Abstract
Free full text

Polycomb Complex 2 Is Required for E-cadherin Repression by the Snail1 Transcription Factor
†
Abstract
The transcriptional factor Snail1 is a repressor of E-cadherin (CDH1) gene expression essential for triggering epithelial-mesenchymal transition. Snail1 represses CDH1, directly binding its promoter and inducing the synthesis of the Zeb1 repressor. In this article, we show that repression of CDH1 by Snail1, but not by Zeb1, is dependent on the activity of Polycomb repressive complex 2 (PRC2). Embryonic stem (ES) cells null for Suz12, one of the components of PRC2, show higher levels of Cdh1 mRNA than control ES cells. In tumor cells, interference of PRC2 activity prevents the ability of Snail1 to downregulate CDH1 and partially derepresses CDH1. Chromatin immunoprecipitation assays demonstrated that Snail1 increases the binding of Suz12 to the CDH1 promoter and the trimethylation of lysine 27 in histone H3. Moreover, Snail1 interacts with Suz12 and Ezh2, as shown by coimmunoprecipitation experiments. In conclusion, these results demonstrate that Snail1 recruits PRC2 to the CDH1 promoter and requires the activity of this complex to repress E-cadherin expression.
The Snail1 transcriptional factor (SNAI1 [accepted HUGO nomenclature]) belongs to a family of transcriptional repressors required for the conversion from an epithelial to a mesenchymal phenotype. This process, known as epithelial-mesenchymal transition (EMT), occurs at the early stages of development and is essential for gastrulation and formation of the third embryonic layer (27). Therefore, elimination of Snai1 expression prevents gastrulation (6). EMT occurs not only during normal embryonic development but also in pathological situations, such as acquisition of the invasive phenotype in epithelial tumors, in which it constitutes the first step for metastasis formation. Expression of Snail1 promotes EMT in epithelial tumor cell lines, a process that is associated with the downregulation of CDH1 expression (1, 4). Indeed, loss of CDH1 expression is crucial for the progression from adenoma to carcinoma (35). Repression of the CDH1 promoter by Snail1 protein requires binding of the C-terminal domain to 5′-CACCTG-3′ elements (E boxes) present in this promoter and the interaction of the SNAG sequence located in the N terminus with histone deacetylases (HDACs) (1, 32). Moreover, Snail1 induces the expression of the Zeb1 transcriptional repressor (19). This factor also binds to the E boxes in the CDH1 promoter, inhibiting the expression of this gene (18). Therefore, Snail1 represses CDH1 expression both directly, through its direct interaction with this promoter, and indirectly, inducing the synthesis of other repressors (33).
Besides CDH1, Snail1 represses the expression of other genes during EMT (33). Among them, inhibition of the transcription of the PTEN gene is associated with a higher level of resistance to apoptosis, another characteristic of cells expressing Snail1 (11). The PTEN promoter also contains E box sequences where Snail1 binds; however, unlike CDH1, Zeb1 does not repress the activity of this promoter (11).
Gene expression is normally associated with acetylation of histone tails, a modification required for the decompaction of chromatin structure and for increased accessibility of transcription factors to the DNA. However, histone tails are modified not only by acetylation but also by phosphorylation, ubiquitinylation, sumoylation, and methylation, with specific consequences for chromatin function (23, 25). Among these modifications, lysine methylation has been found to play an important role in the control of gene expression (reviewed in references 23 and 42). Different enzymes responsible for the specific lysine methylation in histones have been characterized. For instance, lysine 27 on the histone H3 is di- and trimethylated by Ezh2, a component of the Polycomb group of proteins (5, 37). Polycomb proteins (PcG) are required to preserve the repression of the homeotic genes during the development (29) as well as to initiate the inactivation of chromosome X (38). It has been proposed that binding of Drosophila PcG repressive complex 1 (PRC1) blocks the recruitment of transcriptional activating factors, such as SWI/SNF, thus facilitating the formation of a repressive chromatin structure and/or preventing the transcription initiation by prebound factors (8).
In contrast to the PcG target genes in Drosophila that present specific DNA sequences called Polycomb response elements (39), mammalian Polycomb response elements have not yet been identified. Thus, it remains unclear how mammalian PcG complexes (PRC2/3) are recruited to chromatin to regulate expression of specific target genes. Recently, some PcG mammalian target genes have been reported (2, 24, 37). These target genes include several regulators of signaling pathways involved in development and cell differentiation. Binding of the PRC2 members Suz12, Ezh2, and Eed to these promoters, such as the Myt1 promoter, correlates with trimethylation of lysine 27 in histone H3 (2). This mark enables the further association of the chromodomain of Polycomb (Pc), a subunit of PRC1, and silencing of the targeted promoter (37).
Genetic manipulation of the PRC2 components has revealed that activity of this complex is required in early stages of mouse development (12, 28, 31). In fact, depletion of either Suz12 or Ezh2 prevents completion of gastrulation. Here, we report that CDH1 gene expression is deregulated in Suz12-deficient animals. We demonstrate that PRC2 is required for the repression of CDH1 in tumor cell lines and that this repression is associated with Suz12 binding to this promoter and trimethylation of lysine 27 in histone H3. We also demonstrate that Snail1 is responsible for PRC2 recruitment to the CDH 1 promoter.
MATERIALS AND METHODS
Cell lines and reagents.
Cell lines were grown in Dulbecco's modified Eagle's medium-10% fetal bovine serum. The generation and properties of RWP1 cells stably transfected with Snai1-hemagglutinin (HA) has previously been described (34). Cloning and characterization of the wild-type and mutated CDH1 and SNAI1 promoters and the Snai1-P2A mutant have previously been described (34). Mammalian expression vectors encoding the Ezh2 mutant H694L (10) and RNA interference expression plasmids specific for Suz12 (31), Ezh2, or green fluorescent protein (GFP) (20) have previously been described. RNA interference plasmids specific for SNAIL1 were designed (5′-GATCCCCCTCAACTGCAAATACTGCAATTCAAGAGATTGCAGTATTTGCAGTTGATTTTTG GAAA-3′) together with their complementary counterparts, annealed, and subcloned into a pSUPER vector digested with BglII and HindIII. The preparation and use of monoclonal antibodies against Ezh2 (AC22 or BD43), Suz12 (2AO9), and Snail1 has previously been reported (3, 13, 31, 41). The hybridoma E910 was used to analyze the myc tag. Anti-pyruvate kinase was from Sigma; anti-K27me3 and anti-Suz12 were from Abcam, Upstate, and Santa Cruz; anti-E-cadherin was from BD Biosciences; and anti-HA tag was from Roche. Goat anti-rabbit antibody-Alexa Fluo-568 and goat anti-mouse antibody-Alexa Fluo-488 were from Invitrogen.
Derivation, culture of ES cells, and embryoid body formation.
Derivation, culture of embryonic stem (ES) cells, and embryoid body formation (ES differentiation) were performed as described previously (30, 31).
Immunohistochemistry.
Paraffin sections were obtained from embryonic day 7.5 (E7.5) wild-type or Suz12-deficient (31) murine embryos or E9.5, E15, and E18 wild-type embryos. Sections were deparaffined in xylene and rehydrated. Snail1 antigenic recovery was carried out with a pressure cooker for 15 min in Tris-EDTA buffer, pH 9. For the Suz12 immunohistochemistry, antigenic recovery was carried out with 2 N HCl for 30 min at 37°C. The slides were washed in borate buffer and digested in 0.01% trypsin for 2 min. After the endogenous peroxidase was blocked with 4% H2O2 for 15 min, sections were incubated for 2 h in phosphate-buffered saline supplemented with 3% bovine seroalbumin and with monoclonal antibodies anti-Snail1 (12) and anti-Suz12 (31) overnight. E7.5 embryos were stained with rabbit polyclonal antibody anti-Suz12 (Upstate). Bound antibodies were detected using the Envission system (Dako, Glostrup, Denmark), following the manufacturer's instructions. Sections were counterstained with hematoxylin.
Transient transfection and retroviral infection.
RWP-1 cells grown under standard conditions (34) were transiently transfected with Lipofectamine and Plus reagent (Invitrogen). Phoenix Gag-polymerase producer cells were used to generate retroviral stocks, using the calcium phosphate transfection method. SW-620 and HT29-Snail1 (1) were infected with viral supernatant in the presence of polybrene (4 μg/ml; Sigma).
For the luciferase reporter assay, 50 ng of a CDH1 promoter [pGL3-E-cad (−178/+92)], either the wild type or a form with the three E boxes mutated (1), was transfected in RWP-1 cells. Cells were cotransfected with pcDNA3-Snai1-HA in the presence of small interfering RNA (siRNA) against Suz12 (200 ng) or GFP (200 ng) as a control. A simian virus 40-Renilla luciferase plasmid (1 ng) was cotransfected to control the efficiency of transfection. The activities of the firefly and Renilla luciferases were analyzed at 48 h posttransfection according to the manufacturer's instructions.
Real-time RT-PCR.
The expression levels of E-cadherin and Snail1 were determined by real-time reverse transcription-PCR (RT-PCR) (3, 34). Total RNA was extracted 48 h after transfection or infection by using a Gene Elute mammalian total RNA kit (Sigma). Quantitative determination of RNA levels was performed in triplicate, using the primers indicated in Table S1 in the supplemental material. RT-PCR and data collection were performed with an ABI PRISM 7900HT sequence detection system.
ChIP assays.
Chromatin immunoprecipitation (ChIP) assays were performed as previously described (34) in order to detect the endogenous promoter. For the exogenous CDH1 promoter, 1 μg of a CDH1 promoter [pGL3-E-cad (−178/+92)], either the wild type or a form with the three E boxes mutated, were transfected in RWP-1 stably transfected with Snail1 with either the wild type or the P2A mutant. Cells (4 ×106) were cross-linked with 1% formaldehyde for 10 min. Cells were lysed in a buffer IP1 (50 mM Tris, pH 8, 2 mM EDTA, 0.1% NP-40, and 10% glycerol) for 10 min at room temperature. The pellet obtained was then lysed in IP2 buffer (50 mM Tris, pH 8, 10 mM EDTA, and 1% sodium dodecyl sulfate [SDS]). Sonication was performed five times at 40% for 10 seconds (Branson) to generate 200- to 1,500-bp DNA fragments. Immunoprecipitation was carried out with anti-Snail1, anti-Suz12, anti-K27me3, and irrelevant immunoglobulin G (Sigma) in IP buffer (16.7 mM Tris, pH 8, 167 mM NaCl, 1.2 mM EDTA, 1.1% Triton X-100, 0.01% SDS). Samples were treated with elution buffer (100 mM Na2CO3, 1% SDS, and proteinase K) and incubated at 65°C overnight to reverse formaldehyde cross-linking. DNA was purified using a GFX PCR DNA and gel band purification kit (Amersham). Promoter regions were detected by quantitative PCR Sybr green staining (Qiagen). The transfection efficiencies of the two promoters (wild type and mutated) were analyzed by quantitative PCR in the input samples, using specific primers for the luciferase gene. PCR and data collection were performed with the ABI PRISM 7900HT system. The ChIP results were quantified relative to the input amount, according to reference 14.
Immunoprecipitations, pulldown assays, and Western blot analyses.
Snail1-HA stably transfected RWP-1, HT 29 M6-Snai1 cells, and SW-620 were lysed in 50 mM Tris, 2 mM EDTA, 0.1% NP-40, 10% glycerol supplemented with protease and phosphatase inhibitors for 5 min on ice. The samples were centrifuged at 3,000 rpm for 15 min, and the supernatant was discarded. The nuclear pellet was lysed in radioimmunoprecipitation buffer for 30 min at 4°C, and the lysate was subjected to immunoprecipitation with anti-Suz12 (Abcam), monoclonal anti Snail1, or irrelevant immunoglobulin G overnight at 4°C. Protein G-Sepharose beads (Roche) were added for 1 h at 4°C. Precipitations were washed with radioimmunoprecipitation buffer and then resuspended in Laemmli buffer. Proteins were resolved in SDS-polyacrylamide gel electrophoresis gel and analyzed with anti-Suz12 (Santa Cruz) and anti-HA (Roche).
RESULTS
The PRC2 component Suz12 is required for the correct expression of E-cadherin in murine embryos and ES cells.
In order to check whether expression of the Cdh1 gene is controlled by PRC2, we analyzed the E-cadherin expression pattern in murine embryos deficient for one of the essential components of this complex, Suz12. Previous reports indicated that depletion of Suz12 prevents embryo progression beyond E8.5 due to compromised gastrulation (31). The Snail1 and E-cadherin expression levels in the Suz12 knockout murine embryos were analyzed by immunohistochemistry. As shown in Fig. Fig.1,1, whereas control embryos (Suz12+/−) display alternative and inverse distributions of E-cadherin (Fig. (Fig.1a)1a) with respect to Snail1 (Fig. (Fig.1b)1b) in different areas, Suz12 mutants (Suz12−/−) show a high proportion of cells where the expressions of both proteins were simultaneously detected (Fig. 1c and d). In these cells, Snail1 is detected in the nucleus (Fig. (Fig.1d,1d, detail), ruling out the possibility that impaired repression of E-cadherin is a consequence of the altered Snail1 subcellular localization. This result suggests that, in the absence of PRC2 activity, Snail1 cannot properly repress Cdh1.
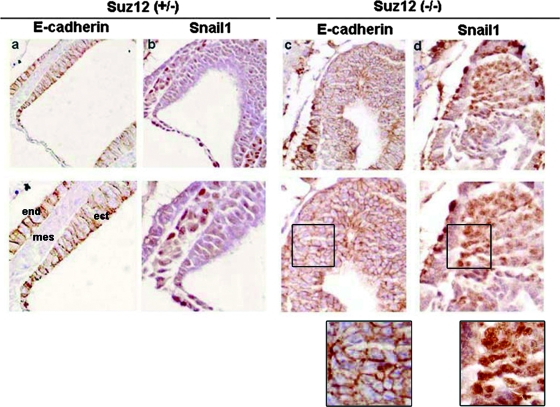
Expression of E-cadherin is altered in Suz12−/− E7.5 embryos. Sagittal sections from E7.5 Suz12+/− or Suz12−/− embryos were immunostained with antibodies against E-cadherin (a and c) or Snail1 (b and d). The lower panels show a higher magnification of the same section. Magnifications: upper panels, ×200; lower panels, ×400. Details of the lower images in panels c and d are also shown. mes, mesoderm; ect, ectoderm; end, endoderm.
Similar results were obtained using ES cells. ES cells were cultured in the absence of leukemia inhibitory factor (LIF) in order to induce differentiation to embryonic bodies. After 6 or 9 days of LIF removal, Cdh1 mRNA levels in Suz12+/− ES cells were completely downregulated, whereas Snail1 mRNA was increased, with a maximum at day 6, as we determined by quantitative RT-PCR (qRT-PCR) (Fig. (Fig.2).2). In Suz12−/− ES cells, Cdh1 mRNA levels were lower at day 0, and they were only partially downregulated during differentiation (approximately 50% of the initial value in these cells). As a consequence, Cdh1 mRNA levels were substantially higher at day 9 of differentiation in Suz12−/− cells than in control ES cells. These higher levels of Cdh1 were not a consequence of impaired Snail1 synthesis in Suz12−/− cells, since Snail1 mRNA was upregulated during differentiation even to a higher extent in these cells than in control cells. Therefore, these results suggest again that Snail1 cannot properly repress Cdh1 in the absence of PRC2 activity.
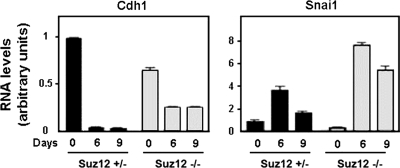
The E-cadherin expression pattern is altered in Suz12−/− ES cells. Embryonic bodies from Suz12+/− or Suz12−/− ES cells were allowed to form hanging drops for 2 days in the absence of LIF and collected at the indicated time points. E-cadherin (Cdh1) and Snail1 (Snai1) mRNA levels were determined by qRT-PCR.
Depletion of PRC2 components affects CDH1 repression by Snail1 in tumor cells.
The involvement of PRC2 in the repressive activity of Snail1 on CDH1 expression was also investigated with tumor cell lines. PRC2 activity was blocked by ectopic expression of an siRNA against Suz12 that significantly decreased the endogenous levels of this protein (see Fig. S1 in the supplemental material). Suz12 knockdown totally prevented the ability of Snail1 to downregulate CDH1 mRNA levels in RWP-1 cells (Fig. (Fig.3A,3A, left panel). The effect of this siRNA on the repressive effect of Snail1 on the CDH1 promoter was studied. As shown in Fig. Fig.3B,3B, expression of Snail1 in epithelial cells decreased the activity of the CDH1 promoter, an effect that was dependent on the integrity of the three E boxes present in this promoter (see also reference 1). Simultaneous expression of Suz12 siRNA diminished the effect of Snail1 on this promoter, although it did not totally abolish it (Fig. (Fig.3B3B).
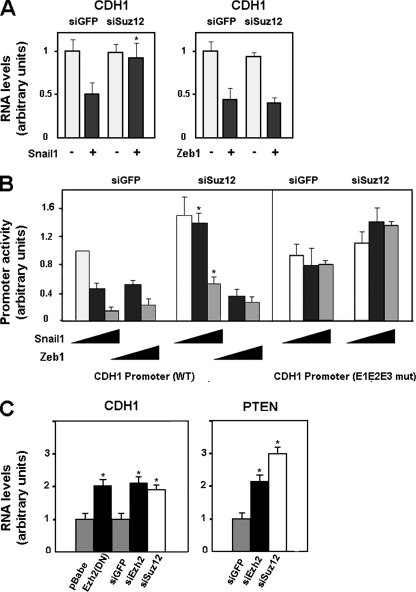
PRC2 downregulation affects CDH1 repression by Snail1 in tumor cell lines. (A) CDH1 mRNA levels were determined by qRT-PCR after coinfecting RWP-1 cells with a retrovirus carrying an siRNA specific for Suz12 and Snail1 or cotransfecting the siRNA for Suz12 and Zeb1 (siSuz12). An siRNA for GFP (siGFP) was used as a control. Cells were selected for 48 h with puromycin after the transfection and before isolation of the RNA. The figure shows the averages ± standard deviations (SD) for three experiments performed in triplicate. With respect to the repression of CDH1 RNA obtained with Snail1 in control cells, the reduced effects of Snail1 in siSuz12 cells were significant, with P values of <0.01 (indicated by asterisks). (B) The activities of the indicated forms of the CDH1 promoter (wild type [WT] and mutated [E1E2E3 mut]) in RWP-1 cells were determined by transient transfection of the pGL3-E-cad (−178/+92) CDH1 promoter, pcDNA-3-Snail1, or pcDNA-3-Zeb1 in the presence of an siRNA against Suz12 or GFP. Averages ± SD (three experiments performed in triplicate) are shown. The asterisks indicate values of repression of the CDH1 promoter that were significantly different in siGFP versus siSuz12 cells, with P values of <0.01. (C) SW-620 cells were infected with a mutant of Ezh2 (H649L), a control plasmid (pBabe), or a retrovirus containing an siRNA specific for Suz12, Ezh2 (siEzh2), or GFP as a control. CDH1 and PTEN mRNA levels were determined by qRT-PCR analysis as described above. The figure shows the averages ± SD for five experiments performed in triplicate. The differences indicated with single asterisks were significant, with P values of <0.01.
As mentioned in the introduction, CDH1 expression is also controlled by the Zeb1 repressor. We checked whether the action of this repressor was also controlled by PRC2. As shown in Fig. Fig.3A3A (right panel), downregulation of CDH1 mRNA levels caused by Zeb1 expression was not affected by Suz12 siRNA. Similar results were obtained when the Zeb1 effect on the CDH1 promoter activity was determined by a reporter assay: depletion of Suz12 did not significantly alter the CDH1 promoter repression by Zeb1 (Fig. (Fig.3B).3B). Therefore, these results suggested that Snail1 and Zeb1 use different mechanisms to directly inhibit CDH1.
We also analyzed the effect of PRC2 interference on CDH1mRNA levels in cells of SW-620, a cell line presenting low E-cadherin and high Snail1 and Zeb1 expression levels (1). As shown in Fig. Fig.3C,3C, disruption of PRC2 activity derepresses the CDH1 gene. Similar results were obtained using siRNA against Ezh2 or Suz12 or siRNA expressing a dominant-negative Ezh2 mutant (H694L) (20) (see Fig. S1 in the supplemental material). A comparable upregulation of CDH1 mRNA levels was obtained after Suz12 siRNA transfection in HT-29 M6 and in RWP-1 cells stably transfected with Snai1 (data not shown).
Snail1 protein also represses the expression of the PTEN gene. Therefore, we also analyzed the effect of PRC2 inactivation on the mRNA levels of this gene. As shown in Fig. Fig.3C,3C, Suz12 siRNA in SW-620 cells also upregulated PTEN mRNA, indicating that the requirement of PRC2 for Snail1 repression is not limited to the CDH1 gene.
Snail1 recruits PRC2 complex to the CDH1 promoter.
By ChIP assays, Cdh1 promoter sequences were detected bound to Snail1 and Suz12 only after differentiation of control ES cells (Fig. (Fig.4A),4A), when the expression of this gene is repressed (Fig. (Fig.2).2). Similar results were observed when we determined the levels of trimethylated K27 in histone H3 (K27me3-H3) in the Cdh1 promoter, since methylation of this lysine is the result of the activity of PRC2. As expected, association of Suz12 and trimethylation of K27 in H3 in the Cdh1 promoter were not detected in Suz12-null cells. Surprisingly, Snail1 was not detected bound to the Cdh1 promoter in these cells either, suggesting that the presence of the Polycomb complex is necessary to stabilize the Snail1 repressive complex at the promoter.
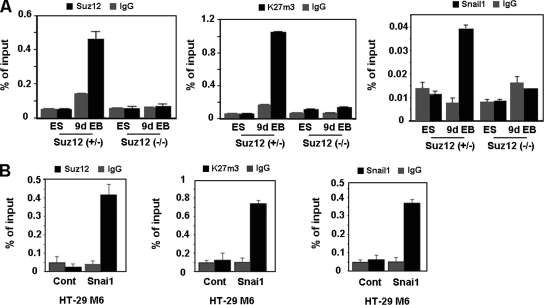
Suz12 binding to the CDH1 promoter is Snail1 dependent. (A) Embryonic bodies from Suz12+/− or Suz12−/− were derived from ES cells which were allowed to form hanging drops for 2 days in the absence of LIF and collected at day 9. Binding of Snail1 and Suz12 and levels of K27me3 in the CDH1 promoter were determined by a ChIP assay at the undifferentiated (ES) and differentiated (9d EB) states. The results show the averages ± SD for two experiments performed in duplicate. IgG, immunoglobulin G. (B) Binding of Snai1-HA and Suz12 and levels of K27me3 in the CDH1 promoter in HT-29 M6 cells stably transfected with Snai1-HA were determined by a ChIP assay. The results show the averages ± SD for three experiments performed in triplicate. Cont, control.
ChIP assays performed with cell lines also confirmed that the recruitment of Suz12 to the CDH1 promoter is Snail1 dependent. As shown in Fig. Fig.4B,4B, binding of Snail1 and Suz12 and levels of K27me3-H3 in the CDH1 promoter were detected in HT-29 M6 clones stably expressing Snai1 but not in the control clones.
We also analyzed whether Suz12 occupancy at the CDH1 promoter was dependent on the integrity of the E boxes located in the CDH1 promoter. We transfected stable RWP-1-Snai1 cells with either the wild-type CDH1 promoter or a promoter containing the mutated E boxes (E1E2E3 CDH1 promoter). ChIP assays were performed to analyze the association of Snail1, Suz12, or K27me3-H3 with ectopic promoters. As shown in Fig. Fig.5A,5A, the amount of the CDH1 promoter immunoprecipitated with Suz12 or K27me3-H3 was substantially lower when binding of Snail1 was prevented by mutation of the three E boxes.
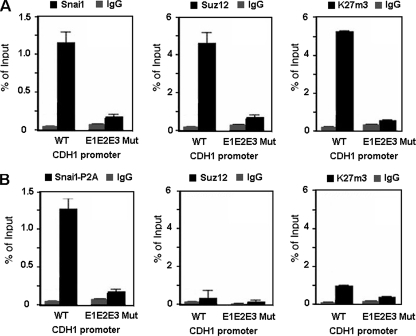
PRC2 binding and activity in the CDH1 promoter require the integrity of the Snail1-binding site and the SNAG domain of Snail1. RWP-1 stably transfected with Snai1 (A) or Snai1-P2A mutant (B) cells was transiently transfected with an exogenous CDH1 promoter, either the wild type (WT) or a form with the three E boxes mutated (E1E2E3 Mut). The transfection efficiencies were the same for the two promoters. Binding of Snai1-HA, Snai1-P2A-HA, and Suz12 and levels of K27me3 in the exogenous CDH1 promoter were determined by a ChIP assay. The results show the averages ± SD for two experiments performed in triplicate. IgG, immunoglobulin G.
We also determined if the Snai1 P2A mutant, a mutant unable to repress transcription (1), was capable to recruit the PRC2 complex to the CDH1 promoter. As shown in Fig. Fig.5B,5B, in contrast to what was observed with the wild-type form, the Snai1-P2A mutant did not induce Suz12 binding to the CDH1 promoter. As a consequence, the levels of K27me3-H3 associated with this promoter were lower than those in cells expressing wild-type Snai1 (Fig. (Fig.5B5B).
Snail1 depletion affected Suz12 binding and K27me3-H3 levels in the CDH1 promoter. As shown in Fig. Fig.6A,6A, a SNAI1-specific siRNA compromised not only the binding of the Snail1 protein to the CDH1 promoter in SW-620 cells but that of Suz12 as well. As expected, the presence of K27me3-H3 in the CDH1 promoter was also decreased in the absence of Snail1.
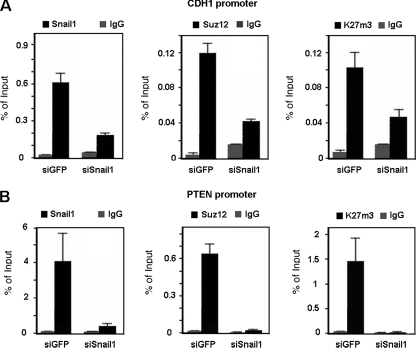
PRC2 recruitment is Snail1 dependent. ChIP assays were carried out as described in Materials and Methods, immunoprecipitating cross-linked nuclear extracts from SW-620 cells infected with a retroviral construct generating SNA1-specific small hairpin RNA (siSnail1) or with an siRNA specific for GFP (siGFP) as a control and selected with puromycin for 48 h. Specific binding for Snail1, Suz12, and K27me3 in the CDH1 promoter (A) and in the PTEN promoter (B) was analyzed. The results show the averages ± SD for two experiments performed in triplicate. IgG, immunoglobulin G.
We also checked whether Snail1 knockdown would also affect binding of Suz12 to the PTEN promoter. Association of Snail1 and Suz12 and levels of K27me3-H3 were observed in the promoter region in SW-620 cells (Fig. (Fig.6B).6B). Downregulation of Snail1 expression by use of the specific siRNA decreased the association of Suz12 and, consequently, the K27me3-H3 levels at the PTEN promoter. We also analyzed other Snail1 target genes, such as the SNAIL1 and MUC1 promoters, and we obtained the same results (not shown). Therefore, recruitment of PRC2 by Snail1 is not restricted to the CDH1 promoter.
Snail1 associates with PRC2 components.
Since recruitment of Suz12 to the CDH1 promoter is Snail1 dependent, we investigated whether Snail1 can interact with components of the PRC2 complex. As shown in Fig. Fig.7,7, the presence of HA-tagged Snail1 was observed in the immunoprecipitates obtained with an antibody against Suz12 in RWP-1 (Fig. (Fig.7A)7A) and in HT 29 M6 stably transfected with Snai1-HA (Fig. (Fig.7B).7B). Association of Snail1 with other PRC2 components was also detected, since Ezh2 was coimmunoprecipitated with Snail1-HA in HT 29 M6-Snai1 cells (Fig. (Fig.7C).7C). Moreover, this association was also observed between the endogenous proteins in SW-620 cells; Ezh2 protein was detected in the immunocomplex obtained with a monoclonal antibody against Snail1 (13) (Fig. (Fig.7D7D).
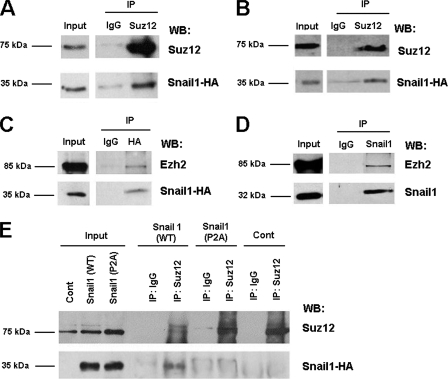
PRC2 components and Snail1 interact in vivo. (A to C) RWP-1 cells (A) and HT-29 M6 (B and C) stably transfected with Snai1-HA were lysed and immunoprecipitated (IP) with antibodies anti-Suz12 (A and B) or anti-HA (C). The immunocomplex was analyzed by Western blotting (WB), using antibodies against HA (A and B), Suz12 (A and B), or Ezh2 (C). IgG, immunoglobulin G. (D) SW-620 cells were lysed and immunoprecipitated with a monoclonal antibody specific for Snail1. The presence of Ezh2 in the immunoprecipitated Snail1 was analyzed by Western blotting (D). (E) RWP-1 cells stably transfected with Snai1-HA or with the Snai1-P2A mutant were immunoprecipitated with an antibody against Suz12. The presence of Snail1-HA and the Snail1-P2A-HA mutant was analyzed by Western blotting. RWP-1 cells stably transfected with pcDNA-3 were used as a control (Cont).
We also performed a coimmunoprecipitation assay with RWP-1 cells stably transfected with the Snail1 P2A mutant or with the wild-type form of this protein. As shown in Fig. Fig.7E,7E, both transfectants express similar amounts of Snail1 protein. However, whereas wild-type Snail1 was observed in Suz12 immunocomplexes, Snail1 P2A was not, suggesting that this mutant was unable to interact with Suz12 and indicating that the same Snail1 protein elements were required for repression, PRC2 binding, and PRC2 recruitment to the CDH1 promoter (Fig. (Fig.66).
Finally, we analyzed Snail1 and Suz12 expression during mouse embryo development in order to characterize whether Snail1-positive cells were also expressing Suz12. As observed in Fig. Fig.88 and according to previous reports (31), Suz12 showed a broader expression than Snail1 and was present in all cells with Snail1 immunoreactivity. More specifically, at E7.5 Snail1 is present only in the mesoderm (Fig. (Fig.8A,8A, left panels), with a contrary distribution to E-cadherin (Fig. (Fig.1).1). Suz12 was detected in the nucleus in this mesenchymal embryonic layer (Fig. (Fig.8A,8A, right panels). At E9.5, we analyzed the mesenchymal cells that are migrating from the neural crest. As we can observe in Fig. Fig.8B8B (left panels) and as expected by RNA analyses (25), Snail1 is present in these cells, which also show expression of Suz12 (Fig. (Fig.8B,8B, right panels). At later stages of development, we focused on hair follicle morphogenesis, a process in which Snail1 is involved (22). As previously reported (13, 22), Snail1 is expressed in the dermal condensate at E15 (Fig. (Fig.8C,8C, left panel) and in the dermal papilla at E18 (Fig. (Fig.8D,8D, left panel), whereas it is absent from the adjacent epidermal cells and the bulb. Suz12 was present in all the cells expressing Snail1, although it showed a more general distribution since it was also detected in the epithelial cells (Fig. 8C and D, right panels).
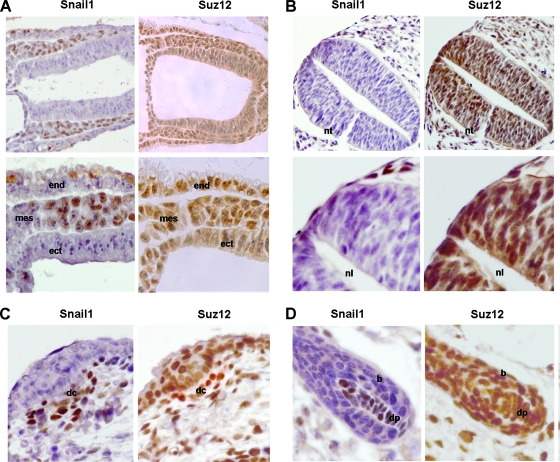
Snail1 and Suz12 are coexpressed in mesenchymal cells from murine embryos. Sagittal sections obtained from murine embryos were analyzed by immunohistochemical analysis of Snail1 and Suz12. (A) Sagittal section from an E7.5 embryo. Magnifications: upper panel, ×200; lower panel, ×400. mes, mesoderm; ect, ectoderm; end, endoderm. (B) E9.5. Magnifications: upper panel, ×200; lower panel, ×400. nt, neural tube; nl, neural lumen. (C) E15. Magnification, ×400. dc, dermal condensate. (D) E18. Magnification, ×400. dp, dermal papilla; b, bulb.
DISCUSSION
In this report, we have investigated the molecular mechanism of CDH1 repression mediated by Snail1. We demonstrate that Snail1 recruits the PRC2 complex to this promoter, since binding of Suz12 is dependent on Snail1 activity and on the integrity of Snail1-binding site in the CDH1 promoter. As a consequence, transcriptional repression by Snail1 associates with trimethylation of lysine 27 in histone H3. This recruitment has a physiological relevance since CDH1 repression by Snail1 is impaired in cells depleted for components of the PRC2 complex, such as Suz12. This altered effect of Snail1 in Suz12 knockdown cells is not limited to CDH1, since the repression of another target gene, the PTEN gene, is also prevented. Moreover, our results indicate that Snail1 can associate with PRC2 components Suz12 and Ezh2, either directly or indirectly, providing a physical explanation for the recruitment of these factors to Snail1-targeted promoters.
Previous results have highlighted the importance of the SNAG domain in the repression of CDH1 gene expression by Snail1 (1). Repression of CDH1 requires the association of the SNAG domain with HDAC1/2, an interaction that is mediated by the corepressor Sin3A (32). Our results suggest that this domain is also required for PRC2 recruitment by Snail1 and for the subsequent methylation of specific associated histones. It is possible that both modifications are interdependent and successive: an initial histone deacetylation is required for the subsequent PRC2 binding and further histone methylation, which ultimately prevents expression of these Snail1 target genes. Several previous genetic and biochemical studies link histone deacetylation to PcG-mediated repression (36, 40). Moreover, since HDACs also associate with the PRC2 complex and since we have not demonstrated that the interaction between Snail1 and PRC2 is direct, it is possible that HDACs are mediating this association.
Our results indicate that PRC2 depletion significantly prevents the downregulation of CDH1 mRNA triggered by Snail1 during development or after expression in tumor RWP-1 cells. However, the effect on cells that have CDH1 already silenced, such as SW-620 cells, is partial. This incomplete effect is not a consequence of CDH1 promoter methylation. Although we have detected binding of DNMT1 to this DNA region, bisulfite genomic sequencing has revealed a lack of promoter methylation in these cells in Snail1 transfectants (data not shown). More likely, this limited upregulation is a consequence of the different repression mechanisms used by Snail1 and other repressors acting on the CDH1 gene, such as Zeb1. We have previously demonstrated that Snail1 induces the expression of Zeb1 (19), which, unlike Snail1, interacts with the CtBP corepressor (7, 9, 18) and does not require PRC2. Several lines of evidence indicate that Zeb1 participates in CDH1 repression in tumor cell lines (9, 17). Moreover, results from our laboratory demonstrate that Zeb1 induction by Snail1 is repressed by E-cadherin overexpression (N. Dave and A. García de Herreros, unpublished results), suggesting that an initial downregulation of E-cadherin is required for Zeb1 expression during EMT. Therefore, it is possible that Zeb1 cannot be induced in cells lacking PRC2 components, explaining why the downregulation of CDH1 initiated by Snail1 presents a high level of dependence of PRC2. However, once Zeb1 is expressed, CDH1 repression is only partially dependent on PRC2 since Zeb1 does not require the action of this complex (Fig. (Fig.3).3). Therefore, depletion of PRC2 only partially upregulates CDH1 mRNA levels in tumor cell lines.
When this article was under revision, Snail1 was shown to interact with the Ajuba LIM and PMRT5 proteins (21, 26). This association is required for E-cadherin repression. Since PRMT5 forms a complex with MEP50 protein, which in turn interacts with Suz12 (15, 16), it is likely that PRMT5 and Ajuba provide the link connecting Snail1 and PRC2, further validating the relevance of our findings.
In conclusion, our results suggest that repression of CDH1 by Snail1 in epithelial cells undergoing EMT requires the association of PRC2 activity with the CDH1 promoter. Therefore, this work provides new insights into the mechanism of Snail1 and associates repression by this factor with epigenetic silencing of CDH1 gene expression.
Acknowledgments
We greatly thank Salvador Aznar-Benitah for the SNAI1-specific siRNA and for helpful discussions and Berta Alsina for her advice. We also thank Marta Garrido and Álvaro Jansà for technical assistance.
This work was supported by Danish National Research Foundation and Danish Medical Research Council grants to K.H. and by grants from the Ministerio de Ciencia y Tecnología (SAF2003-02324 and SAF2006-00339) to A.G.H. Partial support from Instituto Carlos III (RTICCC, C03710) and from the Generalitat de Catalunya (2005SGR00970) is also appreciated. N.H. and M.E were recipients of predoctoral fellowships from the Ministerio de Educación. S.P. was supported by a postdoctoral fellowship from the Ministerio de Educación. V.D. was supported by a La Cierva contract.
Footnotes
Published ahead of print on 2 June 2008.
†Supplemental material for this article may be found at http://mcb.asm.org/.
REFERENCES
Articles from Molecular and Cellular Biology are provided here courtesy of Taylor & Francis
Full text links
Read article at publisher's site: https://doi.org/10.1128/mcb.00323-08
Read article for free, from open access legal sources, via Unpaywall:
https://europepmc.org/articles/pmc2493371?pdf=render
Free to read at mcb.asm.org
http://mcb.asm.org/cgi/content/abstract/28/15/4772
Free after 4 months at mcb.asm.org
http://mcb.asm.org/cgi/content/full/28/15/4772
Free after 4 months at mcb.asm.org
http://mcb.asm.org/cgi/reprint/28/15/4772
Citations & impact
Impact metrics
Citations of article over time
Alternative metrics
Smart citations by scite.ai
Explore citation contexts and check if this article has been
supported or disputed.
https://scite.ai/reports/10.1128/mcb.00323-08
Article citations
Relationship of Signaling Pathways between RKIP Expression and the Inhibition of EMT-Inducing Transcription Factors SNAIL1/2, TWIST1/2 and ZEB1/2.
Cancers (Basel), 16(18):3180, 17 Sep 2024
Cited by: 0 articles | PMID: 39335152 | PMCID: PMC11430682
Review Free full text in Europe PMC
MARCH2, a Novel Oncogene-regulated SNAIL E3 Ligase, Suppresses Triple-negative Breast Cancer Metastases.
Cancer Res Commun, 4(3):946-957, 01 Mar 2024
Cited by: 1 article | PMID: 38457262 | PMCID: PMC10977041
Potential role of epithelial-mesenchymal transition induced by periodontal pathogens in oral cancer.
J Cell Mol Med, 28(1):e18064, 30 Nov 2023
Cited by: 4 articles | PMID: 38031653 | PMCID: PMC10805513
Review Free full text in Europe PMC
Involvement of Epithelial-Mesenchymal Transition (EMT) in Autoimmune Diseases.
Int J Mol Sci, 24(19):14481, 23 Sep 2023
Cited by: 3 articles | PMID: 37833928 | PMCID: PMC10572663
Review Free full text in Europe PMC
ZEB1 Is Regulated by K811 Acetylation to Promote Stability, NuRD Complex Interactions, EMT, and NSCLC Metastasis.
Mol Cancer Res, 21(8):779-794, 01 Aug 2023
Cited by: 0 articles | PMID: 37255406
Go to all (280) article citations
Data
Data behind the article
This data has been text mined from the article, or deposited into data resources.
BioStudies: supplemental material and supporting data
Similar Articles
To arrive at the top five similar articles we use a word-weighted algorithm to compare words from the Title and Abstract of each citation.
Akt2 interacts with Snail1 in the E-cadherin promoter.
Oncogene, 31(36):4022-4033, 12 Dec 2011
Cited by: 26 articles | PMID: 22158034
Snail1 transcriptional repressor binds to its own promoter and controls its expression.
Nucleic Acids Res, 34(7):2077-2084, 14 Apr 2006
Cited by: 101 articles | PMID: 16617148 | PMCID: PMC1440880
A natural antisense transcript regulates Zeb2/Sip1 gene expression during Snail1-induced epithelial-mesenchymal transition.
Genes Dev, 22(6):756-769, 01 Mar 2008
Cited by: 414 articles | PMID: 18347095 | PMCID: PMC2275429
The polycomb group protein Suz12 is required for embryonic stem cell differentiation.
Mol Cell Biol, 27(10):3769-3779, 05 Mar 2007
Cited by: 481 articles | PMID: 17339329 | PMCID: PMC1899991