Abstract
Free full text

Salmonella Exploits Caspase-1 to Colonize Peyer's Patches in a Murine Typhoid Model
Abstract
Salmonella typhimurium invades host macrophages and induces apoptosis and the release of mature proinflammatory cytokines. SipB, a protein translocated by Salmonella into the cytoplasm of macrophages, is required for activation of Caspase-1 (Casp-1, an interleukin [IL]-1β–converting enzyme), which is a member of a family of cysteine proteases that induce apoptosis in mammalian cells. Casp-1 is unique among caspases because it also directly cleaves the proinflammatory cytokines IL-1β and IL-18 to produce bioactive cytokines. We show here that mice lacking Casp-1 (casp-1 −/ − mice) had an oral S. typhimurium 50% lethal dose (LD50) that was 1,000-fold higher than that of wild-type mice. Salmonella breached the M cell barrier of casp-1 −/ − mice efficiently; however, there was a decrease in the number of apoptotic cells, intracellular bacteria, and the recruitment of polymorphonuclear lymphocytes in the Peyer's patches (PP) as compared with wild-type mice. Furthermore, Salmonella did not disseminate systemically in the majority of casp-1 −/ − mice, as demonstrated by significantly less colonization in the PP, mesenteric lymph nodes, and spleens of casp-1 −/ − mice after an oral dose of S. typhimurium that was 100-fold higher than the LD50. The increased resistance in casp-1 −/ − animals appears specific for Salmonella infection since these mice were susceptible to colonization by another enteric pathogen, Yersinia pseudotuberculosis, which normally invades the PP. These results show that Casp-1, which is both proapoptotic and proinflammatory, is essential for S. typhimurium to efficiently colonize the cecum and PP and subsequently cause systemic typhoid-like disease in mice.
Introduction
Salmonellae cause a variety of disease syndromes, ranging from gastroenteritis to systemic infections like typhoid fever. After ingestion, Salmonella reaches the small intestine where it invades the mucosa by crossing the epithelial barrier through M cells overlying the lymphoid follicles of Peyer's patches (PP). Salmonella invasion results in characteristic tissue changes, including infiltration of polymorphonuclear lymphocytes (PMNs) and monocytes, which contribute to the erosion of the intestinal mucosa 1. After Salmonella traverses the epithelial barrier, it encounters tissue macrophages and other host cells in the lamina propria. After replication in the PP, the bacteria enter the lymphatics and bloodstream and spread to deeper tissues 2. Salmonella invasion of host cells depends on bacterial proteins encoded in the chromosomal locus Salmonella pathogenicity island 1 (SPI1). SPI1 genes encode components of a type III secretion apparatus, regulatory proteins, and secreted effector proteins and their chaperones 1. In an oral inoculation, SPI1-defective mutants are attenuated in their ability to cause systemic infections, yet they are fully virulent if the bacteria are injected intraperitoneally 3 4 5 6. Thus, SPI1 appears to be necessary for the initial phase of the disease process.
The SPI1-encoded SipB protein (Salmonella invasion protein) is required to promote inflammation in infected ileum 7, to cause diarrhea in a calf model 1 8, and to promote host cell invasion through aiding in the delivery of additional effector molecules 9 10 11 12. SipB interacts with Casp-1, which we believe leads to its activation within the macrophage cytoplasm, resulting in apoptosis 13. Unlike other mice with targeted deletions in other caspase genes, the casp-1 −/ − mice do not display any defects in apoptotic pathways involved in development, homeostasis, or T cell selection 14. Macrophages from casp-1 −/ − mice are resistant to Salmonella typhimurium–induced apoptosis 13, although they still undergo apoptosis in response to several physiological stimuli 15 16; macrophages from casp-3 −/ − and casp-11 −/ − mice remain susceptible to Salmonella-induced apoptosis (Hersh, D., unpublished observation). In addition to playing a role in the apoptotic pathway in macrophages 17 18, Casp-1 cleaves the proinflammatory cytokines IL-1β and IL-18 to their mature forms 16. The biologically inactive forms of these two cytokines are stored in the cytoplasm of macrophages 19 20 21 22. Indeed, Salmonella infection of cultured macrophages and dendritic cells induces secretion of active IL-1β and IL-18 13 23 24, suggesting that Salmonella-induced apoptosis is proinflammatory. Here we report on the role of the Salmonella-induced apoptotic and inflammatory response in the outcome of this host–pathogen interaction in vivo.
Materials and Methods
Bacterial Strains.
Wild-type S. typhimurium SL1344 25 and the isogenic orgA mutant strain BJ66 3 were grown overnight with aeration in Luria broth (LB) before oral inoculation of mice. Bacteria were grown standing overnight in high-salt LB before inoculation of ligated loops. For gentamicin protection assays performed in peritoneal macrophages, SL1344 was grown overnight with aeration, followed by a 15-min incubation at 37°C in normal mouse serum to opsonize bacteria.
Mice.
8–10-wk-old B10.RIII-H2rH2-T18b(17NS)/Sn casp-1 +/+ nramp s/s (The Jackson Laboratory), C57BL/6 H-2b casp-1 +/+ nramp s/s (The Jackson Laboratory), and B10.RIII-H2r H2-T18b(17NS)/Sn casp-1 −/ − nramp s/s (Merck Research Laboratories) mice were kept under specific pathogen–free conditions in filter-top cages. Interbreeding of mice lacking casp-1, which were backcrossed eight generations onto the B10.RIII background, was performed to obtain the casp-1 −/ − mice. Mice were provided with sterile water and food ad libitum.
Oral Inoculation of Mice.
Wild-type and casp-1 −/ − mice were deprived of food 14 h before orogastric inoculation. For LD50 calculations, 35 casp-1 +/+ and 35 casp-1 −/ − mice were inoculated orogastrically through a gastric tube, with serial 10-fold dilutions (n = 5 mice/inoculum) ranging from 1.38 × 104 to 1.38 × 1010 CFU. The health of the animals was followed for 30 d after inoculation, and deaths were recorded. For colonization expermiments, mice were inoculated orogastrically with the indicated dose of wild-type S. typhimurium SL1344. Mice were killed on days 0.5, 1, 2, and 4 followed by dissection of cecum, PP, mesenteric lymph nodes (MLNs), and spleens. Tissues were homogenized and weighed, and dilutions were plated on Luria agar plates containing Streptomycin (200 μg/ml) to obtain CFU per gram tissue.
Ligated Intestinal Loop Model and Measurement of Gentamicin-protected Bacteria.
Mice were starved for 15 h before they were anesthetized by i.p. inoculation of 1.5 mg pentobarbital sodium as previously described 26. A 10–15-cm intestinal loop was injected with 108 SL1344. At 0.5, 1, 2, and 3 h after infection, PP were dissected away from the small intestine and processed for transmission electron microscopy 26. Separate mice were used for the measurement of gentamicin-protected bacteria. At 0.5 and 3 h, the intestinal loops were flushed three times with 10 ml of PBS containing gentamicin (100 μg/ml), followed by dissection of individual PP, which were then incubated separately with gentle agitation in 1 ml of DMEM supplemented with 10% fetal bovine serum and 100 μg/ml gentamicin for 100 min at 37°C. This was followed by two 10-min washes in 1 ml of PBS to clear the gentamicin. Each PP was homogenized and serial dilutions were plated as described above.
Histology and TUNEL Reactions on Fixed PP.
For histological examinations and TUNEL (TdT dUTP-biotin nick-end labeling) reactions on tissue sections, PP were fixed in 10% buffered neutral formalin, embedded in paraffin, and sectioned. Some sections were then stained with hematoxylin and eosin. Sections for TUNEL reactions were processed as described for the In Situ Cell Death Detection Kit, Fluorescein (Boehringer). The sections were labeled for 1 h at 37°C, rinsed with PBS, incubated in anti-Salmonella polyclonal rabbit antiserum, and finally incubated with anti–rabbit-TRITC antibody (Sigma-Aldrich) and ToTo-3 (Molecular Probes) to stain host cell nuclei. Coverslips were mounted over antiquench and sealed, and tissue sections were analyzed on a confocal microscope (MRC1024; Bio-Rad Laboratories). Tissues for electron microscopy were prepared exactly as previously described 26.
Peritoneal Macrophages and Gentamicin Protection Assay.
Macrophages were elicited to the peritoneum of mice and isolated 4 d after injection of 3% thioglycolate broth. After 2 h, nonadherent cells were removed and the remaining macrophages (2.5 × 105/well) were incubated in DMEM supplemented with 10% heat-inactivated FCS, 50 μg/ml penicillin, 50 μg/ml streptomycin, 50 IU/ml IFN-γ (Sigma-Aldrich), and 50 ng/ml LPS from Escherichia coli (Sigma-Aldrich) at 37°C, 5% CO2 overnight. The monolayers were then washed with fresh tissue culture media before infections. Infections were performed with a multiplicity of infection of 10 to 1. Bacteria were spun onto the cells at 1,000 rpm for 5 min and incubated for 30 min, followed by incubation in 100 μg/ml gentamicin for 90 min. The tissue culture media was then replaced with a minimum inhibitory concentration of gentamicin (10 μg/ml) for the remainder of the assay. At 2, 4, 8, and 24 h the cells were lysed with 1% Triton X-100 for 10 min, diluted with LB, and plated for CFU per well.
Statistical Analysis.
The colonizations of tissue from wild-type and casp-1 −/ − mice were compared by the Mann-Whitney U test. F-test for analysis of variance revealed P < 0.0001 for all tissues on days 1, 2, and 4. The mean gentamicin-protected CFU were compared by Student's t test.
Results
Oral LD50 for S. typhimurium is 1,000-fold Higher in casp-1−/− Mice.
To assess the role of the host protein, Casp-1, during Salmonella infection, we determined the LD50 for mice lacking this enzyme. Mice were inoculated intragastrically with 10-fold serial dilutions of the wild-type S. typhimurium strain SL1344. The infected animals were followed for 30 d and the estimated value for the 50% death point was calculated by the Reed-Muench method 27. The oral LD50 in casp-1 −/ − mice was 1.67 × 108, which was 1,000-fold higher than the 1.56 × 105 LD50 value obtained for wild-type mice. To allow for comparisons with published reports using the susceptible mouse strain C57BL/6, the oral LD50 in C57BL/6 was determined to be 2.4 × 105.
casp-1−/− PP Colonization Is Significantly Lower after Oral Inoculation with S. typhimurium.
We orally infected casp-1 −/ − mice with wild-type S. typhimurium to investigate at what stage of a systemic infection Casp-1 plays a role. Wild-type and casp-1 null mice were inoculated orogastrically with 2 × 107 wild-type S. typhimurium, a dose that is ~100-fold above the LD50 in wild-type mice. casp-1 −/ − mice were colonized in the cecum but not in the PP at 2 and 4 d after inoculation (Fig. 1, a and b). Two out of five casp-1 −/ − mice were colonized in the MLNs at both 2 and 4 d, whereas all of the wild-type mice were colonized (P = 0.032 for 2 d after inoculation; P = 0.0083 for 4 d after inoculation). By 4 d, all wild-type mice were heavily colonized in the spleens, whereas three of the casp-1 −/ − mice were not colonized, with two mice containing fewer than 20 CFU (P = 0.0207). Thus, a dose of wild-type S. typhimurium that is sufficient to colonize and eventually kill all wild-type mice poorly colonized the casp-1 −/ − mice.
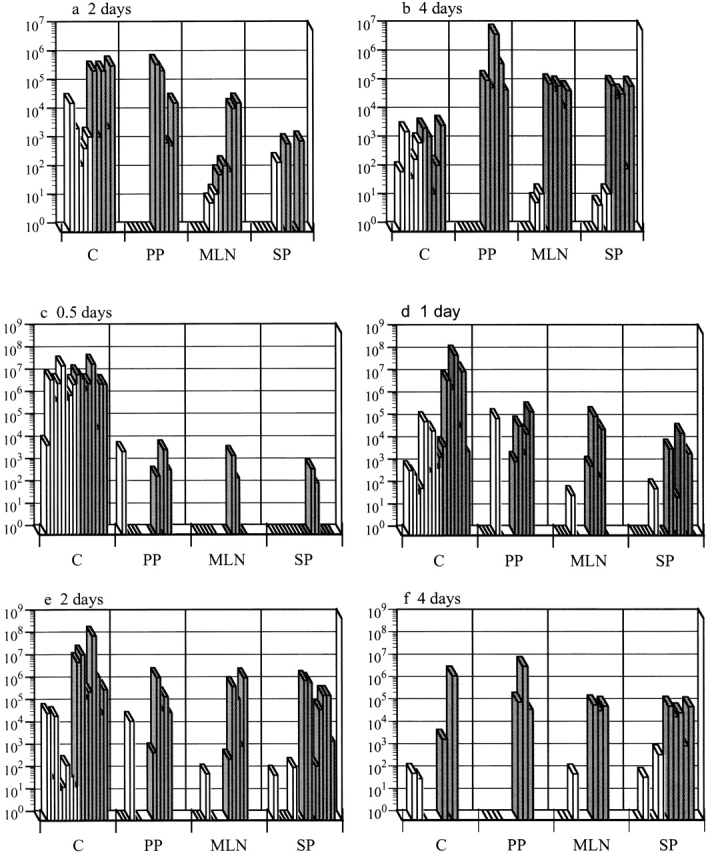
Casp-1−/− mice are resistant to S. typhimurium colonization after an oral infection. Wild-type mice (gray bars) and casp-1− / − mice (white bars) were inoculated intragastrically with wild-type S. typhimurium. The tissue colonization of mice inoculated with 2 × 107 CFU of S. typhimurium SL1344 was determined for (a) 2 and (b) 4 d after inoculation. C, cecum; PP, Peyer's patches; MLN, mesenteric lymph nodes; SP, spleen. n = 5 for both days. Tissue colonization after intragastric inoculation with 1010 CFU of S. typhimurium SL1344 for (c) 0.5, (d) 1, (e) 2, and (f) 4 d after inoculation. Cecum and spleen, n = 8 for days 0.5, 1, and 2; n = 3 for day 4. PP and MLNs, n = 5 for days 0.5, 1, and 2; n = 3 for day 4. Day 1 for PP and MLNs for wild-type compared with casp-1 −/ − mice: P = 0.0236 and P = 0.0343, respectively. Day 2 for PP, MLNs, spleens, and livers: P = 0.0132, P = 0.0343, P = 0.0025, and P = .0283, respectively. Day 4 for PP, MLNs, spleens, and livers: P = 0.0369, P = 0.0038, P = 0.0207, and P = 0.0278, respectively. Rectangles on the x axis represent zero bacterial colonies present in undiluted tissue. These data represent results from one experiment, performed on age-matched casp-1 −/ − and wild-type mice, which is representative of three experiments. The variability in mouse colonization at early times is reproducible and typical of what is seen after an oral inoculation.
We next inoculated mice with 1010 CFU of wild-type S. typhimurium, which is ~100-fold higher than the LD50 for casp-1 null mice. In this experiment, the casp-1 −/ − mice were colonized at early time points in the cecum but not in the PP, whereas wild-type mice were colonized at both sites (Fig. 1c and Fig. d). 1 d after infection with this high dose, the PP and MLNs of wild-type mice had significantly more bacteria than these same tissues in casp-1 −/ − animals (Fig. 1 d). By day 2 of infection, Salmonella were found in large numbers in the spleens of wild-type mice, whereas the casp-1 −/ − mice still were not significantly colonized in any of the tissues tested except the cecum (Fig. 1 e). By day 4 of infection, wild-type but not casp-1 −/ − animals were noticeably ill and bacteria were present in all tissues tested. The number of bacteria on day 4 was significantly higher in all tissues of casp-1 +/+ than in casp-1 −/ − mice, except in the cecum (Fig. 1 f). Eventually the casp-1 −/ − mice died when inoculated with such a high dose, but the time to death was very different from that of wild-type mice. The mean time to death was 5.4 d for wild-type mice, whereas the mean time to death for casp-1 −/ − mice was 9.6 d (P = 0.0106).
Increased Apoptosis in Wild-type PP Correlates with Colonization.
To test whether Salmonella induces apoptosis in PP and whether this apoptosis is Casp-1 dependent, apoptotic cells within PP sections of infected mice were detected by the TUNEL reaction 25. The PP were studied in the ligated intestinal loop system, which allows for the analysis of early time points. At 1 h after infection, wild-type PP contained far more TUNEL+ cells than did casp-1 −/ − or uninfected PP from either mouse background. Furthermore, many apoptotic cells colocalized with bacteria in the dome area of wild-type PP (Fig. 2). Thus, in wild-type mice Salmonella induced many cells in the PP to undergo apoptosis either directly or indirectly, whereas in casp-1 −/ − mice Salmonella induced relatively few cells to undergo apoptosis. Furthermore, Salmonella failed to survive in casp-1 −/ − mice.
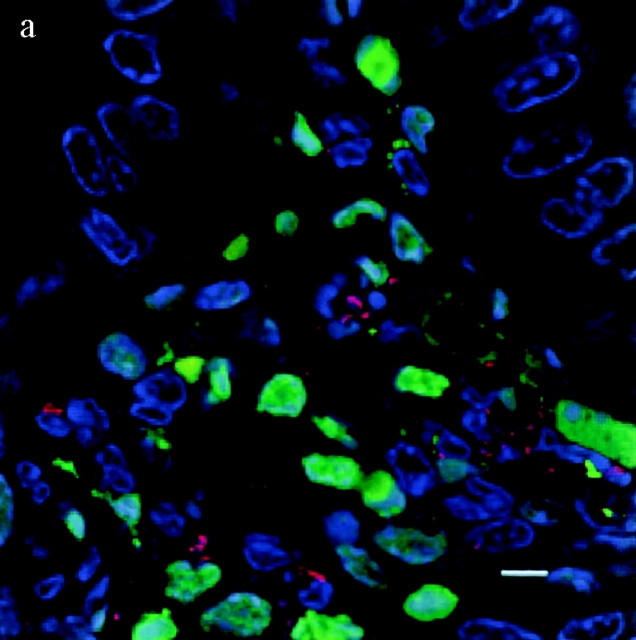
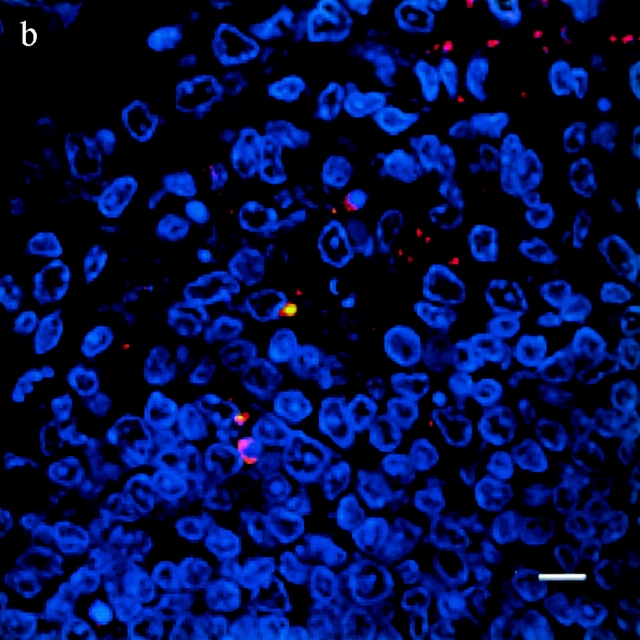
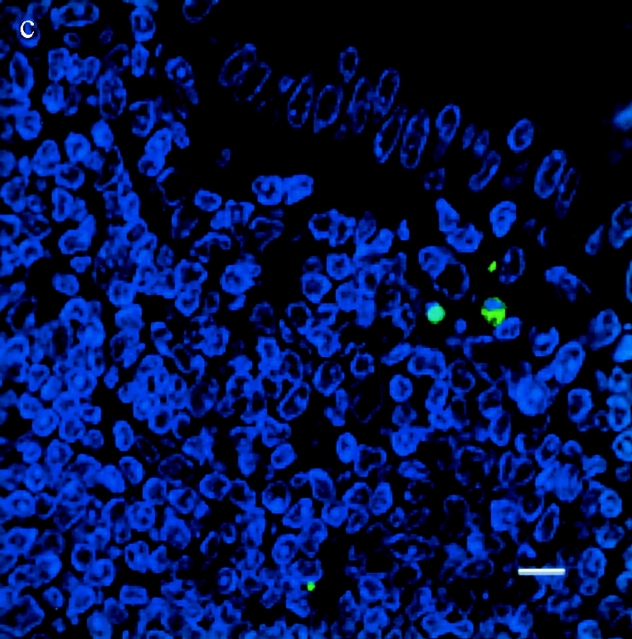
TUNEL+ cells colocalize with S. typhimurium in the dome area of PP from casp-1 +/+ mice. Cross sections of PP from ligated intestinal loops performed in (a) wild-type or (b) casp-1 −/ − mice infected with wild-type Salmonella or (c) wild-type mice inoculated with LB for 1 h were fixed and stained for apoptotic nuclei in green, Salmonella in red, and host cell nuclei in blue. The scalebar represents 8 μm in a and 10 μm in b and c. Images are oriented such that the intestinal lumen is at the top and the lymphoid follicle (not shown) is toward the bottom.
The Early Colonization in Wild-type PP Is Associated with an Increase in Inflammatory Cells and an Increase in Intracellular Salmonella by 3 h in the PP.
Bacterial colony counts and tissue samples for microscopic examination were obtained from the ligated loops of wild-type and casp-1 −/ − mice infected for 0.5, 1, and 3 h. At 0.5 h after infection in both wild-type and casp-1 −/ − mice, Salmonella invaded M cells and produced the characteristic membrane ruffles followed by the destruction of M cells (28; Fig. 3, a and b). At 1 h, the macrophages in the dome area of wild-type PP were killed and extruded into the intestinal lumen, and bacteria were extracellular (Fig. 3 c). By 3 h, the epithelium overlying the PP in wild-type mice was virtually destroyed by invading Salmonella and there were many PMNs containing bacteria that were localized to the dome area (Fig. 3e and Fig. g). In contrast, in PP from casp-1 −/ − mice infected for 1 h, the majority of bacteria were inside monocytes (Fig. 3 d). By 3 h, very few bacteria were observed and the casp-1 −/ − PP, which showed little inflammation, were devoid of PMNs (Fig. 3f and Fig. h). Thus, Salmonella initiates an inflammatory response in casp-1 +/+ mice, presumably due in part to Casp-1–mediated IL-1β and IL-18 activation.
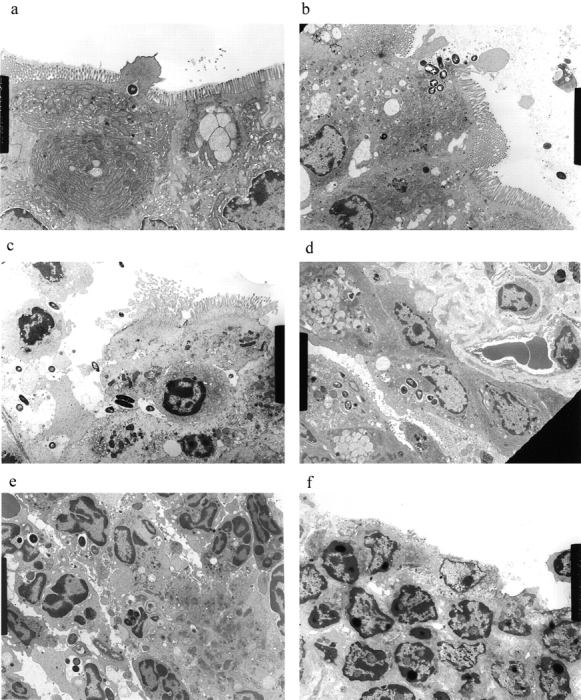
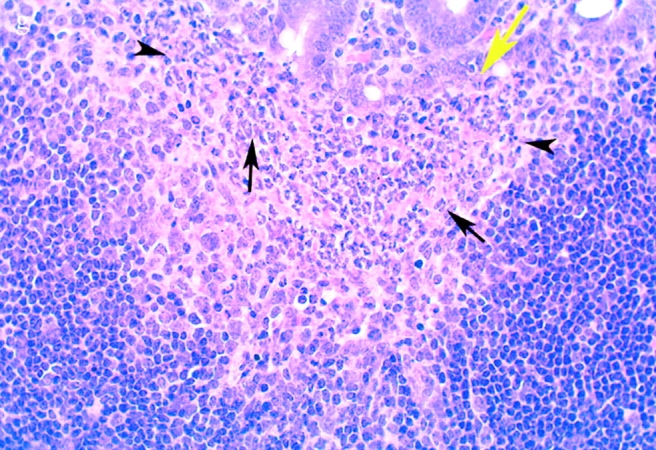
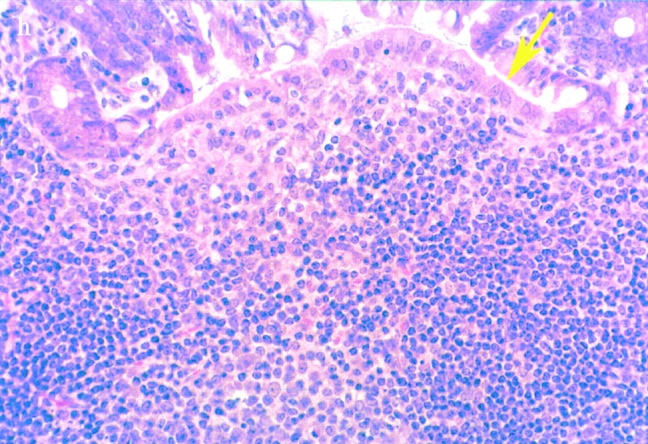
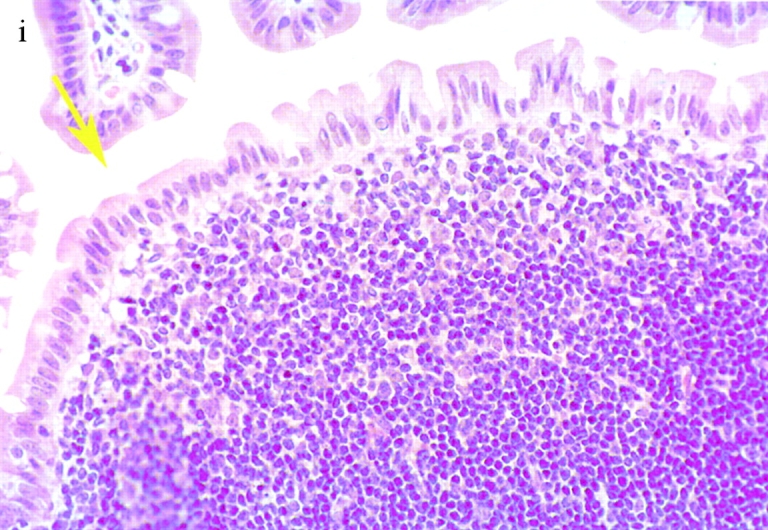
(continues on facing page). S. typhimurium induces inflammation in wild-type PP but not in casp-1 −/ − PP. Transmission electron micrographs of infected PP revealed bacterial invasion of M cells at 0.5 h in wild-type (a) and casp-1 −/ − (b) PP, and at 1 h in (c) extracellular Salmonella in wild-type and (d) intracellular bacteria in casp-1 −/ − PP. At 3 h, wild-type PP dome revealed an infiltration of PMNs and intracellular Salmonella (e), whereas casp-1 −/ − PP revealed an absence of PMNs and bacteria (f). Hematoxylin and eosin stains of PP from casp-1 +/+ (g) mice infected for 3 h revealed clusters of PMNs (black arrows) and pyknotic cells (arrowheads), whereas casp-1 −/ − PP did not (h). Uninfected, wild-type PP are shown for comparison (i). Yellow arrows point to the PP dome area. Serial tissue sections were stained with anti-Salmonella antibody to confirm that the PP shown was infected. All sections are oriented with intestinal lumen at the top and lymphoid follicle at the bottom.
We quantitated the observed decline in bacteria during the 3-h ligated loop experiment. The number of intracellular S. typhimurium within PP after infection was measured using a gentamicin protection assay to enrich for intracellular bacteria 29. Consistent with the histopathological findings, 10-fold more gentamicin-resistant (intracellular) bacteria were enumerated in the casp-1 −/ − mice than in the wild-type mice at this 0.5-h time point (Fig. 4). In contrast to wild-type mice at 3 h, there was a fivefold decrease in bacteria in the casp-1 −/ − animals, consistent with the dwindling numbers seen on microscopic analysis. Thus, wild-type Salmonella can efficiently invade casp-1 −/ − PP, but is unable to survive within them.
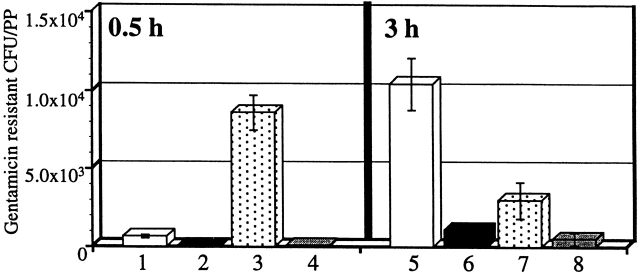
S. typhimurium CFU decrease in casp-1 −/ − PP. Ligated intestinal loops were performed for 0.5 and 3 h and gentamicin-protected CFU per PP was determined. Lanes 1 (n = 9) and 5 (n = 10), casp-1 +/+ mice infected with SL1344. Lanes 2 (n = 9) and 6 (n = 11), casp-1 +/+ mice infected with noninvasive mutant BJ66 3. Lanes 3 (n = 10) and 7 (n = 13,) casp-1 −/ − mice infected with SL1344. Lanes 4 (n = 12) and 8 (n = 12), casp-1 −/ − mice infected with BJ66. Unpaired t test revealed P < 0.0001 and P = 0.0013 for SL1344 in casp-1 −/ − compared with casp-1 +/+ at 0.5 and 3 h, respectively; P = 0.0001 for SL1344 in casp-1 +/+ mice for 0.5 versus 3 h; P = 0.0028 for SL1344 in casp-1 −/ − mice for 0.5 versus 3 h. The noninvasive mutant produced numbers that were not statistically different between the two mouse strains. The mean and standard deviations are shown from two separate experiments.
casp-1−/− and Wild-type Mice Are Equally Susceptible to Intraperitoneally Injected Salmonella.
The oral colonization data suggested that Casp-1 plays a role in the initial intestinal colonization phase of Salmonella infections, but we could not determine what role, if any, Casp-1 might play in the colonization of other tissues. To determine whether Casp-1 is required for Salmonella to colonize extraintestinal organs, the gastrointestinal tract was bypassed by inoculating mice intraperitoneally. Under this protocol, casp-1 −/ − and wild-type mice had similar infection progressions (1.92 × 105 ± 4.06 × 104 and 2.77 × 105 ± 3.29 × 104 CFU/gram per wild-type and casp-1 −/ − spleen, respectively, 2 d after inoculation). Furthermore, these two strains of mice showed equal mortality after i.p. inoculation with 100 wild-type S. typhimurium (Fig. 5). These data show that Casp-1 activity is not needed for successful infection by the i.p. route, but appears to be essential in order for Salmonella to establish a successful gastrointestinal infection by the mucosal route.
Intracellular Survival of Salmonella Is the Same in Macrophages from Wild-type and casp-1−/− Mice in an In Vitro Assay.
Many reports correlate the ability of S. typhimurium to cause mouse typhoid fever with in vitro bacterial survival within cultured macrophages 30 31 32 33. To determine whether the failure of Salmonella to disseminate in casp-1 −/ − mice was due to an increased killing of Salmonella by macrophages from casp-1 −/ − mice, Salmonella interactions with peritoneal macrophages derived from wild-type and casp-1 −/ − mice were compared in vitro. We tested the killing of bacteria under conditions where apoptosis of the macrophages was eliminated either by growing wild-type S. typhimurium to late stationary phase or by using a mutant S. typhimurium that cannot deliver SipB 25. No difference in survival over the course of the 24-h assay was found in the killing of Salmonella (Fig. 6). The initial recovery of intracellular bacteria at 2 h was the same for both wild-type and casp-1 −/ − macrophages. This was followed by similar levels of bacteria killing, nearly 1.5 logs, over the 24-h assay. Therefore, macrophages from casp-1 −/ − mice are not inherently different in their capacity to kill Salmonella compared with wild-type–derived macrophages.
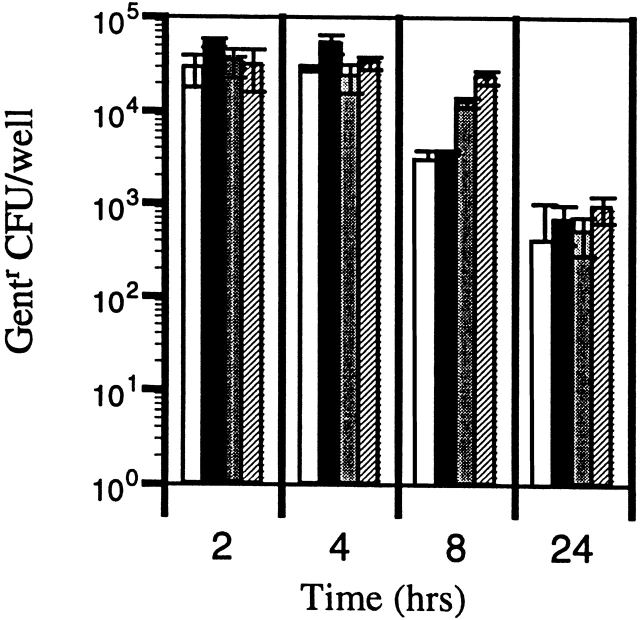
Activated peritoneal macrophages from wild-type and casp-1 −/ − mice kill S. typhimurium in the absence of apoptosis. Peritoneal macrophages from wild-type mice were infected with opsonized, stationary phase SL1344 (white bars) or the noninvasive S. typhimurium mutant, BJ66 (black bars). Peritoneal macrophages from casp-1 −/ − mice were infected with opsonized, stationary phase SL1344 (gray bars) or the noninvasive S. typhimurium mutant, BJ66 (hatched bars). The multiplicity of infection was 10 bacteria per macrophage. Under these conditions, there was no macrophage death as measured by assaying the culture supernatants for the presence of lactate dehydrogenase with a Cytotox96 Cell Death Kit (Promega).
The PP, MLNs, and Spleens of casp-1−/− and Wild-type Mice Are Equally Colonized by Yersinia pseudotuberculosis.
Could another enteric pathogen, which has been shown to induce apoptosis in macrophages in vitro by a Casp-1–independent mechanism 34, colonize the casp-1 −/ − mice? To test this idea, we inoculated wild-type and casp-1 −/ − mice with 109 CFU wild-type Y. pseudotuberculosis, a dose that is fivefold higher than the Y. pseudotuberculosis LD50 for wild-type mice. In contrast to Salmonella, Y. pseudotuberculosis colonized the cecum and PP of both mouse strains (Fig. 7, a and b). The bacteria then spread to the MLNs and spleens of both wild-type and casp-1 −/ − mice in equal numbers (Fig. 7, a and b). Thus, we conclude that the relative inability of Salmonella to colonize the PP of casp-1 −/ − mice was not due to a general antimicrobial compensatory mechanism specific to the genetic deficiency in the Casp-1 knockout mice.
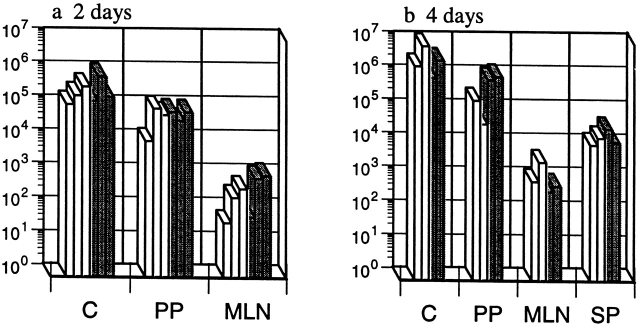
casp-1 −/ − mice are colonized with Y. pseudotuberculosis. Wild-type mice (gray bars) and casp-1 −/− mice (white bars) were inoculated intragastrically with a wild-type Y. pseudotuberculosis strain, YPIIIpYV. C, cecum; PP, Peyer's patches; MLN, mesenteric lymph nodes; SP, spleen. n = 3 for day 2; n = 2 for day 4.
Discussion
Previously we and others have shown that Salmonella induces apoptosis in macrophages and that during in vitro infection of macrophages this induction is dependent on the SPI1-encoded type III secretion protein SipB 13 25 35. We have also shown that Salmonella-induced apoptosis is dependent on the host protein Casp-1 13. We find in this study that mice deficient for the host cysteine protease, Casp-1, are markedly resistant to colonization by wild-type S. typhimurium. Indeed, it requires 1,000-fold more bacteria by oral challenge to kill 50% of the casp-1 −/ − mice as compared with wild-type mice. Yet, when casp-1 −/ − mice are challenged intraperitoneally with Salmonella, there is no difference in susceptibility compared with wild-type mice as measured by spleen colonization or mortality. Thus, Casp-1 plays an essential role, that of allowing for bacterial colonization, in the innate immunity of the gastrointestinal tract to Salmonella infection.
We further addressed this role of Casp-1 in innate immunity by analyzing PP infected in a ligated intestinal loop model by confocal and electron microscopy as well as by a gentamicin protection assay. Salmonella was able to invade and induce membrane ruffling on the surface of M cells in the absence of Casp-1. At 1 h after infection, PP from wild-type but not from casp-1 −/ − mice contained a significant increase in the number of cells undergoing apoptosis, both by electron microscopy and by an apoptotic-specific DNA stain (TUNEL reaction). Although some of the TUNEL+ cells in the dome area of the PP colocalized with Salmonella, we cannot rule out the possibility that after initial bacterium-dependent apoptosis there is rapid cytokine-dependent apoptosis of neighboring cells. Nevertheless, the apoptosis observed within 1 h of PP infection was Casp-1 dependent. The increase in apoptosis in the infected wild-type PP correlated with an increase in intracellular Salmonella and inflammation. Taken together, these data highlight the consequences of the Salmonella–Casp-1 interaction. Early in infection of the PP, the bacteria escape the macrophages by inducing apoptosis. The simultaneous recruitment of PMNs and other cellular elements of the immune system to the site of the infection appears to provide a new intracellular niche for the bacteria. In the casp-1 −/ − mice, the lack of apoptosis and failure to recruit new immune cells results in the bacteria remaining within the host immune cells underlying the follicle-associated epithelium, which are likely to include resident macrophages and dendritic cells. Presumably the intracelluar bacteria in the casp-1 −/ − mice are eliminated by the resident immune cells. Salmonella survive within an intracellular site of wild-type PP, and whether or not PMNs are a safe haven at early times in the PP for intracellular Salmonella as suggested in the spleen remains to be determined 36 37.
Furthermore, Y. pseudotuberculosis, an enteric pathogen that induces Casp-1–independent macrophage apoptosis, infects both wild-type and casp-1 null mice normally. Thus, Y. pseudotuberculosis, which also targets M cells to invade PP 38 39 and induces apoptosis of macrophages in vitro and of Mac-1+ cells in vivo 40 41 42 when administered orally, was able to colonize the cecum, PP, MLNs, and spleens of both wild-type and casp-1 null mice.[Q7] Therefore, the inability of S. typimurium to disseminate from the intestine to the spleens of casp-1 −/ − mice is not due to a mechanism that is nonspecifically inhibiting the dissemination of all enteric pathogens, and instead specifially requires Casp-1.
The data presented here, together with the identification of SipB as necessary for the activation of Casp-1 13, indicate that SPI1-encoded proteins are important for the invasion of intestinal epithelium, which then allows for the subsequent dissemination of bacteria after oral infection 4 5 6. Recently, an SPI1-independent route for bacterial dissemination outside the gastrointestinal tract was described 43. This additional route is dependent on CD-18 cell types, which include macrophages and dendritic cells. It is noteworthy that CD-18–deficient mice are resistant to infection by this pathway. We believe that the five casp-1 −/ − mice that contained low levels of Salmonella in the spleen but were not colonized in the PP (Fig. 1) were colonized via the CD-18–dependent route.
Richter-Dahlfors et al. have shown that S. typhimurium can induce apoptosis of macrophages in the liver after an intravenous route of inoculation 44. The roles of Casp-1 and SipB were not investigated. Our i.p. inoculation data indicate that, similar to SipB, Casp-1 is not necessary for Salmonella virulence once the bacteria have breached the gastrointestinal barrier. Thus, the apoptosis found in the liver 44 is either Casp-1 independent or not critical for Salmonella survival and replication in the spleen and liver.
The induction of Casp-1–dependent apoptosis immediately following Salmonella entry into PP coincided with the recruitment of PMNs and other immune cells and is associated with tissue destruction. Our data do not indicate whether both the proapoptotic and the proinflammatory activities of Casp-1 are necessary for Salmonella to successfully colonize the PP. We are currently conducting experiments to address the relative contributions of these proinflammatory cytokines to PP colonization and systemic spread. Shigella, the causative agent of bacterial dysentery, which induces Casp-1–dependent apoptosis in macrophages through IpaB, a homologue of SipB, also initiates inflammation by a Casp-1–dependent mechanism 29 45. Thus, Salmonella and Shigella appear to share the initial steps in setting up a gastrointestinal infection even though they produce quite different diseases.
The mechanisms of immunity to Salmonella require the action of many cytokines and soluble factors including IL-18, TNF-α, IL-12, IFN-γ, and nitric oxide 9 10 11 12 23 46 47 48 49 50 51 52 53 54 55 56. Thus, it is likely that once the bacteria breach the mucosal surface to enter the PP, there are multiple host defense and bacterial virulence factors that come into play. However, we have shown that the absence of a single host cell enzyme, Casp-1, renders mice resistant to Salmonella gastrointestinal infection. Thus, in wild-type mice, Casp-1 activation is a critical step in the establishment of a systemic disease.
Since Casp-1 can trigger a proinflammatory response, which is considered an antimicrobial host response, it is perhaps initially counterintuitive to learn that a host enzyme that initiates a proinflammatory response is required by Salmonella to produce a successful infection. One interpretation of this result is that Salmonella does not require the proinflammatory response at all and that the proapoptotic Casp-1 activity is all that is necessary for PP colonization. Even so, it seems remarkable that in casp-1 −/ − mice the invading Salmonella do not simply avoid phagocytosis by entering the more numerous cell populations within the PP, such as B and T lymphocytes or the adjacent epithelium. Furthermore, the findings that some Salmonella mutants that are unable to induce apoptosis in vitro are not attenuated for virulence 57 indicate that this interpretation is too simplistic. The alternative explanation, which we favor, is that it is not only the capacity of Salmonella to induce apoptosis of PP macrophages that is required, but the inflammatory cascade induced by Salmonella is also required for bacteria to cross cellular barriers and allow for their eventual replication and persistence in the reticuloendothelial system. The precise timing with which this pathogen triggers this cascade is probably important to the establishment of infection. Our results underscore the idea that bacterial pathogens have evolved to not only circumvent or subvert a host defense mechanism, but indeed to directly manipulate the host innate immune system.
Acknowledgments
We thank Joan Mecsas, Corrella Detweiler, David Discher, and Igor Brodsky for critical reading of the manuscript. We thank John Mudgett at Merck Research Laboratories for providing the casp-1 −/ − mice.
This work was funded in part by National Institutes of Health Grant AI-42780 (to A. Zychlinsky), Public Health Service grant RO1 AI-26195, and the Defense Advanced Research Project Agency (to S. Falkow). Additional funds were provided by Protein Design Labs.
Footnotes
Abbreviations used in this paper: Casp-1, Caspase-1; LB, Luria broth; MLNs, mesenteric lymph nodes; PMNs, polymorphonuclear lymphocytes; PP, Peyer's patches; SPI1, Salmonella pathogenicity island 1; TUNEL, TdT dUTP-biotin nick-end labeling.
References
- Darwin K.H., Miller V.L. Molecular basis of the interaction of Salmonella with the intestinal mucosa. Clin. Microbiol. Rev. 1999;12:405–428. [Europe PMC free article] [Abstract] [Google Scholar]
- Jones B.D., Falkow S. Salmonellosishost immune responses and bacterial virulence determinants. Annu. Rev. Immunol. 1996;14:533–561. [Abstract] [Google Scholar]
- Jones B.D., Falkow S. Identification and characterization of a Salmonella typhimurium oxygen-regulated gene required for bacterial internalization. Infect. Immun. 1994;62:3745–3752. [Europe PMC free article] [Abstract] [Google Scholar]
- Baumler A.J., Tsolis R.M., Valentine P.J., Ficht T.A., Heffron F. Synergistic effect of mutations in invA and lpfC on the ability of Salmonella typhimurium to cause murine typhoid. Infect. Immun. 1997;65:2254–2259. [Europe PMC free article] [Abstract] [Google Scholar]
- Behlau I., Miller S.I. A PhoP-repressed gene promotes Salmonella typhimurium invasion of epithelial cells. J. Bacteriol. 1993;175:4475–4484. [Europe PMC free article] [Abstract] [Google Scholar]
- Penheiter K.L., Mathur N., Giles D., Fahlen T., Jones B.D. Non-invasive Salmonella typhimurium mutants are avirulent because of an inability to enter and destroy M cells of ileal Peyer's patches. Mol. Microbiol. 1997;24:697–709. [Abstract] [Google Scholar]
- Galyov E.E., Wood M.W., Rosqvist R., Mullan P.B., Watson P.R., Hedges S., Wallis T.S. A secreted effector protein of Salmonella dublin is translocated into eukaryotic cells and mediates inflammation and fluid secretion in infected ileal mucosa. Mol. Microbiol. 1997;25:903–912. [Abstract] [Google Scholar]
- Tsolis R.M., Adams L.G., Ficht T.A., Baumler A.J. Contribution of Salmonella typhimurium virulence factors to diarrheal disease in calves. Infect. Immun. 1999;67:4879–4885. [Europe PMC free article] [Abstract] [Google Scholar]
- Collazo C.M., Galan J.E. The invasion-associated type III system of Salmonella typhimurium directs the translocation of Sip proteins into the host cell. Mol. Microbiol. 1997;24:747–756. [Abstract] [Google Scholar]
- Fu Y., Galan J.E. The Salmonella typhimurium tyrosine phosphatase SptP is translocated into host cells and disrupts the actin cytoskeleton. Mol. Microbiol. 1998;27:359–368. [Abstract] [Google Scholar]
- Kaniga K., Uralil J., Bliska J.B., Galan J.E. A secreted protein tyrosine phosphatase with modular effector domains in the bacterial pathogen Salmonella typhimurium . Mol. Microbiol. 1996;21:633–641. [Abstract] [Google Scholar]
- Wood M.W., Rosqvist R., Mullan P.B., Edwards M.H., Galyov E.E. SopE, a secreted protein of Salmonella dublin, is translocated into the target eukaryotic cell via a sip-dependent mechanism and promotes bacterial entry. Mol. Microbiol. 1996;22:327–338. [Abstract] [Google Scholar]
- Hersh D., Monack D.M., Smith M.R., Ghori N., Falkow S., Zychlinsky A. The Salmonella invasin SipB induces macrophage apoptosis by binding to caspase-1. Proc. Natl. Acad. Sci. USA. 1999;96:2396–2401. [Europe PMC free article] [Abstract] [Google Scholar]
- Los M., Wesselborg S., Schulze-Osthoff K. The role of caspases in development, immunity, and apoptotic signal transductionlessons from knockout mice. Immunity. 1999;10:629–639. [Abstract] [Google Scholar]
- Li P., Allen H., Banerjee S., Franklin S., Herzog L., Johnston C., Mcdowell J., Pakind M., Rodman L., Salfeld J. Mice deficient in IL-1β-converting enzyme are defective in production of mature IL-1β and resistant to endotoxic shock. Cell. 1995;80:401–411. [Abstract] [Google Scholar]
- Fantuzzi G., Dinarello C.A. Interleukin-18 and interleukin-1 betatwo cytokine substrates for ICE (caspase-1) J. Clin. Immunol. 1999;19:1–11. [Abstract] [Google Scholar]
- Hilbi H., Moss J.E., Hersh D., Chen Y., Arondel J., Banerjee S., Flavell R.A., Yuan J., Sansonetti P.J., Zychlinsky A. Shigella-induced apoptosis is dependent on caspase-1 which binds to IpaB. J. Biol. Chem. 1998;273:32895–32900. [Abstract] [Google Scholar]
- Hilbi H., Chen Y., Thirumalai K., Zychlinsky A. The interleukin 1beta-converting enzyme, caspase 1, is activated during Shigella flexneri-induced apoptosis in human monocyte-derived macrophages. Infect. Immun. 1997;65:5165–5170. [Europe PMC free article] [Abstract] [Google Scholar]
- Black R.A., Kronheim S.R., Cantrell M., Deeley M.C., March C.J., Prickett K.S., Wignall J., Conlon P.J., Cosman D., Hopp T.P. Generation of biologically active interleukin-1 beta by proteolytic cleavage of the inactive precursor. J. Biol. Chem. 1988;263:9437–9442. [Abstract] [Google Scholar]
- Kostura M.J., Tocci M.J., Limjuco G., Chin J., Cameron P., Hillman A.G., Chartrain N.A., Schmidt J.A. Identification of a monocyte specific pre-interleukin 1 beta convertase activity. Proc. Natl. Acad. Sci. USA. 1989;86:5227–5231. [Europe PMC free article] [Abstract] [Google Scholar]
- Fantuzzi G., Puren A.J., Harding M.W., Livingston D.J., Dinarello C.A. Interleukin-18 regulation of interferon gamma production and cell proliferation as shown in interleukin-1beta-converting enzyme (caspase- 1)-deficient mice. Blood. 1998;91:2118–2125. [Abstract] [Google Scholar]
- Pirhonen J., Sareneva T., Kurimoto M., Julkunen I., Matikainen S. Virus infection activates IL-1 beta and IL-18 production in human macrophages by a caspase-1-dependent pathway. J. Immunol. 1999;162:7322–7329. [Abstract] [Google Scholar]
- Mastroeni P., Clare S., Khan S., Harrison J.A., Hormaeche C.E., Okamura H., Kurimoto M., Dougan G. Interleukin 18 contributes to host resistance and gamma interferon production in mice infected with virulent Salmonella typhimurium . Infect. Immun. 1999;67:478–483. [Europe PMC free article] [Abstract] [Google Scholar]
- Marriott I., Hammond T.G., Thomas E.K., Bost K.L. Salmonella efficiently enter and survive within cultured CD11c+ dendritic cells initiating cytokine expression. Eur. J. Immunol. 1999;29:1107–1115. [Abstract] [Google Scholar]
- Monack D.M., Raupach B., Hromockyj A.E., Falkow S. Salmonella typhimurium invasion induces apoptosis in infected macrophages. Proc. Natl. Acad. Sci. USA. 1996;93:9833–9838. [Europe PMC free article] [Abstract] [Google Scholar]
- Jones B.D., Ghori N., Falkow S. Salmonella typhimurium initiates murine infection by penetrating and destroying the specialized epithelial M cells of the Peyer's patches. J. Exp. Med. 1994;180:15–23. [Europe PMC free article] [Abstract] [Google Scholar]
- Reed L.J., Muench H. A simple method of estimating fifty percent endpoints. Am. J. Hyg. 1938;27:493–497. [Google Scholar]
- Jones B., Pascopella L., Falkow S. Entry of microbes into the hostusing M cells to break the mucosal barrier. Curr. Opin. Immunol. 1995;7:474–478. [Abstract] [Google Scholar]
- Zychlinsky A., Thirumalai K., Arondel J., Cantey J.R., Aliprantis A.O., Sansonetti P.J. In vivo apoptosis in Shigella flexneri infections. Infect. Immun. 1996;64:5357–5365. [Europe PMC free article] [Abstract] [Google Scholar]
- Fields P.I., Swanson R.V., Haidaris C.G., Heffron F. Mutants of Salmonella typhimurium that cannot survive within the macrophage are avirulent. Proc. Natl. Acad. Sci. USA. 1986;83:5189–5193. [Europe PMC free article] [Abstract] [Google Scholar]
- Alpuche-Aranda C.M., Berthiaume E.P., Mock B., Swanson J.A., Miller S.I. Spacious phagosome formation within mouse macrophages correlates with Salmonella serotype pathogenicity and host susceptibility. Infect. Immun. 1995;63:4456–4462. [Europe PMC free article] [Abstract] [Google Scholar]
- Lissner C.R., Swanson R.N., O'Brien A.D. Genetic control of the innate resistance of mice to Salmonella typhimuriumexpression of the Ity gene in peritoneal and splenic macrophages isolated in vitro. J. Immunol. 1983;131:3006–3013. [Abstract] [Google Scholar]
- van Dissel J.T., Leijh P.C., van Furth R. Differences in initial rate of intracellular killing of Salmonella typhimurium by resident peritoneal macrophages from various mouse strains. J. Immunol. 1985;134:3404–3410. [Abstract] [Google Scholar]
- Monack D., Falkow S. Apoptosis as a common bacterial virulence strategy. International Journal of Medical Microbiology. 2000;290:7–13. [Abstract] [Google Scholar]
- Chen L.M., Kaniga K., Galan J.E. Salmonella spp. are cytotoxic for cultured macrophages. Mol. Microbiol. 1996;21:1101–1115. [Abstract] [Google Scholar]
- Dunlap N.E., Benjamin W.H., Jr., Berry A.K., Eldridge J.H., Briles D.E. A ‘safe-site’ for Salmonella typhimurium is within splenic polymorphonuclear cells. Microb. Pathog. 1992;13:181–190. [Abstract] [Google Scholar]
- Dunlap N.E., Benjamin W.H., Jr., Briles D.E. The intracellular nature of Salmonella infection during the early stages of mouse typhoid. Immunol. Ser. 1994;60:303–312. [Abstract] [Google Scholar]
- Clark M.A., Hirst B.H., Jepson M.A. M-cell surface beta1 integrin expression and invasin-mediated targeting of Yersinia pseudotuberculosis to mouse Peyer's patch M cells. Infect. Immun. 1998;66:1237–1243. [Europe PMC free article] [Abstract] [Google Scholar]
- Pepe J.C., Wachtel M.R., Wagar E., Miller V.L. Pathogenesis of defined invasion mutants of Yersinia enterocolitica in a BALB/c mouse model of infection. Infect. Immun. 1995;63:4837–4848. [Europe PMC free article] [Abstract] [Google Scholar]
- Monack D.M., Mecsas J., Ghori N., Falkow S. Yersinia signals macrophages to undergo apoptosis and YopJ is necessary for this cell death. Proc. Natl. Acad. Sci. USA. 1997;94:10385–10390. [Europe PMC free article] [Abstract] [Google Scholar]
- Mills S.D., Boland A., Sory M.P., van der Smissen P., Kerbourch C., Finlay B.B., Cornelis G.R. Yersinia enterocolitica induces apoptosis in macrophages by a process requiring functional type III secretion and translocation mechanisms and involving YopP, presumably acting as an effector protein. Proc. Natl. Acad. Sci. USA. 1997;94:12638–12643. [Europe PMC free article] [Abstract] [Google Scholar]
- Monack D.M., Mecsas J., Bouley D., Falkow S. Yersinia-induced apoptosis in vivo aids in the establishment of a systemic infection of mice. J. Exp. Med. 1998;188:2127–2137. [Europe PMC free article] [Abstract] [Google Scholar]
- Vazquez-Torres A., Jones-Carson J., Baumler A.J., Falkow S., Valdivia R., Brown W., Le M., Berggren R., Parks W.T., Fang F.C. Extraintestinal dissemination of Salmonella via CD18-expressing phagocytes. Nature. 1999;401:804–808. [Abstract] [Google Scholar]
- Richter-Dahlfors A., Buchan A.M.J., Finlay B.B. Murine salmonellosis studied by confocal microscopySalmonella typhimurium resides intracellularly inside macrophages and exerts a cytotoxic effect on phagocytes in vivo. J. Exp. Med. 1997;186:569–580. [Europe PMC free article] [Abstract] [Google Scholar]
- Hersh D., Weiss J., Zychlinsky A. How bacteria initiate inflammationaspects of the emerging story. Curr. Opin. Microbiol. 1998;1:43–48. [Abstract] [Google Scholar]
- Hormaeche C.E., Joysey H.S., Desilva L., Izhar M., Stocker B.A. Immunity induced by live attenuated Salmonella vaccines. Res. Microbiol. 1990;141:757–764. [Abstract] [Google Scholar]
- Kagaya K., Watanabe K., Fukazawa Y. Capacity of recombinant gamma interferon to activate macrophages for Salmonella-killing activity. Infect. Immun. 1989;57:609–615. [Europe PMC free article] [Abstract] [Google Scholar]
- Kincy-Cain T., Clements J.D., Bost K.L. Endogenous and exogenous interleukin-12 augment the protective immune response in mice orally challenged with Salmonella dublin . Infect. Immun. 1996;64:1437–1440. [Europe PMC free article] [Abstract] [Google Scholar]
- Maskell D.J., Hormaeche C.E., Harrington K.A., Joysey H.S., Liew F.Y. The initial suppression of bacterial growth in a salmonella infection is mediated by a localized rather than a systemic response. Microb. Pathog. 1987;2:295–305. [Abstract] [Google Scholar]
- Mastroeni P., Arena A., Costa G.B., Liberto M.C., Bonina L., Hormaeche C.E. Serum TNF alpha in mouse typhoid and enhancement of a Salmonella infection by anti-TNF alpha antibodies. Microb. Pathog. 1991;11:33–38. [Abstract] [Google Scholar]
- Mastroeni P., Villarreal-Ramos B., Hormaeche C.E. Effect of late administration of anti-TNF alpha antibodies on a Salmonella infection in the mouse model. Microb. Pathog. 1993;14:473–480. [Abstract] [Google Scholar]
- Mastroeni P., Skepper J.N., Hormaeche C.E. Effect of anti-tumor necrosis factor alpha antibodies on histopathology of primary Salmonella infections. Infect. Immun. 1995;63:3674–3682. [Europe PMC free article] [Abstract] [Google Scholar]
- Mastroeni P., Harrison J.A., Chabalgoity J.A., Hormaeche C.E. Effect of interleukin 12 neutralization on host resistance and gamma interferon production in mouse typhoid. Infect. Immun. 1996;64:189–196. [Europe PMC free article] [Abstract] [Google Scholar]
- Mastroeni P., Harrison J.A., Robinson J.H., Clare S., Khan S., Maskell D.J., Dougan G., Hormaeche C.E. Interleukin-12 is required for control of the growth of attenuated aromatic-compound-dependent salmonellae in BALB/c micerole of gamma interferon and macrophage activation. Infect. Immun. 1998;66:4767–4776. [Europe PMC free article] [Abstract] [Google Scholar]
- Nauciel C., Espinasse-Maes F. Role of gamma interferon and tumor necrosis factor alpha in resistance to Salmonella typhimurium infection. Infect. Immun. 1992;60:450–454. [Europe PMC free article] [Abstract] [Google Scholar]
- Umezawa K., Akaike T., Fujii S., Suga M., Setoguchi K., Ozawa A., Maeda H. Induction of nitric oxide synthesis and xanthine oxidase and their roles in the antimicrobial mechanism against Salmonella typhimurium infection in mice. Infect. Immun. 1997;65:2932–2940. [Europe PMC free article] [Abstract] [Google Scholar]
- Ahmer B.M., van Reeuwijk J., Watson P.R., Wallis T.S., Heffron F. Salmonella SirA is a global regulator of genes mediating enteropathogenesis. Mol. Microbiol. 1999;31:971–982. [Abstract] [Google Scholar]
Articles from The Journal of Experimental Medicine are provided here courtesy of The Rockefeller University Press
Full text links
Read article at publisher's site: https://doi.org/10.1084/jem.192.2.249
Read article for free, from open access legal sources, via Unpaywall:
https://rupress.org/jem/article-pdf/192/2/249/1128905/000593.pdf
Citations & impact
Impact metrics
Citations of article over time
Article citations
Harnessing Pyroptosis for Cancer Immunotherapy.
Cells, 13(4):346, 16 Feb 2024
Cited by: 3 articles | PMID: 38391959 | PMCID: PMC10886719
Review Free full text in Europe PMC
Salmonella manipulates the host to drive pathogenicity via induction of interleukin 1β.
PLoS Biol, 22(1):e3002486, 18 Jan 2024
Cited by: 0 articles | PMID: 38236896 | PMCID: PMC10826948
Short-term dietary changes can result in mucosal and systemic immune depression.
Nat Immunol, 24(9):1473-1486, 14 Aug 2023
Cited by: 13 articles | PMID: 37580603 | PMCID: PMC10457203
Unraveling the role of type 1 fimbriae in Salmonella pathogenesis: insights from a comparative analysis of Salmonella Enteritidis and Salmonella Gallinarum.
Poult Sci, 102(8):102833, 03 Jun 2023
Cited by: 0 articles | PMID: 37356296 | PMCID: PMC10404763
Understanding the Mechanism of Antimicrobial Resistance and Pathogenesis of Salmonella enterica Serovar Typhi.
Microorganisms, 10(10):2006, 11 Oct 2022
Cited by: 6 articles | PMID: 36296282 | PMCID: PMC9606911
Review Free full text in Europe PMC
Go to all (150) article citations
Data
Similar Articles
To arrive at the top five similar articles we use a word-weighted algorithm to compare words from the Title and Abstract of each citation.
General and specific host responses to bacterial infection in Peyer's patches: a role for stromelysin-1 (matrix metalloproteinase-3) during Salmonella enterica infection.
Mol Microbiol, 64(1):94-110, 01 Apr 2007
Cited by: 16 articles | PMID: 17376075
Role of sigma factor RpoS in initial stages of Salmonella typhimurium infection.
Infect Immun, 65(5):1814-1823, 01 May 1997
Cited by: 84 articles | PMID: 9125566 | PMCID: PMC175223
The lpf fimbrial operon mediates adhesion of Salmonella typhimurium to murine Peyer's patches.
Proc Natl Acad Sci U S A, 93(1):279-283, 01 Jan 1996
Cited by: 153 articles | PMID: 8552622 | PMCID: PMC40222
Salmonella-induced macrophage death: the role of caspase-1 in death and inflammation.
Microbes Infect, 3(14-15):1201-1212, 01 Nov 2001
Cited by: 74 articles | PMID: 11755408
Review
Funding
Funders who supported this work.
NIAID NIH HHS (3)
Grant ID: AI-42780
Grant ID: R01 AI026195
Grant ID: R01 AI-26195