Abstract
Free full text

Efficient Presentation of Phagocytosed Cellular Fragments on the Major Histocompatibility Complex Class II Products of Dendritic Cells
Abstract
Cells from the bone marrow can present peptides that are derived from tumors, transplants, and self-tissues. Here we describe how dendritic cells (DCs) process phagocytosed cell fragments onto major histocompatibility complex (MHC) class II products with unusual efficacy. This was monitored with the Y-Ae monoclonal antibody that is specific for complexes of I-Ab MHC class II presenting a peptide derived from I-Eα. When immature DCs from I-Ab mice were cultured for 5–20 h with activated I-E+ B blasts, either necrotic or apoptotic, the DCs produced the epitope recognized by the Y-Ae monoclonal antibody and stimulated T cells reactive with the same MHC–peptide complex. Antigen transfer was also observed with human cells, where human histocompatibility leukocyte antigen (HLA)-DRα includes the same peptide sequence as mouse I-Eα. Antigen transfer was preceded by uptake of B cell fragments into MHC class II–rich compartments. Quantitation of the amount of I-E protein in the B cell fragments revealed that phagocytosed I-E was 1–10 thousand times more efficient in generating MHC–peptide complexes than preprocessed I-E peptide. When we injected different I-E– bearing cells into C57BL/6 mice to look for a similar phenomenon in vivo, we found that short-lived migrating DCs could be processed by most of the recipient DCs in the lymph node. The consequence of antigen transfer from migratory DCs to lymph node DCs is not yet known, but we suggest that in the steady state, i.e., in the absence of stimuli for DC maturation, this transfer leads to peripheral tolerance of the T cell repertoire to self.
During the maintenance of tolerance (1–3) and the rejection of malignant cells (4) and transplants (5–12), there is a transfer of antigens from tissues to the MHC class I and II products of APCs. Mechanisms underlying this “cross-presentation” phenomenon need to be elucidated, and a priori may simply involve the release of peptides from donor cells (e.g., tumor, transplant, self-tissue) to recipient APCs. Recent tissue culture studies have used T cell readouts to demonstrate that dendritic cells (DCs)1 can present antigen to influenza-specific CTLs after transfer of a dominant matrix peptide from apoptotic cells (13, 14). Here we directly monitor the formation of specific MHC class II–peptide complexes in vitro and in vivo after the phagocytosis of cell fragments. We find that DCs, known for strong costimulatory functions, are also unusually efficient at forming MHC–peptide complexes from phagocytosed cells, some 1–10 thousand times more efficiently than an exposure to preprocessed peptide. A comparable phenomenon is observed in vivo when short-lived migratory DCs migrate via afferent lymphatics to the T cell areas of the lymph node. Antigen is transferred from the injected DCs to the recipient DCs in the lymph node, a phenomenon that may underlie cross-priming after transplantation, and cross-tolerance to self-tissues in the steady state.
Materials and Methods
Mice.
Adult 6–8-wk-old mice (BALB/c, C57BL/6, C57BL/6 × DBA/2 F1) of both sexes were from Taconic Farms Inc. (Germantown, NY) and Japan SLC (Hamamatsu, Japan), and B10.A(4R) from The Jackson Laboratory (Bar Harbor, ME). B6.I-E transgenic mice, line 107 (15), were provided by Dr. R. Flavell (Yale University, New Haven, CT).
Cells.
DCs were generated from bone marrow progenitors by culture in rGM-CSF as described (16). The cultures were set up in 24-well plates (Costar Corp., Cambridge, MA) and used at day 6 when the wells were covered with aggregates of immature DCs. By days 7–8, aggregates release ~105 mature DCs/well (16). Immature but not mature DCs are phagocytic (17). B blasts were induced for 3–4 d with LPS (Escherichia coli 0111:B4, 25 μg/ ml; Sigma Chemical Co, St. Louis, MO), or for 2 d with anti-μ (5 μg/ml F(ab′)2 goat anti–mouse IgM; Jackson ImmunoResearch Labs, West Grove, PA) and IL-4 (5 ng/ml; GIBCO BRL, Gaithersburg, MD). Both types of B blasts gave similar results in tests for antigen transfer. Small B cells were spleen cells passed over Sephadex G10 columns and depleted of T cells with antibodies (Thy-1, TIB 99; CD4, L3T4; and CD8, TIB 211; all from American Type Culture Collection, Rockville, MD) and complement. Human cells were plastic adherent monocytes from PBMCs and EBV-transformed B cell lines. Necrosis was induced either by freeze–thawing three to four times, or in the case of mouse B cells, with monoclonal J11d and rabbit complement in MEM. Apoptosis was induced by exposure to UV light. The surface of most cells became annexin V–positive after 8–10 h.
Antibodies.
The Y-Ae hybridoma was provided by Dr. C. Janeway, Jr. (Yale University, New Haven, CT) and grown serum-free. The IgG2b antibody was purified on protein A–Sepharose, biotinylated, and used in the FACS® along with PE-streptavidin to detect MHC class II–peptide complexes (18). A mouse IgG2b anti–human TCR antibody was the nonreactive control (PharMingen, San Diego, CA). The cells were double labeled for FACS® and for microscopy with FITC antibodies to CD8, CD11c, CD86, I-Ab (AF6-120.1), I-Ad (39-10-8), and I-E (14-4-4S; all from PharMingen).
Capture of Cell-derived Peptides In Vitro.
Bone marrow DCs were generated in 24-well plates for 6 d in GM-CSF, gently washed to remove mature floating DCs, and then fed B blasts, human cells, or peptide (residues 56–73 of I-Eα). Alternatively, the 6-d DCs were added to the B blasts. During uptake, the DCs matured with high levels of surface CD86.
Confocal Microscopy.
Cells were resuspended in serum-free RPMI 1640, attached to Alcian Blue–coated glass coverslips for 20–30 min at 37°C, fixed in 4% paraformaldehyde for 20 min at room temperature, washed three times in PBS, and permeabilized for 15 min in PS (0.05% saponin, 10 mM Hepes, pH 7.4, 10 mM glycine, pH 8, 10% goat serum, RPMI 1640). All incubations and washes were then in PS. Cells were incubated in primary antibody (see figure legends) for 30 min at room temperature, washed four times in PBS, followed by secondary FITC- or Texas Red–conjugated antibody for 30 min at room temperature, washed four times in PS, once in PBS, once in water, and mounted in Mowiol (Calbiochem Corp., La Jolla, CA) on glass slides for analysis by immunofluorescence and confocal microscopy (0.5–1.0-μ optical sections). The observed double labeling of phagocytic vacuoles for donor B cell antigens and recipient DC MHC II was verified by an examination of perpendicular sections through the phagosomes.
Antigen-presentation Assays.
The 20.6 T–T hybridoma recognizes the MHC II–peptide complex that is detected with Y-Ae mAb (19). Graded doses of APCs, which had been fixed in 1.0% HCHO for 10 min at room temperature, were added to 2 × 104 hybridoma cells in flat-bottomed microtest plates. At 24 h, IL-2 in the supernatants was detected using a growth assay for Con A–stimulated T blasts.
Quantitation of I-E Molecules in B Cells.
Mature I-E heterodimers were quantified in a binding assay with 125I-labeled 14-4-4S mAb (PharMingen). The B blasts were fixed in 4% PFA and in some cases, 0.05% saponin, to include intracellular pools. To verify that the bulk of the I-E in 4-d B blasts was recognized by 14-4-4S, whole cell lysates were lysed in 50 mM Tris, pH 7.4, 100 mM NaCl, 0.5% Triton X-100, and protease inhibitors. Lysates were precleared with preimmune rabbit sera and zysorbin (Zymed Laboratories, Inc., So. San Francisco, CA). Protein A–Sepharose CL-4B (Amersham Pharmacia Biotech, Inc., Piscataway, NY) was incubated with rabbit anti–mouse IgG2a bridging antibody and then with 14-4-4S (or control Ig). Total and immunoadsorbed lysates were run on 10% SDS-PAGE, transferred to nitrocellulose, and blotted for total I-Eα using a rabbit antiserum specific for the cytoplasmic tail of I-Eα (provided by Dr. R. Germain, National Institutes of Health, Bethesda, MD).
Capture of Cell-derived Peptides In Vivo.
2 × 106 I-E+ DCs from BALB/c or B6.I-E transgenic mice were injected in PBS into the footpads of C57BL/6 (H-2b) mice. At 2 d, the popliteal lymph nodes were frozen in OCT embedding medium, sectioned, fixed in absolute acetone for 10 min, and stained successively with biotin–Y-Ae (or anti–human TCR IgG2b as control), avidin–alkaline phosphatase conjugates, rat antibody to B220 to label B cell follicles, and peroxidase-labeled mouse anti–rat Ig. We thereby located blue Y-Ae+ cells relative to B cells in brown. Negative controls were mice that lacked I-E on the donor B blasts (B10.A[4R] or C57BL/6) or I-Ab in the recipients (CBA/J, H-2k). To identify Y-Ae+ cells in the FACS®, DCs were enriched from the nodes (20) and double labeled with biotin Y-Ae (or nonreactive IgG2b mAb as control), PE-streptavidin, and different FITC conjugates to CD8, B220, CD11c, I-Ab, and I-Ad.
Results
Transfer of Antigen from B Blasts to the MHC Class II Products of DCs.
To demonstrate that DCs could present peptides from other cells, we cultured C57BL/6 DCs (I-Eα−, I-Ab+) with activated BALB/c B blasts (I-Eα+, I-Ab−) and monitored reactivity with Y-Ae mAb. Y-Ae is specific for an MHC class II–peptide complex formed when I-Ab presents the I-Eα 56–73 peptide (18). The DCs were from 6-d GM-CSF–stimulated marrow cultures, known to have phagocytic activity, whereas mature nonphagocytic DCs predominate at days 7–8 (17). When H-2b DCs were exposed for 20 h to 1 μM I-Eα peptide, the specific MHC– peptide detected by Y-Ae developed (Fig. (Fig.11 A). Y-Ae signal also developed when I-E+ B blasts, but not I-E− B blasts, were added at a ratio of 2–10 blasts per DC (Fig. (Fig.11 A). The Y-Ae+ cells had mature DC markers (CD86high, CD11c+, I-Ab+, I-Ad−; Fig. Fig.11 B). No Y-Ae was detected when B blasts were added to H-2d DCs, and 4-d blasts gave much stronger Y-Ae signals than unstimulated B cells (not shown).
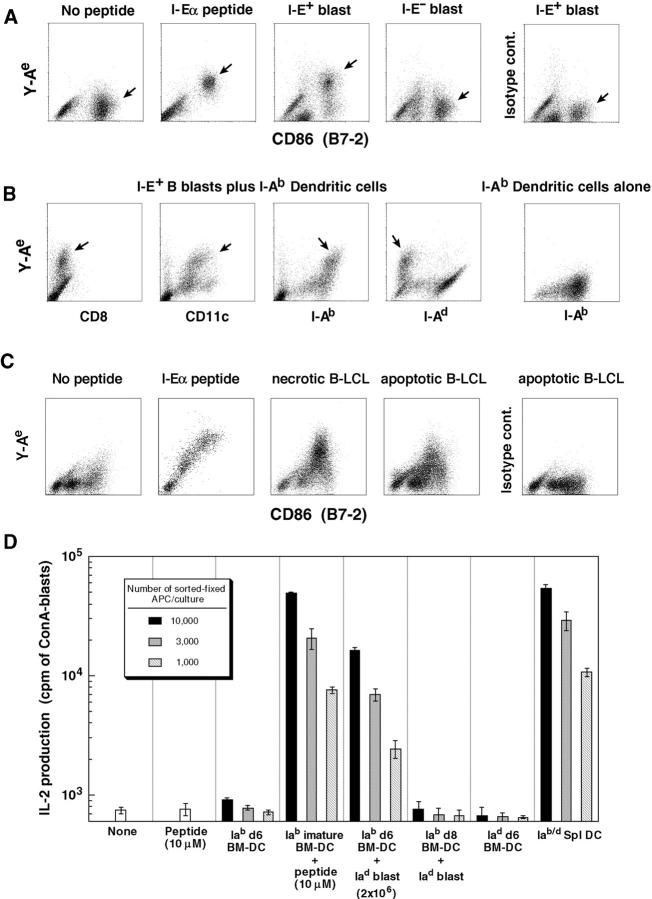
Development of MHC–peptide complexes consisting of a B cell– derived I-Eα peptide and DC-derived, I-Ab MHC II products. (A) C57BL/6 I-Ab DCs were cultured for 20 h with no peptide, 10 μM peptide, or 2 × 106 B blasts from H-2d BALB/c (I-E+) or H-2b C57BL/6 (I-E−) mice. CD86-rich DCs (arrows) acquired I-E peptide (Y-Ae stain) from I-E+ B blasts. No labeling was seen with an IgG2b isotype control to Y-Ae (right). (B) As in A, but DC–BALB/c B blast cocultures (or DCs alone, right) were labeled with other mAbs to show that CD11c+, I-Ab+, I-Ad− DCs selectively acquired Y-Ae (arrows). (C) EBV-transformed human B cell lines (3 cells/DC; necrotic and apoptotic) served as a source of I-Eα peptide for mouse DCs. An isotype-matched IgG2b antibody was used as a control for the specific Y-Ae labeling that developed on the CD86-rich DCs. (D) As in A, but the DCs were sorted as CD11c/CD86+ cells from the 20-h cultures, fixed in 1% formaldehyde for 10 min to block further processing, and added in graded doses to a T–T hybridoma that is specific for the same epitope as the Y-Ae mAb. At 24 h, medium was tested in an IL-2 bioassay ([3H]TdR uptake).
Xenograft immunity also involves presentation of xenogeneic peptides by recipient MHC II products (11). Human HLA-DRα has the identical sequence to mouse I-Eα 56–73 (21, 22). A Y-Ae signal developed when mouse DCs were cultured with either apoptotic or necrotic human B lymphoblastoid cell lines (Fig. (Fig.11 C) or monocytes (not shown).
The functionality of the Y-Ae epitope was tested using a hybridoma that responds to a similar I-Ab/I-Eα peptide complex (19). After a 20-h exposure to either I-Eα peptide or B blasts, the DCs were almost as potent APCs as DCs expressing endogenous I-Ab complexes (I-Ab × I-E+ F1 mice), as indicated by IL-2 production (Fig. (Fig.11 D).
Antigen Processing Is Required for Transfer of Peptide from B Blasts to DCs.
To approach mechanisms, we first ruled out release of peptide from B blasts to DCs. Y-Ae labeling did not develop if the cells were separated by Transwell filters, or if B blasts were added to mature DCs that express high surface MHC II but are nonphagocytic (Fig. (Fig.22 A). Both apoptotic (induced by exposing the B blasts to UV light 10 h before addition to the DCs; not shown) and necrotic B blasts (induced by freeze–thawing in Fig. Fig.11 C, and by antibody and complement–mediated lysis in Fig. Fig.22 A) were processed by DCs. A fraction (20–40%) of mouse B cells and human EBV lines die during overnight culture, and we could not demonstrate an increase in antigen transfer if these cells were induced to apoptose or necrose before coculture with immature DCs (compare B blast with Ab/C in Fig. Fig.22 A).
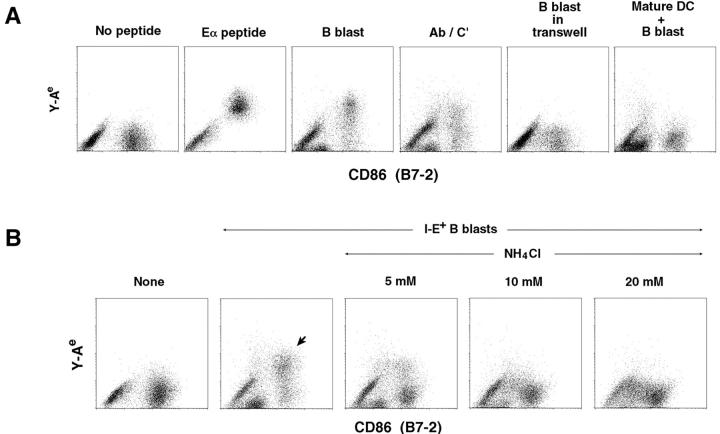
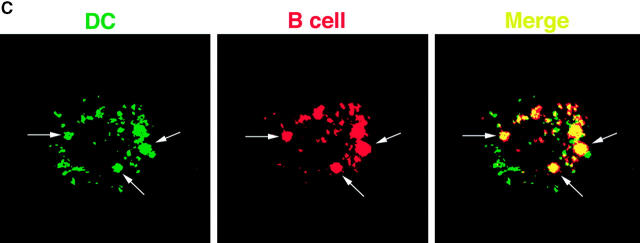
Antigen transfer requires cellular processing. (A) B blasts were separated from immature DCs (6-d bone marrow cultures) by a Transwell filter (0.45-μ pores), or added to mature DCs (nonadherent cells from day 8 marrow cultures; see Materials and Methods). Although Y-Ae signals developed on immature day 6 marrow DCs upon exposure to B blasts, including B blasts killed with antibody and complement (Ab/C′), no Y-Ae developed if the DCs were separated by a filter or if mature nonphagocytic DCs were used. (B) Graded doses of NH4Cl were added to cocultures of I-E+ B blasts and I-Ab DCs, and Y-Ae epitope formation was measured 20 h later. The block was fully reversible within 12 h (see Fig. Fig.3).3). (C) I-Ad/I-E+ B blasts were cultured for 6 h with I-Ab+/I-E− bone marrow– derived DCs. B cell fragments (red) were visualized by confocal microscopy using mAbs to B cell markers I-Ad (mAb 39-10-8), I-E (mAb 14-4-4S), and B220 (mAb RA3-3A1/6.1; middle, B220 stain is shown). Immature DCs (left) were identified using antibody specific for I-Ab (biotin AF6-120.2). Merging of the two images (right) showed that the B cell fragments had been internalized into MHC II+ structures (arrows) in immature DCs. Most DCs only had one to two B cell fragments, but this micrograph was selected to emphasize the location of most B cell fragments in MIICs.
Since the findings suggested that peptides had to be processed by immature phagocytic DCs (17), we tested if NH4Cl, which inhibits acidification and proteolysis within endocytic vacuoles, could block the formation of the Y-Ae epitope. This was indeed the case, with a complete block being observed at 10 mM (Fig. (Fig.22 B). The NH4Cl block was reversible.
We then used confocal microscopy to look directly for the internalization of B blasts by DCs. We rarely observed profiles of intact B cells in DCs, but after 6 h of coculture, most DCs contained B cell fragments (I-Ad, I-Ed, and B220+) in vacuoles that had markers of MHC II–rich compartments or MHC II compartments (MIICs) (MHC II, LAMP+, and H-2M+; Fig. Fig.22 C, arrows).
Phagocytosis of B Blasts Precedes Formation of MHC II–Peptide Complexes by DCs.
To demonstrate that phagocytosis preceded the formation of the Y-Ae epitope, B blasts were fed to DCs in 20 mM NH4Cl. After 20 h, DCs were FACS® separated from residual B blasts and recultured for 12 h without NH4Cl. NH4Cl completely blocked Y-Ae epitope development (Fig. (Fig.33 A, top), even within saponin-permeabilized cells (not shown), but the Y-Ae reappeared after removal of NH4Cl (Fig. (Fig.33 A, bottom). The block imposed by NH4Cl seemed primarily at the level of I-E processing, rather than peptide loading, since addition of I-Eα peptide for 20 h to NH4Cl cultures led to strong Y-Ae signals comparable to nonblocked cultures (Fig. (Fig.33 B).
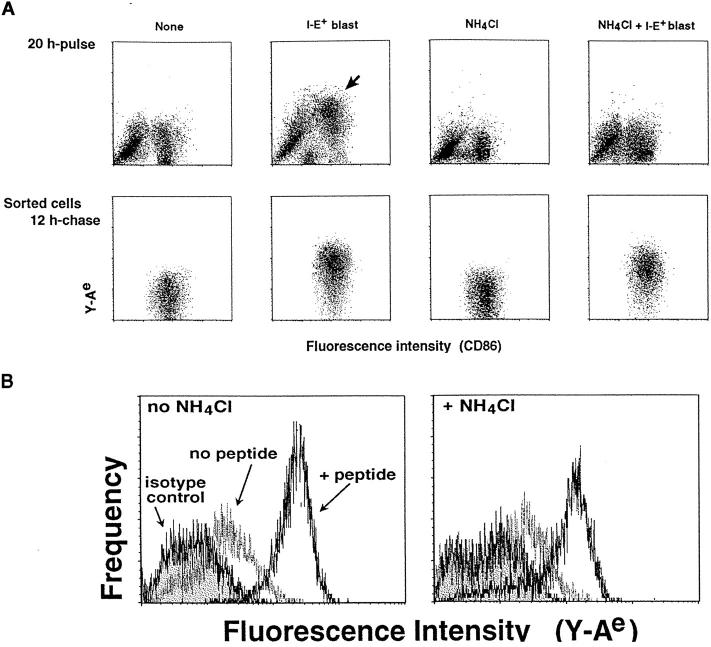
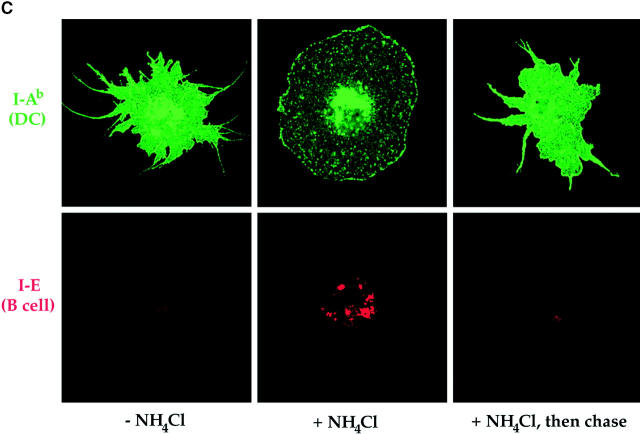
Antigen transfer is preceded by phagocytosis. (A) Top, the formation of Y-Ae after 20 h of DC–B blast coculture with or without 20 mM NH4Cl. The DCs then were sorted as double positive for PE-CD11c and FITC-CD86, or in a second experiment, just FITC-CD86+, and cultured for 12 h without NH4Cl (bottom). Y-Ae (biotin Y-Ae followed by Cychrome-Avidin) quickly regenerated in DCs that had been blocked by NH4Cl. No Y-Ae was found in saponin-permeabilized samples of NH4Cl-treated cultures. (B) Immature DCs were cultured with or without 20 mM NH4Cl and 10 μM preprocessed I-E peptide for 15 h, and then Y-Ae levels were measured on the FACS®. CD86 (not shown) and Y-Ae epitope formed in the presence of NH4Cl. (C) DCs prepared exactly as in A were examined by confocal microscopy. DC MHC II (I-Ab, green) was visualized relative to B cell MHC II (I-E, red). When immature DCs were allowed to internalize B blasts for 20 h in the absence of NH4Cl, B cell fragments were largely degraded (although fragments were detected after just 6 h; see Fig. Fig.22 C), and the DCs acquired a mature phenotype with characteristic dendrites and high MHC II on the plasma membrane (left). However, if B blasts were internalized in the presence of 20 mM NH4Cl, abundant I-E+ fragments were found within DCs in MHC II+ phagolysosomes, and the MHC II was arrested intracellularly (middle). Upon removal of the NH4Cl and culture for an additional 12 h, the DCs matured and the internalized B cell fragments largely disappeared, presumably due to degradation (right).
When DCs were sorted from 20-h B blast–DC cocultures and examined by confocal microscopy, DCs no longer had B cell fragments (vs. 6 h in Fig. Fig.22 C), unless NH4Cl-treated (Fig. (Fig.33 C, middle). When NH4Cl-treated B blast–containing DCs were chased for 12 h without NH4Cl, the phagocytosed B cells were degraded and high levels of surface MHC II accumulated (Fig. (Fig.33 C, right).
The Unusual Efficiency of Processing of Phagocytosed B Cells.
In kinetic studies comparing Y-Ae expression derived from preprocessed I-Eα peptide with B blasts, we found that MHC–peptide complexes appeared after 5 h of culture, reached a plateau at 15–25 h, and remained stable for 3 d; 1 μM peptide gave comparable Y-Ae signals to 106 B blasts (Fig. (Fig.44 A).
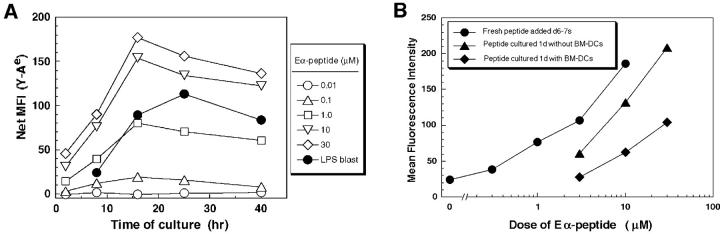
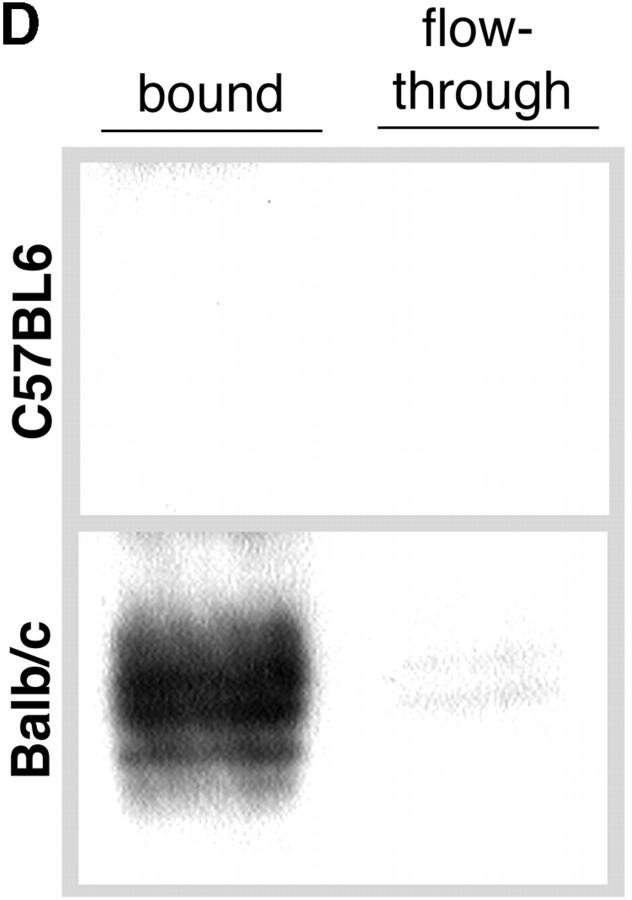
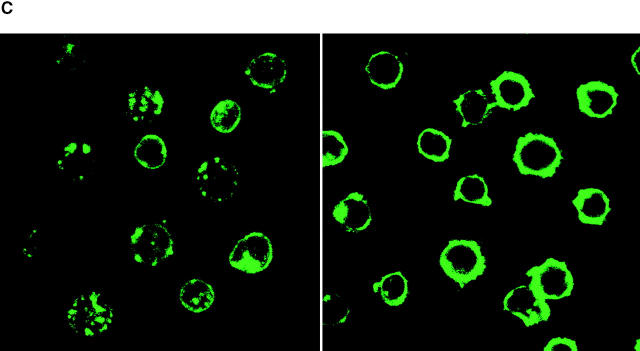
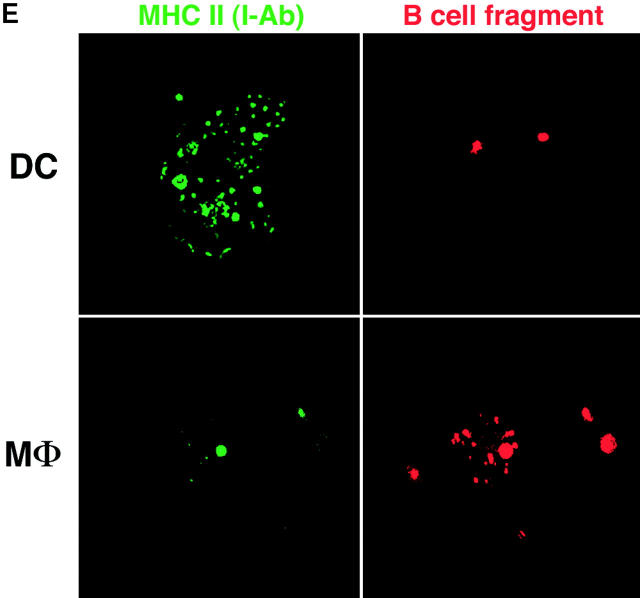
Efficiency of antigen transfer after phagocytosis. (A) As in Fig. Fig.11 A, C57BL/6 DCs were cultured for the indicated times with doses of I-Eα peptide, or 106 B blasts from BALB/c mice, and double labeled for Y-Ae and CD86. Y-Ae signals (Mean Fluorescence Index) are shown for CD86+ mature DCs. (B) Assays for the stability of the I-E peptide during culture. Graded doses of I-E peptide were added to day 6 marrow DCs, and the levels of Y-Ae (Mean Fluorescence Intensity) were measured 20 h later (circles). At this time, supernatants were harvested from the cultures (diamonds) in parallel with peptide that was cultured in the absence of cells for 20 h (triangles). Both sources of peptide were then added to fresh cultures of immature marrow DCs, and Y-Ae epitope formation was compared 20 h later. (C) Distribution of MHC II in fresh B cells (left) and 4-d splenic B blasts (right). The latter are processed best by DCs and express ~1.5 × 105 I-E molecules/cell, mostly mature heterodimers (see text). MHC II was visualized with a rabbit polyclonal antibody to I-Eα. The majority is on the cell surface of blasts, but in intracellular vacuoles in fresh B cells. (D) Most of the I-E in B blasts consists of mature I-E heterodimers. 4-d LPS-induced B blasts from I-E+ BALB/c and I-E− C57BL/6 mice were lysed and reacted with mAb 14-4-4S to mature I-E molecules. The immune complexes were bound to beads and then a rabbit anti–I-E antibody, which recognizes all forms of I-E, was used to blot bound and nonbound fractions. Most I-E molecules from B blasts were bound by 14-4-4S. (E) Macrophages versus immature DCs are the main site for phagocytosis of B cell fragments in 6-d GM-CSF–stimulated mouse marrow cultures. Immature MHC II–rich (green) DCs phagocytose B cell fragments (B220 stain, red), but firmly adherent MHC II–low macrophages contained more numerous intracellular B cell fragments.
We then measured the level of I-E in the B blasts. Fixed and fixed–permeabilized blasts had 1.5–2.0 × 105 mature I-E molecules/cell, using 125I-labeled 14-4-4S antibody (23), corresponding to 0.3 nM I-E protein in a 1-ml culture. 14-4-4S recognizes mature MHC II heterodimers (24) and not invariant chain–associated or unassembled forms of I-E, but we believe that 14-4-4S binding reflects the majority of I-E molecules in the B blasts for the following reasons. First, using a rabbit antiserum that detects all forms of I-E, we found that I-E was localized almost exclusively to the surface of 4-d B blasts, whereas in fresh B cells, most I-E was intracellular in MIICs (Fig. (Fig.44 B). In addition, the I-E in 4-d B blasts was primarily in the mature 14-4-4S detectable form. The plasma membrane was devoid of detectable invariant chain staining, and whole cell lysates adsorbed with 14-4-4S lacked reactivity with rabbit anti–I-E (Fig. (Fig.44 C).
We verified that free I-E peptide was not being quickly degraded upon addition to DCs. If the medium was removed from DCs that had been pulsed with I-Eα peptide and added to fresh DCs, the Y-Ae signal was 10–30% that seen with fresh peptide (Fig. (Fig.44 D). Therefore, even if every I-E chain were successfully processed to form an MHC– peptide complex, B blasts (0.3 nM I-E concentration) would still be 3,000 times more efficient than free I-Eα peptide (1 μM) for charging the I-Ab molecules of DCs to form the Y-Ae epitope. This is an underestimate, since phagocytosis of B cell fragments by contaminating MHC II− macrophages greatly reduced the pool of I-E available to DCs. In fact, macrophages had 10–20 B cell fragments on average versus 1–7 fragments per DC (Fig. (Fig.44 E).
Efficient Uptake of Donor DCs by Recipient DCs in the T Cell Areas of Lymph Node.
We next asked if DCs could process antigens from other cells in vivo. We tried injecting into the footpads of C57BL/6 mice many different cell types that expressed the I-E peptide and that had been cultured 10 h after being induced to apoptose with UV light treatment. Only infrequent cells in the T cell area expressed Y-Ae 2 d later. However, when we injected living, marrow-derived BALB/c DCs into C57BL/6 mice, large Y-Ae+ cells were found throughout the T cell area, but not in the B cell follicles or in the macrophage-rich subcapsular sinus and medullary regions (Fig. (Fig.55 A). These profiles were shown to be recipient DCs by FACS® analysis (Fig. (Fig.55 B). The DCs were first enriched on dense Nycodenz or BSA columns (20), and then the preparations were double labeled, e.g., for the CD11c integrin that is abundant on DCs. All the Y-Ae was found on CD11c+ B220− I-Ab+ cells, i.e., recipient DCs. The transfer of I-E peptide from injected DCs to recipient DCs was efficient, since 2 × 106 DCs contain <10 × 1011 I-E molecules or 0.05 μg of I-E protein.
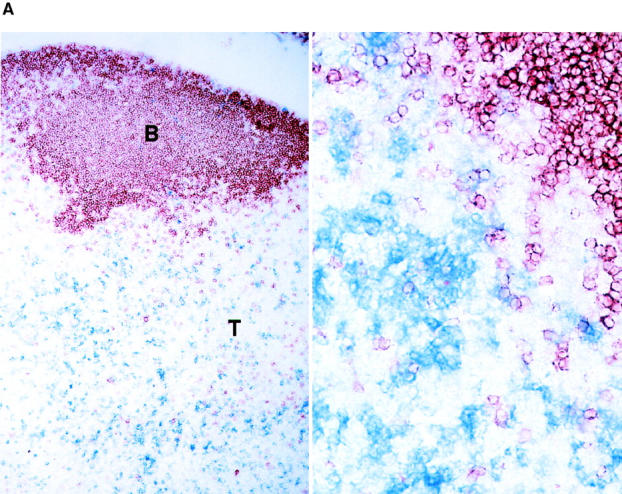
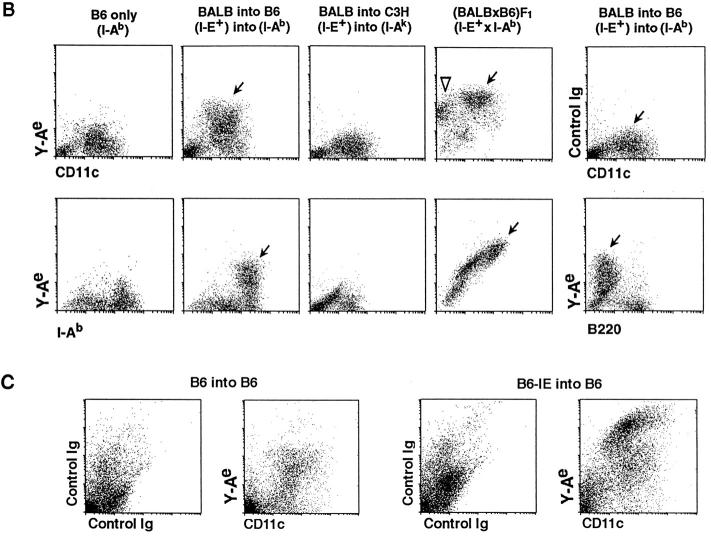
Antigen transfer from injected DCs to recipient DCs in vivo in lymph node. (A) 2 × 106 mature, marrow-derived I-E+ BALB/c DCs were injected into the footpads of C57BL/6 I-Ab mice. 2 d later, sections of popliteal nodes were stained for Y-Ae (blue) and B220 B cell marker (brown) and photographed at low and high power (original magnifications: ×50 and ×200). Blue profiles are scattered throughout the T cell areas. No blue Y-Ae staining was seen in several controls: a nonreactive isotype-matched antibody to Y-Ae, and donor or recipient mice that lacked either the I-E peptide or the I-Ab presenting molecule (see text). (B) As in A, but the DCs in the recipient nodes were enriched for FACS® analyses (reference 20). The cells were double labeled for Y-Ae or isotype-matched control mAb (y-axis) and different cellular markers (x-axis). The Y-Ae signals that developed were compared with the expression of “endogenous” Y-Ae in BALB/c × B6 F1 mice. Y-Ae+ DCs (arrows) and B cells (white arrowhead) are shown. In C57BL/6 mice, most recipient CD11c+, I-Ab+, B220− DCs processed I-E and expressed Y-Ae. (C) As in B, but the DCs were from B6.I-E transgenic (right) or B6 mice (left). Processing by host CD11c+ DCs to form the Y-Ae epitope occurs when the donor and recipient DCs differ only in terms of I-E expression.
As negative controls, we found that Y-Ae signals did not develop with H-2k C3H recipients that lacked I-Ab (Fig. (Fig.55 B) or with B10.A(4R) allogeneic donor DCs that lacked the I-E peptide (not shown). Since the donor DCs we used initially were from BALB/c mice and were incompatible with C57BL/6 at several major and minor histocompatibility loci, we injected DCs from C57BL/6 I-E transgenic mice. The B6.I-E transgenic cells were weak immunogens, since the cell numbers in the draining lymph nodes did not increase relative to controls injected with B6 DCs. Nonetheless, Y-Ae+ DCs appeared in large numbers in the draining lymph nodes by day 2 (Fig. (Fig.55 C). I-E+ donor DCs could only be detected in the nodes at day 1, and even then were outnumbered by recipient I-E− DCs by 20–50-fold (not shown). Therefore, I-E peptides can be transferred from donor to recipient DCs, even in the absence of a major immunogenic stimulus.
Discussion
A new approach has become available to study the formation of MHC–peptide complexes by APCs. mAbs have been isolated that specifically recognize complexes formed between an MHC class I (25) or class II (18, 26, 27) product and a peptide. These antibodies can be used to visualize antigen presentation, i.e., the expression of MHC–peptide complexes, at the single cell level rather than the classical indirect assay of T cell stimulation by bulk populations of APCs. We have benefited in this paper from the first such antibody, Y-Ae, which was isolated by Murphy et al. (28) and shown to recognize a complex of I-Ab presenting a peptide from another MHC class II product, I-Eα (18, 29). MHC molecules often present peptides derived from other MHC products (30), so this kind of complex is not uncommon. Moreover, the Y-Ae complex can be particularly abundant, especially on DCs within the lymphoid tissues of F1 mice formed from the cross of C57BL/6 (I-Ab+) and either DBA/2 or BALB/c (I-E+; reference 20) mice. The use of Y-Ae proved valuable for outlining a newly recognized function for DCs, the processing of peptides from other cells.
This processing of cellular fragments was uncovered in studies of HLA-A2.1+ DCs from human blood (13, 14). The DCs would present an influenza matrix peptide from HLA-A2.1− cells that were either infected or transfected with the influenza matrix gene. MHC class I–peptide complex formation was monitored with functional assays, i.e., presentation to CD8+ CTLs. The complexes only formed when apoptotic cells were given to DCs, whereas our current studies indicate that processing of cellular fragments onto MHC class II occurs with both apoptotic and necrotic cells.
It is not surprising that immature DCs present antigens from endocytosed particulates, as shown for bacteria (17, 31, 32). However, what is surprising is the efficiency with which peptides can be extracted from phagocytosed cellular fragments, in our case from the plasma membrane of B blasts (Fig. (Fig.4).4). The finding (Fig. (Fig.3)3) that antigen transfer requires acidic compartments implies that the MIICs of DCs are processing and presentation machines. A protein from phagocytosed cells can be presented 1–10 thousand times better than preprocessed peptide, even though the latter is reasonably stable in culture and is administered to the same DCs that are fed dying B cells. In ongoing experiments in which we have monitored MHC–peptide formation by marrow-derived DCs (and in reports where other APCs have been studied [26, 27]), 1–10 μM concentrations of soluble protein and preprocessed peptide must be added to observe MHC class II–peptide formation. In contrast, when I-E protein in B blasts is offered to immature DCs, <1 nM protein leads to strong signals for MHC–peptide formation, and preprocessed peptide in parallel is >1,000 times less efficient. This calculation assumes that every I-E protein molecule is successfully processed to form an MHC–peptide complex. Therefore, we suspect that the success with which DCs process particulate antigens into MHC–peptide complexes reflects two things: (a) increased antigen uptake during adsorptive phagocytosis plus (b) the capacity of DCs to extract peptides from internalized protein for presentation on MHC II, as opposed to complete destruction down to the level of amino acids.
Our results also visualize a possible mechanism whereby antigens in peripheral tissues can be presented in lymph nodes to account for cross-priming in the case of transplants and cross-tolerance in the case of self-tissues. Foreign antigens in allografts and xenografts (5–12) are known to prime the recipient's immune system in association with recipient MHC II products, whereas self-antigens in pancreatic islets are known to tolerize the immune system in association with MHC I (1, 2) and II (3) products on lymph node APCs. The very act of transplantation causes DCs to differentiate, to express for example very high levels of MHC II and the CD86 costimulator, and to leave the transplant (33, 34). In contrast in the steady state, DCs would not be expected to be stimulated during their traffic through tissues to afferent lymph and then to the T cell areas of lymph nodes. Migratory DCs almost certainly die in the T cell areas, since DCs are not found in thoracic duct lymph (35–37). We find that migratory DCs can be processed, distributing their peptides widely and efficiently to other DCs to form MHC–peptide complexes. The latter, detected by the Y-Ae antibody, are unlikely to form in the periphery, since the turnover of DCs in lymphoid tissues requires 1–2 wk (38), yet most recipient DCs in lymph nodes formed Y-Ae in just 1–2 d.
The distinction between cross-priming and cross-tolerance may emanate from the maturation state of the incoming DC. If the DC has been activated in the peripheral tissues to express high levels of accessory molecules like CD86, then some of the incoming DCs should initiate the immune response. In this case, CD40L and TNF-related activation–induced cytokine (TRANCE) on the activated T cell would maintain DC viability (39). In contrast, when self-antigens from noninflamed tissues are brought to the T cell areas in the steady state, e.g, if immature DCs would phagocytose apoptotic cells during the normal turnover of tissues, T cell stimulation would not occur and the DCs would die quickly. Then presentation may be initiated by longer-lived recipient DCs in the lymph node that phagocytose the migratory DCs and express proposed regulatory and tolerogenic functions (40, 41).
We propose that in the steady state, antigens from apoptotic cells are picked up by peripheral DCs and are processed by lymph node DCs to purge the T cell repertoire of self-reactivity. DCs tolerize developing thymocytes (42– 44). Yet many self-antigens may not have access to the thymus, especially during development of the T cell repertoire in fetal and neonatal life (45). It may be essential for DCs to be able to tolerize the repertoire peripherally, since it seems obligatory that some self-antigens would be presented on DC MHC products whenever foreign antigens were being captured and presented. For example, when the I-E peptide we studied here is a self-antigen, the levels of MHC II–self peptide complexes are very high on DCs (20). DCs in the periphery are poised to capture antigens and to mature into strong stimulators of T cell immunity, events that are induced by inflammatory cytokines and microbial products (for reviews, see references 46 and 47). Because of the findings in this paper, i.e., the capacity of DCs to efficiently present peptides from phagocytosed cells and to do so in vivo during processing of migratory DCs, we suggest the following pathway to explain the capacity of the immune system to distinguish foreign from self-antigens. When DCs are presenting foreign, inflammatory, and dangerous antigens (45, 48), the DCs are mature, longer-lived, and stimulate T cells directly, but reactivity to self is avoided because self-reactive T cells have previously been inactivated. This inactivation of self-reactive T cells occurs via DCs in the thymus, but also in the periphery by the efficient transfer of self from peripheral to lymph node DCs.
Acknowledgments
The authors thank Dr. C. Janeway, Jr. for 107 I-E transgenic mice, and both Drs. C. Janeway, Jr. and R. Germain for critical antibodies.
This work is supported by grants from the National Institutes of Health (AI-13013 and AI-39672 to R.M. Steinman, and AI-34098 and GM-33904 to I. Mellman), the Yamanouchi Fund, and the Ministry of Education, Science and Culture of Japan (08282104, 10153226, and 10044268 to K. Inaba).
References
Articles from The Journal of Experimental Medicine are provided here courtesy of The Rockefeller University Press
Full text links
Read article at publisher's site: https://doi.org/10.1084/jem.188.11.2163
Read article for free, from open access legal sources, via Unpaywall:
http://jem.rupress.org/content/jem/188/11/2163.full.pdf
Citations & impact
Impact metrics
Article citations
In situ endoscopic photodynamic therapy combined with immature DC vaccination induces a robust T cell response against peritoneal carcinomatosis.
J Immunother Cancer, 12(11):e009752, 05 Nov 2024
Cited by: 0 articles | PMID: 39500528 | PMCID: PMC11552574
Engineering strategies for apoptotic bodies.
Smart Med, 3(3):e20240005, 14 Jul 2024
Cited by: 0 articles | PMID: 39420952 | PMCID: PMC11425054
Review Free full text in Europe PMC
Exploring dendritic cell subtypes in cancer immunotherapy: unraveling the role of mature regulatory dendritic cells.
Ups J Med Sci, 129, 12 Apr 2024
Cited by: 0 articles | PMID: 38716077 | PMCID: PMC11075441
Review Free full text in Europe PMC
Progress of PD-1/PD-L1 signaling in immune response to liver transplantation for hepatocellular carcinoma.
Front Immunol, 14:1227756, 20 Jul 2023
Cited by: 2 articles | PMID: 37545535 | PMCID: PMC10399574
Review Free full text in Europe PMC
CD5 expression by dendritic cells directs T cell immunity and sustains immunotherapy responses.
Science, 379(6633):eabg2752, 17 Feb 2023
Cited by: 22 articles | PMID: 36795805 | PMCID: PMC10424698
Go to all (377) article citations
Similar Articles
To arrive at the top five similar articles we use a word-weighted algorithm to compare words from the Title and Abstract of each citation.
The formation of immunogenic major histocompatibility complex class II-peptide ligands in lysosomal compartments of dendritic cells is regulated by inflammatory stimuli.
J Exp Med, 191(6):927-936, 01 Mar 2000
Cited by: 234 articles | PMID: 10727455 | PMCID: PMC2193115
High levels of a major histocompatibility complex II-self peptide complex on dendritic cells from the T cell areas of lymph nodes.
J Exp Med, 186(5):665-672, 01 Aug 1997
Cited by: 165 articles | PMID: 9271582 | PMCID: PMC2199017
Efficient targeting of protein antigen to the dendritic cell receptor DEC-205 in the steady state leads to antigen presentation on major histocompatibility complex class I products and peripheral CD8+ T cell tolerance.
J Exp Med, 196(12):1627-1638, 01 Dec 2002
Cited by: 845 articles | PMID: 12486105 | PMCID: PMC2196060
Control of MHC class II antigen presentation in dendritic cells: a balance between creative and destructive forces.
Immunol Rev, 207:191-205, 01 Oct 2005
Cited by: 111 articles | PMID: 16181337
Review
Funding
Funders who supported this work.
NIAID NIH HHS (5)
Grant ID: AI-34098
Grant ID: AI-39672
Grant ID: R37 AI034098
Grant ID: R01 AI013013
Grant ID: AI-13013