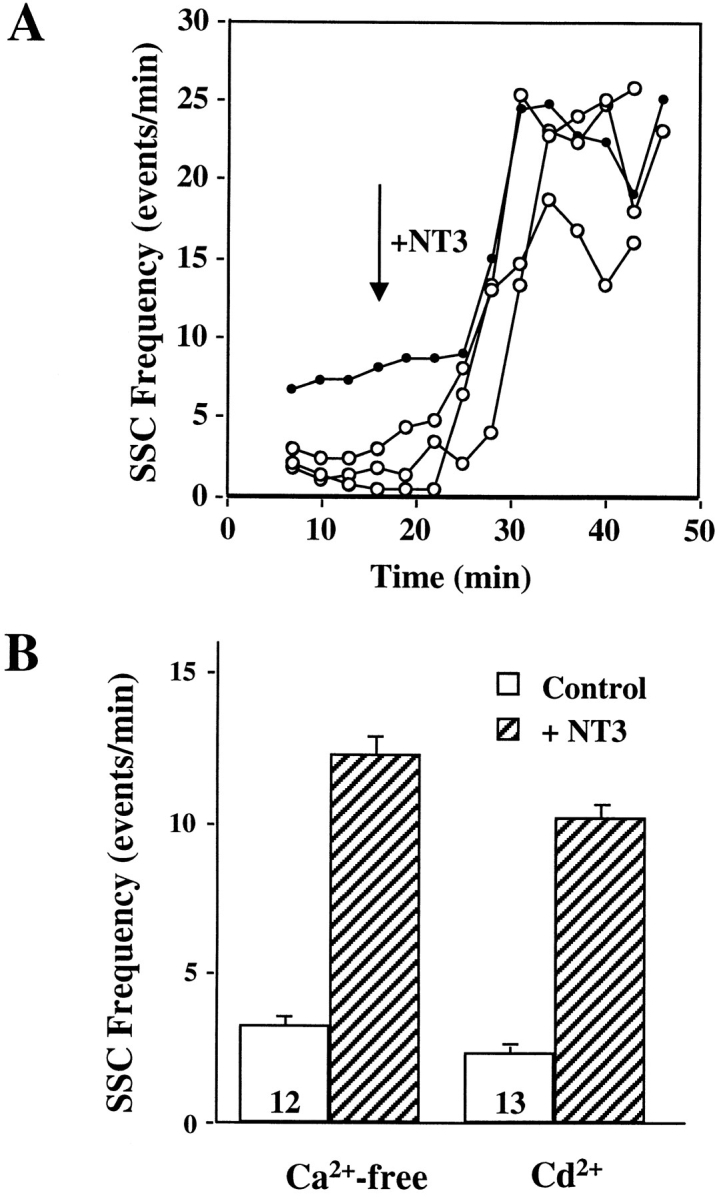
NT3-induced synaptic potentiation does not require Ca2+ influx. (A) Time courses of NT3 effect on spontaneous synaptic activity in zero external Ca2+ (open circles). The culture medium was replaced by Ca2+-free solution. The SSC frequency was monitored before and after NT3 application. Each point represents averaged SSC frequency in 3 min of recording. An example of recordings in normal medium (filled circles) is included for comparison. (B) The effect of NT3 on SSC frequency in Ca2+-free medium (n = 12) or in medium containing the Ca2+ channel blocker, Cd2+ (CdCl2, 0.4 mM, n = 13). For each synapse, a time course of SSC frequency was first constructed on a minute-to-minute basis. SSC frequencies are averaged from a 10-min recording right before NT3 application for controls, and a 10-min period starting from the highest number after NT3 application for NT3-treated groups. Error bars in this and all other figures are SEM. NT3 induced significant increases in SSC frequency in both conditions (P < 0.005, t test). Similar methods were used to calculate averaged SSC frequencies in all other figures, unless indicated otherwise.
Role of Intracellular Ca2+ Stores
We next determined whether the NT3 effect is mediated by an increase in Ca2+ release from intracellular Ca2+ stores. Thapsigargin inhibits Ca2+-ATPase activity and therefore has frequently been used to deplete all intracellular Ca2+ stores (Thastrup et al. 1990). Application of thapsigargin (2 μM) in both normal (n = 5, data not shown) and Ca2+-free media (Fig. 3 A) elicited a transient increase in SSC frequency, which returned to control levels within 20–60 min. NT3 no longer elicited any changes in SSC frequency in thapsigargin-treated synapses (Fig. 3A and Fig. B). When intracellular Ca2+ stores were depleted by thapsigargin, application of hypertonic solution (sucrose, 500 mM) to the synapses in Ca2+-free medium still elicited a transient but marked increase in transmitter release, suggesting that there are still synaptic vesicles in the nerve terminals (data not shown). Thus, the lack of NT3 effect in thapsigargin-treated synapses was not due to vesicle depletion. These results suggest that an increase in [Ca2+]i due to Ca2+ release from intracellular stores may contribute to the facilitation of transmitter release by induced NT3.
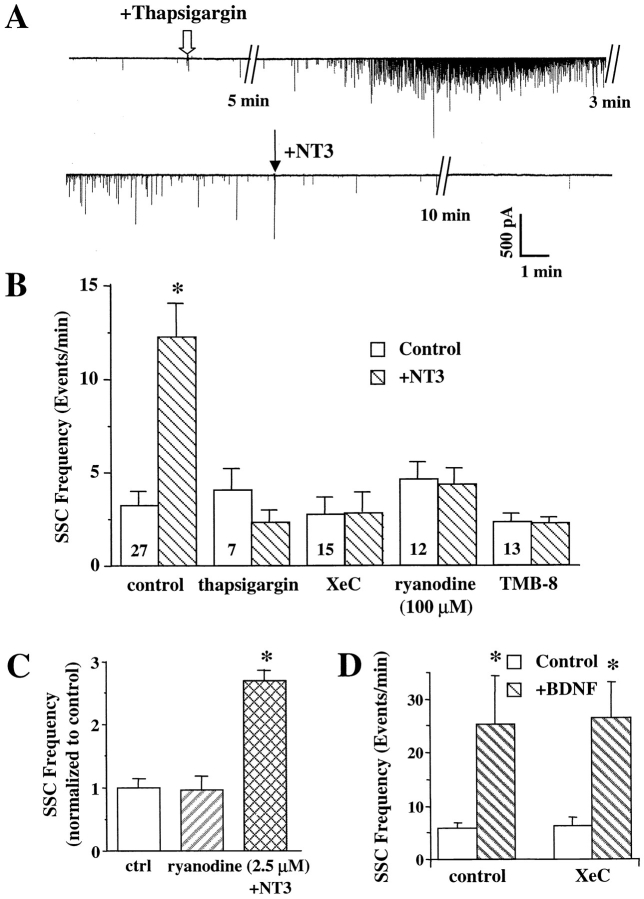
Enhancement of transmitter release by NT3, but not BDNF, requires Ca2+ release from intracellular Ca2+ stores. All experiments were carried out in Ca2+-free conditions, except that shown in D. The cultures were incubated with various drugs for 15–60 min, and SSCs were recorded continuously to monitor the effects of the drug and NT3 application. Thapsigargin was used to deplete Ca2+ from intracellular stores. Asterisk indicates data that are significantly different from the rest (P < 0.01, ANOVA test followed by post hoc comparison). (A) An example showing that thapsigargin (2 μM) prevents the NT3 effect. Application of thapsigargin (open arrow) elicited a transient but marked increase in SSC frequency due to the Ca2+ release from intracellular stores. Application of NT3 after SSC frequency returned to normal could no longer increase the SSC frequency. (B) Summary of the drug effects. Final concentration of the drugs in the culture media: thapsigargin, 2 μM; XeC, 1 μM; TMB-8, 30 μM; and ryanodine as an antagonist, 100 μM. The number of synapses recorded are indicated in the control bar of each pair. Asterisk indicates P < 0.005, t test. SSC frequencies are calculated in the same way as Fig. 2. (C) Summary of the effects of ryanodine as an agonist (2.5–5 μM). The SSC frequencies from a single synapse are counted for a 10-min period in control and a 10-min period after ryanodine application, and then a 10-min period after NT3 application. The data are then averaged and normalized to controls (n = 9). Note that ryanodine at the low concentration could not block the NT3 effect. (D) BDNF-induced synaptic potentiation is independent of Ca2+ release from intracellular stores through IP3 receptors. BDNF was applied to culture dishes in the presence (right, n = 5) or absence (left, n = 5) of the IP3 receptor inhibitor XeC (1 μM).
There are two major pathways for the release of Ca2+ from intracellular stores: the inositol 1, 4, 5-trisphosphate (IP3) receptor and the ryanodine receptor (Berridge 1998). Application of the IP3 receptor inhibitor xestospongin C (XeC; 1 μM) (Gafni et al. 1997) prevented the increase of SSC frequency elicited by NT3 (Fig. 3 B). The release of Ca2+ from IP3 receptors could further trigger Ca2+-induced Ca2+ release from ryanodine receptors (Berridge 1998). Low concentration of ryanodine (2.5–5 μM) may be used as a ryanodine receptor agonist, whereas high concentration (100 μM) may serve as an antagonist. In Ca2+-free medium, application of ryanodine at high concentration (100 μM) had no effect on basal SSCs, but prevented the increase in SSC frequency induced by NT3 (Fig. 3 B) (P > 0.5, ANOVA). Pretreatment of the cultures with another ryanodine receptor antagonist 8-(dethylamino)octyl 3, 4, 5-trimethoxybenzoate (TMB-8) (30 μM) (Hunt et al. 1990) also blocked the NT3 effects (Fig. 3B). Unlike thapsigargin, however, ryanodine at lower concentration (2.5–5 μM) was not sufficient to elicit a consistent increase in SSC frequency (Fig. 3 C). Furthermore, application of NT3 in the presence of low concentration of ryanodine still elicited an increase in SSC frequency (Fig. 3 C). Thus, the synaptic action of NT3 is primarily mediated by the Ca2+ release from IP3 receptors, which further triggers Ca2+ release from the ryanodine receptors.
We then tested whether BDNF, which requires Ca2+ influx to enhance transmitter release (Stoop and Poo 1996), also depends on Ca2+ release from intracellular stores. Application of BDNF (50 ng/ml) elicited a fivefold increase in SSC frequency in normal Ca2+-containing medium (Fig. 3 D). In cultures pretreated with XeC (1 μM) to block IP3 receptors, BDNF elicited the same magnitude of synaptic potentiation (Fig. 3 D), suggesting that the Ca2+ release from intracellular stores is not required for BDNF-induced synaptic potentiation. Thus, BDNF and NT3, two proteins from the same neurotrophin family, can both potentiate neurotransmitter release, but they use totally different intracellular mechanisms.
The Effect of NT3 Requires Continuous Activation of CaMKII
The release of Ca2+ from intracellular Ca2+ stores induced by NT3 may trigger the activation of the presynaptic CaMKII. CaMKII has been shown to enhance transmitter release in adult squid giant synapses and mammalian brain synaptosomes, presumably due to an increase in the availability of readily releasable synaptic vesicles at the nerve terminals (Llinas et al. 1985; Nichols et al. 1990). We first tested whether CaMKII is involved in modulating transmitter release at the developing NMJ in the Xenopus culture system using KN62, a frequently used inhibitor for CaMKII (Tokumitsu et al. 1990). We found that bath application of KN62 (3 μM) rapidly and reversibly reduced the amplitude of evoked synaptic currents. The average evoked synaptic current amplitudes before and 10 min after KN62 application were 1.77 + 0.36 nA and 1.01 + 0.24 nA, respectively (n = 8, P < 0.01, t test). In contrast, application of KN62 had little effect on the spontaneous release of neurotransmitters (Fig. 4A and Fig. B). These results are consistent with the idea that CaMKII may regulate transmitter release when the terminal [Ca2+]i is elevated, but may not be very effective at the quiescent level of [Ca2+]i. Since NT3 acts presynaptically at the NMJ and CaMKII is capable of regulating transmitter release, we determined whether CaMKII is involved in the synaptic action of NT3 at the developing NMJ. Pretreatment of the nerve–muscle cultures with KN62 completely prevented the increase of SSC frequency elicited by NT3 (Fig. 4). The average SSC frequencies before and 10 min after KN62 remained unchanged (before KN62; 5.9 ± 0.6 events/min; after KN62, 5.6 ± 0.6 events/min; n = 8, P > 0.1). These results suggest that CaMKII is necessary for the NT3 regulation of neurotransmitter release.
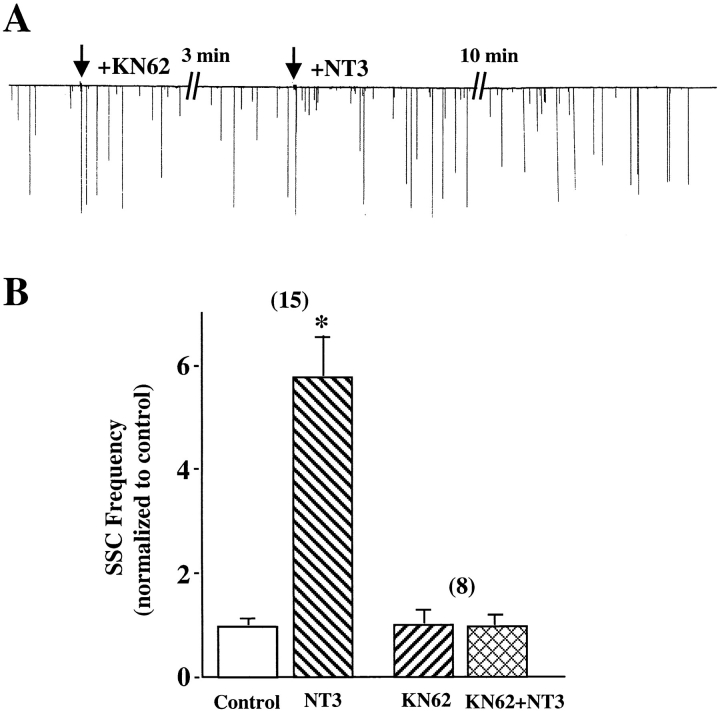
Effect of KN62 on NT3-induced synaptic potentiation. (A) A sample recording showing that pretreatment of the neuromuscular synapse with KN62 prevents the NT3-induced facilitation of transmitter release. (B) Blockade of NT3-induced synaptic potentiation of SSC frequency by KN62. In KN62 groups, the nerve–muscle cultures were pretreated with KN62 for 10–20 min before application of NT3. The number of synapses recorded are indicated above each pair of columns. In each experiment, SSC frequency was calculated from a 10-min recording period, and then the number after NT3 treatment was normalized to that before NT3 application. Asterisk indicates P < 0.01, t test.
KN62 may also inhibit other Ca2+/calmodulin-dependent protein kinases (Enslen et al. 1994). To ensure that the NT3 effect is indeed mediated by CaMKII, we loaded a specific peptide inhibitor (CaMKII-pep) (Ishida et al. 1995) into the presynaptic neurons using embryo injection techniques (Alder et al. 1992; Lu et al. 1992). CaMKII-pep was injected together with rhodamine-dextran into one of the blastomeres of Xenopus embryos at the two-cell stage. The embryos were allowed to develop until stage 22 before being used to prepare the nerve–muscle co-cultures. Introduction of the CaMKII-pep did not appear to affect the development and morphology of the Xenopus embryos at the time of cell culture (not shown). The spinal neurons and the myocytes 1 d after cultures also exhibited normal morphology (Fig. 5 A). It has been shown that the rhodamine fluorescence faithfully reflects the cells containing the coinjected exogenous proteins (Alder et al. 1992; Lu et al. 1992). In cultures derived from injected embryos, substantial numbers of neurons and myocytes were CaMKII-pep–positive, as indicated by the rhodamine fluorescence (Fig. 5 A, N+ and M+).
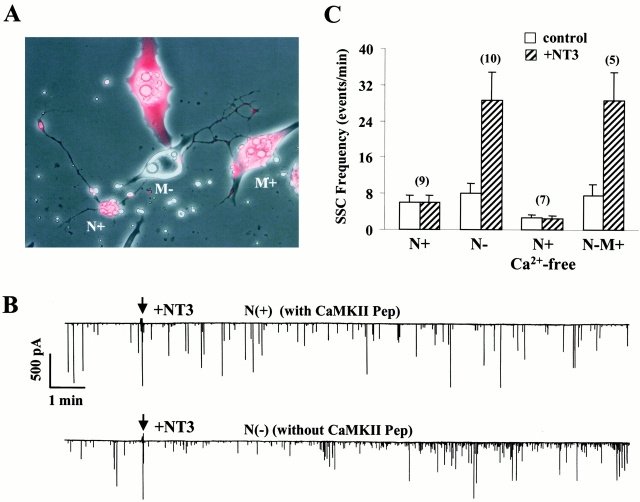
Inhibition of CaMKII prevents the effect of NT3. A peptide inhibitor for CaMKII (CaMKII-pep), together with rhodamine-dextran, was injected into Xenopus embryo at the two-cell stage, and the nerve–muscle co-cultures were prepared from the injected embryos. (A) Super-imposed phase and fluorescence micrographs of the 1-d-old nerve–muscle co-cultures showing cells loaded with (+) or without (−) CaMKII-pep. N, spinal neurons; M, myocytes. (B) A pair of recordings showing that loading of CaMKII peptide into presynaptic neurons N(+) prevented the NT3-induced increase in SSC frequency, whereas NT3 still enhanced transmitter release in a N(−) neuron in the same culture. (C) Summary of the effect of CaMKII-pep loaded into either presynaptic spinal neurons or postsynaptic myocytes. The number of synapses recorded are indicated above each pair of columns. In all N− conditions, application of NT3 elicited a significant increase in SSC frequency (P < 0.01, t test).
Fig. 5 B shows SSCs recorded from a pair of synapses in which the presynaptic neurons were loaded with or without CaMKII-pep into normal Ca2+ medium, respectively. At the CaMKII-pep (−) synapse, application of NT3 still resulted in a marked increase in SSC frequency (Fig. 5B and Fig. C). In contrast, loading of CaMKII-pep into the presynaptic neurons completely prevented the effects of NT3 (Fig. 5B and Fig. C). Similar to KN62, loading of CaMKII-pep to the presynaptic neurons did not affect basal spontaneous transmitter release, but blocked the effect of NT3 (Fig. 5 C). Similar results were obtained in Ca2+-free medium (Fig. 5 C). Furthermore, when CaMKII-pep was loaded into the postsynaptic myocytes (M+), NT3 was still capable of eliciting a significant increase in SSC frequency (Fig. 5 C). Taken together, these results strongly suggest that potentiation of transmitter release at the developing neuromuscular synapses by NT3 is achieved through the activation of CaMKII in the presynaptic neurons, but not the postsynaptic muscle cells.
Does the NT3-induced potentiation require a continuous activation of CaMKII? To address this question, we applied KN62 after synaptic transmission was potentiated by NT3. Fig. 6 A shows that within ~20–30 min after NT3 application, the increase in SSC frequency reached the peak. Application of KN62 at the peak gradually suppressed the SSC frequency. Quantitative analysis indicated that KN62 virtually reversed the NT3 effect (Fig. 6 B). Thus, continuous activity of CaMKII appears to be necessary for NT3 modulation of transmitter release at the developing neuromuscular synapses.
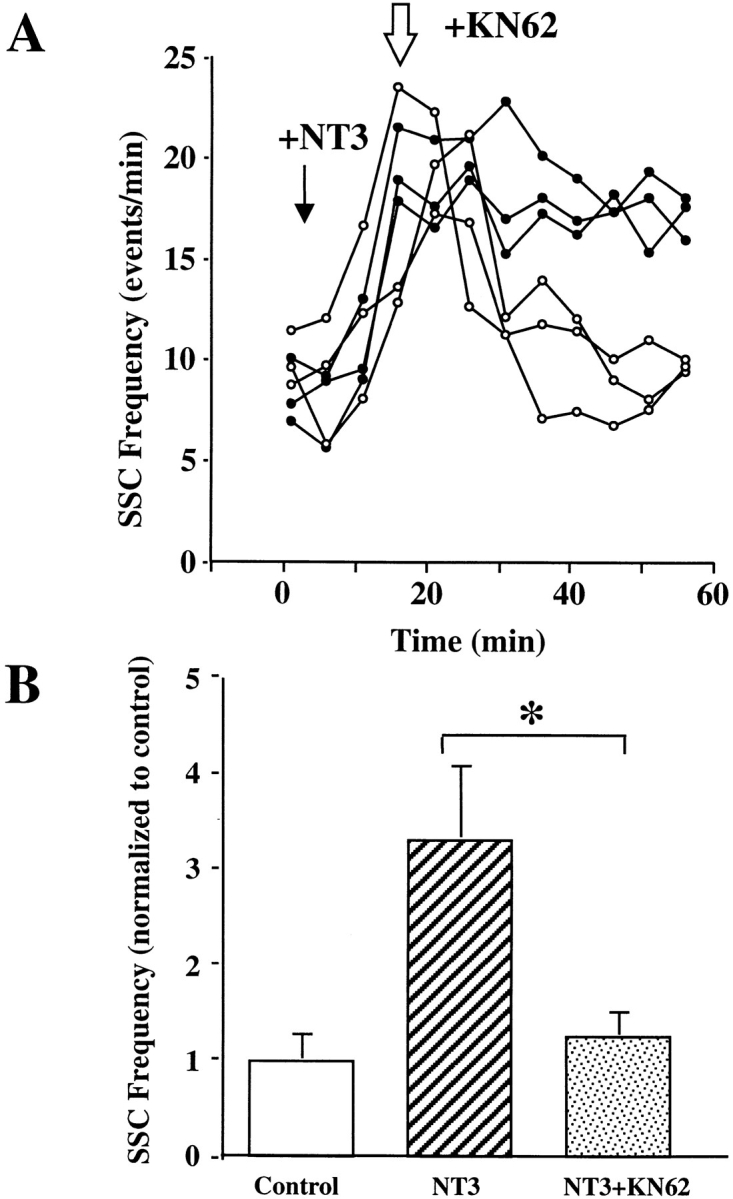
Reversal of NT3 effects by addition of KN62 after NT3 application. (A) Examples showing the time courses that KN62 reverses the NT3 effect. NT3 was first applied (filled arrow) to the culture medium to elicit an increase in the frequency of SSCs. After the SSC frequency reached the peak, KN62 (open arrow) was either applied (open circles) or not applied (filled circles), and synaptic currents were continuously monitored. (B) Summary of the reversal effect of KN62. SSC frequencies are calculated in the same way as Fig. 3 C. n = 5. Asterisk indicates P < 0.01, t test.
Discussion
Previous work has shown that neurotrophins rapidly potentiate synaptic transmission through presynaptic mechanisms. The acute potentiation of transmitter release by BDNF is accompanied by a rise in [Ca2+]i in both the NMJ (Stoop and Poo 1995) and at the CNS synapses (Berninger and Garcia 1993; Marsh and Palfrey 1996; Sakai et al. 1997; Li et al. 1998). However, it is unclear whether and how the increase in [Ca2+]i mediates the neurotrophin-induced synaptic potentiation and which downstream signaling events are involved. In this paper we report a surprising finding that the acute potentiation of transmitter release by NT3 at the neuromuscular synapses is independent of Ca2+ influx from extracellular sources. Instead, this potentiation is mediated by Ca2+ released from intracellular stores through IP3 and ryanodine receptors. Thus, the mechanisms by which BDNF and NT3 modulate transmitter release could be quite different. Furthermore, we demonstrated that Ca2+ released from intracellular stores is capable of activating CaMKII, and the continuous activation of CaMKII is required for the effect of NT3. Taken together, this study provides, to our knowledge, the first evidence for a link between neurotrophins and CaMKII. These findings may provide new insights into the general mechanisms of neurotransmitter release and exocytosis, and how neurotrophic factors may regulate these processes.
In addition to extracellular Ca2+, the release of Ca2+ from intracellular stores could either modulate or contribute directly to transmitter release (Berridge 1998). Signals that result in the opening of either IP3 receptors or ryanodine receptors can generate local increases in [Ca2+]i, which in turn participates in the exocytotic process. Although still a fairly new concept, transmitter release triggered or modulated by the release of Ca2+ from intracellular stores has been shown in a number of systems such as the cholinergic synapse in Aplysia, reticulospinal synapse in lamprey, and sympathetic nerve terminals (Smith and Cunnane 1996; Cochilla and Alford 1998; Mothet et al. 1998). An important question then is whether neurotrophins, which are capable of eliciting an IP3 signal through the activation of phospholipase-γ pathway (Segal and Greenberg 1996), can serve as endogenous neuromodulators to regulate synaptic transmission under physiological conditions. In this study, we have provided strong evidence that NT3 potentiates transmitter release by stimulating Ca2+ release from intracellular stores. We have shown that NT3 increased transmitter release in Ca2+-free or Cd2+-containing medium, and that pretreatment with thapsigargin prevented the NT3 effect. Moreover, inhibition of IP3 receptors blocked the NT3 effect. Thus, NT3 induces the release of Ca2+ through IP3 receptors at the terminals of developing spinal neurons, leading to an increase in spontaneous transmitter secretion. It is conceivable that similar mechanisms are used for NT3 to enhance evoked synaptic transmission, although we could not test this possibility because most of our experiments have to be done in Ca2+-free medium. Consistent with our results, neurotrophins have been shown to induce an increase in [Ca2+]i in hippocampal neurons (Berninger and Garcia 1993; Marsh and Palfrey 1996), possibly by enhancing the release of Ca2+ from intracellular stores (Sakai et al. 1997; Li et al. 1998). The release of Ca2+ from IP3 receptors could further trigger Ca2+-induced Ca2+ release from ryanodine receptors (Berridge 1998). We found that the acute modulation of transmitter release by NT3 was blocked by the ryanodine receptor antagonist TMB-8 or a high concentration of ryanodine (100 μM). However, activation of the ryanodine receptor alone by low concentrations of ryanodine (2.5–5 μM) was not sufficient to enhance transmitter release, and application of NT3 on top of that still increased SSC frequency. Thus, both IP3 receptors and ryanodine receptors are involved in the acute effect of NT3, but the primary effect of NT3 is probably on the IP3 receptors. Although the electrophysiological analysis clearly indicates that NT3 potentiates neurotransmitter release through presynaptic mechanisms, our pharmacological experiments can not formally establish that NT3 acts on presynaptic terminals directly. We can not rule out the possibility that NT3 initially acts on postsynaptic muscle cells to trigger the release of Ca2+ from intracellular stores, leading to the secretion of some retrograde signal to activate presynaptic CaMKII.
Although our results suggest a role of Ca2+ release from internal stores in NT3-induced synaptic potentiation, a previous study has shown that the acute potentiation by BDNF in the same preparation requires external Ca2+ (Stoop and Poo 1996). BDNF binds and interacts almost exclusively with the TrkB receptor, whereas NT3 binds primarily to the TrkC receptor (Kaplan and Stephens 1994). It is possible that the activation of TrkB triggers Ca2+ influx, whereas that of TrkC is coupled to internal Ca2+ stores in the developing spinal neurons. Indeed, we found that inhibition of Ca2+ release from internal stores can not block the BDNF-induced synaptic potentiation. Similarly, both BDNF and NT3 attract growth cone turning in these developing spinal neurons, but the intracellular mechanisms that mediate the turning responses to the two factors are completely different (Song et al. 1998). The BDNF effect requires Ca2+ influx into the terminals and elevation of [cAMP]i, whereas the NT3 effect is independent of extracellular Ca2+. In this study, we show that the activation of IP3 receptors is required for the synaptic effect of NT3, but not for that of BDNF. Thus, although both enhance synaptic transmission at developing neuromuscular synapses, the two factors may require Ca2+ from difference sources, one extracellular and one intracellular.
The potentiation of transmitter release usually occurs at least 5–10 min after NT3 application (Fig. 1, Fig. 2, and Fig. 6; see also Lohof et al. 1993; Xie et al. 1997). This time course implies that NT3-induced Ca2+ release modulates the transmitter release mechanisms, rather than contributing directly to the triggering of the exocytosis process. The NT3 modulation is known to be presynaptic in nature (Lohof et al. 1993). What are the presynaptic targets downstream of Ca2+ release induced by NT3? CaMKII may serve as an excellent candidate, because its role in transmitter release is relatively well-defined (Llinas et al. 1985; Lin et al. 1990; Nichols et al. 1990; Stanton and Gage 1996; Jin et al. 1998; for review see Greengard et al. 1993). Extensive studies indicate that the activation of CaMKII is triggered by Ca2+ influx through extracellular sources. An important finding in this study is that CaMKII can also be activated by Ca2+ released from internal stores through IP3 and ryanodine receptors. We showed that even in the complete absence of Ca2+ influx, the NT3-induced potentiation of transmitter release can be blocked by the CaMKII inhibitors CaMKII-pep or KN62. Furthermore, we found that KN62 can reverse established synaptic potentiation after NT3 application in Ca2+-free medium. These results not only provide a link between internal Ca2+ stores and CaMKII activation, but also point to CaMKII as a downstream signaling mediator for NT3-induced synaptic potentiation. Since it is difficult to test whether the activation of CaMKII alone is sufficient to mimic the NT3 effect, we can not rule out the possible involvement of other processes that may also contribute to the NT3-induced synaptic potentiation.
Acknowledgments
The authors wish to express their gratitude to Drs. Doug Fields, Lucas Pozzo-Miller, Stanko Stojikovic, and members of the Lu laboratory for helpful discussions and critical comments on the manuscript. We would also like to thank Regeneron Pharmaceuticals for providing recombinant NT3.
Footnotes
Z.-P. Xie's current address is Department of Biology, Tsinghua University, Beijing, China.
Abbreviations used in this paper: BDNF, brain-derived neurotrophic factor; [Ca2+]i, Ca2+ concentration(s); CaMKII, Ca2+/calmodulin-dependent kinase II; CNS, central nervous system; IP3, inositol 1, 4, 5-trisphosphate; NMJ, neuromuscular junction; NT, neurotrophin; SSC, spontaneous synaptic current; XeC, xestospongin C.
References
- Alder J., Xie Z.-P., Valtorta F., Greengard P., Poo M.-M. Antibodies to synaptophysin interfere with transmitter secretion at neuromuscular synapses. Neuron. 1992;9:759–768. [Abstract] [Google Scholar]
- Berninger B., Garcia D.E. BDNF and NT-3 induce intracellular Ca2+ elevation in hippocampal neurons. Neuroreport. 1993;4:1303–1306. [Abstract] [Google Scholar]
- Berridge M.J. Neuronal calcium signaling. Neuron. 1998;21:13–26. [Abstract] [Google Scholar]
- Cabelli R.J., Horn A., Shatz C.J. Inhibition of ocular dominance column formation by infusion of NT-4/5 or BDNF. Science. 1995;267:1662–1666. [Abstract] [Google Scholar]
- Cabelli R.J., Shelton D.L., Segal R.A., Shatz C.J. Blockade of endogenous ligands of trkB inhibits formation of ocular dominance columns. Neuron. 1997;19:63–76. [Abstract] [Google Scholar]
- Chapman P.F., Frenguelli B.G., Smith A., Chen C.M., Silva A.J. The alpha-Ca2+/calmodulin kinase IIa bidirectional modulator of presynaptic plasticity. Neuron. 1995;14:591–597. [Abstract] [Google Scholar]
- Cochilla A.J., Alford S. Metabotropic glutamate receptor-mediated control of neurotransmitter release. Neuron. 1998;20:1007–1016. [Abstract] [Google Scholar]
- Enslen H., Sun P., Brickey D., Soderling S.H., Klamo E., Soderling T.R. Characterization of Ca2+/calmodulin-dependent protein kinase IV. Role in transcriptional regulation. J. Biol. Chem. 1994;269:15520–15527. [Abstract] [Google Scholar]
- Figurov A., Pozzo-Miller L., Olafsson P., Wang T., Lu B. Regulation of synaptic responses to high-frequency stimulation and LTP by neurotrophins in the hippocampus. Nature. 1996;381:706–709. [Abstract] [Google Scholar]
- Gafni J., Munsch J.A., Lam T.H., Catlin M.C., Costa L.G., Molinski T.F., Pessah I.N. Xestosponginspotent membrane permeable blockers of the inositol 1,4,5-trisphosphate receptor. Neuron. 1997;19:723–733. [Abstract] [Google Scholar]
- Gonzalez M., Ruggiero F.P., Chang Q., Shi Y.J., Rich M.M., Kraner S., Balice-Gordon R.J. Disruption of Trkb-mediated signaling induces disassembly of postsynaptic receptor clusters at neuromuscular junctions. Neuron. 1999;24:567–583. [Abstract] [Google Scholar]
- Gottschalk W.A., Jiang H., Tartaglia N., Feng L., Figurov A., Lu B. Signaling mechanisms mediating BDNF modulation of synaptic plasticity in the hippocampus. Learn. Mem. 1999;6:243–256. [Europe PMC free article] [Abstract] [Google Scholar]
- Greengard P., Valtorta F., Czernik A.J., Benfenati F. Synaptic vesicle phosphoproteins and regulation of synaptic function. Science. 1993;259:780–785. [Abstract] [Google Scholar]
- Hunt J.M., Silinsky E.M., Hirsh J.K., Ahn D., Solsona C. The effects of TMB-8 on acetylcholine release from frog motor nerveinteractions with adenosine. Eur. J. Pharmacol. 1990;178:259–266. [Abstract] [Google Scholar]
- Ishida A., Kameshita I., Okuno S., Kitani T., Fujisawa H. A novel highly specific and potent inhibitor of calmodulin-dependent protein kinase II. Biochem. Biophys. Res. Commun. 1995;212:806–812. [Abstract] [Google Scholar]
- Jin P., Griffith L.C., Murphey R.K. Presynaptic calcium/calmodulin-dependent protein kinase II regulates habituation of a simple reflex in adult Drosophila . J. Neurosci. 1998;18:8955–8964. [Europe PMC free article] [Abstract] [Google Scholar]
- Kaplan D.R., Stephens R.M. Neurotrophin signal transduction by the Trk receptor. J. Neurobiol. 1994;25:1404–1417. [Abstract] [Google Scholar]
- Kokaia M., Asztely F., Olofsdotter K., Sindreu C.B., Kullmann D.M., Lindvall O. Endogenous neurotrophin-3 regulates short-term plasticity at lateral perforant path-granule cell synapses. J. Neurosci. 1998;18:8730–8739. [Europe PMC free article] [Abstract] [Google Scholar]
- Korte M., Carroll P., Wolf E., Brem G., Thoenen H., Bonhoeffer T. Hippocampal long-term potentiation is impaired in mice lacking brain-derived neurotrophic factor. Proc. Natl. Acad. Sci. USA. 1995;92:8856–8860. [Europe PMC free article] [Abstract] [Google Scholar]
- Li Y.X., Zhang Y., Lester H.A., Schuman E.M., Davidson N. Enhancement of neurotransmitter release induced by brain-derived neurotrophic factor in cultured hippocampal neurons. J. Neurosci. 1998;18:10231–10240. [Europe PMC free article] [Abstract] [Google Scholar]
- Lin J.-W., Sugimori M., Llinas R.R., McGuinness T.L., Greengard P. Effects of synapsin I and calcium/calmodulin-dependent protein kinase II on spontaneous neurotransmitter release in the squid giant synapse. Proc. Natl. Acad. Sci. USA. 1990;87:8257–8261. [Europe PMC free article] [Abstract] [Google Scholar]
- Liou J.C., Fu W.M. Regulation of quantal secretion from developing motoneurons by postsynaptic activity-dependent release of NT-3. J. Neurosci. 1997;17:2459–2468. [Europe PMC free article] [Abstract] [Google Scholar]
- Liou J.C., Yang R.S., Fu W.M. Regulation of quantal secretion by neurotrophic factors at developing motoneurons in Xenopus cell cultures. J. Physiol. 1997;503:129–139. [Abstract] [Google Scholar]
- Llinas R., McGuinness T.L., Leonard C.S., Sugimori M., Greengard P. Intra-terminal injection of synapsin I or calcium/calmodulin-dependent protein kinase II alters neurotransmitter release at the squid giant synapse. Proc. Natl. Acad. Sci. USA. 1985;82:3035–3039. [Europe PMC free article] [Abstract] [Google Scholar]
- Lohof A.M., Ip N.Y., Poo M.M. Potentiation of developing neuromuscular synapses by the neurotrophins NT-3 and BDNF. Nature. 1993;363:350–353. [Abstract] [Google Scholar]
- Lu B., Figurov A. Role of neurotrophins in synapse development and plasticity. Rev. Neurosci. 1997;8:1–12. [Abstract] [Google Scholar]
- Lu B., Greengard P., Poo M.-M. Exogenous synapsin I promotes functional maturation of developing neuromuscular synapses. Neuron. 1992;8:521–529. [Abstract] [Google Scholar]
- Ma L., Reis G., Parada L.F., Schuman E.M. Neuronal NT-3 is not required for synaptic transmission or long-term potentiation in area CA1 of the adult rat hippocampus. Learn. Mem. 1999;6:267–275. [Europe PMC free article] [Abstract] [Google Scholar]
- Marsh H.N., Palfrey H.C. Neurotrophin-3 and brain-derived neurotrophic factor activate multiple signal transduction events but are not survival factors for hippocampal pyramidal neurons. J. Neurochem. 1996;67:952–963. [Abstract] [Google Scholar]
- McAllister A.K., Lo D.C., Katz L.C. Neurotrophins regulate dendritic growth in developing visual cortex. Neuron. 1995;15:791–803. [Abstract] [Google Scholar]
- McAllister A.K., Katz L.C., Lo D.C. Opposing roles for endogenous BDNF and NT-3 in regulating cortical dendritic growth. Neuron. 1997;18:767–778. [Abstract] [Google Scholar]
- McAllister A.M., Katz L.C., Lo D.C. Neurotrophins and synaptic plasticity. Annu. Rev. Neurosci. 1999;22:295–318. [Abstract] [Google Scholar]
- Mothet J.P., Fossier P., Meunier F.M., Stinnakre J., Tauc L., Baux G. Cyclic ADP-ribose and calcium-induced calcium release regulate neurotransmitter release at a cholinergic synapse of Aplysia. J. Physiol. 1998;507:405–414. [Abstract] [Google Scholar]
- Nichols R.A., Sihra T.S., Czernik A.J., Nairn A.C., Greengard P. Calcium/calmodulin-dependent protein kinase II increases glutamate and noradrenaline release from synaptosomes. Nature. 1990;343:647–651. [Abstract] [Google Scholar]
- Patterson S.L., Abel T., Deuel T.A., Martin K.C., Rose J.C., Kandel E.R. Recombinant BDNF rescues deficits in basal synaptic transmission and hippocampal LTP in BDNF knockout mice. Neuron. 1996;16:1137–1145. [Abstract] [Google Scholar]
- Riddle D.R., Lo D.C., Katz L.C. NT-4-mediated rescue of lateral geniculate neurons from effects of monocular deprivation. Nature. 1995;378:189–191. [Abstract] [Google Scholar]
- Sakai N., Yamada M., Numakawa T., Ogura A., Hatanaka H. BDNF potentiates spontaneous Ca2+ oscillations in cultured hippocampal neurons. Brain Res. 1997;778:318–328. [Abstract] [Google Scholar]
- Segal R.A., Greenberg M.E. Intracellualr signaling pathways activated by neurotrophic factors. Annu. Rev. Neurosci. 1996;19:463–489. [Abstract] [Google Scholar]
- Smith A.B., Cunnane T.C. Ryanodine-sensitive calcium stores involved in neurotransmitter release from sympathetic nerve terminals of the guinea-pig. J. Physiol. 1996;497:657–664. [Abstract] [Google Scholar]
- Song H.J., Ming G., Fon L.E., Bellocchio E., Edwards R.H., Poo M.M. Expression of a putative vesicular acetylcholine transporter facilitates quantal transmitter packaging. Neuron. 1997;18:815–826. [Abstract] [Google Scholar]
- Song H., Ming G., He Z., Lehmann M., Tessier-Lavigne M., Poo M. Conversion of neuronal growth cone responses from repulsion to attraction by cyclic nucleotides. Science. 1998;281:1515–1518. [Abstract] [Google Scholar]
- Stanton P.K., Gage A.T. Distinct synaptic loci of Ca2+/calmodulin-dependent protein kinase II necessary for long-term potentiation and depression. J. Neurophysiol. 1996;76:2097–2101. [Abstract] [Google Scholar]
- Stoop R., Poo M.M. Potentiation of transmitter release by ciliary neurotrophic factor requires somatic signaling. Science. 1995;267:695–699. [Abstract] [Google Scholar]
- Stoop R., Poo M.M. Synaptic modulation by neurotrophic factorsdifferential and synergistic effects of brain-derived neurotrophic factor and ciliary neurotrophic factor. J. Neurosci. 1996;16:3256–3264. [Europe PMC free article] [Abstract] [Google Scholar]
- Thastrup O., Cullen P.J., Drobak B.K., Hanley M.R., Dawson A.P. Thapsigargin, a tumor promoter, discharges intracellular Ca2+ stores by specific inhibition of the endoplasmic reticulum Ca2+-ATPase. Proc. Natl. Acad. Sci. USA. 1990;87:2466–2470. [Europe PMC free article] [Abstract] [Google Scholar]
- Tokumitsu H., Chijiwa T., Hagiwara M., Mizutani A., Terasawa M., Hidaka H. KN-62, 1-[N,O-bis(5-isoquinolinesulfonyl)-N-methyl-L-tyrosyl]-4-phenylpiperazine, a specific inhibitor of Ca2+/calmodulin-dependent protein kinase II. J. Biol. Chem. 1990;265:4315–4320. [Abstract] [Google Scholar]
- Wang T., Xie K.W., Lu B. Neurotrophins promote maturation of developing neuromuscular synapses. J. Neurosci. 1995;15:4796–4805. [Europe PMC free article] [Abstract] [Google Scholar]
- Wang X.H., Poo M.M. Potentiation of developing synapses by postsynaptic release of neurotrophin-4. Neuron. 1997;19:825–835. [Abstract] [Google Scholar]
- Xie K., Wang T., Olafsson P., Mizuno K., Lu B. Activity-dependent expression of NT-3 in muscle cells in cultureimplication in the development of neuromuscular junctions. J. Neurosci. 1997;17:2947–2958. [Europe PMC free article] [Abstract] [Google Scholar]
Articles from The Journal of Cell Biology are provided here courtesy of The Rockefeller University Press
Full text links
Read article at publisher's site: https://doi.org/10.1083/jcb.149.4.783
Read article for free, from open access legal sources, via Unpaywall:
https://rupress.org/jcb/article-pdf/149/4/783/1291278/0002067.pdf
Citations & impact
Impact metrics
Citations of article over time
Article citations
Neurotrophin-3 Rescues Striatal Synaptic Plasticity in Model of Neurodegeneration by PLC Signaling Activation.
CNS Neurol Disord Drug Targets, 23(12):1488-1498, 01 Jan 2024
Cited by: 0 articles | PMID: 38859788
Alignment of Skeletal Muscle Cells Facilitates Acetylcholine Receptor Clustering and Neuromuscular Junction Formation with Co-Cultured Human iPSC-Derived Motor Neurons.
Cells, 11(23):3760, 24 Nov 2022
Cited by: 2 articles | PMID: 36497020 | PMCID: PMC9738074
The Calcium/Calmodulin-Dependent Kinases II and IV as Therapeutic Targets in Neurodegenerative and Neuropsychiatric Disorders.
Int J Mol Sci, 22(9):4307, 21 Apr 2021
Cited by: 18 articles | PMID: 33919163 | PMCID: PMC8122486
Review Free full text in Europe PMC
CaMKII requirement for the persistence of in vivo hippocampal mossy fiber synaptic plasticity and structural reorganization.
Neurobiol Learn Mem, 139:56-62, 27 Dec 2016
Cited by: 2 articles | PMID: 28039086
Brain-derived neurotrophic factor inhibits neuromuscular junction maturation mediated by inTracellular Ca(2+) and Ca(2+)/calmodulin-dependent kinase.
Muscle Nerve, 53(4):593-597, 01 Apr 2016
Cited by: 1 article | PMID: 26248508
Go to all (49) article citations
Data
Similar Articles
To arrive at the top five similar articles we use a word-weighted algorithm to compare words from the Title and Abstract of each citation.
PI-3 kinase and IP3 are both necessary and sufficient to mediate NT3-induced synaptic potentiation.
Nat Neurosci, 4(1):19-28, 01 Jan 2001
Cited by: 120 articles | PMID: 11135641
Postsynaptic secretion of BDNF and NT-3 from hippocampal neurons depends on calcium calmodulin kinase II signaling and proceeds via delayed fusion pore opening.
J Neurosci, 27(39):10350-10364, 01 Sep 2007
Cited by: 125 articles | PMID: 17898207 | PMCID: PMC6673152
Postsynaptic TRPC1 function contributes to BDNF-induced synaptic potentiation at the developing neuromuscular junction.
J Neurosci, 31(41):14754-14762, 01 Oct 2011
Cited by: 10 articles | PMID: 21994391 | PMCID: PMC3213207
BDNF-induced local protein synthesis and synaptic plasticity.
Neuropharmacology, 76 Pt C:639-656, 16 Apr 2013
Cited by: 314 articles | PMID: 23602987
Review