Abstract
Free full text

Self, Mother and Abstract Other: an fMRI study of reflective social processing
Abstract
Using fMRI, we studied the neural correlates of self-referential processing by comparing BOLD signal changes during self and mother conditions of a self-reference effect (SRE) task. Conjunction analysis of these two conditions showed several common areas of significant activation, including the medial aspects of the superior frontal gyri, left inferior frontal gyrus, bilateral temporal poles, left superior temporal sulcus and left precuneus. The locations of the 7 strongest peak activations for the self condition and for the mother condition were compared on a subject-by-subject basis in native space. Of the 119 pairs of peaks explored, 87% were located within 2 voxels of each other, demonstrating the commonality of the brain regions subserving both self- and mother-referential processing within an individual subject. In group analyses of the self-referential vs. mother-referential contrast, small differences in activation strength were observed in the left superior frontal sulcus, right cingulate gyrus and the left fusiform gyrus. Greater activation for mother than for self was observed in bilateral temporal lobes. Subjects also performed a social attribution task (SAT) in which they inferred mental states about interacting geometric shapes. Activations from this visual theory of mind task were compared with the activations demonstrated during self-referential processing. Striking similarities were found, including overlapping activations in bilateral medial prefrontal cortices, left inferior frontal gyrus and precuneus. These data suggest that reflective analysis of self, mother and abstract others relies predominantly on the same neural architecture.
Introduction
The ability of the human brain to result in the experience of having a self is an oft-discussed, but little-understood, phenomenon. Neuroimaging researchers have recently joined the philosophers, psychologists and neurologists attempting to elucidate theoretical and neurofunctional models of the self. A key question has been how neural activity in the brain typically gives rise to a stable and singular reference we call “self” (Damasio, 1999). The experience of having a self is inextricably linked to the understanding that self is distinct from “other.” However, when we think about others we think about them using similar categories and thought processes that we employ when thinking about ourselves, and vice versa. Accordingly, attempts have been made to determine if circuitry related to self-processes are shared by other processes. It remains unclear whether the brain functions in a unique way when performing certain cognitive tasks that relate to the self, or whether there are general mechanisms used in common for considering one’s self and close others (Gillihan and Farah, 2005; Mitchell et al., 2005; Keysers and Gazzola, 2007).
One method of studying the self was introduced by Rogers and colleagues (1977). In their work, subjects made different types of judgments about words, and subsequently performed a recall task based on those words. Rogers’ subjects demonstrated superior recall for the words about which they had made self-referential judgments when compared, for example, to the words about which they had made semantic judgments. This phenomenon of superior recall of self-referred information has been termed the “self reference effect” (SRE). The SRE suggests that the self somehow functions as “a unique mnemonic structure” within the memory system (Symons and Johnson, 1997). As such, the SRE provides a tool with which to investigate whether this “unique” aspect of the self is perhaps subserved by a unique neural circuit.
Because the SRE entails the superior recall of information that has been processed relative to oneself, the degree of the effect depends in large part on the comparison task; i.e. recall that is superior to what? A recent meta-analysis concluded that the SRE is most robust when compared to semantic processing control tasks, and somewhat less robust when an other-referential (OR) control task is used (Symons and Johnson, 1997). Furthermore, the magnitude of the SRE depends on the degree of intimacy that the subject has with the designated other. This has led researchers to consider the SRE as an extension of the depth-of-processing paradigm in memory. In other words, the concept of a person, be it self or intimately-known other, provides a “superordinate schema” (Rogers et al., 1977) by which elaboration and organization can occur. The question is whether accessing a superordinate schema about one’s “self” is neurofunctionally different than accessing a superordinate schema about an “other,” and secondarily, if such differences are best explained by considering different depths of processing rather than “selfness vs. otherness.”
Neuroimaging studies that have focused on the Self Referential (SR) vs. OR comparison have produced mixed results. Some investigators have designated “others” that are not intimately known by the subjects (e.g. George Bush, the Danish Queen, and the Canadian prime minister) (Craik et al., 1999; Kelley et al., 2002; Lou et al., 2004). These studies consistently demonstrate a SR vs. OR effect, both behaviorally and neurofunctionally.
An increasing number of studies are utilizing a more intimately known “other.” Table 1 presents a summary of these studies, including the nature of the intimate other designated for the other-referential condition, locations of significant activations from the Self vs. Other contrasts, and whether or not the studies included a condition in which participants were asked if traits were “positive” or “socially desirable.” As can be seen in Table 1, a consensus about the neurofunctional correlates of self-referential processing has not yet emerged from these studies, though Brodmann’s Area 10 (BA 10) in the dorsomedial prefrontal cortex continues to be defined as a region of interest (Moran et al., 2006). The anterior cingulate gyrus (BA 32) was also identified in 3 of these 6 studies (D’Argembeau et al., 2007; Heatherton et al., 2006; Zhang et al., 2006).
Table 1
Summary of SRE encoding studies that have used an intimately known other.
Authors | Year | Designated Other | Desirability Condition? | Regions activated in Self vs. Other contrast†,* | ||||
---|---|---|---|---|---|---|---|---|
x | y | z | BA | |||||
Zhu, Ying. Zhang, Li. Fan, Jin. Han, Shihui. | 2007 | Mother | No | −4 | 46 | −6 | 10 | ventral MPFC |
| ||||||||
D’Argembeau, Arnard Ruby, Perrine Colette, Fabienne et al. | 2007 | Close Friend or Relative | No** | −10 | 46 | 18 | 9 | L dorsal MPFC |
10 | 44 | 20 | 9 | R dorsal MPFC | ||||
−8 | 48 | −4 | 10 | L ventral MPFC | ||||
12 | 43 | −2 | 10 | R ventral MPFC | ||||
0 | 34 | −2 | 32 | ant. cingulate gyrus | ||||
−4 | −52 | 38 | 7 | precuneus | ||||
| ||||||||
Heatherton, Todd F. Wyland, Carrie L. Macrae, C. Neil et al. | 2006 | Best Friend | No | 0 | 39 | 17 | 32 | ant.cingulate gyrus |
−9 | 55 | 3 | 10 | middle frontal gyrus | ||||
6 | 58 | 3 | 10 | middle frontal gyrus | ||||
−45 | 23 | −11 | 47 | L inferior frontal gyrus | ||||
48 | 34 | −12 | 47 | R inferior frontal gyrus | ||||
45 | 14 | −3 | 47 | R inferior frontal gyrus | ||||
59 | −38 | −1 | 21 | R mid.temporal gyrus | ||||
12 | 23 | 54 | 6 | superior frontal gyrus | ||||
−48 | −68 | −14 | 37 | L fusiform gyrus | ||||
| ||||||||
Zhang, Li. Zhou, Tiangang. Zhang, Jian. Liu, Zuxiang. Fan, Jin. Zhu, Ying. | 2006 | Mother | Yes | −14 | 40 | 4 | 32 | ant. cingulate gyrus |
−10 | 48 | −2 | 10 | ant. cingulate gyrus | ||||
| ||||||||
Ochsner, Kevin N. Beer, Jennifer S. Robertson, Elaine R. et al. | 2005 | Close Other | Yes | No areas activated at P < .005 | ||||
| ||||||||
Schmitz, Taylor W. Kawahara-Baccus, T. Johnson, Sterling C. | 2004 | Close Friend or Relative | Yes | 26 | 51 | 12 | -- | R DLPFC |
−28 | 45 | 12 | -- | L DLPFC | ||||
30 | −39 | −10 | -- | parahippocampal gyrus |
Three facets of the current study design set it apart from other SR vs. OR studies. First, all previous studies employed a design whereby subjects are shown a single word, and asked “Does this word describe you/other?” In the current study, each stimulus consisted of a pair of adjectives, and subjects were instructed to “Choose the word that best describes you/your mother.” This enabled us to equate words within a pair for likeability thereby minimizing the desirability effect of the adjectives themselves. Furthermore, presenting pairs of stimuli and forcing a choice as opposed to a yes/no answer resulted in a longer response time in pilot studies, thereby possibly enhancing the magnitude of the BOLD signal for each of the main tasks.
Second, because intimacy has effected the magnitude of the self-reference effect (Symons and Johnson, 1997), it is important to consider the degree of intimacy between the subject and the other that is designated in the OR condition. However, using an intimately-known other creates a second problem, as it is inherently difficult to control for intimacy in real-life relationships across subjects. We speculate that individuals will have a more fully-developed concept of a person and their attributes the longer that they have known that person. In the current study, we designated “mother” as the “other” in the OR condition because we reasoned that there would be less between-subject variability in the time that each subject had known their mothers, as opposed to the variability that might be seen in the length of friend or partner relationships. Moreover, compared to conditions involving friends or partners, we hoped that the degree of emotional arousal evoked by the decision making process during the mother condition would be more comparable to the emotionality of making decisions about the self. This speculation is based on the unique role that parents have in identity formation and attachment (Zentner and Renaud, 2007; Fonagy et al., 2007). In other words, mother was selected in order to use a comparison condition that was as close as possible to being self, while still being “other.”
Third, we speculate that including a condition where subjects explicitly make desirability judgments primes the participant to notice and consider the likeability of the stimuli that carries over into the self and other conditions. Thus, subjects in our study were not asked to consider the desirability of the adjectives.
Materials and Methods
20 healthy participants (11 females, 9 males, mean age ± s.d = 26.4 ± 3.9), with normal or corrected-to-normal visual acuity, were recruited from the Yale University community. All descriptions of the study informed subjects that they would be asked “personal questions about themselves and their mothers” during the experiment. Participants were screened for neurological and psychiatric disorders through administration of the Adult Self Report Inventory (Gadow et al., 1999), and for history of significant neurological or psychiatric disorders in first-degree relatives using an in-house self report survey. All subjects gave written informed consent for this study and were paid for their participation. This study was approved by the Human Investigations Committee at Yale University School of Medicine.
Of the 20 participants recruited, 3 were subsequently excluded from the study. One participant was found to have a gross atypical structural variation of the hippocampus and surrounding gyri and could not be anatomically aligned with other normal controls. One subject did not perform the task as directed, rendering the functional data unusable. The third subject was an age outlier. Results reported here reflect data analyzed from the remaining subjects, comprised of 10 males and 7 females (mean age ± s.d. = 21.5 ± 1.8).
Experimental Tasks
All 17 subjects completed three runs of the referential processing task. After the first 6 subjects had completed the experiment, we decided to also have the subjects perform the SAT task. Thus, the subject group for the SAT task is comprised of 11 participants. Subjects were fully trained for each experimental task prior to entering the magnet. All stimuli were projected from behind the subject, and were viewed via a prism mirror system mounted on the head coil above the subject’s nose.
Referential Processing Task (RPT)
This task is a variant of the original self-reference task first described by Rogers and colleagues in 1977 (Rogers et al., 1977). We chose a block-design for this study because we wanted each participant to consider their self-concept or mother-concept for as long and as deeply as possible. The block design alternated between three conditions– Self, Mother and Letter, with the stimuli being word pairs that participants were required to choose between (e.g. honest or kind). Participants held a button box in each hand and indicated their choice of the left or right word with the corresponding button.
Words for the active task blocks came from a list of normalized adjectives with likeability ratings (Anderson, 1968). This list was divided by likeability into thirds, and the most and least likeable words were excluded. Word pairs were formed with similarly likeable words, and were balanced for word length and number of syllables. Within each condition, each word was used twice, but in different pairings. Each condition was comprised of 45 word pairs (9 blocks of 5 word pairs), and each of the 3 functional runs contained 3 blocks of each condition. A 3500ms prompt screen (either “Self”, “Mother”, or “A”) introduced each block. The stimulus duration was also 3500ms, and the interstimulus interval was 500ms, resulting in a total block length of 20s. Task blocks were separated by 10s rest periods, comprised of two grey fixation points in the same location as the word pairs, upon a black screen. Blocks were counterbalanced for order of condition appearance within runs.
The Mother condition was completely parallel to the Self condition, except that the participant chose the word from the pair that best describes his or her mother.
“Letter” was a control condition, requiring participants to choose the word that contained the letter “a.” Participants were instructed to read both words during this condition, even if they found the “a” word first.
Social Attribution Task
The original social attribution task (SAT) was the work of Heider and Simmel (Heider et al., 1944), and it was adapted to fMRI by Schultz and colleagues (Schultz et al., 2003). The task employs short (approximately 15s) movies of simple geometric shapes—a diamond, a circle and a square—moving around a box. The box has one side that opens like a hinged door, and the shapes move in, out and around the box in varied ways. In the “Social” condition, these animations are designed so that the shapes are personified and follow a social script. For instance, the shapes can chase each other, play hide-and-seek, or have a fight. The subject watching these movies is asked to decide whether the shapes are “all friends” or “not all friends.” This condition was designed to engage social perceptual and social reasoning processes in the absence of any actual representations of specific people.
The control “Bumper Cars” condition uses the exact same triangle, circle, square, and hinged box. However, in this condition the shapes all move around the center box, and come into contact with each other and diverge from that contact to varying degrees and with different velocities. The subject is asked to imagine that the shapes are “bumper cars” and to decide by watching how they bounce off of each other if the bumper cars are the same or different weights. Thus, subjects have a social context in mind while viewing and making inferences in the first set of movies, and a physical context in mind while viewing and making inferences in the second set.
Subjects were presented with a prompt question, either “People…All friends?” or “Bumper cars…Same weight?” followed by the 15s movie. Subjects were asked to answer the prompt question as soon as the movie was over, but not before. Each condition was comprised of 10 movies, presented alternately across two runs.
Data Acquisition
The referential processing task was programmed and presented using E-Prime software, version 1.1. The SAT task was programmed and presented using PsyScope version 11.1 (freeware). Images were back-projected onto a screen near the end of the MRI gantry. Subject’s heads were immobilized using foam wedges and a strip of tape across the forehead. Responses and response times were collected via fiber-optic button boxes.
Imaging was performed on a Siemens Trio 3 Tesla scanner with a standard quadrature head coil. Thirty-four axial inplane anatomical images were collected using the following pulse sequence: TR = 300ms, TE = 4. 3D structural images were collected with an MP-RAGE sequence (TR = 2530, TE = 3.66, TI = 1100, flip angle = 7, yielding 1 mm3 voxel size). Functional single shot echo planar data was collected with TR = 1950 ms, TE = 25, flip angle = 60, voxel size = 4 mm3 across 34 slices in the same location as the inplane anatomical scans.
Data Analysis
All data analysis was performed using Brain Voyager QX Software (Brain Innovation, Maastricht, Netherlands). Functional runs were pre-processed using 3-dimension (3D) motion correction and spatial smoothing (FWHM = 8mm). No data deviated more than 2 mm in x, y, or z within a run. Linear drift effects were corrected for using a standard temporal filter function. Echo planar data was coregistered with each individual’s high-resolution 3D anatomical data set. The 3D image was then transformed into standardized 3D Talairach coordinate space (Talairach and Tournoux, 1988) using the standard landmarks with piecewise linear warping. Functional data was re-sampled to 3mm3 when it was transformed into 3D Talaraich coordinate space.
Data was modeled following the block design protocol using a general linear model (GLM). Within each block, individual trials were modeled with the standard Boynton hemodynamic response function based on trial level response latencies; pilot data showed that this mixed-block design provided increased sensitivity and statistical power (Boynton et al., 1996). Using the GLM, random effects group composite t-maps were created for specific contrasts. Statistical significance was evaluated using false discovery rate (FDR) < .05, which provides family wise control for multiple comparisons (Benjamini et al., 2001). For the comparison Self vs. Mother, we explored the data with an uncorrected alpha of .001, as there were no regions that survived the more rigorous FDR < .05 threshold.
We also wanted to determine the degree to which each individual subject recruited the same or different brain regions during both self- and mother-referential processing. In order to better determine the actual anatomical relationship between self-peaks and mother-peaks in each subject, the Self vs. Letter and Mother vs. Letter comparisons were analyzed on a subject-by-subject basis in native space. In the majority of subjects, there were so many significant activations that we needed to designate a manageable number of peaks to assess. Our first priority was to assess the main activations used by an individual subject. However, we also wanted to account secondarily for the regions of interest in this study (DMPFC, anterior cingulate and precuneus in particular). We chose to include the top 7 peaks because this was the lowest number of regions that enabled the activations of interest to be captured in the highest number of subjects. The coordinates of the peak voxel of the 7 strongest activations for each individual for Self vs. Letter were recorded. These 7 peaks were then cross-referenced with the Mother vs. Letter contrast, and the location of the peak voxel of the closest distinct and significant activation was recorded. For each individual, the triangulated distance between these peaks was calculated.
Results
Part I. Referential Processing
Behavioral Results
An analysis of variance (ANOVA) contrasting the three encoding conditions (Self, Mother and Letter) revealed a significant main effect of response time (F[2,50]=17.97, p < .0001). Posthoc pairwise analyses showed that response times for Mother and Self conditions did not differ significantly (t[16]=0.19, p=.85), but that response times for each of these were significantly longer than for the Letter task (Self vs. Letter, t[16] = 8.38, p <.0001; Mother vs. Letter t[16] = 8.12, p <.0001). Mean response time for Self was 1965ms ± 487, for Mother 1971ms ± 481 and for Letter, 1312ms ± 302.
fMRI Results
Figure 1 shows sagittal and axial statistical parametric maps for the contrast Self vs. Letter (Figure 1a) and Mother vs. Letter (Figure 1b). Figure 1c shows the results of a conjunction analysis demonstrating significant conjoined activations in both Self vs. Letter and Mother vs. Letter. Areas conjointly activated include the bilateral frontal, left inferior frontal, left posterior and anterior cingulate gyri. Average time courses extracted from the peak activations in the dorsomedial prefrontal cortex, left inferior frontal gyrus and precuneus are shown for all three contrasts. Table 2 shows the Talairach coordinates, approximate Brodmann’s area, t scores and alpha values for the peak activations from the conjunction analysis.
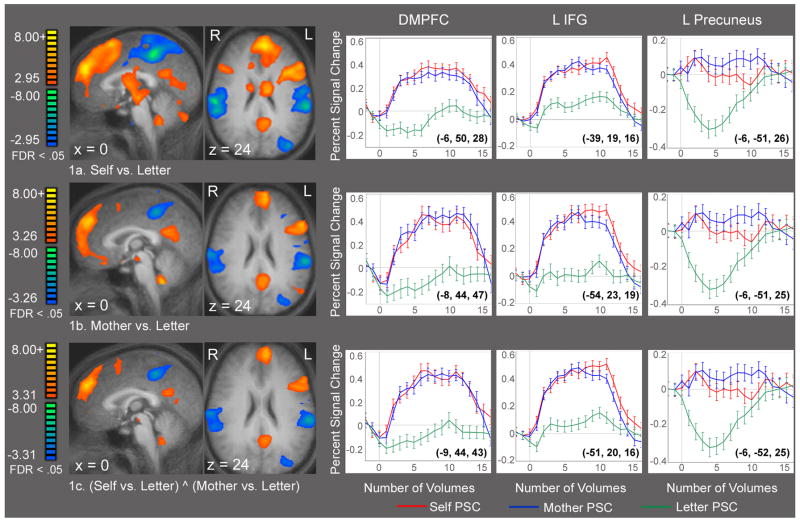
Figure 1a–c. Referential Processing. Composite t-maps displayed on averaged structural brain, n = 17. False discovery rate (FDR) of .05 was used for all comparisons in this figure. 1a–b. Statistical activation maps demonstrating recruitment of similar regions in the Self vs. Letter and Mother vs. Letter contrasts. 1c. Conjunction analysis (Self vs. Letter) ^ (Mother vs. Letter) demonstrating those brain regions that were activated in both Self and Mother conditions. Data shown in 1c combines data from 1a and 1b, but excludes regions that were only activated during either self- or mother-referential processing. Average time courses extracted from the dorsal medial prefrontal cortex, left inferior frontal gyrus and left precuneus regions are also provided for each contrast, showing percent signal change over volumes of time. Talairach coordinates of the peak voxel is in parentheses on each graph. See Table 2 for Talairach coordinates and t-values of the peak voxel for all significant activations of the conjunction analysis.
Table 2
Regions of Peak Activations during Both Self and Mother Conditions, Conjunction Analysis: (Self vs. Letter) ^ (Mother vs. Letter), FDR < .05. Positive activations only.
Talairach Coordinates
| ||||||
---|---|---|---|---|---|---|
Brain Region | BA | x | y | z | T score | P value |
Left DMPFC* | 8 | −9 | 44 | 43 | 8.67 | 1.9 × 10−7 |
Bilateral DMPFC | 9/10 | −6 | 50 | 31 | 8.44 | 2.7 × 10−7 |
Left inferior frontal gyrus | 45 | −51 | 20 | 16 | 8.17 | 4.2 × 10−7 |
Left lateral orbital gyrus | 47 | −45 | 23 | −2 | 7.19 | 2.0 × 10−6 |
Left temporal pole | 38 | −45 | 23 | −23 | 5.80 | 2.7 × 10−5 |
Left precuneus | 23/31 | −6 | −52 | 25 | 5.29 | 7.4 × 10−5 |
Left superior temporal sulcus | 21/22 | −48 | −37 | 1 | 5.16 | 9.5 × 10−5 |
Right DMPFC | 8 | 15 | 26 | 49 | 4.47 | 3.9 × 10−4 |
Right temporal pole | 38 | 42 | 14 | −23 | 4.07 | 8.9 × 10−4 |
Left cuneus | 17/18 | −15 | −55 | 4 | 3.75 | 1.8 × 10−3 |
Right cuneus | 17/18 | 12 | −70 | 7 | 3.81 | 1.5 × 10−3 |
Table 3 is a summary of the degree to which each subject recruited the same regions during both self and mother conditions, as measured by a peak to peak triangulation. Selecting the top 7 peaks for each subject yielded the following regions of interest: DMPFC in 15 subjects, superior frontal gyrus (BA 8) in 15 subjects, precuneus in 12 subjects and anterior cingulate gyrus in 8 subjects. Most notably, of the 119 pairs of peaks assessed, 104 (or 87%) pairs were located within 2 voxels of each other, suggesting that each person activated nearly the exact same tissue for self and mother judgments.
Table 3
Anatomical distance between peak voxels of activations during Self vs. Letter and Mother vs. Letter on a subject-by-subject basis in non-talairached space.
Subject | No. of peaks within 2 voxels (11.31mm) | Avg. distance b/w peaks mm. (± s.d.) |
---|---|---|
1 | 6 | 3.94 (2.07) |
2 | 7 | 4.63 (2.03) |
3 | 6 | 3.76 (2.20) |
4 | 7 | 1.25 (1.64) |
5 | 6 | 2.55 (1.26) |
6 | 5 | 3.49 (2.89) |
7 | 5 | 2.12 (2.69) |
8 | 6 | 1.80 (1.64) |
9 | 5 | 4.80 (1.61) |
10 | 6 | 4.75 (3.95) |
11 | 5 | 1.10 (2.50) |
12 | 7 | 4.92 (2.37) |
13 | 7 | 4.31 (2.68) |
14 | 7 | 5.20 (2.02) |
15 | 7 | 3.96 (2.29) |
16 | 5 | 2.05 (1.94) |
17 | 4 | 3.64 (3.27) |
average | 5.94 (.96) | 3.43 (1.35) |
total | 104/119 |
Figure 2 shows statistical parametric maps for the Self vs. Mother contrast from the referential processing task. Greater activation for Self is depicted in three regions (labeled A-C on Figure 2), including the right anterior cingulate gyrus, right cingulate gyrus and the left superior frontal sulcus.1 Figures 2d–e show greater activation for mother than for self in bilateral periamygdaloid and temporal pole regions. Average time courses extracted from these five regions are also provided in Figure 2. Of particular note, the time course data for the temporal pole activations suggest there was an apparent deactivation during self-referential processing, with no increase during mother-reflection, leading to relatively greater activity during mother than self. The Talairach coordinates, approximate Brodmann’s areas, t scores and alpha values for all peaks present in this comparison at p < .001 are shown in Table 4.
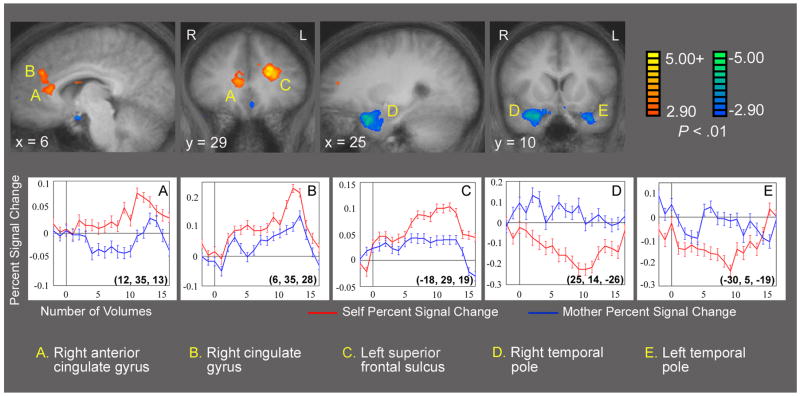
Figure 2 a–e. Referential Processing: Self vs. Mother. Composite t-maps displayed on averaged structural brain, n = 17. Alpha value of < .01 was used for this display. Regions of the right anterior cingulate gyrus, right cingulate gyrus and left superior frontal sulcus are marked a-c. Regions of bilateral temporal lobes are marked d and e. Average time courses extracted from these five regions are provided, showing percent signal change over volumes of time, demonstrating a deactivation for Self in bilateral temporal lobes. Talairach coordinates of the peak voxel is in parentheses on each graph. See Table 4 for Talairach coordinates and t-values of the peak voxels for all significant activations for this contrast.
Table 4
Regions of Peak Activations from Referential Processing Task: Self vs. Mother. P < .001 uncorrected.
Self >.Mother | ||||||
---|---|---|---|---|---|---|
Talairach Coordinates
| ||||||
Brain Region | BA | x | y | z | T score | P value |
Left superior frontal sulcus* | 9 | −18 | 29 | 19 | 5.22 | 8.3 × 10−5 |
Right anterior cingulate gyrus | 32 | 12 | 35 | 13 | 4.45 | .0004 |
Right cingulate gyrus | 32 | 6 | 35 | 28 | 3.94 | .001 |
Left fusiform gyrus† | 37 | −36 | −43 | −8 | 4.78 | .0002 |
| ||||||
Mother > Self | ||||||
Right temporal pole (STG) | 38 | 25 | 14 | −26 | 4.72 | .0002 |
Left temporal pole (STG) | 38 | −30 | 5 | −19 | 4.34 | .0005 |
Left anterior cingulate gyrus | 32 | −3 | 26 | −8 | 3.88 | .001 |
STG = superior temporal gyrus
In order to ascertain the nature of the differences in BOLD signal change seen in Figure 2, these regions were explored further using the contrasts Self vs. Letter and Mother vs. Letter. Comparing these contrasts showed that the activations displayed in Figure 2a–c do not represent regions that were recruited only during self-referential processing. Rather, these changes in BOLD signal depict differences in the strength of shared activations. However, there was one exception—the greater activations for mother as compared with self seen in the bilateral temporal lobes did exist as a true task difference.
Part 2. Social Attribution Task
Behavioral Results
During the SAT, participants were instructed to make their responses after the movie had ended, thus response latencies are not informative. The mean percentages correct for the Friends and Bumper Car conditions were 90.0 ±9.4 and 80.5 ±10.9 respectively (t(df) = 2.38, p = .03).
fMRI Results
Figure 3a–c shows statistical activation maps for the social attribution task, Friends vs. Bumper Cars contrast, using a FDR <.05. This comparison resulted in a widely distributed set of significant activations. Peak areas included in this network are bilateral MPFC, bilateral ventral pathway including the fusiform face area, right amygdala (left amygdala activation was present, but slightly below threshold), bilateral superior temporal sulcus (STS), and right precuneus. These results are consistent with our earlier data from this task in which there was no significant difference in performance between conditions (Schultz et al., 2003). Table 5 contains the coordinates, t scores and alpha values for these peaks.
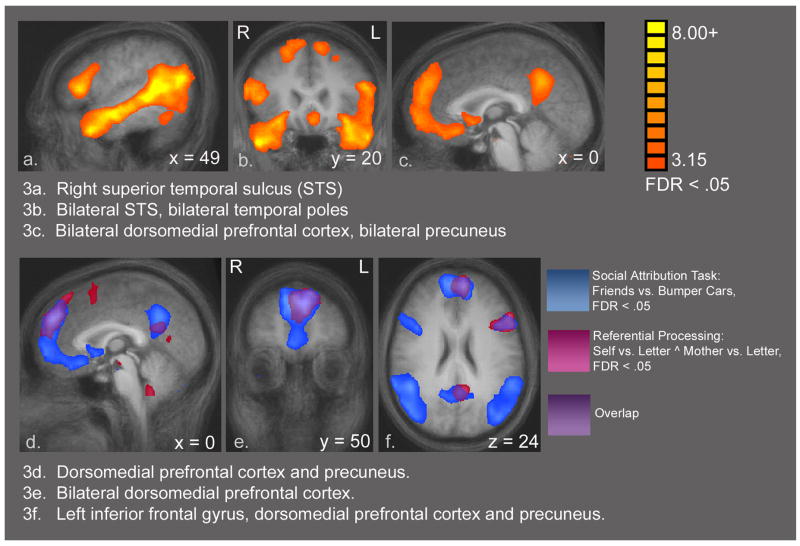
Figure 3a–c. SAT Task: Friends vs. Bumper Cars. Composite t-maps displayed on averaged structural brain, n = 11. FDR of .05 was used. The comparison Friends vs. Bumper Cars demonstrates a network of strong activations including bilateral STS, temporal poles, dorsomedial prefrontal cortices and precuneus. Only positive activations are shown. Talairach coordinates, t-values and alpha values for the peak voxels for all significant activations are provided in Table 5. 3d–f: Overlay Display: SAT and (Self vs. Letter ^ Mother vs. Letter). These images combine activation maps from the referential processing task (17 subjects) and the SAT (11 subjects) in order to show areas of overlap between these two very different tasks. This is not a statistical conjunction or contrast analysis, but simply an overlay display tool. Red areas are the activations from the comparison (Self vs. Letter ^ Mother vs. Letter). Blue areas are the SAT activations from the comparison Friends vs. Bumper Cars. Areas of overlap are consequently shown in purple, and include bilateral medial prefrontal cortices, left inferior frontal gyrus, precuneus and the left superior temporal sulcus (not visible in these views).
Table 5
Regions of Peak Activations from Social Attribution Task: Friends vs. Bumper Cars. FDR <.05, positive activations only.
Talairach Coordinates
| ||||||
---|---|---|---|---|---|---|
Brain Region | BA | x | y | z | T score | P value |
Right STG (posterior) | 22/39 | 45 | −43 | 10 | 14.66 | 4.3 × 10−8 |
Right STS (anterior) | 22/38 | 57 | −7 | −11 | 13.92 | 7.1 × 10−8 |
Left STG (posterior) | 22/39 | −48 | −52 | 16 | 13.17 | 1.2 × 10−7 |
Right STS (anterior) | 22/38 | 54 | −4 | −14 | 12.88 | 1.5 × 10−7 |
Right STS (middle) | 22/21 | 51 | −31 | 1 | 12.41 | 2.1 × 10−7 |
Left middle temporal gyrus | 39 | −39 | −67 | 10 | 11.92 | 3.1 × 10−7 |
Left STS (horizontal posterior) | 21/37 | −51 | −52 | 7 | 11.51 | 4.3 × 10−7 |
Right fusiform gyrus | 37 | 33 | −37 | −16 | 9.01 | 4.0 × 10−6 |
Right inferior frontal gyrus | 45 | 45 | 23 | 7 | 8.76 | 5.0 × 10−6 |
Right DMPFC | 9 | 11 | 53 | 28 | 8.45 | 7.0 ×10−6 |
Left inferior frontal gyrus | 10/47 | −45 | 32 | −2 | 7.98 | 1.2 × 10−5 |
Left fusiform gyrus | 37 | −33 | −37 | −8 | 7.02 | 3.6 × 10−5 |
Right precuneus | 31 | 6 | −58 | 31 | 6.85 | 4.5 × 10−5 |
Right amygdala | -- | 24 | −1 | −17 | 6.56 | 6.4 × 10−5 |
Left DMPFC | 9/10 | −12 | 41 | 37 | 6.23 | 9.8 × 10−5 |
STG = superior temporal gyrus, STS = superior temporal sulcus, DMPFC = dorsomedial prefrontal cortex
Figure 3d–f combines activation maps from the self-referential processing task (see Figure 1c), and the SAT (see Figure 3a–c) in order to show areas of overlap between these two different tasks. It is important to note that this overlay map is not a statistical analysis, but is rather a display tool used to show similarities between two different composite t-maps. Each t-map includes the maximum number of participants (17 for the referential processing task, and 11 for the SAT). In order to ensure that the 6 additional subjects were not driving these results, we ran an exploratory GLM of the self-reference task with just the 11 subjects who had also performed the SAT task. This GLM yielded slightly weaker activations in the same locations as for 17 subjects, ensuring that the 6 subjects are contributing only to the statistical power of the analysis. Only positive activations are shown in the overlay. Areas of overlap between the referential-processing and the abstract social processing tasks include bilateral MPFC, left inferior frontal gyrus, precuneus, and left STS (not shown).
Discussion
This study used a novel version of the SRE paradigm in order to compare BOLD signal changes during self-referential processing and mother-referential processing. Though we expected to see differences particular to the self, what emerged from the data was a strongly integrated network that was recruited for both mother-referential and self-referential processing (see Figure 1, Table 2). Subject-by-subject localization of the peak voxels of these shared activations demonstrated that individual subjects were recruiting the same neural tissue for both conditions (Table 3). Moreover, we demonstrated self-other overlap using both a verbal other task (self referential processing) and a nonverbal task (the social attribution task), and thus the results appear generalizable beyond the specific task manipulations used here (see Figure 3, Table 5). This overlap across tasks is consistent with findings from Saxe et al. who compared a self reference task (self vs. semantic) and a false belief task in the same subjects, and emphasized the overlap in midline regions (MPFC and precuneus) between the self task and the false belief task (Saxe et al., 2006). The current study design utilized comparison judgments, which drove cognitive processes very strongly, as indicated by the robust activations observed. Despite this, the only unique region of the brain that was recruited during self-referential processing was a controversial finding in the temporal poles (Figure 2, Table 4).
In the context of a very robust set of shared activations, the BOLD signal changes that differed between the Self and Mother conditions presented as small, fine-grained differences. Each of the activations seen in Figure 2a–c depicts differences in signal strength in regions that are recruited during both self- and mother-referential processing (see Ochsner et al., 2005 for similar results). Of particular note, we did not see greater activations for Self vs. Mother in the medial prefrontal cortex (BA 10), which other studies have demonstrated (see Table 1, also Northoff et al., 2006 for an extensive review). We attribute the lack of difference between self and mother in this region to intentional elements of the current design, including the choice of “mother” as a closely matched other in terms of intimacy and affective salience (see Zhang et al., 2006 for similar results from a self vs. mother comparison), and the use of paired stimuli that enabled subjects to avoid the conflict-laden process of answering the yes/no or Likert scale question used in all previous studies (“Does this word describe you/your mother?”). Within this paradigm, strong activations in the DMPFC were observed during both self and mother-referential processing, indicating that though this area may be an essential region for self-referential processing, it was not “unique” to the self when the task was structured to avoid potential confounds.
The only regions showing unique activations in the Self vs. Mother contrast were the bilateral temporal poles, which showed greater activation for mother than for self (Figure 2d–e). By comparison, these activations were weaker than those shared in common by the two referential tasks. Exact localization of these activations is complicated by subject-by-subject anatomical heterogeneity, as well as data distortion in this area that is attributable to the proximity of air-filled sinuses (Olson et al., 2007). Furthermore, as shown in the time course data extracted from these regions (Figure 2d–e), these activations do not appear to be driven by an above-baseline activation for mother. Rather, the significance of these activations derives from a seemingly event-locked deactivation below baseline for self. This deactivation was also present when we explored this region in the contrast Self vs. Baseline, while in the comparison Mother vs. Baseline, an activation above baseline was seen in this region. All of these findings together lead us to believe that the deactivation of Self below baseline is a real finding. Trying to understand the significance of this finding is not as straightforward.
Cues may be taken from recent work done on biphasic or dampening patterns of BOLD signal change demonstrated in hippocampal regions (Astur and Constable, 2004; Rekkas et al. 2005; Meltzer et al., 2007). In these studies, deactivations in the hippocampus were observed during tasks that were proven to require recruitment of the hippocampus. As such, the deactivations were known to be in some way related to increased activity. If what we have seen in the current study is similar to these deactivations, the temporal poles may in fact be more active during the self condition. Such a claim might be particularly interesting in the context of a suggestion by Olson et al., who propose that the temporal poles are involved in the storage of perception-emotion linkages, “forming the basis of personal semantic memory” (Olson et al., 2007). However, given that the basic physiological significance of the deactivation pattern of signal change in the temporal poles is not yet established, it is difficult to do anything more than speculate about the significance of this finding.
Overall, the differences between self-referential processing and mother-referential processing are quite small, and in most cases, represent differences in degree of activation rather than the recruitment of unique “self” regions. What is clear from the data is that self-referential processing predominantly relies on a concerted neural network that is recruited during self processing, mother processing, and more abstract social processing.
We believe that the similarity of the self and other networks results from a thoroughly integrated system of self-cognition and other-cognition that is a product of early child development. Within the resultant network, the skills, ideas, beliefs, organizational systems and assumptions used in dealing with the internal world of one’s own mind are also used when dealing with the external social world. As such, these data provide a neurofunctional representation of an interdependent system of social and self cognition that many clinicians, researchers and developmentalists endorse (Aron and Fraley, 1999; Decety and Chaminade, 2003; Mitchell et al., 2006; Saxe et al., 2006; Keysers and Gazzola, 2007; Uddin et al., 2007). A recent fMRI study by Pfeifer and colleagues has highlighted the developmental nature of self and other-cognition (Pfeifer et al., 2007). Their study demonstrated differences during self-referential processing between children and adults, with children demonstrating greater activation during the self condition than the other condition (Harry Potter) in the regions of the MPFC, Brodmann’s Area 10, and the anterior cingulate cortex (Pfeifer et al. 2007). As the authors point out, these findings could be explained by the different degrees of intimacy and emotionality between self and Harry Potter. Nonetheless, even if their results are taken more broadly as social cognitive tasks, the neurofunctional differences between child and adult systems remain relevant.
There are several limitations to the current study. The contrasts used in the peak-to-peak comparison of individual subjects were Self vs. Letter and Mother vs, Letter, leaving open the possibility that the regions investigated were driven by a social cognitive process more generally, as opposed to self and mother processing in particular. The use of a less intimately known other as a baseline condition would obviate central aspects of this problem (see Zhu et al., 2007, Ochsner et al., 2005 and Lou et al., 2004, for such comparisons). The results of this analysis should be interpreted accordingly. Conclusions drawn from the overlay map (Figure 3) should also be made cautiously, as this figure is a visual display of two separate analyses, and is not itself a comparative statistical analysis.
Conclusions
Our data suggest that cognitive processes that relate to the self are not neurofunctionally as unique as we intuitively experience them to be. Furthermore, these results underscore the fact that an integrated functional relationship exists between social processing about others and social processing about the self. William James describes this experientially with regards to one’s immediate family, “[w]hen they die, a part of our very selves is gone. If they do anything wrong, it is our shame. If they are insulted, our anger flashes forth as readily as if we stood in their place” (James, 1918).
Due to the nature of our task design, we can only discuss reflective aspects of self and other referential processing; “pre-reflective” and automatic self and other representations may involve different brain regions, such as the insula (Keysers and Gazzola, 2007) or other mirror neuron systems that were not engaged by the tasks employed here. Given these limitations, we suggest that reflective social processes are an outward projection of processes originating in the development and understanding of one’s self. If this view is accurate, it has rather profound implications. For example, in those with great skill in understanding others, we would predict a well-differentiated understanding of one’s own self. Likewise, in disorders of social cognition we would predict Theory of Mind deficits concomitant with deficits in utilizing and accessing a nuanced understanding of oneself. Recent data from a SRE study in individuals with autism spectrum disorders suggest that in individuals with high-functioning autism, impairments in self-reference, self-focus tasks, and measures of alexithymia, coexist with Theory of Mind deficits (Lombardo et al. 2007).
If it is indeed the case that reflective processes about others derive from and rely on the same neural architecture that supports an understanding of self, it may in fact be implausible in future fMRI studies to subtract out reflective “self processes” from social cognitive processes. Given the developmental model suggested here, the intriguing question is not so much what makes something uniquely “self” in the brain, but rather, what makes something “other” or “nonself.”
Acknowledgments
We would like to thank Cheryl Klaiman, Linda Mayes, James Leckman and Andrés Martin for their invaluable support and input, and Terry Hickey and Karen Martin for their technical expertise during scanning.
Footnotes
1The peak of the latter activation (labeled C) is located in white matter in the group map. When explored on subject-by-subject basis in native space, this activation sourced from the superior frontal sulcus in 8 out of 17 subjects, from left anterior cingulate gyrus in 5 subjects, and from white matter on 3 subjects. The depth and location of sulci in the frontal lobes varied widely between subjects, and likely accounts for the appearance of a white matter location in the group-averaged activation map.
Publisher's Disclaimer: This is a PDF file of an unedited manuscript that has been accepted for publication. As a service to our customers we are providing this early version of the manuscript. The manuscript will undergo copyediting, typesetting, and review of the resulting proof before it is published in its final citable form. Please note that during the production process errors may be discovered which could affect the content, and all legal disclaimers that apply to the journal pertain.
References
- Anderson NH. Likableness Ratings of 555 Personality-Trait Words. Journal of Personality & Social Psychology. 1968;9:272–279. [Abstract] [Google Scholar]
- Aron A, Fraley B. Relationship closeness as including other in the self: Cognitive underpinnings and measures. Social Cognition. 1999;17:140–160. [Google Scholar]
- Astur RS, Constable RT. Hippocampal dampening during a relational memory task. Behav Neurosci. 2004;118:667–675. [Abstract] [Google Scholar]
- Benjamini Y, Drai D, Elmer G, Kafkafi N, Golani I. Controlling the false discovery rate in behavior genetics research. Behav Brain Res. 2001;125:279–284. [Abstract] [Google Scholar]
- Boynton GM, Engel SA, Glover GH, Heeger DJ. Linear systems analysis of functional magnetic resonance imaging in human V1. J Neurosci. 1996;16:4207–4221. [Europe PMC free article] [Abstract] [Google Scholar]
- Craik FIM, Moroz TM, Moscovitch M, Stuss DT, Winocur G, Tulving E, Kapur S. In search of the self: A positron emission tomography study. Psychological Science. 1999;10:26–34. [Google Scholar]
- Damasio A. The feeling of what happens: body and emotion in the making of consciousness. 1. Harcourt Brace; New York; San Diego: 1999. [Google Scholar]
- D’Argembeau A, Ruby P, Collette F, Degueldre C, Balteau E, Luxen A, Maquet P, Salmon E. Distinct Regions of the Medial Prefrontal Cortex Are Associated with Self-referential Processing and Perspective Taking. J Cogn Neurosci. 2007;19:935–944. [Abstract] [Google Scholar]
- Decety J, Chaminade T. When the self represents the other: a new cognitive neuroscience view on psychological identification. Conscious Cogn. 2003;12:577–596. [Abstract] [Google Scholar]
- Fonagy P, Gergeley G, Target M. The parent-infant dyad and the construction of the subjective self. J Child Psychol Psychiatry. 2007;48:288–328. [Abstract] [Google Scholar]
- Gadow K, Sprafkin J, Weiss M. Adult Symptom Inventory [pamphlet] 1999. [Google Scholar]
- Gillihan Seth J, Farah Martha J. Is Self Special? A Critical Review of Evidence from Experimental Psychology and Cognitive Neuroscience. Psychol Bull. 2005;131:76–97. [Abstract] [Google Scholar]
- Heatherton T, Wyland CL, Macrae NC, Demos KE, Denny BT, Kelley WM. Medial prefrontal activity differentiates self from close others. Scan. 2006;1:18–25. [Europe PMC free article] [Abstract] [Google Scholar]
- Heider F, Heider F, Simmel M. An experimental study of apparent behavior. American Journal of Psychology. 1944;57:243–259. [Google Scholar]
- James W. The Principles of Psychology. Authorized. Dover; New York: 1918. [Google Scholar]
- Kelley WM, Macrae CN, Wyland CL, Caglar S, Inati S, Heatherton TF. Finding the self? An event-related fMRI study. J Cogn Neurosci. 2002;14:785–794. [Abstract] [Google Scholar]
- Keysers C, Gazzola V. Integrating simulation and theory of mind: from self to social cognition. Trends in Cognitive Sciences. 2007;11:194–196. [Abstract] [Google Scholar]
- Lombardo MV, Barnes JL, Wheelwright SJ, Baron-Cohen S. Self-referential cognition and empathy in autism. PLoS ONE. 2007;2(9):e883. [Europe PMC free article] [Abstract] [Google Scholar]
- Lou HC, Luber B, Crupain M, Keenan JP, Nowak M, Kjaer TW, Sackeim HA, Lisanby SH. Parietal cortex and representation of the mental Self. Proc Natl Acad Sci USA. 2004;101:6827–6832. [Europe PMC free article] [Abstract] [Google Scholar]
- Mitchell JP, Banaji MR, Macrae CN. The Link between Social Cognition and Self-referential Thought in the Medial Prefrontal Cortex. Journal of Cognitive Neuroscience. 2005;17:1306–1315. [Abstract] [Google Scholar]
- Mitchell JP, Macrae CM, Banaji MR. Dissociable Medial Prefrontal Contributions to Judgments of Similar and Dissimilar Others. Neuron. 2006;50:655–663. [Abstract] [Google Scholar]
- Meltzer JA, Negishi M, Constable RT. Biphasic hemodynamic responses influence deactivation and may mask activation in block-design fMRI paradigms. Hum Brain Mapp. 2007 in press. [Europe PMC free article] [Abstract] [Google Scholar]
- Moran JM, Macrae CN, Heatherton TF, Wyland CL, Kelley WM. Neuroanatomical evidence for distinct cognitive and affective components of self. Journal of Cognitive Neuroscience. 2006;18:1586–1594. [Abstract] [Google Scholar]
- Northoff G, Heinzel A, de Greck M, Bermpohl F, Dobrowolny H, Panksepp J. Self-referential processing in our brain-A meta-analysis of imaging studies on the self. Neuroimage. 2006;31:440–457. [Abstract] [Google Scholar]
- Ochsner KN, Beer JS, Robertson ER, Cooper JC, Gabrieli JD, Kihsltrom JF, D’Esposito M. The neural correlates of direct and reflected self-knowledge. Neuroimage. 2005;28:797–814. [Abstract] [Google Scholar]
- Olson IR, Plotzker A, Ezzyat Y. The Enigmatic temporal pole: a review of findings on social and emotional processing. Brain. 2007;130:1718–1731. [Abstract] [Google Scholar]
- Pfeifer JH, Lieberman MD, Dapretto M. "I Know You Are But What Am I?": Neural Bases of Self- and Social Knowledge Retrieval in Children and Adults. Journal of Cognitive Neuroscience. 2007;19:1323–37. [Europe PMC free article] [Abstract] [Google Scholar]
- Rekkas PV, Westerveld M, Skudlarski P, Zumer J, Pugh K, Spencer DD, Constable RT. Neural correlates of temporal-order judgments versus those of spatial-location: deactivation of hippocampus may facilitate spatial performance. Brain Cogn. 2005;59:103–113. [Abstract] [Google Scholar]
- Rogers TB, Kuiper NA, Kirker WS. Self-reference and the encoding of personal information. J Pers Soc Psychol. 1977;35:677–688. [Abstract] [Google Scholar]
- Saxe R, Moran J, Scholz J, Gabrieli J. Overlapping and non-overlapping brain regions for theory of mind and self reflection in individual subjects. Scan. 2006;1:229–234. [Europe PMC free article] [Abstract] [Google Scholar]
- Schmitz TW, Kawahara-Baccus TN, Johnson SC. Metacognitive evaluation, self-relevance and the right prefrontal cortex. Neuroimage. 2004;22:941–947. [Abstract] [Google Scholar]
- Schultz RT, Grelotti DJ, Klin A, Kleinman J, Van der Gaag C, Marois R, Skudlarski P. The role of the fusiform face area in social cognition: implications for the pathobiology of autism. Philos Trans R Soc Lond B Biol Sci. 2003;358:415–427. [Europe PMC free article] [Abstract] [Google Scholar]
- Symons CS, Johnson BT. The self-reference effect in memory: a meta-analysis. Psychol Bull. 1997;121:371–394. [Abstract] [Google Scholar]
- Talairach J, Tournoux P. In: Co-planar stereotaxic atlas of the human brain: an approach to medical cerebral imaging. Thieme G, editor. Thieme Medical Publishers; Stuttgart; New York, New York: 1988. [Google Scholar]
- Uddin LQ, Iacoboni M, Lange C, Keenan JP. The self and social cognition: The role of cortical midline structures and mirror neurons. Trends in Cognitive Sciences. 2007;11:153–157. [Abstract] [Google Scholar]
- Zentner M, Renaud O. Origins of Adolescents’ Ideal Self: An Intergenerational Perspective. J Pers Soc Psychol. 2007;3:557–574. [Abstract] [Google Scholar]
- Zhang Li, Zhou Tiangang, Zhang Jian, Liu Zuxiang, Fan Jin, Zhu Ying. In Search of the Chinese Self: an fMRI Study. Science in China: Series C Life Sciences. 2006;49:89–96. [Abstract] [Google Scholar]
- Zhu Ying, Zhang Li, Fan Jin, Han Shihui. Neural basis of cultural influence on self-representation. Neuroimage. 2007;34:1310–1316. [Abstract] [Google Scholar]
Full text links
Read article at publisher's site: https://doi.org/10.1016/j.neuroimage.2008.03.058
Read article for free, from open access legal sources, via Unpaywall:
https://europepmc.org/articles/pmc2559963?pdf=render
Citations & impact
Impact metrics
Article citations
Neural Correlates of Antisocial Behavior: The Victim's Perspective.
Brain Sci, 13(3):474, 10 Mar 2023
Cited by: 0 articles | PMID: 36979284 | PMCID: PMC10045997
The Self-Concept Is Represented in the Medial Prefrontal Cortex in Terms of Self-Importance.
J Neurosci, 43(20):3675-3686, 07 Apr 2023
Cited by: 3 articles | PMID: 37028931 | PMCID: PMC10198449
Chinese cognitive processing of ToM: Distinctions in understanding the mental states of self, close others, and strangers.
Front Psychol, 14:895545, 06 Feb 2023
Cited by: 0 articles | PMID: 36814647 | PMCID: PMC9939515
Cognitive cost of empathizing with mothers and strangers by Chinese college students.
Heliyon, 8(9):e10306, 19 Aug 2022
Cited by: 0 articles | PMID: 36091955 | PMCID: PMC9450072
Do shapes have feelings? Social attribution in children with autism spectrum disorder and attention-deficit/hyperactivity disorder.
Transl Psychiatry, 11(1):493, 25 Sep 2021
Cited by: 6 articles | PMID: 34564704 | PMCID: PMC8464598
Go to all (70) article citations
Similar Articles
To arrive at the top five similar articles we use a word-weighted algorithm to compare words from the Title and Abstract of each citation.
Common and distinct networks for self-referential and social stimulus processing in the human brain.
Brain Struct Funct, 221(7):3475-3485, 13 Sep 2015
Cited by: 24 articles | PMID: 26365506
Neural Activity During Mental Rotation in Deaf Signers: The Influence of Long-Term Sign Language Experience.
Ear Hear, 39(5):1015-1024, 01 Sep 2018
Cited by: 2 articles | PMID: 29298164
Neuronal correlates of theory of mind and empathy: a functional magnetic resonance imaging study in a nonverbal task.
Neuroimage, 29(1):90-98, 24 Aug 2005
Cited by: 552 articles | PMID: 16122944
Executive functions in obsessive-compulsive disorder: An activation likelihood estimate meta-analysis of fMRI studies.
World J Biol Psychiatry, 17(5):378-393, 07 Dec 2015
Cited by: 25 articles | PMID: 26642972
Review
Funding
Funders who supported this work.
NIMH NIH HHS (3)
Grant ID: R01 MH073084-01
Grant ID: R01 MH073084
Grant ID: R25 MH077823