Abstract
Free full text

Versatile Photosensitizers for Photodynamic Therapy at Infrared Excitation
Abstract
A new type of photosensitizers used in photodynamic therapy, which is based on photon upconverting nanoparticles, is reported. These photosensitizers are excitable with infrared irradiation, which has several times larger tissue penetration depth than the currently available ones. They are brought close to the target cancer cells through antigen-antibody interaction with good specificity and versatility. The design is also flexible in that various photosensitive molecules can be potentially adopted into the design. Results from in vitro experiments demonstrate their promise of becoming the next generation photodynamic therapy drugs.
Photodynamic therapy (PDT) is gaining acceptance as a technique for cancer treatment in recent years. [1-3] PDT utilizes a photosensitizer, working as a light-sensitive drug, to treat the target tissue locally upon the irradiation of light with appropriate wavelengths. It is generally accepted that the mechanism of PDT is based on the interaction between the excited photosensitizer and surrounding molecules, generating reactive oxygen species (ROS), such as singlet oxygen. ROS can cause oxidative damage to biological substrates and ultimately cell death. One of the key components in effective and efficient PDT is the photosensitizers, also called PDT drugs. Features most desired for an ideal PDT drug include: 1) Be specific to the target tissue; 2) Have a high photochemical reactivity, i.e., can effectively produce ROS when exposed to appropriate irradiation; 3) Exhibit little toxicity in the dark; and 4) Can be excited at a wavelength in the region where tissue penetration of irradiation is at a maximum. Here we report the design of a type of versatile photosensitizers, which could potentially satisfy all the above requirements and become the next generation PDT drugs, based on photon upconverting nanoparticles (PUNPs).
Photon upconverting materials convert lower-energy light to higher-energy light through excitation with multiple photons. They have been widely used for some time in such applications as display, bioassay, and bioimaging, to name a few. [4] In this application, we take advantage of the fact that such materials would adsorb infrared irradiation and emit visible light to further excite the photosensitizing molecules. The particles of nanometer size would also facilitate the delivery and excretion of the proposed photosensitizer as PDT drug.
The optimal spectral window for biological tissue penetration of irradiation is around 800 nm to 1 μm. Yet, single photons with infrared wavelengths are usually energetically too low for singlet oxygen generation. Multiphoton excitation using infrared light as irradiation source has been proposed and explored. [5-9] Experiment to combine photon upconverting materials with well-established PDT drug was mentioned, albeit with very limited efficiency in generating singlet oxygen.[10]
The new design of versatile PDT photosensitizers we propose is schematically shown in Figure 1. PUNPs are first coated with a porous, thin layer of silica. During the coating process, photosensitizing molecules with high absorbance in the spectral window matching the emission of the PUNPs are doped, so that the resulting silica layer contains a certain amount of these photosensitizing molecules. Finally, an antibody, specific to antigens expressed on the target cell surface, is covalently attached to the silica-coated nanoparticles. When the thus-prepared nanoparticles are irradiated by infrared light, emission from the PUNPs will be absorbed by the photosensitizing molecules coated on their surfaces. Subsequently, excited photosensitizing molecules will interact with surrounding groundstate molecular oxygen, generating singlet oxygen, leading to oxidative damage of the neighboring cells to which the nanoparticles are attached via specific antigen-antibody binding. As a proof of concept, we carried out experiments to demonstrate the feasibility of this design.
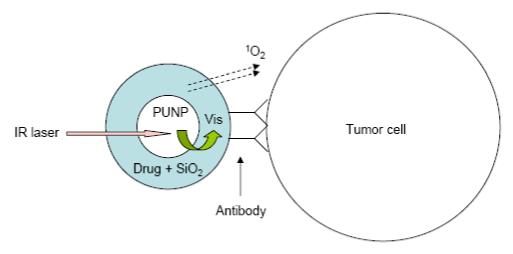
Schematic of the design of the versatile photosensitizer based on PUNPs. Dimensions not drawn to scale.
The PUNPs used in this study are NaYF4:Yb3+,Er3+ nanoparticles, which have been recognized as one of the most efficient photon upconverting phosphors. [11] They are synthesized following the procedures reported in the literature [12] with some modifications. When excited by an infrared (974 nm) source, strong visible bands appear around 537 nm and 635 nm. The silica coating was realized through sol-gel reaction. Merocyanine 540 (M-540) is used as photosensitizing molecule, and doped into the silica layer during the coating process. M-540 is a molecule that can produce singlet oxygen and other reactive oxygen species, and has been used before in photodynamic therapy as a photosensitizer with a visible light source. [13] Both the emission spectrum of NaYF4:Yb3+,Er3+ nanoparticles and the absorption spectrum of M-540 are shown in Figure 2, displaying a good overlap between the nanoparticles’ emission and M-540’s absorption. The photon upconverting property of the nanoparticles was not affected by the silica coating, as confirmed by their photoluminescence spectrum. The presence of M-540 in the silica coating could be readily confirmed by the change in color of the nanoparticles, to slightly yellowish.
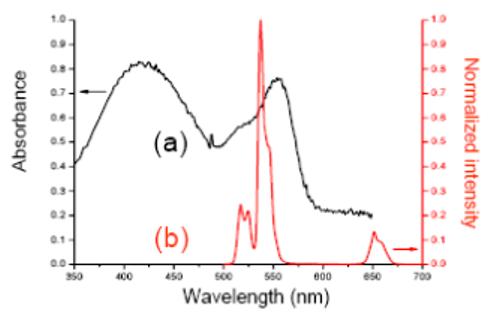
(a) Absorbance spectrum of Merocyanine 540. (b) Emission spectrum of PUNPs used in this study, excited by a 974 nm laser.
Generation of singlet oxygen by these PUNPs-based photosensitizers was detected chemically, using the disodium salt of 9, 10-anthracenedipropionic acid (ADPA) as a singlet oxygen sensor. [14] When excited at around 374 nm, the fluorescence emission of ADPA displays two strong bands centering around 400 nm and 420 nm, respectively. In the presence of singlet oxygen, ADPA is bleached to its non-fluorescent endoperoxide. By measuring the decrease of the ADPA’s fluorescence bands, we can monitor the generation of singlet oxygen.
Fluorescence measurements to detect the generation of singlet oxygen from the PUNPs-based photosensitizers were carried out using a spectrofluorometer. An M-540-coated nanoparticles suspension in buffer was mixed with a small amount of ADPA. The solution was first irradiated for several minutes at 540 nm using a Xenon lamp, while the 402 nm emission peak of ADPA (excited at 374 nm) was measured at different time intervals. The decrease of the ADPA fluorescence intensity over time confirmed the singlet oxygen generation from the M-540-coated nanoparticles under visible light irradiation matching M-540’s absorption band. The solution was then illuminated by an infrared laser of 974 nm, which also led to the decrease of ADPA fluorescence intensity. The fluorescence emission of ADPA at 402 nm was measured at different time intervals, as shown in Figure 3.
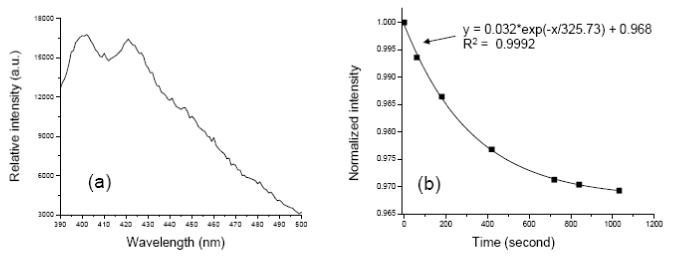
Emission spectrum of ADPA, excited at 374 nm. (b) Change of normalized intensity of ADPA’s emission peak at 402 nm over time, when the mixture containing ADPA and M-540-coated PUNPs was irradiated by a 974 nm laser. The line represents the fitting of the data points, using the listed equation with correlation coefficient R2.
It has been shown in previous reports that the intensity decrease of ADPA emission follows an exponential decay over time, as ADPA being quenched by the generated singlet oxygen. [15,16] The data points in Figure 3 could be nicely fit into an exponential decay function, with a correlation coefficient of 0.9992. This suggests that the kinetics of the M-540-coated PUNPs generating singlet oxygen is very similar to that of other silica-based nanoparticles described in the literature.[15,16] The major difference is that the M-540-coated PUNPs were excited by IR irradiation, while those others were excited by visible irradiation. This difference would allow the penetration depth to increase several times for the PUNPs-based PDT drugs, a highly desired feature in clinical applications.
In vitro PDT tests were performed to confirm the photodynamic cytotoxicity of the M-540-coated PUNPs towards MCF-7/AZ breast cancer cells. MCF-7/AZ cells express high levels of MUC1/episialin. A mouse monoclonal antibody, anti-MUC1/episialin, which is highly specific towards the MCF-7/AZ cells, was covalently attached to the M-540-coated PUNPs, following similar procedures described previously. [17] Next, two sets of experiments were carried out. In the first experiment, MCF-7/AZ cells in suspension were incubated with Trypan Blue, a vitality dye that does not stain the cell unless the cell membrane is damaged, and the antibody-functionalized M-540-coated PUNPs. Subsequently, MCF-7/AZ cells were placed onto an inverted microscope, and illuminated by an IR laser, coupled through the microscope objective. Optical images of MCF-7/AZ cells were taken at different time intervals to monitor cell viability. Images of MCF-7/AZ cells before IR irradiation and after 36 minutes of IR irradiation are shown in Figure 4a and 4b, respectively. After IR irradiation, MCF-7/AZ cells displayed shrinkage, and stained with Trypan Blue, indicating induced cell death. In the second experiment, MCF-7/AZ cells in suspension were incubated with propidium iodide (PI), a fluorescence dye that stains the nuclei red once the cell is dead or dying, and the antibody-functionalized M-540-coated PUNPs. Using the same microscope system, fluorescence images of MCF-7/AZ cells were taken at different time intervals to monitor cell viability while they were illuminated by the IR laser. Images of MCF-7/AZ cells before IR irradiation and after 43 minutes of IR irradiation are shown in Figure 4c-4e (optical and fluorescence), respectively. The fluorescence of the cell in the upper left corner (Figure 4d) increased over time under IR irradiation, indicating the dying process of the cell. Note that, in both experiments, cells in the same dishes, undergoing same treatment with the antibody-functionalized M-540-coated PUNPs and respective dyes but without IR irradiation, do not display cell death during the same period of time, which confirms that the cell killing is directly associated with the IR irradiation. Control experiments with IR irradiation but without nanoparticles had also been done, which showed that IR irradiation alone did not cause cell death.
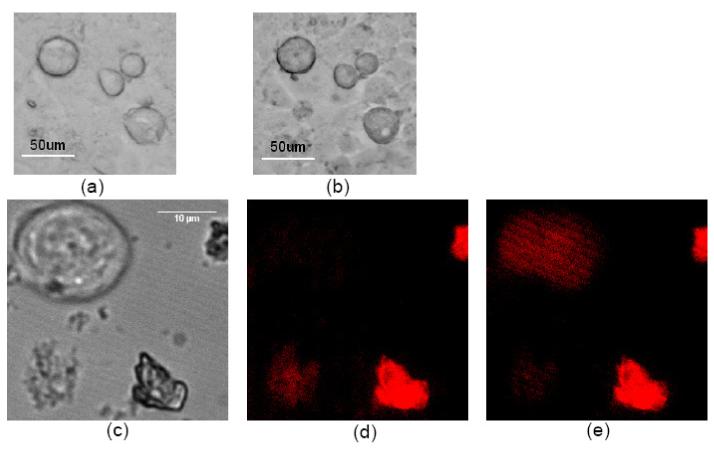
(a) MCF-7/AZ cells incubated with Trypan Blue and antibody-functionalized M-540-coated PUNPs modified with antibody on surface. (b) MCF-7/AZ cells in (a) after 974 nm laser irradiation for 36 minutes. (c) Optical image of MCF-7/AZ cells incubated with PI and M-540-coated PUNPs modified with antibody on surface. (d) Fluorescence image of MCF-7/AZ cells in (c) before 974 nm laser irradiation. (e) Fluorescence image of MCF-7/AZ cells in (c) after 974 nm laser irradiation for 43 minutes.
These results demonstrate that photosensitizers of the proposed design have great potential to become the next generation PDT drugs. Compared to the PDT drugs currently available, the proposed PUNPs-based photosensitizers display the following advantages: 1) Excitable at a wavelength in the infrared region where tissue penetration of light is several times deeper. 2) Highly specific. Unlike the current PDT drugs relying on the relatively greater affinity of the drug for the malignant cells, the photosensitizers are brought to the target through antigen-antibody interaction. 3) Expanding the pool of photosensitizing molecules as PDT drugs. The fact that photosensitizing molecules are encapsulated inside the silica layer allows the use of some molecules which would otherwise be unlikely candidates. In addition, there are a lot more green- or blue-absorbing, than red-absorbing, molecules available to be considered as PDT drugs. 4) Versatile for different types of tumors. There are a variety of antibodies and other ligands available for different types of tumors, which can be attached to the silica surface of the PUNPs to target the desired sites in vivo. [18] Indeed, in vivo PDT studies using these newly-designed photosensitizers are planned to be carried out next, along with the optimization of parameters, such as the type and amount of photosensitizing molecules loaded in the silica layer, the amount of antibody coated on the silica surface, and the intensity of the infrared irradiation. (Supporting Information available.)
ACKNOWLEDGMENT
This work was partially supported by the start-up fund to P. Z. from New Mexico Tech, and by Grant Number RR-016480 from the National Center for Research Resources (NCRR), a component of the National Institutes of Health (NIH).
REFERENCES
Full text links
Read article at publisher's site: https://doi.org/10.1021/ja0700707
Read article for free, from open access legal sources, via Unpaywall:
https://europepmc.org/articles/pmc2528873?pdf=render
Citations & impact
Impact metrics
Article citations
Quantum dots for bone tissue engineering.
Mater Today Bio, 28:101167, 03 Aug 2024
Cited by: 0 articles | PMID: 39205871 | PMCID: PMC11350444
Review Free full text in Europe PMC
Towards overcoming obstacles of type II photodynamic therapy: Endogenous production of light, photosensitizer, and oxygen.
Acta Pharm Sin B, 14(3):1111-1131, 04 Nov 2023
Cited by: 3 articles | PMID: 38486983 | PMCID: PMC10935104
Review Free full text in Europe PMC
Photoswitchable upconversion nanoparticles with excitation-dependent emission for programmed stepwise NIR phototherapy.
iScience, 26(10):107859, 09 Sep 2023
Cited by: 1 article | PMID: 37766981 | PMCID: PMC10520541
Development of an Fe2+ sensing system based on the inner filter effect between upconverting nanoparticles and ferrozine.
RSC Adv, 13(37):26313-26322, 01 Aug 2023
Cited by: 1 article | PMID: 37671000 | PMCID: PMC10475975
Photodynamic therapy in oral cancer: a review of clinical studies.
Med Oncol, 40(3):91, 07 Feb 2023
Cited by: 18 articles | PMID: 36749489
Review
Go to all (197) article citations
Data
Data behind the article
This data has been text mined from the article, or deposited into data resources.
BioStudies: supplemental material and supporting data
Similar Articles
To arrive at the top five similar articles we use a word-weighted algorithm to compare words from the Title and Abstract of each citation.
In vivo action spectra, absorption and fluorescence excitation spectra of photosensitizers for photodynamic therapy.
J Photochem Photobiol B, 28(1):101-102, 01 Apr 1995
Cited by: 5 articles | PMID: 7791000
Enhanced Photodynamic Therapy by Improved Light Energy Capture Efficiency of Porphyrin Photosensitizers.
Curr Treat Options Oncol, 24(9):1274-1292, 06 Jul 2023
Cited by: 3 articles | PMID: 37407889
Review
Quantum dot-folic acid conjugates as potential photosensitizers in photodynamic therapy of cancer.
Photochem Photobiol Sci, 10(5):842-851, 09 Apr 2011
Cited by: 26 articles | PMID: 21479314
In vivo excitation of photosensitizers by infrared light.
Photochem Photobiol, 62(2):333-338, 01 Aug 1995
Cited by: 17 articles | PMID: 7480140
Funding
Funders who supported this work.
NCRR NIH HHS (3)
Grant ID: P20 RR016480
Grant ID: P20 RR016480-07
Grant ID: RR 016480