Abstract
Free full text

The effector T cells of diabetic subjects are resistant to regulation via CD4+FOXP3+ Treg
Abstract
Defects in immune regulation have been implicated in the pathogenesis of diabetes in mouse and in man. In vitro assays using autologous regulatory (Treg) and responder (Teff) T cells have shown that suppression is impaired in diabetic subjects(1). In this study we addressed whether the source of this defect is intrinsic to the Treg or Teff compartment of diabetic subjects. We first established that in T1D individuals similar levels of impaired suppression were seen, irrespective of whether natural Treg (nTreg) or adaptive regulatory T cells (aTreg) were present. Then using aTreg we examined the ability of T1D aTreg to suppress Teff of healthy controls, as compared to the ability of control aTreg to suppress Teff of diabetic subjects. Taking this approach we found that the aTregs from T1D subjects function normally in the presence of control Teff, and that the T1D Teff were resistant to suppression in the presence of control aTreg. This escape from regulation was seen with nTreg as well and was not transferred to control Teff co-incubated with T1D Teff. Thus, the “defective regulation” in T1D is predominantly due to the resistance of responding T cells to Treg, and is a characteristic intrinsic to the T1D Teff. This has implications with respect to pathogenic mechanisms which underlie the development of disease and the target of therapies for T1D.
Introduction
A loss of effective immune regulation results in autoimmunity. One mechanism by which regulation is maintained is via CD4+CD25+FOXP3+ regulatory T cells. A complete lack of regulatory T cells (Treg) results in autoimmunity including the development of diabetes in mice with a mutation in Foxp3(scurfy)(2). Depletion of Treg accelerates disease, while adoptive transfer of Treg prevents and cures disease in several murine models of diabetes(3). Yet, in several models of autoimmunity, including diabetes, inflammation and tissue destruction progress despite the presence of functional Treg at the site of inflammation, indicating that a resistance to Treg by T effectors (Teff) may contribute to the development of autoimmunity. These models demonstrate the multiple mechanisms by which immune regulation may be lost in T1D; inadequate numbers of Treg, Treg intrinsic functional defects, or the presence of pathogenic Teff cells resistant to Treg.
In man mutations of FOXP3(IPEX) also result in the development of diabetes(4) However, evidence that a defect in either the number or function of Tregs plays a role in the development of T1D is more limited due to an inability to examine the affected tissue directly. To date studies of Treg number in the peripheral blood of T1D subjects have shown no differences when compared to healthy subjects(5,6) and functional assessment of Treg isolated from the peripheral blood have given variable results(1,5). However, several groups have reported that CD4+CD25+ Treg directly isolated from patients with T1D demonstrate impaired suppression of autologous responder cells (7,8).
In humans the CD4+CD25+FOXP3+ population may be thymically derived or be induced in the periphery. When cells are directly isolated from the blood the source of Treg is not known, so defects in either thymically or peripherally derived regulatory T cells may play a role in T1D. In this paper we examine the function of adaptive Treg (aTreg), generated in vitro from the CD4+CD25- population of control and T1D subjects. We find that while adaptive Treg can be generated from individuals with T1D, they have impaired ability to suppress autologous CD4+ responder T cells. Importantly, we find that this impaired suppression is predominantly due to a resistance of Teff cells from individuals with diabetes to suppression by Treg and not to an intrinsic defect in Treg from diabetic patients. Further, the effector T cells of T1D subjects also display resistance to suppression by Treg directly isolated from the blood (nTreg). A defect intrinsic to an aTreg was identified in only one of 13 individuals with T1D tested. These findings demonstrate that a loss of immune regulation can result from either Treg or Teff intrinsic defects with the resistance of Teff to the suppression of Treg being the dominant mechanism by which pathogenic T cells escape regulation in T1D.
Materials and methods
Subjects
Blood samples from 18 healthy donors, 13 T1D and 3 T2D subjects were obtained from participants in the Benaroya Research Institute Immune Mediated Disease Registry and the BRI JDRF Center for Translational Research. The research protocols were approved by the IRB at Benaroya Research Institute and Children’s Hospital and Regional Medical Center.
Antibodies and reagents
Antibodies for flow cytometric analysis and sorting include: anti-CD4, CD25, and CD69 from BD Pharmingen (San Jose, CA); and FOXP3 (clone: 206D) with its IgG1 control (clone: MOPC-21) purchased from Biolegend (San Diego, CA). Activation was performed with purified anti-CD3 (UCHT1) and anti-CD28 (CD28.2) BD Pharmingen (San Jose, CA); CFSE was purchased from Invitrogen (Carlsbad, CA). IL-2 was purchased from Chiron (Emeryville, CA). Cells were cultured in RPMI 1640 HEPES media containing 10% PHS, Penicillin/Streptomycin and Na-Pyruvate
Induction and Isolation of CD4+CD25+FOXP3+ Treg
PBMC were isolated from human peripheral blood by centrifugation over Ficoll-Hypaque gradients. CD4+ T cells were isolated via negative selection using the CD4 T cell isolation kit (Miltenyi Biotec, Auburn, CA). aTreg were induced from the CD4+CD25− T cells obtained from total CD4+ T cells by negative selection with Miltenyi CD25 microbeads. The positive fraction of the CD4+ no-touch magnetic sort was used as antigen presenting cells (APC) in the induction culture. For induction of aTreg CD4+CD25− T cells were cultured in 24-well plates with irradiated autologous APC (5000 rad) at a ratio of 1:2, activated with soluble anti-CD3 (5 μg/ml) and maintained for 10 days in media. IL-2 was added on day 6, at a concentration of 200 IU/ml. FACS analysis with anti- CD4, CD25, CD69 and FOXP3 was performed on day 9–10 to determine % of CD25+ cells which expressed FOXP3. Regulatory T cells from the induction culture or peripheral blood were isolated by cell sorting of the 5% of T cells with the highest CD25 expression via a FACS Vantage (Becton Dickinson, San Jose, CA) (Supplemental Figure 1).
CFSE-based polyclonal suppression assays
Autologous and/or allogeneic CD4+CD25− T cells were thawed and CFSE- labeled in a PBS staining solution of 0.8 μM at 37°C. 1.5×105 CFSE-labeled responder cells were cultured in round-bottom 96-well plates in duplicate. Varying concentrations of Treg were added as indicated in figure legends and cells were stimulated with Dynabeads(M-280 Tosylactivated, Dynal A.S., Oslo, Norway), which were preincubated with anti-CD3 (5 μg/ml) and anti-CD28 (5 μg/ml) at a ratio of 2:1 (beads:responder cells). Analysis was performed on day 4 by flow cytometry (FACS Calibur): cells were analyzed for proliferation by CFSE dye dilution and expression of CD4, CD25 and FOXP3. Data was acquired using CellQuest Pro software (BD Bioscience, San Jose, CA) and analyzed by FlowJo (Tree Star, Ashland, OR). Percent suppression was determined based on the percentage of dividing CFSE-labeled CD4+CD25− T cells in the co-culture as compared to the percentage of dividing CFSE-labeled CD4+CD25− T cells when cultured alone.
Co-culture suppression assays
0.75×105 CFSE-labeled responder cells were co-cultured with either autologous or allogeneic 0.75×105 unlabeled responder cells in round-bottom 96-well plates in duplicate. Regulatory T cells from peripheral blood were isolated by cell sorting of the 5% of T cells with the highest CD25 expression via a FACS Vantage. Isolated naturally occurring Tregs were cultured with Teff co-cultures at a ratio of 1:4 and were activated with anti-CD3/CD28 coated beads. The percentage of suppression was assessed on day 4 by flow cytometry and analysis was performed as described in the CFSE-based polyclonal suppression assay.
Statistical analysis
Statistical analysis reported in this paper include the Student t-test with the Welch’s correction and an ANOVA using Tukey’s multiple comparison test and linear regression analysis. All curves were based on a non-linear regression analysis performed with one site binding hyperbole with 95% confidence interval. These analysis were performed using PRISM with the exception of linear regression which was performed with “R” (www.r-project.org).
Results
Suppression by adaptive Tregs is impaired in individuals with type 1 diabetes
CD4+CD25+FOXP3+ adaptive Treg can be induced from peripheral blood CD4+CD25- T cells under a variety of conditions in vitro. We have established a method for the induction of adaptive Treg in which the CD4+CD25- population is activated with autologous irradiated APC and soluble anti-CD3 followed by addition of low dose IL-2 on day 6. On day 10 a subset of CD4 T cells which express FOXP3 and have suppressive function can be isolated by sorting the CD25bright population. These cells when co-incubated with CD4+CD25- responder T cells suppress proliferation in a contact dependent, cytokine independent manner (9,10). In order to compare the function of adaptive Treg isolated from diabetic and healthy subjects we utilized a CFSE based suppression assay to measure % inhibition over a range of aTreg: Teff ratios. In these assays we isolated adaptive Treg from the induction cultures on day 10 (see supplemental figure 1), then co-cultured these cells with CFSE labeled CD4+CD25- responder cells activated with beads coated with αCD3/αCD28. Proliferation of the responder cells was measured by FACS on day 4 and the percent inhibition was calculated by dividing the % of responder cells that have undergone cell division in the co-culture by the % that have undergone division when cultured in the absence of Treg(Figure 1a). Using this approach samples from healthy subjects (n=12) were tested over a range of aTreg:Teff ratios. This assay yielded a consistent level of suppression among the control subjects (Figure 1b). This suppression was similar for autologous and allogeneic responder populations (data not shown), and a similar level of suppression was seen when Treg directly isolated from the blood (nTreg) were utilized in this assay (Figure 1b). In all cases the suppression required cell contact (data not shown).
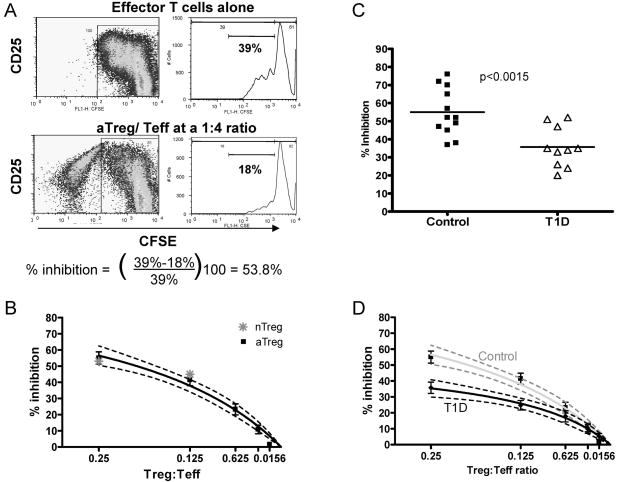
Adaptive Treg suppression is impaired in subjects with T1D. A) CSFE based analysis of suppression: FACS plots represent proliferating CFSE-labeled Teff cells when cultured alone and activated with anti-CD3/anti-CD28 coated beads or co-incubated with Treg. Histograms show the % of proliferating Teff cells cultured alone or after gating out the Treg population. The % of inhibition was determined by comparing the % of proliferating Teff cells cultured alone to the % of proliferating Teff cells in co-culture. B) Suppression assays using aTreg of control subjects and either autologous or allogeneic Teff from controls show a consistent level of suppression.( ■ mean of data from all assays, best fit curve by nonlinear regression with 95% confidence intervals shown) (n=12). Suppression when nTreg are used (
) Representative of n=4. C) Comparison of % inhibition at a ratio of 1:4 aTreg:Teff for control subjects (■) as compared to T1D subjects (
) (n=10) (p= 0.0015). D) % Suppression displayed over a range of aTreg: Teff ratios demonstrates diminished suppression among T1D subjects, data is presented as mean of all assays (
) and as a curve representing non-linear regression and the 95% confidence interval (black line n=10). This is shown in comparison to the control curve in grey.
To assess the function of adaptive Treg in the setting of T1D we induced and isolated aTreg from CD4+CD25- cells of T1D subjects (n = 10). We then tested their ability to suppress autologous CD4+CD25- T cell proliferation in the CFSE assay described above. At a ratio of 1:4, the adaptive Treg from individuals with diabetes were unable to suppress autologous Teff to the same degree as that seen with control aTreg/Teff co-cultures (p<0.0015)(Figure 1c). This decrease in suppression was significant over a range of Treg:Teff ratios (Figure 1d). These findings are consistent with studies performed by others in which case the ability of T1D CD4+CD25bright Treg, directly isolated from the blood, to suppress proliferation of autologous Teff was measured by use of H3-thymidine (7,8).
The difference in % suppression was not linked to an impairment in activation of T1D responder T cells, as the percent of cells proliferating in response to bead stimulation alone was no different for T1D and control subjects (mean = 41% sd=14.6 and mean = 41% sd=3.5 respectively). Previous work from our group has shown a correlation between suppressive function and expression of FOXP3 among aTreg induced and isolated in this manner (10). The percent of FOXP3+ cells among the CD4+CD25bright cells used in these assays were no different between the control and T1D group and ranged from 54% to 85% in both groups. To evaluate whether a decrease in the %FOXP3+ cells contributed to the diminished function of these aTreg, we reanalyzed the suppression data with respect to the ratio of FOXP3+ cells to Teff at the inception of culture and also demonstrated impaired inhibition (data not shown). Thus, the impaired suppression of Teff cells by aTregs from individuals with T1D is not due to a decrease in the total number of FOXP3+ cells in the culture or the level of activation of the Teff population.
T1D aTreg suppress control Teff to the same degree as control aTreg
The impaired suppression found in assays utilizing both Teff and aTreg of T1D subjects implies that there is either an intrinsic defect of the adaptive Tregs or resistance of the Teff population to suppression. To test these possibilities, we co-cultured adaptive Tregs from individuals with T1D with allogeneic Teff from healthy controls and evaluated the resulting % inhibition. Over a range of Treg to Teff ratios, Teff cells from a T1D subject were less well suppressed than those of a healthy control (Figure 2a). This was seen using aTreg from 12 of 13 T1D subjects studied. In contrast, there was no difference in the mean degree of suppression using Teff cells from control subjects whether the source of adaptive Tregs was from controls or T1D subjects (Figure 2b).
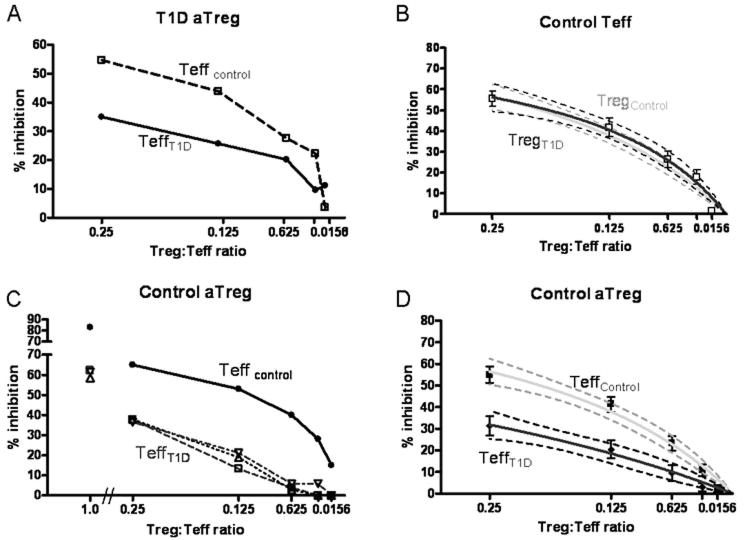
Impaired suppression is intrinsic to the T1D Teff population. A) aTreg isolated from a T1D subject were utilized in a suppression assay with Teff from a control subject (dashed) or T1D subject (solid). B) Combined data from multiple experiments demonstrates that the % inhibition seen when T1D aTreg are co-incubated with control Teff (□) (n= 13), Best fit curve shown as a black line with 95% confidence interval and is no different than that seen with control aTreg (grey line). C) Control aTreg were coincubated with Teff from a control subject (black) or Teff from three different T1D subjects (open symbols with dashed lines) (n=3). D) Combined data for suppression assays in which control aTreg are co-incubated with T1D Teff (n=11) in black with 95% confidence intervals. This is shown in comparison to the control curve in grey.
T1D effector cells are resistant to regulation by aTreg
Based on the above findings we next addressed whether the cause of impaired suppression in these T1D subjects was due to the resistance of T1D effectors to the suppressive function of Treg. To test this possibility we co-cultured adaptive Treg derived from control subjects with Teff from either control or T1D subjects. Figure 2c demonstrates the result from one assay in which aTreg from a control subject were co-incubated with allogeneic responder cells of either a control or T1D subjects. This assay demonstrates that the Teff population derived from T1D subjects were less well suppressed as compared to the allogeneic control Teff. This finding was consistently seen with all of the T1D effector cells tested (n=11) (Figure 2d). Although suppression was enhanced at greater Treg:Teff ratios, suppression to the level of that seen with control subjects was not achieved even at a ratio of 1:1 where the % inhibition reached was 80% and 50% for control and T1D Teff respectively. To further examine the impact of the source of Treg and Teff on % inhibition, a linear regression model was used. When log transformed data was analyzed with respect to the source of Treg, no significant relationship was observed. However, when the analysis was performed with respect to the source of Teff, it clearly demonstrates that the source of Teff in this system is adequate to explain the differences in % inhibition seen (p<0.0001). These findings indicate that the defect in suppression seen in assays using T1D derived cells are due to the resistance of T1D Teff cells to suppression via aTreg.
T1D Teff are resistant to nTreg isolated from healthy and T1D subjects
To determine whether the resistance to suppression of T1D effector T cells was unique to adaptive Treg or extended to natural Treg, we next tested the ability of Treg directly isolated from control subjects (nTreg) to suppress T1D Teff. The nTreg population was isolated from the peripheral blood of control subjects based on level of expression of CD25. We co-incubated these cells with Teff from either control or T1D subjects. At a Treg to Teff ratio of 1:4, we have found that nTreg produce a similar level of suppression as adaptive Treg when using control Teff (Figure 1b and Figure 3). Yet when nTreg were co-incubated with T1D Teff we found that % suppression was significantly lower when compared to control Teff co-incubated with the same nTreg (p<0.001) (Figure 3). This difference was also apparent when the studies were performed at a Treg:Teff ratio of 1:1 and the results of these assays were comparable to assays performed with adaptive Treg (Figure 3). These findings indicate that T1D Teff are refractory to regulation irrespective of the source of CD4+CD25+FOXP3+ Treg.
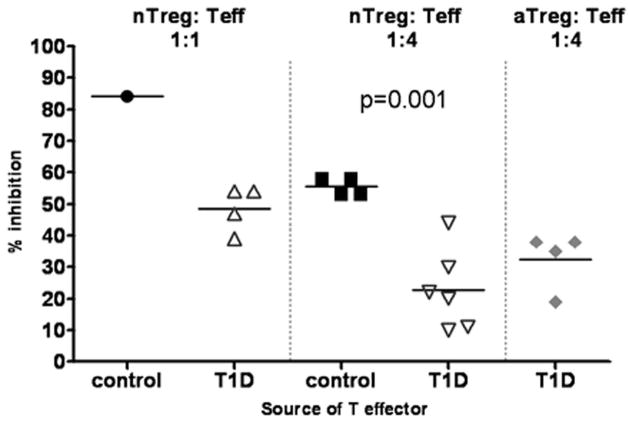
T1D Teff are resistant to nTreg. T1D Teff were co-incubated with nTreg directly isolated from the peripheral blood, %inhibition at a Treg:Teff ratio of 1:1 is shown for, nTreg with control Teff (●) (n=1) and nTreg with T1D Teff () (n=4) at a Treg:Teff ratio of 1:1, nTreg with control Teff (■) (n=4) and nTreg with T1D Teff (
) (n=6) at a ratio of 1:4. For comparison the level of suppression at 1:4 seen with control aTreg co-incubated with the same T1D Teff cells shown in the nTreg assays is shown (
).
The resistance of T1D Teff to suppression is intrinsic to the T1D Teff cells
The identification of a defect in Teff of all of the T1D subjects studied suggests a common mechanism at play. Metabolic factors are one such factor which may influence the responsiveness of Teff in individuals with T1D. The T1D subjects in our study were all under relatively good glucose control with a mean blood glucose of 191 mg/dl sd = 18.2 at the time of the draw, and a mean HgA1C of 6.99, sd= 0.24. To test the impact of mild persistent hyperglycemia on Teff response to Treg, we examined the response of CD4+CD25- Teff cells isolated from individuals with type 2 diabetes (T2D). These subjects had poorer glucose control as compared to the T1D subjects in this study (mean glu=227 mg/dl, mean HgA1C=8.2), yet the Teff of these individuals suppressed well and behaved in a similar manner to control Teff (Figure 4). The composition of the CD4+CD25- population used as responders is another potential reason for differences in the level of suppression seen in these assays, as memory T cells are resistant to regulation and are known to be increased in number in subjects with T1D. However, we found no difference in the % of responder cells with the memory marker CD45RO+ between control and diabetic cells used in this study (data not shown). Further, a contribution to this resistance was not associated with the HLA class II type of the subject as control subjects with the diabetes associated alleles (DR0401 or 0301) demonstrated no resistance to regulation.
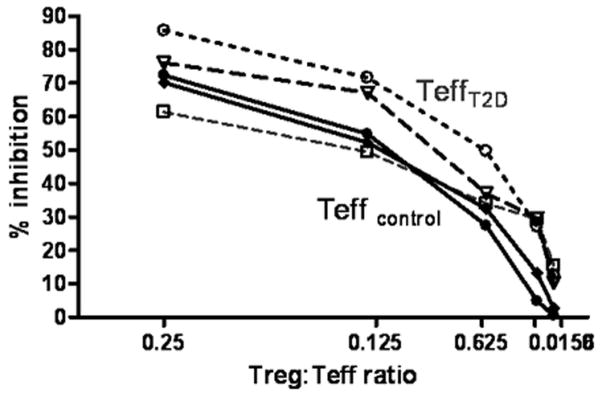
T2D Teff are not resistant to regulation. Control aTreg were co-incubated with allogeneic control Teff or T2D Teff and % inhibition was measured on day 4. Open symbols with dotted lines represent data from T2D Teff, solid symbols with solid lines are data for control Teff. Unpaired t test with Welch’s correction showed no statistically significant differences at a ratio of 1:4, 1:8, 1:16, or at 1:32. At a ratio of 1:64 (p= 0.03).
To further address the mechanisms by which Teff cells from T1D subjects are less sensitive to suppression, Teff from control subjects were co-incubated with those of T1D subjects in the presence of control nTreg. In these assays, either the control or T1D Teff were stained with CFSE so that % inhibition could be examined for each Teff type. Using this approach, we found that the % inhibition of control Teff was not significantly altered in the presence of T1D Teff, while the T1D Teff co-cultured with control Teff, continued to display a decrease in % inhibition similar to that seen when T1D Teff alone are present in the co-culture. The difference in the % inhibition between T1D and control Teff remains significant in these co-cultures (p=0.0034) (Figure 5). These findings indicate that resistance of Teff from T1D subjects is due to a characteristic intrinsic to the Teff and is not due to a soluble factor secreted by Teff nor is it transferable by contact with other Teff or Treg in culture with them.
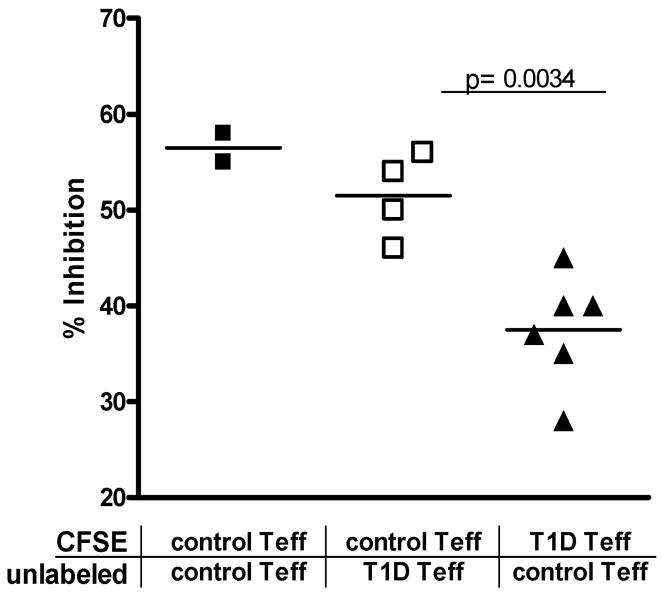
Impairment in T1D Teff suppression is not transferable to control Teff. Suppression assays were performed with control nTreg, co-cultured at a 1:4 ratio with Teff cells. 50% of Teff cells were labeled with CFSE, while the other Teff were unlabeled. Proliferation of CFSE labeled cells was examined on day 4. Data is shown for cultures containing control Teff only, or T1D Teff and Control Teff in co-culture. % inhibition is calculated based on proliferation of Teff in cultures without Treg. The % inhibition of T1D Teff was consistently lower than control Teff from the same culture p=0.0034.
Dual mechanisms of impaired regulation are at play in T1D
Although the majority of T1D subjects examined in this study appear to show a defect in regulation at the level of the Teff, one subject appeared to have a defect in both the adaptive Treg and Teff populations. When this individual’s Teff cells were co-incubated with adaptive Treg of a control subject, suppression was low (20% at a ratio of 1:4) indicating a resistance of the effectors to control aTreg. Further, when the aTreg of this subject were co-incubated with control Teff a lack of suppression was also seen (Figure 6). Together this data indicates that a combined defect in Treg and Teff can be present in T1D. This combined defect could result in a more profound impairment of suppressive function.
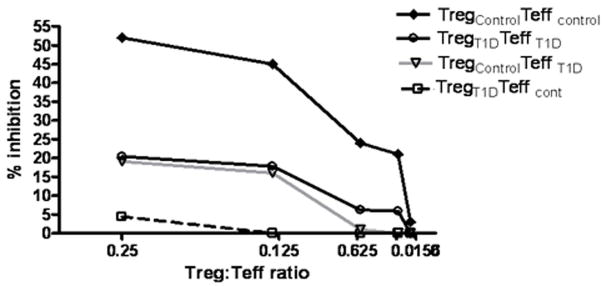
Impaired aTreg and Teff function in one T1D subject. aTreg were induced from a control and diabetic subject. Suppression assays were performed with autologous Teff from the diabetic subject or allogeneic control Teff. % inhibition is shown for T1D Teff with autologous aTreg (○) is severely impaired, suppression is decreased when control Teff or aTreg are used in combination with T1D aTreg (□) or T1D Teff () respectively and does not attain the level seen with the control aTreg plus control Teff (
). This represents 1 of two experiments.
Discussion
Our findings confirm that T cell regulation via the FOXP3+ Treg is impaired in individuals with T1D. However, the defects in regulation are not intrinsic to Tregs but are predominantly the result of Teff cells which are resistant to Treg suppression. These findings are consistent with previous published reports of impaired function of T1D nTreg (7,8) but now localizes the defect in regulation to the T effector population.
The importance of Treg number and function in the development of autoimmunity is well established, including studies in the NOD mouse, which demonstrate the ability to prevent and treat disease by increasing Treg numbers or function(3,11). However several recent studies have demonstrated that pathogenic CD4 T cells can become resistant to Treg in several murine models autoimmunity. In the DO11.10 RIP-mOVA model of diabetes, Clough et al. have shown that the pathogenic T cells found in the draining lymph nodes of the pancreas develop a resistance to Treg suppression (12). In the NOD mouse there is increasing resistance of Teff to Treg with age (13,14) which appear to be due to intrinsic characteristics of the NOD Teff (personal communication Dr. Christophe Benoist). Similar findings have been seen with other models of chronic inflammation including murine models of MS (15) and bone marrow transplant (16). In each of these cases the resistance develops over time and in part offers an explanation for the development of disease despite the presence of Treg in the effected tissue. In addition studies of individuals with active SLE have demonstrated Teff resistance to Treg, extending these findings to human disease (17).
The ability of Teff to evade suppression has been linked to the stage of maturation and lineage commitment of the CD4 T cell population. The mechanisms by which these Teff populations evade suppression have been attributed to multiple factors including the production of cytokines which impede Treg function or changes intrinsic to the Teff. Several different cytokines have been shown to impair Treg function these include: TNF-α (15,18), IL-4 (19), IL-6 (15), IL-12 (20), IL-7, IL-15 (21) and recently, IL-21 has been added to this list (12,22). Cell intrinsic resistance to suppression has been shown to be present in the CD4 memory and Th17 T cells (16). The mechanisms by which these CD4 T cells become resistant to Tregs is unknown, although one study demonstrated that the level of cell surface glycosylation can influence the sensitivity of effector T cells to Treg (23). In the current study we find that T1D Teff do not transfer resistance to regulation to control Teff in co-culture. This indicates that the secretion of a soluble factor is not a predominant mechanism by which suppression is evaded, but suggests that the mechanism is intrinsic to the T1D Teff cells themselves.
In the case of T1D, the resistance of Teff to suppression may be influenced by the factors noted above as well as metabolic and underlying genetic factors. In our studies the resistance to suppression could be seen in the CD4+CD25- T cells taken from the peripheral blood suggesting a global defect in suppression, not one which was site specific, or antigen specific in nature. Chronic hyperglycemia is a characteristic of T1D which could contribute to the difference between T1D and control subjects, yet when a population of T2D subjects was examined there was no evidence of a defect in suppression, indicating that hyperglycemia is not a factor. Expansion of the CD4+ memory T cells pool has been described in T1D (24), and could contribute to the resistance of the Teff population of T1D subjects, however, we found no significant difference in the relative % of CD4+CD45RO+ T cells among the CD4+CD25- Teff cells of the T1D and control samples used in these assays. Among the genes known to be associated with T1D which could also alter Teff responsiveness, are the HLADRB1 0301 and 0401 alleles, and the PTPN22 1858T variant of the protein tyrosine phosphatase Lyp. When we examined the function of Teff derived from healthy subjects who carry these genes we found no resistance to suppression (unpublished data). Our current studies suggest a global defect intrinsic to Teff, and although a unique mechanism may be the source of the Teff resistance, multiple mechanisms may be at play.
In one notable case, we demonstrate that a subject with severe impairment of suppression has not only resistant Teff but also a Treg intrinsic defect. The mechanism by which the Treg function was impaired in this subject is still unclear. Multiple mechanisms by which Treg suppress Teff are possible, these include mechanisms that require cell- cell contact, secretion of inhibitory cytokines such as IL-35, IL-10, TGF-β, the production of other soluble factors such as Ho-1 and adenosine or by a more indirect pathway via the antigen presenting cell. In the assay system used in this paper, no APC are present in the suppression assay, and the suppression is lost if a transwell is used. This implicates a cell-contact dependent mechanism, or one in which secretion of soluble factors is required in close proximity to the Teff.
Taken together, these studies indicate that impaired regulation is present in individuals with T1D. The mechanism by which regulation is impaired may differ among T1D subjects, but our findings indicate that resistance of Teff to Treg may be the predominant mechanism by which regulation is evaded in individuals with diabetes. Further studies will need to be done to identify at what point Teff resistance develops and whether it precedes the onset of disease. The mechanism by which this Teff intrinsic defect occurs warrants further study. Although Treg are not defective in the majority of T1D subjects, these findings still support the logic of devising immunotherapies which target Treg, as increased Treg numbers both in mouse models and in our in vitro assay enhance regulation. However, the prominence of resistant Teff in this process indicates that therapies which also target the Teff should be pursued in T1D. Further, new approaches will require a better understanding of the mechanisms by which T effectors attain resistance to Treg.
Supplementary Material
Supp Fig 1
Supplemental Figure 1:Schematic figure of adaptive Treg generation and isolation.
Acknowledgments
Richard Wang- statistical analysis, K. Arugamanathan and Xiaoping Wu for cell sorting, Megan Tatum for technical assistance, Heather Vendettuoli, Christine Chan for subject recruitment and the Translational Research Program Clinical Core. We thank Dr. Gerald Nepom, Dr. Steven Ziegler and Dr. Christophe Benoist for their critical review of this manuscript.
Funding for this research was provided by the BRI JDRF Center for Translational Research, JDRF Research Award (33-2008-398) to JHB, Dana Foundation Grant, RO1 DK072457 to JHB, Deutsche Forschungsgemeinschaft fellowship (1083/2-1) to AS
Footnotes
2Abbreviations: Type 1 Diabetes (T1D), Adaptive regulatory T cells (aTreg), Regulatory T cell (Treg), Effector T cell (Teff), Naturally occurring regulatory T cell (nTreg)
Disclosures
The authors have no financial conflict of interest.
Reference List
Full text links
Read article at publisher's site: https://doi.org/10.4049/jimmunol.181.10.7350
Read article for free, from open access legal sources, via Unpaywall:
http://www.jimmunol.org/content/181/10/7350.full.pdf
Citations & impact
Impact metrics
Citations of article over time
Alternative metrics
Smart citations by scite.ai
Explore citation contexts and check if this article has been
supported or disputed.
https://scite.ai/reports/10.4049/jimmunol.181.10.7350
Article citations
Regulatory CD4<sup>+</sup> T cells redirected against pathogenic CD8<sup>+</sup> T cells protect NOD mice from development of autoimmune diabetes.
Front Immunol, 15:1463971, 16 Sep 2024
Cited by: 0 articles | PMID: 39351219 | PMCID: PMC11439686
Mechanisms underlying the development of type 1 diabetes in ART-treated people living with HIV: an enigmatic puzzle.
Front Immunol, 15:1470308, 27 Aug 2024
Cited by: 0 articles | PMID: 39257582 | PMCID: PMC11383789
Review Free full text in Europe PMC
Blood immune cell profiling in adults with longstanding type 1 diabetes is associated with macrovascular complications.
Front Immunol, 15:1401542, 01 Jul 2024
Cited by: 1 article | PMID: 39011037 | PMCID: PMC11246869
Defining Human Regulatory T Cells beyond FOXP3: The Need to Combine Phenotype with Function.
Cells, 13(11):941, 30 May 2024
Cited by: 1 article | PMID: 38891073 | PMCID: PMC11172350
Review Free full text in Europe PMC
The immunology of type 1 diabetes.
Nat Rev Immunol, 24(6):435-451, 02 Feb 2024
Cited by: 11 articles | PMID: 38308004
Review
Go to all (210) article citations
Data
Data behind the article
This data has been text mined from the article, or deposited into data resources.
BioStudies: supplemental material and supporting data
Similar Articles
To arrive at the top five similar articles we use a word-weighted algorithm to compare words from the Title and Abstract of each citation.
Attenuated IL-2R signaling in CD4 memory T cells of T1D subjects is intrinsic and dependent on activation state.
Clin Immunol, 181:67-74, 20 Jun 2017
Cited by: 8 articles | PMID: 28645874 | PMCID: PMC5564451
Altered Suppressor Function of Regulatory T Cells in Type 1 Diabetes.
Iran J Immunol, 12(4):240-251, 01 Dec 2015
Cited by: 6 articles | PMID: 26714416
Effector T Cell Resistance to Suppression and STAT3 Signaling during the Development of Human Type 1 Diabetes.
J Immunol, 201(4):1144-1153, 13 Jul 2018
Cited by: 18 articles | PMID: 30006377
Renegade homeostatic cytokine responses in T1D: drivers of regulatory/effector T cell imbalance.
Clin Immunol, 151(2):146-154, 24 Feb 2014
Cited by: 15 articles | PMID: 24576418
Review
Funding
Funders who supported this work.
NIDDK NIH HHS (5)
Grant ID: R01 DK072457
Grant ID: R01 DK072457-03
Grant ID: R01 DK072457-04
Grant ID: R01 DK072457-01A1
Grant ID: R01 DK072457-02