Abstract
Free full text

The Prolonged Life-Span of Alveolar Macrophages
Abstract
To further examine the half-life of alveolar macrophages, chimeric CD 45.2 mice were generated through bone marrow transplantation of donor CD 45.1 cells. Before administration of donor cells, recipient mice were divided into two cohorts: the first cohort received total body irradiation; the second cohort also received irradiation—however, the thorax, head, and upper extremities were shielded with lead. Flow cytometric analysis was then performed on blood, peritoneal, and bronchoalveolar lavage cells over time to quantify engraftment. The data generated for the unshielded cohort of mice revealed a macrophage half-life of 30 days. In the shielded cohort, however, we found that by 8 months there was negligible replacement of recipient alveolar macrophages by donor cells, despite reconstitution of the blood and peritoneum by donor bone marrow. Consistent with these findings, the mean fluorescent intensity of alveolar macrophages remained stable over a 4-week period after in vivo PKH26 dye loading. Together, these data show that previous alveolar macrophage half-life studies were confounded by the fact that they did not account for the toxic effects of irradiation conditioning regimens, and demonstrate that the bone marrow does not significantly contribute to the alveolar macrophage compartment during steady-state conditions.
Alveolar macrophages (AMs), which were first established to originate from the bone marrow in 1968, serve as the first line of defense against inhaled pathogens, and function as a reservoir for chronic pathogens such as HIV and Tb (1–5). Since these cells are central to the lung's innate immune system, historically there has been considerable interest in understanding basic elements of AM biology, including intrinsic half-life.
To date, AM half-life estimations have been based largely on measurements of alveolar macrophage turnover in chimeric mice generated through irradiation conditioning regimens and bone marrow transplantation. Using such techniques, a large body of work has established a half-life of between 10 and 30 days for the murine AM (6–11). The first such study was published by Godleski and Brain, wherein they concluded a half-life of 2 weeks for AMs (6). Subsequently, a bone marrow origin and a 2-week half-life was confirmed by van Furth and van oud Alblas (10).
By employing newer techniques and reagents, including flow cytometry and the use of green fluorescent protein–expressing donor cells, a half-life of 30 days was more recently reported (11). These studies are, however, all confounded by the uncertain effect of total body irradiation conditioning on AM turnover itself. While initially thought to be relatively radiation resistant, other studies indicate that the AM is sensitive to the level of radiation that accompanies transplant conditioning regimens (12, 13). Rather than identifying their intrinsic half-life, studies in transplant chimeras may, thus, be primarily measuring the rate of AM repopulation by the bone marrow in the setting of a toxic exposure. To more precisely determine AM half-life, we generated bone marrow chimeras in irradiated mice in which their thorax was, and was not, shielded with lead during transplantation. Using this method, we found that the half-life of AM is in fact much longer than previously reported.
MATERIALS AND METHODS
Animals
The animal protocols were approved by the Animal Care and Use Committee of the Boston University School of Medicine and the National Institutes of Health. C57BL/6 mice expressing the alloantigen CD45.2 or CD45.1 were purchased from Jackson Labs (Bar Harbor, ME). Animals were housed under standard conditions in the Laboratory Animal Science Center.
Irradiation and Bone Marrow Transplantation
C57BL/6 CD45.2 (8–10 wk old) mice were divided into two cohorts and subjected to a single dose of 9.5 Gy of ionizing radiation from a cesium radiator. All mice were anesthetized with a single intraperitoneal injection of ketamine/xylazine (100 mg/kg and 10 mg/kg, respectively) before irradiation. The first cohort received total body irradiation, whereas the second cohort had only their abdomen, pelvis, and hind limbs irradiated, while their head, thorax, and forelimbs were shielded by a 2-inch lead screen. Donor bone marrow (BM) was obtained from a C57BL/6 CD45.1 mouse by dissecting out femurs, tibias, fibulas, and ischiums, which were then ground with a mortar and pestle and serially washed with Hank's Balanced Salt Solution (HBSS; Invitrogen, Carlsbad, CA) supplemented with 2% fetal bovine serum (FBS) (HBSS+). After discarding bony fragments, BM was re-suspended in HBSS+ at a concentration of 17 × 106 cells/ml. Irradiated mice were then anesthetized with isoflurane and given 3.4 million BM cells via retro-orbital injection. All mice were given acidulated water and soft gel food for 14 days after irradiation and BM transplantation.
Isolation of Circulating Leukocytes, Peritoneal Macrophages, and Alveolar Macrophages
To obtain peripheral leukocytes, 100 μl of blood was obtained via retro-orbital puncture with a heparinized capillary tube. Erythrocytes were removed from the sample by incubating with red blood cell lysis buffer (Sigma-Aldrich, St. Louis, MO), before washing with PBS supplemented with 2% FBS (PBS+) and pelleting at 800 × g for 5 minutes at 4°C; the supernatant was discarded and cells were re-suspended in PBS+ before antibody staining. To obtain peripheral blood mononuclear cells (PBMCs), blood was placed in 4 ml of DMEM with 2% FBS, layered onto 3 ml of Ficoll, and centrifuged at 800 × g for 30 minutes at room temperature. The buffy coat was removed, washed with PBS+, and pelleted at 800 × g for 5 minutes at 4°C; the supernatant was discarded and cells were re-suspended in PBS+ before antibody staining.
To obtain AMs, mice were killed by cervical dislocation and bled via transection of the abdominal aorta before perfusion of lungs with 3 ml of ice-cold PBS injected through the right ventricle. The trachea was then cannulated with a blunt 22-gauge needle and tied in place. Bronchoalveolar lavage (BAL) was performed with PBS containing 0.5 mM ethylenediaminetetraacetic acid (EDTA). Aliquots of 0.5 to 1 ml were instilled into the lungs under direct observation to ensure that all segments of lung were inflated, and aspirated back into the syringe. This was repeated five times per mouse. Peritoneal macrophages were obtained by flushing 10 ml of 0.5 mM EDTA in ice-cold PBS into the peritoneal cavity, which was then aspirated back.
Flow Cytometry
Immunostaining was performed using fluorochrome-conjugated monoclonal rat anti-mouse antibodies directed at CD45.1, CD45.2, and CD11b. All antibodies were purchased from BD Pharmingen (San Jose, CA). Propidium iodide (PI) was used at a concentration of 2 μg/ml (Sigma-Aldrich) to exclude dead cells in all analyses. Flow cytometric analysis was performed on either a Mo-Flo instrument (Cytomation, Fort Collins, CO) or a FACScan instrument (Becton Dickinson, San Jose, CA). A 488-nm air-cooled argon-ion laser was used for excitation of fluorescein isothiocyanate (FITC), phycoerythrin (PE), and PI. A 647-nm argon-krypton diode laser was used for excitation of allophycocyanin (APC). Data analysis for all flow cytometry experiments was performed using FloJo software.
PKH26 Labeling and Analysis
One hundred microliters of PKH26 (Red Fluorescent Phagocytic Cell Linker Kit; Sigma-Aldrich) at a 1:5 dilution was injected intravenously via retro-orbital injection into 6-week-old C57BL/6 mice. BAL cells from mice were obtained at 2 and 28 days after injection and analyzed for fluorescent intensity in the FL-2 channel. PI was used at a concentration of 2 μg/ml to exclude dead cells in all analyses. Mean fluorescent intensity (MFI) at each time point was computed by averaging the MFIs obtained from each mouse.
Histology/Cytology
Three months after transplant, shielded and unshielded mice were killed before removal of lungs for paraffin embedding. Random lung sections were then stained with hematoxylin and eosin and examined by light microscopy. In another set of mice, AMs were isolated from three shielded and three unshielded mice 10 weeks after transplant and stained with Diff-Quick staining reagents (Fisher Scientific, Hanover Park, IL) or with a mac-3 antibody (BD Pharmingen) before examination. An average of 500 cells were counted per animal under a ×40 objective; using standard cytologic criteria, cells were identified as macrophages or nonmacrophages.
Statistical Analysis
Data are expressed as mean ± SEM. Differences in chimerism between peripheral blood leukocytes, CD11b phenotype, and BAL macrophages were calculated using a paired t test. An unpaired t tests were used to compare differences in CD45 phenotypes between cohorts. Statistical significance was defined as P < 0.05.
RESULTS
Bone marrow chimeric mice were generated by bone marrow transplantation from alloantigen-expressing CD 45.1 donor mice into CD 45.2 recipients. Before transplantation of cells, recipient mice were divided into two cohorts. The first cohort received total body irradiation with 9.5 Gy. The second cohort also received 9.5 Gy, but their thorax, head, and upper extremities were shielded with lead, thereby solely irradiating the abdomen, pelvis, and lower extremities. The AM in the shielded mice were, therefore, protected from any untoward effects from the irradiation. Using CD 45.1– and CD 45.2–specific fluorescent antibodies, flow cytometric analysis was performed on peripheral blood leukocytes (PBL) and BAL cells of both cohorts at sequential time points to quantify engraftment and relative half-life (2 wk to 8 mo after transplant). There was a statistically significant difference in the PBL chimerism between the shielded and unshielded cohorts of mice at all time points (P value 2.52 × 10−12). This difference was expected, as the shielding process also protects the bone marrow of the skull, ribs, sternum, forelimbs, and cervical and thoracic vertebrae. As a result, the shielded cohort of mice demonstrated subtotal engraftment of donor marrow, although the majority of circulating PBL were of donor origin (62% ± 1.9%).
In unshielded mice, 20 to 40% of AMs by 4 weeks and greater than 90% by 8 weeks after transplant were of donor phenotype (Figure 1). This yields an approximate half-life of around 30 days for AMs, consistent with previous reports using similar techniques. Donor-derived PBL chimerism was 80% at 4 weeks, and greater than 95% at subsequent time points. By 16 weeks, there was no statistically significant difference detected between the chimerism in the PBL and BAL. In the shielded cohort, however, we found that by 33 weeks (8 mo) there was negligible replacement (1–5%) of recipient AMs by donor cells, despite reconstitution of recipient blood by donor marrow (Figure 1). The difference in chimerism between PBL and BAL was statistically significant at all time points in the shielded cohort of mice.



Chimerism of peripheral blood leukocytes (PBL) and bronchoalveolar lavage (BAL) cells over time. (A) Representative flow cytometric analyses of donor (PE, y-axis) versus recipient (FITC, x-axis) chimerism in the PBL and BAL of shielded and unshielded animals at 4, 8, and 33 weeks after transplant. Boxed areas in BAL plots represent alveolar macrophages (AMs) of recipient phenotype. (B) Percent donor cells (CD45.1) in PBL (squares) and BAL (triangles) of unshielded and shielded mice 2 to 33 weeks after transplant. A paired two-tailed t test was used to compare PBL and BAL chimerism at each time point. *Statistically significant difference (P < 0.05). Number of animals for each time point is indicated except for Week 33, where n = 3 for shielded and n = 2 for unshielded animals. (C) Summary of P values for each time point.
To ensure that the discrepancy between the chimerism found in the BAL and PBL of shielded mice was not due to a preferential distribution of cells of the monocyte lineage from recipient marrow, we analyzed PBMCs, after separation by Ficoll density gradient to remove erythrocytes and neutrophils, for expression of the monocyte marker CD11b (Figure 2) (14). In the shielded cohort, 75% of the PBMCs are of donor phenotype; similarly, 75% of the CD11b PBMCs demonstrate a donor origin. Therefore, the discrepancy in chimerism observed between the BAL and PBL of the shielded cohort is not due to a skewed distribution of monocytes from recipient marrow.

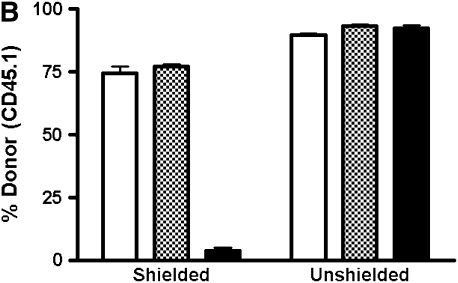

Chimerism in peripheral blood mononuclear cells (PBMCs), CD11b+, and peritoneal lavage cells. (A) Graph showing percentage of PBMCs of donor origin that express the monocyte marker CD11b in shielded and unshielded mice 6 weeks after transplant (n = 3 for each group). Lightly shaded bars, % CD45; darkly shaded bars, % CD11b. (B) Graph showing percent of cells expressing a donor phenotype in peritoneal lavage (PL; open bars), PBL (shaded bars), and BAL (solid bars) of shielded and unshielded animals 10 weeks after transplant (n = 3 for each group). (C) Representative flow cytometric analysis of donor (PE, y-axis) versus recipient (FITC, x-axis) phenotypes from PL, PBL, and BAL of a shielded and unshielded animal.
To confirm that the discrepancy in AM chimerism observed between the two cohorts of mice was due to regional shielding, we analyzed the chimerism in the peritoneal lavage. The peritoneum is an ideal site to investigate, as it is predominantly populated by macrophages in the unchallenged state, yet, in our model, was subjected to the same dose of radiation in both cohorts of mice. While peritoneal macrophages are phenotypically different from AM, they are tissue macrophages of the monocyte lineage. In contrast to the BAL, we found that the chimerism of macrophages in the peritoneal compartment mirrored the chimerism of the PBL in both cohorts of mice; demonstrating that the effect of the regional shielding was specific for the thorax. These data suggest that peritoneal, and perhaps all, tissue macrophages are sensitive to 9.5 Gy of radiation, leading to death and subsequent replacement by circulating monocytes (Figure 2).
To confirm that there were no differences in the gross ultrastructural or inflammatory effects resulting from irradiation and shielding, we performed hematoxylin and eosin staining on lung sections, and performed cytologic and Mac-3 staining of BAL cells (see Figure E1 in the online supplement) (15). By light microscopy, we could not distinguish any differences in lung histology or obvious inflammatory changes in shielded and unshielded cohorts. We examined the BAL of three shielded and three unshielded mice 10 weeks after transplant, and assessed the percentage of AM after staining with Diff-Quik; macrophages constituted 96% (± 0.005) of shielded, 97% (± 0.007) of unshielded, and 97% (± 0.008) of wild-type BAL cells.
We next set out to determine whether AMs are simply long-lived cells or, as has been suggested in several studies, maintained through local proliferation (7, 8, 12, 13, 16–18). To help distinguish between these possibilities, AMs were labeled by the intravenous injection of the red fluorescent dye PKH26; this is a highly amphipathic dye that is known to be taken up by macrophage populations and stable for approximately 1 month (19). One important characteristic of PKH26 labeling is that fluorescence intensity is halved at each cell division (20, 21). We thus reasoned that if AMs undergo local proliferation or if they are replenished by a local progenitor, there should be a decrement in MFI over time that is associated with a shift in the overall distribution of fluorescent intensities. On the other hand, if AMs are long-lived, MFI should remain stable over time and there should be no shift. For this study, we determined the MFI of AMs recovered via BAL at 2 days and 1 month after injection. Notably, we detected no difference in the MFI or in the distribution of fluorescent intensities at 2 days and 1 month (Figure 3). This finding effectively excludes local proliferation or progenitor-related reconstitution within this time frame, consistent with an intrinsic prolonged AM life-span.
DISCUSSION
The AM is one of the central components of the innate immune system of the lung. Since a monocyte origin for the macrophage was first demonstrated by Virolainen in 1968 there has been much speculation about their kinetics (1). The preponderance of evidence over the years has come down on the side of the AM as a short-lived cell, continuously replenished by the circulating pool of monocytes (6, 7, 10, 11). The minority viewpoint argues that, while the AM is a short-lived cell, they undergo renewal from local proliferation (8, 12, 13, 22, 23).
In 1982 Sawyer and coworkers showed that resident macrophage populations in BAL and peritoneal lavage remained unaffected after inducing a severe monocytopenia with strontium-89; however, they only made observations out to 15 days (22). Nevertheless, the authors concluded that “local proliferation must account” for the sustained levels of tissue macrophages observed in these compartments (22). Similarly, in 1982 and then in 1987 Tarling and colleagues published two articles that examined the effect of regional radiation, as well as multiple low doses of radiation, on AM kinetics (12, 13). Both studies showed preservation of AM out to 45 days; their conclusion for these findings was that there must be local proliferation to maintain the AM pool.
Our data effectively exclude a significant contribution of local proliferation supporting the AM. On the other hand, recent data based on using conditional ablation and adoptive transfer of AM through an elaborate transgenic mouse system support a role for local proliferation (23). It is important to note, however, that in this study proliferation was observed after a direct injury to the AM population. Indeed, a complex relationship between injury, monocyte recruitment, and macrophage proliferation is further supported by the findings of Maus and coworkers that showed accelerated repopulation of the AM compartment after BMT (< 2 mo) in the setting of endotoxin-induced lung inflammation (9).
To reiterate, our PKH labeling experiment showed little evidence for active proliferation of AM under steady-state conditions. If the half-life of the AM were as little as even 4 months, we should have been able to detect this by PKH labeling. Given the linear relationship between PKH labeling and MFI, a 4-month half life would have resulted in a 12.5% reduction in MFI at 1 month (i.e., the MFI should have decreased from 670 to 590); however, we detected no change in MFI.
Taken together, our data conclusively and reproducibly show the murine AM to be a very long-lived cell, with a life-span not dissimilar to the animal itself. We speculate that the short half-lives evidenced in previous studies were confounded by the toxic effects of pre-conditioning regimens and, thus, do not describe the true homeostatic kinetics of the AM itself. These findings have significant bearing on the design and interpretation of experiments relating to AM biology. These findings may also explain, in part, why pulmonary macrophages serve as an attractive niche for chronic pathogens such as HIV and Tb.
Notes
This work as supported by grants R21 HL082778 (to A.F), K08 HL077138 (to R.S.), and 5R21 HL086414 (to D.K.).
This article has an online supplement, which is accessible from this issue's table of contents at www.atsjournals.org
Originally Published in Press as DOI: 10.1165/rcmb.2007-0224RC on January 10, 2008
Conflict of Interest Statement: None of the authors has a financial relationship with a commercial entity that has an interest in the subject of this manuscript.
References
Articles from American Journal of Respiratory Cell and Molecular Biology are provided here courtesy of American Thoracic Society
Full text links
Read article at publisher's site: https://doi.org/10.1165/rcmb.2007-0224rc
Read article for free, from open access legal sources, via Unpaywall:
https://europepmc.org/articles/pmc2274942?pdf=render
Free to read at intl-ajrcmb.atsjournals.org
http://intl-ajrcmb.atsjournals.org/cgi/content/abstract/38/4/380
Free after 12 months at intl-ajrcmb.atsjournals.org
http://intl-ajrcmb.atsjournals.org/cgi/content/full/38/4/380
Free after 12 months at intl-ajrcmb.atsjournals.org
http://intl-ajrcmb.atsjournals.org/cgi/reprint/38/4/380.pdf
Citations & impact
Impact metrics
Article citations
Genetic absence of PD-L1 does not restore CD8<sup>+</sup> T cell function during respiratory virus infection and delays virus clearance.
J Virol, 98(10):e0079724, 23 Sep 2024
Cited by: 0 articles | PMID: 39311697
What could be the function of the spinal muscular atrophy-causing protein SMN in macrophages?
Front Immunol, 15:1375428, 28 May 2024
Cited by: 0 articles | PMID: 38863697
Review
Trained immunity inducers in cancer immunotherapy.
Front Immunol, 15:1427443, 16 Jul 2024
Cited by: 1 article | PMID: 39081326 | PMCID: PMC11286386
Review Free full text in Europe PMC
The guardians of pulmonary harmony: alveolar macrophages orchestrating the symphony of lung inflammation and tissue homeostasis.
Eur Respir Rev, 33(172):230263, 01 Apr 2024
Cited by: 1 article | PMID: 38811033 | PMCID: PMC11134199
Review Free full text in Europe PMC
Diesel exhaust particles induce polarization state-dependent functional and transcriptional changes in human monocyte-derived macrophages.
Am J Physiol Lung Cell Mol Physiol, 326(1):L83-L97, 12 Dec 2023
Cited by: 0 articles | PMID: 38084400
Go to all (130) article citations
Data
Data behind the article
This data has been text mined from the article, or deposited into data resources.
BioStudies: supplemental material and supporting data
Similar Articles
To arrive at the top five similar articles we use a word-weighted algorithm to compare words from the Title and Abstract of each citation.
Optimal timing to repopulation of resident alveolar macrophages with donor cells following total body irradiation and bone marrow transplantation in mice.
J Immunol Methods, 292(1-2):25-34, 01 Sep 2004
Cited by: 55 articles | PMID: 15350509
Resident alveolar macrophages are replaced by recruited monocytes in response to endotoxin-induced lung inflammation.
Am J Respir Cell Mol Biol, 35(2):227-235, 16 Mar 2006
Cited by: 132 articles | PMID: 16543608
Macrophage Turnover Kinetics in the Lungs of Mice Infected with Streptococcus pneumoniae.
Am J Respir Cell Mol Biol, 38(1):105-113, 09 Aug 2007
Cited by: 58 articles | PMID: 17690327
Conditions of retinal glial and inflammatory cell activation after irradiation in a GFP-chimeric mouse model.
Invest Ophthalmol Vis Sci, 51(9):4831-4839, 30 Apr 2010
Cited by: 26 articles | PMID: 20435601
Funding
Funders who supported this work.
NHLBI NIH HHS (3)
Grant ID: R21 HL082778
Grant ID: 5R21 HL086414
Grant ID: K08 HL077138