Abstract
Free full text

Specific microbiota direct the differentiation of Th17 cells in the mucosa of the small intestine
SUMMARY
IL-17-producing T-helper cells (Th17) are potent effectors of inflammation, but little is known about the requirements for their differentiation in vivo at steady state. We found that specific commensal microbiota are required for Th17 cell differentiation in the lamina propria (LP) of the small intestine. Differentiation of Th17 cells correlated with presence of Cytophaga-Flavobacter-Bacteroidetes bacteria in the intestine, was independent of TLR, IL-21 or IL-23 signaling, but required appropriate activation of TGF-β. Absence of Th17 cell-inducing bacteria was accompanied by increased Foxp3+ regulatory T cells in the LP. Our results suggest that the composition of the microbiota regulates the Th17:Treg balance in the lamina propria and may thus influence intestinal immunity, tolerance, and susceptibility to inflammatory bowel diseases.
INTRODUCTION
The gastrointestinal tract of mammals is normally colonized with an extraordinarily large number of highly diverse microorganisms termed the commensal microbiota. The commensal microbiota provides multiple benefits for the host. In addition to occupying luminal niches to out-compete pathogenic bacteria, providing a protective biofilm, and participating in the processing of foods and in energy metabolism, it is also actively involved in immune regulation and homeostasis (Backhed et al., 2005; Macpherson and Harris, 2004). Uptake of bacteria by M cells, detection by interdigitating dendritic cells that extend processes in the lumen, and activation of innate immune receptors on epithelial cells are some of the known mechanisms by which signals from the luminal microbiota are transmitted to the immune system (Kelsall and Leon, 2005). It has been known for some time that germ-free animals possess an underdeveloped immune system. Introduction of commensal bacteria leads to increase in CD4+ T cell numbers, induction of secretory IgA (sIgA) and development of organized gut-associated lymphoid tissues (GALT) (Macpherson and Harris, 2004). All of these effects have been ascribed to the presence of commensal bacteria per se. However, little is known as to whether distinct components of the commensal microbiota participate in the induction of particular immune mechanisms.
The composition of the microbiota has been suggested to influence susceptibility to inflammatory bowel diseases (IBD) (Elson et al., 2005; Sartor, 2008), which are mediated by both innate and adaptive arms of the host immune system (Coombes et al., 2005; Rakoff-Nahoum et al., 2004; Strober et al., 2007). It is thus possible that distinct members of the commensal microbiota engage specific components of the immune system and in such a way participate in the regulation of intestinal immune homeostasis (Backhed et al., 2005; Macpherson and Harris, 2004; Rakoff-Nahoum et al., 2004). Whether specific commensal microorganisms regulate the homeostasis of effector T cells in the lamina propria is an important question that is only now beginning to be addressed. For example, it has been reported that the gut commensal, Bacteroides fragilis affects systemic Th1 responses through the action of the bacterial-derived polysaccharide A (PSA) (Mazmanian et al., 2005). The presence of PSA also induced an IL-10-dependent T cell response that protected mice from Helicobacter hepaticus-induced colitis, consistent with the notion that the composition of the intestinal microbiota can influence susceptibility to inflammatory bowel diseases (Mazmanian et al., 2008).
The lamina propria of the small intestine at steady state contains large numbers of two homeostatically regulated and developmentally related populations of CD4 T cells, IL-17+ helper Th17 cells and Foxp3+ regulatory T cells (Treg) (Bettelli et al., 2006; Ivanov et al., 2006; Zhou et al., 2008). Th17 cells produce the cytokines IL-17 (also known as IL-17A), IL-17F, and IL-22. Among these, IL-17 has been the most thoroughly studied and is considered the signature effector cytokine for this subset. Th17 cells have been shown to participate in inflammatory responses and to have critical functions in host defense against bacterial and fungal pathogens, particularly those encountered at mucosal surfaces (Aujla et al., 2008; Aujla et al., 2007; Bettelli et al., 2007; Khader et al., 2007; McKenzie et al., 2006; Ouyang et al., 2008). Th17 cell differentiation in vitro from naïve T cells requires the coordinated action of multiple cytokines, including TGF-β, IL-6, IL-21, and IL-23 (Bettelli et al., 2006; Korn et al., 2007; Langrish et al., 2005; Mangan et al., 2006; Nurieva et al., 2007; Veldhoen et al., 2006; Zhou et al., 2007). These cytokines have also been reported to be required for Th17 cell differentiation in vivo in the context of protective responses to mucosal pathogens or inflammatory reactions (Korn et al., 2007; Mangan et al., 2006; Nurieva et al., 2007). Despite considerable recent progress in understanding of Th17 cell responses, our knowledge of the signals and requirements for Th17 cell differentiation in vivo at steady state in the absence of autoimmune inflammation and of their role in protective immunity remains fragmentary.
Here we show that Th17 cells are induced in the small intestinal lamina propria in response to specific components of the commensal microbiota. Th17 cells appeared in the intestine only after colonization with specific pathogen-free microbiota, and their differentiation was inhibited by treating mice with selective antibiotics. In addition, mice obtained from different commercial vendors had marked differences in the number of Th17 cells in their lamina propria, and animals that lacked Th17 cells acquired them after they were co-housed with Th17 cell-sufficient mice. Differentiation of intestinal Th17 cells was correlated with the presence of members of the Cytophaga-Flavobacter-Bacteroides phylum, but was independent of TLR signaling and of IL-21 and IL-23, cytokines implicated in Th17 cell differentiation and/or function. Reductions in the proportion of Th17 cells were accompanied by increases in Foxp3+ regulatory T cells (Tregs), which share with Th17 cells a requirement for TGF-β. The composition of the intestinal microbiota thus regulates the balance between Th17 and Treg cells in the lamina propria and is likely to influence intestinal immunity, tolerance, and IBD susceptibility.
RESULTS
Th17 Cells Are Specifically Enriched in the Small Intestinal Lamina Propria
CD4+ T cells that express IL-17 (Th17 cells) were previously found to be present in large numbers in the small intestine (SI) lamina propria (LP) (Ivanov et al., 2006). We have expanded our analysis of IL-17-producing cells by examining expression of intracellular IL-17 or GFP in cells obtained from additional tissues of wild type mice and mice heterozygous for insertion of GFP in the locus encoding RORγt, a transcription factor required for Th17 cell differentiation (Eberl et al., 2004; Ivanov et al., 2006). In earlier studies, all IL-17+ T cells in the SI LP were shown to be contained within the cell fraction that expressed RORγt-GFP (Ivanov et al., 2006). Most IL-17+ cells in lung and liver were also GFP+, but not all GFP+ cells expressed IL-17, consistent with what was previously observed in the SI LP (Ivanov et al., 2006) (Figure 1A). In the small and large intestines and in organized gut-associated lymphoid tissues (GALT), 80-90% of the IL-17+ cells were CD4+TCRαβ + (Th17 cells). In contrast, more cell types produced IL-17 in the lung than in the intestine, and only a small proportion of these (less than 10%) were Th17 cells (CD4+TCRβ+αGalCer/CD1d-) (Figure 1B and Suppl Fig 1C, 1D). In the lung, a large proportion of TCRγδ+ T cells expressed IL-17 and RORγt (35 and 65% of γδ T cells, respectively) (Figure 1C, 1D and Suppl Fig 1B). This is consistent with the recent demonstration that a large fraction of antigen-inexperienced CD122lo γδ T cells in lung produce IL-17 upon stimulation (Jensen et al., 2008). However, only a very small proportion of TCRαβ +CD4+ T cells in the lung expressed IL-17 or RORγt at steady state (fewer than 1% in both cases) (Figure 1C and 1D). Additionally, ~15% of the iNKT cells, identified by αGalCer/CD1d tetramer staining, expressed both IL-17 and RORγt(GFP) and were NK1.1- (Figure 1C, 1D and Suppl Fig 1B). Thus, the types of IL-17- and RORγt-expressing cells in the lung and other non-gut-related tissues were markedly different from those in the LP and GALT. It should also be noted that, in our mouse colony, the proportion of CD4+ T cells that expressed IL-17 was markedly lower in the large intestine (LI) and cecum than in the small intestine (2-3% in the LI as compared to 10-15% in the SI) (Ivanov et al., 2006); however, similarly to the SI, 80-90% of the IL-17 expressing cells were CD4+ in these locations (Ivanov et al., 2006 and data not shown). These results suggest that, at steady state, the SI LP provides a unique environment for the differentiation of Th17 cells.
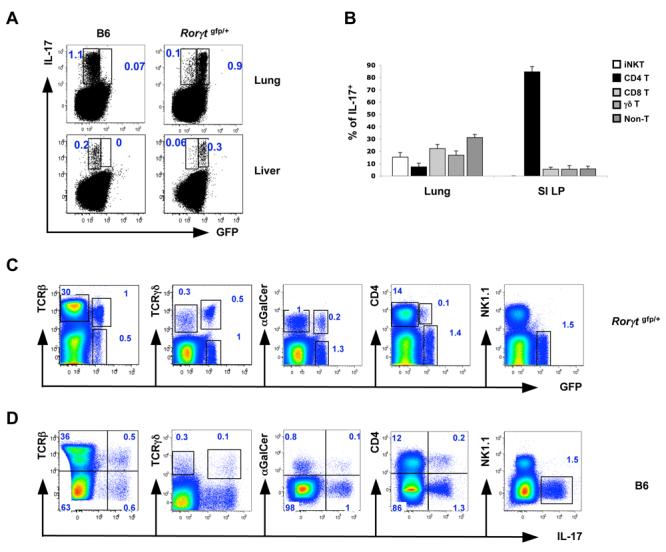
(A) Expression of GFP and IL-17 in total cells from lung and liver of Rorγt gfp/+ and C57BL/6 mice. Data are representative of 3 independent experiments. Lung and liver cells were isolated as described in Methods. All plots were gated on lymphocytes.
(B) Representation of different cell populations among the IL-17+ cell fraction in lungs and small intestines (SI LP) of C57BL/6 mice. Data are combined from three independent experiments. N = 5 mice for lung and N = 8 mice for the SI LP. Error bars represent standard deviation of the mean.
(C, D) Expression of GFP (C) and IL-17 (D) in total cells from lung of Rorγt gfp/+ (C) and C57BL/6 (D) mice. Data are representative of 3 independent experiments. Lung cells were isolated as described in Methods. All plots are gated on lymphocytes.
Role of Cytokine and TLR Signaling in SI LP Th17 Cell Differentiation
Because TGF-β, IL-6, IL-21 and IL-23 are all involved in Th17 cell differentiation in vitro or in the context of inflammatory immune responses (Bettelli et al., 2006; Korn et al., 2007; Langrish et al., 2005; Mangan et al., 2006; Nurieva et al., 2007; Veldhoen et al., 2006; Zhou et al., 2007), we examined their involvement in SI LP Th17 cell differentiation. We previously showed that LP Th17 cell differentiation at steady state requires IL-6 and STAT3 (Ivanov et al., 2006; Zhou et al., 2007). To investigate the role of TGF-β, we examined Tgfb1C33S/C33S knock-in mice (Yoshinaga et al., manuscript in preparation), which have normal production of TGF-β but abnormal TGF-β activation by the extracellular matrix due to prevention of covalent binding to latent TGF-β binding protein 1 (LTBP-1) (see Methods). In this model, conversion of the cysteine at position 33 to serine leads to disruption of the large latent complex (LLC) between the inactive form of TGF-β and the extracellular matrix. This leads to normal production of latent TGF-β, but abnormal TGF-β activation by the extracellular matrix. These mice have a phenotype similar to TGF-β1-deficient mice, but the phenotype is much delayed and less severe and animals survive to adulthood (Yoshinaga et al., manuscript in preparation). Dissociation of TGF-β activation from the extracellular matrix led to a marked reduction in Th17 cells in the SI LP of 6 week old mice (Figure 2A). As in TGF-β1-deficient mice, there was also an increase in IFNγ-producing CD4+ T cells, consistent with a reduction in Treg cells (Suppl Fig 2).
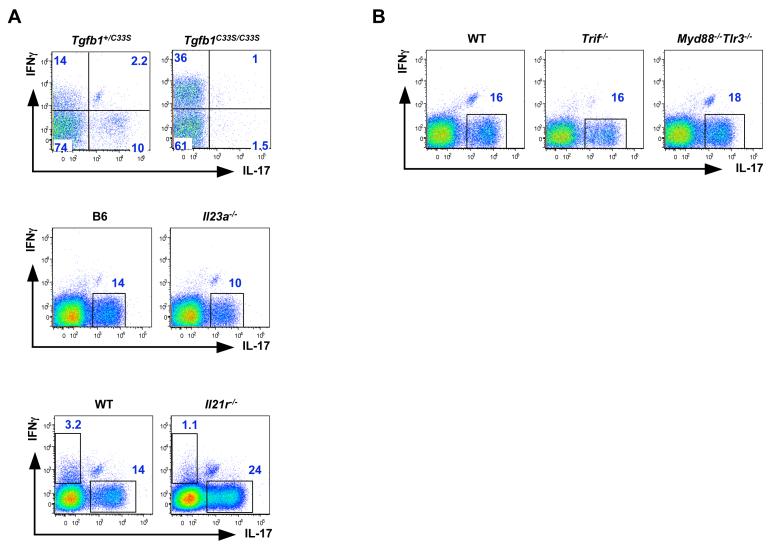
(A) Requirement for TGF-β, but not for IL-21R or IL-23, in SI LP Th17 cell differentiation.
(B) TLR signaling is dispensable for SI LP Th17 cell differentiation.
Lamina propria lymphocytes were isolated from the small intestines of mice with the indicated genotypes as described in the Methods. Immediately after isolation the cells were incubated for 4 hours with PMA/Ionomycin and GolgiPlug (BD). All plots represent individual mice and were gated on TCRβ+CD4+ lymphocytes. For analysis of IL-23 requirement, Il23a-/- mice from Jackson Laboratory were co-housed for 17 days with Taconic B6 mice to equalize the intestinal microbiota. N = 5 (Tgfb1C33S/C33S), 6 (Il21r-/-), 3 (IL23a-/-), 2 (Trif-/-), 2 (Myd88-/-,Tlr3-/-).
IL-21 and IL-23, like IL-6, signal through the induction of STAT3 phosphorylation and, in the presence of TGF-β, induce Th17 and repress Treg cell differentiation (Korn et al., 2007; Nurieva et al., 2007; Yang et al., 2007; Zhou et al., 2007; Zhou et al., 2008). IL-21 was also reported to be required for the differentiation of Th17 ce in adjuvant-induced inflammation, yet Th17 cells were present, and even consistently increased in number, in the SI LP of mice defective for the IL-21 receptor (Figure 2A). Mice deficient for IL-23 (Il23a-/-) also had Th17 cells in the SI LP, consistent with the notion that IL-23 may be required mostly for the maintenance or expansion of this population (Weaver et al., 2006; Weaver et al., 2007). However, there was a decrease in their proportions that was mostly due to the loss of Th17 cells that also expressed IL-22. In addition, TCRγδ T cells expressed very little IL-17 in the absence of IL-23 (Suppl Fig 3). Thus, different cytokines may be required for the differentiation of different subpopulations of Th17 cells.
We also investigated whether signaling downstream of TLRs, which detect microbial products and have been implicated in Th17 cell differentiation (Uematsu et al., 2008; Veldhoen et al., 2006), is required for induction of the Th17 lineage. Both MyD88- and TRIF- deficient mice had normal numbers of Th17 cells in the SI LP. In addition, mice deficient for both MyD88 and TLR3 (which signals by way of TRIF) had Th17 cell numbers similar to wild type controls (Figure 2B and Ivanov et al., 2006). We therefore conclude that TLR-signaling as well as IL-1R signaling, which also requires MyD88, are dispensable for the induction of Th17 cells in the SI LP.
Small Intestine LP Th17 Cells in Ontogeny
We next examined the appearance of LP Th17 cells during ontogeny. Neither GFP(RORγt)-expressing nor IL-17+ CD4+ T cells were detected in the small intestines of 4 day old mice (Figure 3). In comparison, at this age, RORγt+ and IL-17+ γδ T cells were present in adult (6+ week old) proportions (Suppl Fig 4A and 4B). Thus, although IL-17-producing cells are found in the gut of newborn mice, they are not Th17 cells. TCRβ+CD4+IL-17+ (and GFP+) cells did not appear in the SI LP until day 25, and by day 33 they represented most of the IL-17-producing population (Figure 3). The appearance of Th17 cells in the LP correlated with the colonization of the intestines with normal commensal bacteria upon weaning, as indicated by the change in the size of the cecum in the examined mice (Suppl Fig 4C). Proportions and numbers of GFP- and IL-17-expressing γδ T cells did not change significantly during ontogeny (Suppl Fig 4A and data not shown). In addition, at day 4 a significant proportion (~25%) of lymphoid tissue inducer (LTi)-like CD4+CD3- cells, that are found in cryptopatches and isolated lymphoid follicles in gut (Mebius, 2003), also expressed IL-17 (Suppl Fig 4B). Their proportion decreased during ontogeny and in adult 8-week old mice only about 3% of these cells from gut expressed IL-17 (2.9 ± 1.2 %, N=10). Notably, in 8-week old mice a large proportion of the CD4+CD3- LTi-like cells expressed IL-22 (Suppl Fig 4D).
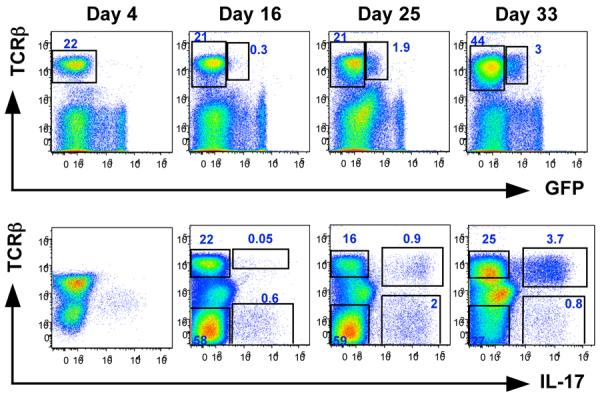
Lamina propria lymphocytes were isolated from the small intestines of Rorγt gfp/+ mice at the indicated ages. For day 4 and day 16 studies, small intestines from 3-4 pups from the same litter were combined in order to obtain sufficient cell numbers for analysis. For day 25 and 33, plots represent individual mice. Top panels — surface staining and RORγt-GFP analysis; bottom panels — intracellular staining for IL-17 after 4 hour stimulation with PMA/Ionomycin in the presence of GolgiPlug (BD). Data are representative of two independent experiments with 2-3 samples/mice in each. Plots are gated on lymphocytes.
LP Th17 Cells Do Not Develop in the Absence of Intestinal Microbiota
Because of the timing of Th17 cell appearance in the newborn gut, we investigated whether intestinal microbiota may have a role in the differentiation of these cells. To this end we examined Th17 cell representation in the small intestines of germ-free mice, which are completely devoid of bacteria and fungi. Germ-free 129S6 and C57BL/6 mice had few, if any, detectable Th17 cells in the LP and the presence of IL-17 mRNA in total RNA from terminal ileum was at the threshold of detection (Figure 4A, 4B and Suppl Fig 5). In addition, germ-free Swiss-Webster (SW) mice from Taconic Farms, examined immediately after receipt, were completely devoid of SI LP Th17 cells (Figure 4A and Suppl Fig 6). Because Th17 and Treg cells are thought to be closely related in their differentiation programs (Bettelli et al., 2006; Zhou et al., 2008), we examined the frequency of Foxp3+ cells in the SI LP of germ-free mice by flow cytometry (Figure 4C and Suppl Fig 7). The proportion of Foxp3+ cells was consistently increased in germ-free mice of all examined strains compared to age-matched conventionally raised specific pathogen-free (SPF) control mice of the same strain, even though the total number of CD4+ T cells was reduced 2-3 fold (Suppl Fig 7 and data not shown). In addition, IL-17-producing γδ T cells were only slightly reduced in the absence of intestinal microbiota (Figure 4D). Thus, the lack of Th17 cells in the absence of bacteria is specific and is not due to a general immune system deficiency.
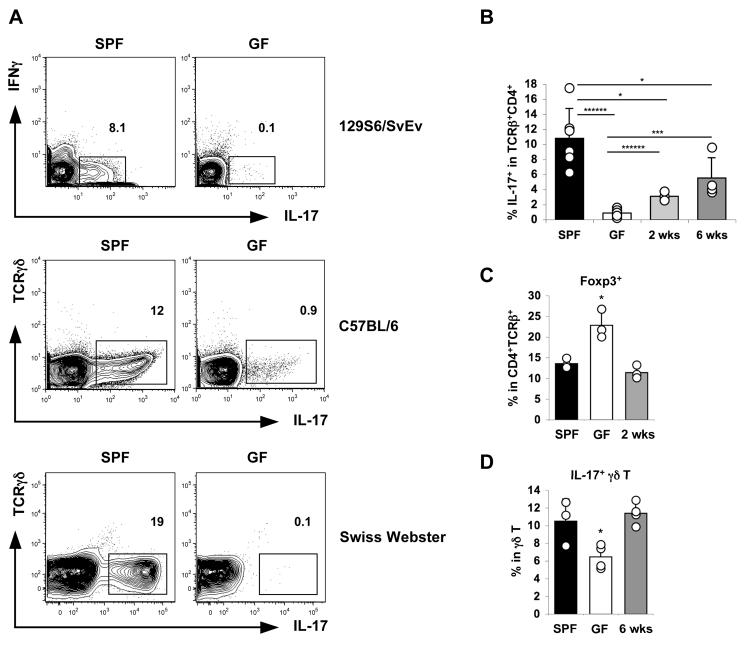
(A) SI LP Th17 cells in 4-month-old 129S6/SvEv, C57BL/6, and 6-week-old Swiss-Webster germ-free (GF) or conventionally raised (SPF) mice. Representative plots shown are samples from individual mice gated on TCRβ+CD4+ cells. Two independent experiments with identical results were performed for each strain and 5-9 mice from each group were analyzed.
(B) SI LP Th17 cells in 6-month-old C57BL/6 SPF, GF, and GF mice reconstituted with fecal slurries from SPF mice for the indicated times. Data are combined from two independent experiments with identical results. A total of 6 SPF, 7 GF, and 7 SPF reconstituted GF mice were analyzed.
(C) Proportion of Foxp3+ cells within the TCRβ+CD4+ SI LP lymphocytes from the mice in (B).
(D) Proportion of IL-17+ cells within the TCRγδ + SI LP lymphocytes from the mice in (B).
Error bars represent standard deviation of the mean. Statistics, unpaired Student t test. *p<0.05, ***p<0.005, ******p < 0.0001. Lines represent the two populations being compared for the statistical analysis.
To directly determine if the scarcity of Th17 cells in the LP was due to the absence of microbiota, we colonized germ-free animals with fecal homogenates from SPF mice. This treatment induced the presence of Th17 cells in the LP as well as IL-17 mRNA in the terminal ileum by 2 weeks after colonization, and a further increase was observed by 6 weeks (Figure 4B and Suppl Fig 5). In addition, both Foxp3+ Treg and IL-17-expressing γδ T cell proportions returned to the values observed in SPF mice (Figure 4C and 4D). Thus, SI LP Th17 cells do not develop in the absence of intestinal microbiota and are induced by the introduction of SPF fecal microbiota into adult germ-free animals.
A Specific Component of the Intestinal Microbiota Is Required for the Development and Maintenance of SI LP Th17 Cells
We next investigated the role of intestinal microbiota in maintenance of Th17 cells in the gut of adult mice by treating the animals with a cocktail of four antibiotics reported to ablate most of the intestinal commensal bacteria (Rakoff-Nahoum et al., 2004). After four weeks of treatment we observed a 50% decrease in the proportion of SI LP Th17 cells within the TCRβ+CD4+ population (Figure 5A and 5C), but not in other IL-17-expressing cells (Suppl Fig 8).
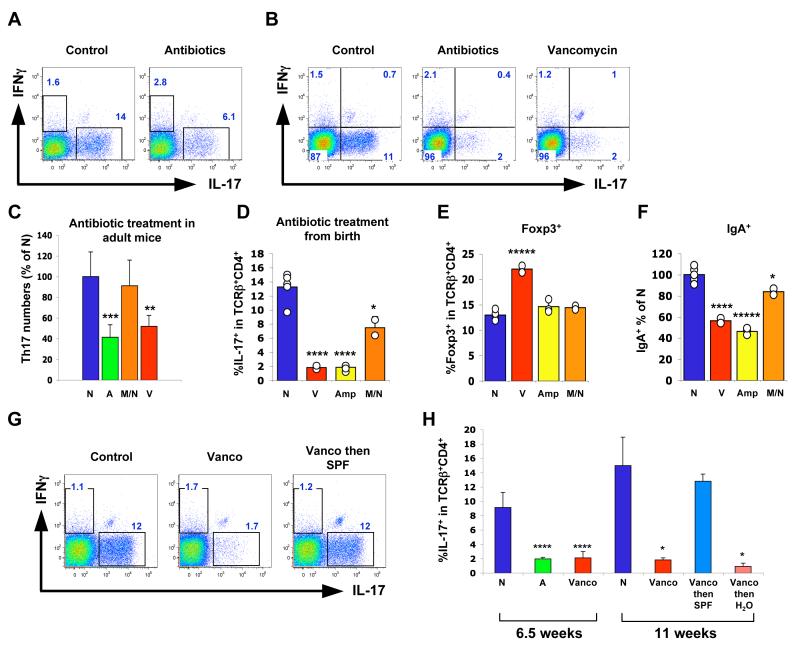
(A, C) Th17 cells in SI LP of 16-week-old C57BL/6 mice treated with antibiotics in the drinking water for 4 weeks. In (A), all four antibiotics were included. In (C), N — no treatment (N=9), A — all four antibiotics (N=6), M/N — metronidazole plus neomycin sulfate (N=9), V — vancomycin only (N=6). 2-3 independent experiments for each condition were performed with similar results. Combined histograms from all experiments (C) as well as representative plots from individual mice gated on TCRβ+CD4+ lymphocytes (A) are shown.
(B, D, E) Lamina propria lymphocyte populations in mice treated with antibiotics in the drinking water from birth. Representative data from individual mice (gated on TCRβ+CD4+ lymphocytes) are shown from one of multiple experiments. Error bars represent standard deviation of the mean. N — no treatment, V — vancomycin only, Amp — Ampicillin only, M/N — metronidazole plus neomycin sulfate.
(F) Relative proportions of IgA+ cells. Data were combined from two experiments. Percentage of IgA+ cells in the lymphocyte gate was calculated for each condition and the data are presented as percentage of the untreated control (N) mean value.
(G, H) Mice were treated with vancomycin (Vanco) or all four antibiotics (A) from birth. At 6.5 weeks of age some mice were transferred into cages with regular water with (Vanco then SPF) or without (Vanco then H2O) co-housing with SPF mice not treated with any antibiotics. Th17 cell numbers in the SI LP were analyzed 4.5 weeks later. Results shown are from one of two independent experiments with similar results. Individual mouse plots (G) were gated on TCRβ+CD4+ lymphocytes. Histogram (H) is from one of two independent experiments with similar results. N = 4 mice for the 6.5 week time point and N = 2 mice for the 11 week time point; error bars represent standard deviation of the mean. Statistics, unpaired Student t test. *p<0.05, ** p<0.01, *** p<0.005, ****p<0.001, *****p<0.0005. For the statistical analysis, in all cases the corresponding population was compared to the WT (untreated) control.
When mice were treated with the antibiotic cocktail from birth, SI LP Th17 cells were decreased by more than 80% by 6-7 weeks of age and the residual Th17 cells had less IL-17 by intracellular staining (Figure 5B and 5D). These results suggest that intestinal bacteria are required for SI LP Th17 cell development, as well as partially for SI LP Th17 cell maintenance in the gut once the cells have been established.
We then asked if treatment with individual antibiotics influences the number of intestinal Th17 cells. Treatment of adult or neonate mice with vancomycin alone, which inhibits predominantly Gram-positive bacteria, as well as some Gram-negative species, led to a similar decrease in Th17 cells to that observed with the cocktail (Figure 5B-D and Suppl Fig 9). In contrast, treatment with a combination of metronidazole, which targets anaerobes, and neomycin sulfate, which targets Gram-negative bacteria, did not significantly decrease SI LP Th17 cell numbers in adult mice and led to only a partial decrease in the proportion of IL-17+CD4+ T cells in mice treated with the antibiotics from birth (Figure 5C and 5D). However, with this treatment we observed a substantially greater depletion of IL-22+ Th17 cells compared to IL-22- Th17 cells (Suppl Fig 10). Ampicillin, which, similarly to vancomycin, inhibits preferentially Gram-positive bacteria, also reduced LP Th17 cell numbers (Figure 5D). Both vancomycin and ampicillin treatment, but not metronidazole/neomycin treatment, also resulted in a two-fold decrease in the number of IgA-producing cells in the LP. There was an almost two-fold increase in the proportion of Foxp3+ cells among the SI LP CD4+ T cells in vancomycin-treated mice (Figure 5E and 5F).
To determine if a transmissible component of the microbiota could influence restoration of Th17 cells following antibiotic treatment, newborn mice were exposed to vancomycin for 6.5 weeks and were then transferred to cages alone or with untreated control mice for an additional 4.5 weeks. Mice kept on vancomycin for the entire period contained very few SI LP Th17 cells compared to controls kept on regular water. SI LP Th17 cell numbers and IL-17 mRNA levels in terminal ileum were recovered completely only in mice co-housed with untreated controls (Figures 5G, 5H and Suppl Fig 9). Indeed, mice taken off antibiotics but not co-housed with untreated mice failed to recover SI LP Th17 cell numbers even after 6.5 weeks (Figure 5H and data not shown). These results demonstrate that a vancomycin (and ampicillin)-sensitive component of the commensal bacteria, but not all bacteria, mediates the induction of Th17 cells in the small intestine.
Differences in the Commensal Microbiota Influence Differentiation of SI LP Th17 Cells
Our results with antibiotic treatment suggested that specific components of intestinal microbiota induce Th17 cell differentiation. Therefore we examined mice obtained from different SPF facilities to determine if they differ in number of Th17 cells in the SI LP. C57BL/6 mice obtained from the SPF facilities of several academic medical centers and commercial vendors had similar proportions of SI LP Th17 cells, typically 10-15% of total CD4+ T cells. In contrast, C57BL/6 mice obtained from the Jackson Laboratory consistently had only 1-2% SI LP Th17 cells (Figure 6A).
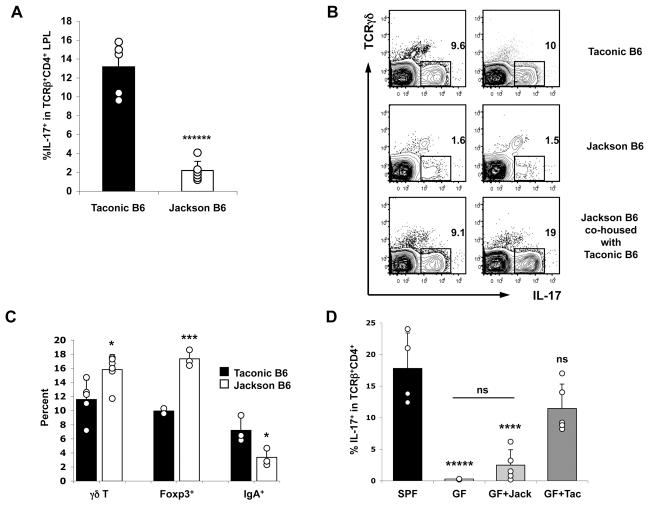
(A, C) LPL populations in 10-week-old C57BL/6 mice from Taconic Farms (black bars) or The Jackson Laboratory (white bars). Data are combined from two out of three experiments with similar results. Error bars represent standard deviation from the mean. In (C) γδ T refers to percentage of IL-17+ cells in the TCRγδ + lymphocyte gate; Foxp3 refers to percentage of Foxp3+ cells within the TCRβ+CD4+ lymphocyte gate; and IgA+ refers to percentage of IgA+ cells within the lymphocyte gate.
(B) Jackson B6 mice were co-housed with Taconic B6 mice for 2 weeks before analyzing SI LP Th17 cells. Data are representative of two experiments with identical results (total of 4-6 mice in each group). Individual plots represent individual mice (2 mice of each group are shown) and were gated on TCRβ+CD4+ lymphocytes. (D) 4 week-old Swiss-Webster germ-free (GF) mice were colonized with homogenized cecal content from Jackson B6 (GF+Jack) or Taconic B6 (GF+Tac) mice and SI LP Th17 cells were analyzed on days 10 and 12 after colonization (results combined from both days). All GF mice were littermates. SPF, age and sex-matched controls raised under conventional conditions. N = 4 (SPF, GF) and 5 (GF+Jack, GF+Tac). Statistics, unpaired Student t test. *p<0.05, ***p<0.005, ****p<0.001, *****p<0.0005, ****** p < 0.0001, ns, not statistically significant (p>0.05). For statistics on (D), p values refer to comparisons to the SPF group, except on the horizontal line, which compares GF+Jack to the GF group.
To address the possibility that the lack of SI LP Th17 cells may be due to a genetic difference between C57BL/6 mice from the Jackson Laboratory and those in other facilities, we co-housed Jackson mice with mice obtained from Taconic Farms, thus allowing for horizontal transfer of intestinal bacteria between the two strains. SI LP Th17 cell numbers in Jackson mice “recovered” after only 2 weeks of co-housing with Taconic B6 animals (Figure 6B). In contrast, Jackson mice that were kept in separate cages did not acquire SI LP Th17 cells (Figure 6B). Jackson B6 mice also had almost a two-fold increase in the proportion of Foxp3+ cells and fewer IgA-producing cells compared to those from Taconic Farms (Figure 6C). Both of these trends were similar to those observed with the other “Th17 cell-deficient” models (germ-free and antibiotic-treated mice). These results further strengthen the conclusion that a specific component of the intestinal microbiota, rather than simply the presence of bacteria, is required for induction of SI LP Th17 cell differentiation.
To directly demonstrate the existence of a Th17 cell-inducing component of the microbiota in mice from different commercial sources, we colonized 4-week-old Swiss-Webster germ-free mice (Taconic Farms) that lack Th17 cells (Suppl Fig 6) with cecal contents from Jackson B6 or Taconic B6 mice by oral gavage. 10 and 12 days later the presence of SI LP Th17 cells in the colonized mice was compared to that of littermate germ-free mice and to age- and sex-matched mice raised under conventional conditions (SPF) (Figure 6D). All animals were successfully colonized as demonstrated by the presence of intestinal bacteria in the gut, which was monitored by changes in cecum size and Gram staining (Suppl Fig 11 and data not shown). Introduction of microbiota from Taconic B6 mice led to recovery of normal proportions of Th17 cells at day 10 after colonization. In contrast, mice colonized with microbiota from Jackson B6 mice had few SI LP Th17 cells, although there was some increase compared to non-colonized GF mice housed under the same conditions (Figure 6D).
Comparison of the Composition of the Intestinal Microbiota between Th17 Cell-Sufficient and Th17 Cell-Deficient Mice
In an attempt to identify Th17-cell inducing organisms, we colonized germ-free mice with several defined intestinal bacterial species. However, germ-free mice colonized with Enterococcus faecalis or with the defined bacterial “cocktail” altered Schaedler’s flora (ASF) (Dewhirst et al., 1999) lacked Th17 cells and similarly to germ-free mice had increased proportions of Foxp3+ cells and reduced proportion of IgA+ cells (Suppl Fig 12 and data not shown).
More than 90% of the commensal intestinal bacteria in both mice and humans fall into two major classes — Gram-negative members of the Cytophaga-Flavobacteria-Bacteroides (CFB) phylum and Gram-positive bacteria of the phylum Firmicutes (Ley et al., 2008; Lupp et al., 2007). Gram-staining of cecal material revealed a large diversity of Gram-positive and Gram-negative commensal bacteria in control Taconic B6 animals (Figure 7A). After vancomycin treatment, which eliminates Th17 cells, only Gram-positive cocci and diplococci and Gram-negative E. coli-like γ-proteobacteria were discernible. In contrast to the decreased morphological diversity observed after vancomycin treatment, the microbiota in Jackson B6 mice, which also lack Th17 cells, was still morphologically highly diverse (Figure 7A) supporting our finding that only a specific component of the microbiota and not simply the presence of bacteria is required for Th17 cell induction. Similarly, SYBR green staining showed the loss of diverse filamentous bacteria after vancomycin treatment (Figure 7B). This diversity was recovered after introduction of SPF microbiota from Taconic B6 mice, which have abundant Th17 cells. However, the reduction in Th17 cells was not due to the loss of the filamentous bacteria as a class because they were present in Jackson B6 mice (Figure 7B).
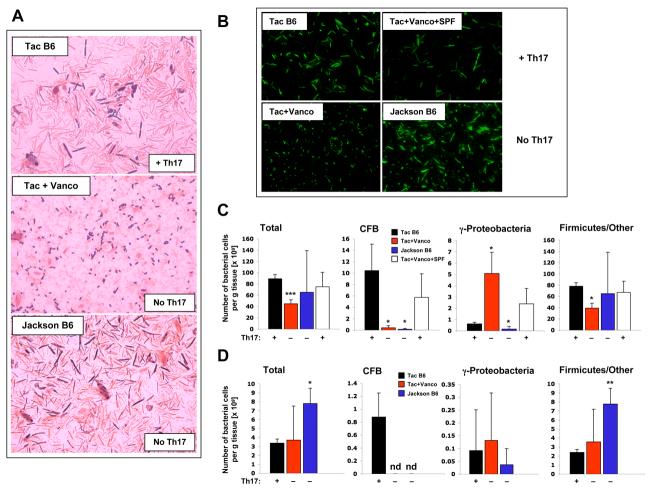
(A) Gram staining of undiluted cecal material from 8-week-old Taconic B6 mice, Jackson B6 mice, and Taconic B6 mice treated with vancomycin (Vanco) from birth.
(B) SYBR green staining of cecal bacteria after dilution and filtration of the solid matter in Taconic B6, Jackson B6, vancomycin-treated from birth, and vancomycin-treated mice exposed subsequently to SPF mice to recover the SI LP Th17 cells.
(C) FISH analysis of intestinal bacteria from ceca of Taconic B6 (Tac B6) mice, mice treated from birth with vancomycin (Tac+Vanco), vancomycin-treated mice subsequently co-housed with non-treated mice (Tac+Vanco+SPF), and Jackson B6 (Jackson B6) mice, performed as described in the Methods.
(D) FISH analysis of intestinal bacteria from terminal ileum of Taconic B6 (Tac B6), mice treated from birth with vancomycin (Tac+Vanco), and Jackson B6 (Jackson B6) mice, performed as described in the Methods. For (C) and (D) data are presented as number of bacteria per gram tissue. Error bars represent standard deviation of the mean. 3 samples for each group were analyzed. Statistics, unpaired Student t test. p-values were calculated in comparison to the control Taconic B6 mice value. *p<0.05, ** p<0.01, *** p<0.005, nd — not detected.
Vancomycin is a glycopeptide antibiotic that in vitro inhibits proper cell wall synthesis preferentially in Gram-positive bacteria. However, in the gut, vancomycin treatment led to a loss of both Gram-positive and Gram-negative species (Figure 7). Indeed, the bacterial communities in the intestine exist in a very well balanced equilibrium and thus the elimination of one group of microorganisms may lead to the loss of a number of other species that depend on this group for their survival. Hence treatment, even with antibiotics with a narrow specificity, would be expected to reduce much more than just the antibiotic-sensitive species. It is thus difficult to predict what organisms are involved in Th17 cell differentiation purely based on their antibiotic sensitivity in vivo.
To examine in more detail the composition of the microbiota, we performed fluorescent in situ hybridization (FISH) of commensal bacteria isolated from ceca of Th17 cell-sufficient mice (Taconic B6 mice and mice treated first with vancomycin and then exposed to normal SPF bacteria) and Th17 cell-deficient mice (Jackson B6 mice and mice treated with vancomycin from birth) using probes specific for CFB or γ-proteobacteria (a phylum that represents a very minor component of the conventional microbiota, but are known to expand during intestinal inflammation (Lupp et al., 2007)) combined with an eubacterial probe that identifies all bacterial cells. Firmicute numbers were calculated after subtracting the numbers of CFB and γ-proteobacteria positive cells from the total eubacteria probe positive cells (Methods and Lupp et al., 2007). Notably, in all groups the presence of Th17 cells in the SI LP correlated with the presence of large numbers of the CFB phylum, thus directly implicating this group in Th17 cell differentiation (Figure 7C). To ask if similar changes in the microbiota composition are also present in the small intestine, we performed the FISH analysis on bacteria isolated from the terminal ileum of Taconic B6, Jackson B6, and mice treated with vancomycin from birth. Similarly to the cecum, CFB members were not present in the Th17 cell-deficient Jackson B6 and vancomycin-treated animals (Figure 7D).
DISCUSSION
The biology of Th17 cells has elicited considerable excitement in recent years due to the recognition that these cells are involved in a wide variety of inflammatory conditions and autoimmune diseases. There has been extensive investigation of the cytokine requirements and genetic regulation in Th17 cell differentiation both under well-defined conditions in vitro and in the context of inflammatory stimuli in vivo (Bettelli et al., 2007; Ivanov et al., 2007; Stockinger and Veldhoen, 2007). The role of Th17 cells in normal physiology and the requirements for their differentiation at sites where they are most prevalent has received comparatively little attention. We previously reported that Th17 cells are abundant in the small intestinal lamina propria at steady state (Ivanov et al., 2006). In this report, we have shown that the small intestine provides an environment that uniquely favors the differentiation of Th17 cells, which are scarce in other organs and at other mucosal sites. The composition of the commensal intestinal bacteria was found to have a crucial role in the differentiation of SI LP Th17 cells and in their balance with Treg cells, which also make up a large proportion of CD4+ T cells in the intestinal mucosa. Our results indicate that only a subset of bacterial species can induce Th17 cell differentiation, and suggest that unique innate immune signaling pathways, distinct from the TLR-mediated signals that can be initiated by numerous microorganisms, are required for this process.
Commensal bacteria have been shown to have a critical role in intestinal epithelial cell repair and homeostasis, acting through TLR-dependent mechanisms (Rakoff-Nahoum et al., 2004). It is not clear how different members of the microbiota specifically affect different branches of innate or acquired mucosal immunity. However, it has been reported that certain bacterial species are more potent immune stimulators than others (Talham et al., 1999); (Umesaki et al., 1999). In support of this notion, recent studies have identified Bacteroides fragilis as capable of inducing Th1 systemic immunity as well as IL-10 producing regulatory T cells in the colonic lamina propria (Mazmanian et al., 2005; Mazmanian et al., 2008). We found that simply the presence of commensal bacteria, even with a diverse composition, as in C57BL/6 mice from the Jackson Laboratories, is not sufficient to induce Th17 cells in the lamina propria. Instead, specific vancomycin-sensitive species in the commensal microbiota appear to be required. In our experiments, the presence of Th17 cells in the mucosa correlated with the presence of members of the CFB phylum, implicating these bacteria as Th17 cell inducers. In contrast, the abundance of γ-Proteobacteria and Firmicutes did not correlate with presence of Th17 cells. γ-Proteobacteria were present in high numbers in the cecum only after vancomycin treatment, but were scarce in Jackson B6 mice (Figure 7C) and total numbers of Firmicutes were actually increased in the ileum of Th17 cell-deficient Jackson B6 mice (Figure 7D). However, the species composition of the Firmicutes phylum was not investigated in this study and thus we cannot rule out the involvement of individual species from this phylum. The exact nature of components of the microbiota that induce Th17 cells awaits a more detailed species-specific comparison of the microbiome of Th17 cell-sufficient and Th17 cell-deficient mice.
Induction of SI LP Th17 cells by specific microbiota is likely to involve activation of distinct innate signaling pathways in epithelial cells or different subsets of antigen presenting cells in the lamina propria (Denning et al., 2007; Fujiwara et al., 2008; He et al., 2007; Mucida et al., 2007; Uematsu et al., 2008). Our results with TLR signaling-deficient mice rule out an absolute requirement for this pathway in Th17 cell induction. Instead, combined with the results with mice from different sources, they suggest that activation of other microbial pattern recognition pathways or production of a metabolite by subsets of commensal organisms in the gut direct the differentiation of IL-17 producing T-helper cells. While this paper was under review, another study reported the absence of Th17 cells in large intestine of germ-free mice and showed that TLR signaling is not required for their accumulation in the lamina propria (Atarashi et al., 2008). That study showed that luminal ATP can drive the differentiation of colonic Th17 cells, most likely by activating a specific population of intestinal DCs (Atarashi et al., 2008). Combined, the two studies suggest that similar signals from the intestinal microbiota drive Th17 cell differentiation in both small and large intestine. Our study demonstrates that the presence of even very diverse populations of intestinal bacteria is not sufficient to induce Th17 cell differentiation, but that a specific subset of the intestinal microbiota is required. Although ATP can clearly induce Th17 cell differentiation as demonstrated by Atarashi et al., it is unclear at this point if this is the main mechanism of Th17 cell induction in vivo and whether it involves bacterial-derived ATP. It is formally possible that Th17 cell-inducing bacteria represent a small intestinal subpopulation that specifically produces ATP. On the other hand, ATP may be produced by many types of bacteria, in which case there may be bacterial species-specific sequestration of ATP accessibility to dendritic cells or engagement of ATP-independent mechanisms for induction of Th17 cell differentiation in the intestine.
An unexpected finding was that neither the IL-21 nor the IL-23 ligand-receptor interaction was required for the differentiation of Th17 cells in the SI LP. We and others showed that IL-21 is induced by IL-6 in vitro in naïve CD4+ T cells and contributes significantly towards production of IL-17 in response to the combination of IL-6 and TGF-β(Korn et al., 2007; Nurieva et al., 2007; Zhou et al., 2007). Normal or even enhanced differentiation of mucosal Th17 cells in IL-21R-deficient mice suggests that cytokines other than IL-21 can mediate the inductive effect of IL-6 in vivo in the intestinal lamina propria. Consistent with our data, two recent studies have demonstrated that IL-21 is dispensable for Th17 cell differentiation in vivo in a model of autoimmunity (Coquet et al., 2008; Sonderegger et al., 2008). In contrast to studies on IL-21, there is a consensus that IL-23 is required in vivo for Th17 cells to mediate inflammatory and protective immune responses (McKenzie et al., 2006; Weaver et al., 2007). In vitro, IL-23 can enhance production of IL-17, particularly at low TGF-β concentrations (Zhou et al., 2008). It is therefore difficult to explain why Th17 cells were present in the lamina propria of Il23a-/- mice. We noted that lack of IL-23 selectively depleted IL-22-producing Th17 cells in the SI LP at steady state. Thus, IL-23 may selectively influence the differentiation of distinct Th17 cell subpopulations. Mice lacking IL-23 have been shown to succumb to intestinal infection with Citrobacter rodentium even though there was little effect on IL-17 levels in the colon (Mangan et al., 2006). Absence of IL-22 results in similarly increased susceptibility to C. rodentium infection, and the IL-22 response was shown to require IL-23 and to be independent of adaptive immunity (Zheng et al., 2008). Therefore, IL-22 may execute an innate effector function that has been appropriated by Th17 cells, and its expression may be more dependent than that of IL-17 on IL-23R signaling.
We also show in this report that appropriate activation of TGF-β by the extracellular matrix is necessary for steady-state Th17 cell differentiation. TGF-β, depending on its concentration and the presence of other cytokines, controls the balance between Foxp3+ Treg and Th17 cell differentiation in vitro (Bettelli et al., 2006; Zhou et al., 2008). Of note, the mutation that we have generated in TGF-β leads to abnormal targeting of TGF-β to the extracellular matrix. It does not decrease the amount of TGF-β precursor, nor does it decrease the level of activated TGF-β in the serum (data not shown). In addition, all mechanisms of TGF-β activation are still in place. Thus, this mutation may lead to activation of TGF-β at a different location or by different mechanisms, which may result in changes in the local concentration of active TGF-β, and thus affect Th17 as well as Foxp3+ Treg cell production. Accordingly, Th17 cells were not present in the lamina propria in these mice. Another study recently reported that lack of αvβ8, one of the major TGF-β activators, on DCs led to loss of Foxp3+ Tregs in the lamina propria (Travis et al., 2007). However Th17 cell differentiation was not investigated in that study. The mechanism of αvβ8 activation is not expected to be affected in our model. Nevertheless we also found that Foxp3+ cell proportions in the lamina propria were greatly reduced in our mice. Combined, these data suggest that the concentration of TGF-β established by the appropriate activation mechanism at the appropriate location is crucial for Th17 and Foxp3+ Treg cell differentiation in the lamina propria.
Our findings demonstrate the importance of the composition of the intestinal bacteria in regulating the balance and homeostasis of different helper T cell populations in the lamina propria and further emphasize the critical role that the microbiota play in the development of the immune system. In addition to controlling the Th17:Treg balance, the composition of the commensal microbiota may also have a role in regulating levels of different Th17 cell subpopulations (IL-22+, IL-17F+, IL-10+, and IFNγ +) whose functions in disease and host defense have yet to be defined. The representation of different effector T cell populations generated by a particular commensal repertoire will dictate the nature and robustness of the immune responses in the intestine. Elucidation of intestinal microbial species and products that influence the differentiation of the various effector CD4+ T cell subsets promises to provide a better understanding of how the intestinal host-microbial relationship regulates host defense and inflammation.
EXPERIMENTAL PROCEDURES
Mice
C57BL/6 mice were obtained from Taconic Farms, Jackson Laboratory, or Charles River and housed under SPF conditions in isolator cages. Rorγt gfp/+ mice were described previously (Eberl et al., 2004). Il23a-/- mice on the C57BL/6 background were kindly provided by Dan Cua, Schering-Plough Biofarma, and were obtained from the Jackson Laboratory. Il21r-/- mice on the C57BL/6 background were a kind gift from Warren Leonard, NIH. Trif-/- mice on the C57BL/6 background were obtained from the Jackson laboratory. Myd88-/-/Tlr3-/- mice on C57BL/6 background were a kind gift from Thomas Moran, Mount Sinai. Mice with a knock-in mutation of Cysteine 33 to Serine in the LAP portion of the TGF-β gene were generated in the laboratory of Dan Rifkin, NYU and were on a mixed C57BL/6/129 background (Yoshinaga et al., manuscript in preparation). Swiss-Webster SPF, germ-free, or altered Schaedler’s flora (ASF) associated mice were obtained from Taconic Farms. Germ-free mice on the C57BL/6 and 129S6 background and mice monocolonized with Enterococcus faecalis were housed at the National Gnotobiotic Rodent Resource Center and Gnotobiotic Animal Core, Center for Gastrointestinal Biology and Disease at the University of North Carolina at Chapel Hill.
Co-housing and Microbiota Reconstitution
For co-housing experiments mice were transferred into an isolator cage with the corresponding line for the indicated time. All mice were housed at the Skirball Institute SPF facility in regular isolator cages. Jackson mice were housed in isolator cages in the same room and on the same diet for at least 4 weeks without change in SI LP Th17 cell numbers. For the microbiota reconstitution experiments, two approaches were used: Germ-free C57BL/6 mice were transferred out of the germ-free isolators, housed in sterile microisolator cages for 2 days and then conventionalized by swabbing their mouths with homogenized fecal pellets from SPF Charles River C57BL/6 mice and by placing bedding and feces from the SPF mice into the cages. Alternatively, germ-free Taconic Swiss-Webster mice were transferred out of the germ-free container into autoclaved sterile microisolator cages and gavaged with 200 ul of cecal bacteria obtained by homogenizing the full contents of two cecums from the corresponding donor mice in 50 ml water (the remaining homogenate was distributed on the bedding and food in the cage).
Cell Isolation and Flow Cytometry
Lamina propria lymphocytes (LPL) were isolated as described before (Ivanov et al., 2006). For LPL isolation from 4-,8-, or 16- day old mice intestines from 2-5 pups were pooled and subjected to the same protocol. Lung and liver lymphocytes were isolated using the same protocol, but without EDTA treatment. For intracellular cytokine staining, immediately after isolation, the cells were incubated for 4 hours with 50 ng/ml PMA (Sigma), 750 ng/ml Ionomycin (Sigma) and 10 μg/ml GolgiPlug (BD) in a tissue culture incubator at 37°C. Surface and intracellular cytokine staining was performed as described before (Ivanov et al., 2006). Flow cytometry was performed on LSR II (BD Biosciences) or CyAn ADP (Becton-Coulter (Dako)). All antibodies were purchased from eBioscences or BD Pharmingen. Anti-mouse IL-22 monoclonal antibody was a kind gift from Jean-Christophe Renauld, Universite Catholique de Louvain and was conjugated to Alexa-647.
Antibiotic Treatment
For ablation of intestinal bacteria, an antibiotic cocktail of 1 g/L each of Ampicillin (sodium salt, SIGMA), Neomycin sulfate (Fisher), Metronidazole (Fisher) and 0.5 g/L Vancomycin hydrochloride (Fisher) was used as previously described (Rakoff-Nahoum et al., 2004). Individual antibiotics or combinations of two antibiotics were also used. Antibiotics were added into the drinking water on a weekly basis. For treatment from birth, mice were raised in cages in which breeding pairs were set and kept on the indicated antibiotic. Control cages were kept on regular water. Antibiotic activity was gauged by changes in cecum size due to bacterial death, as well as by Gram-staining of cecal material that demonstrated depletion of different bacterial groups with different treatments in all cases.
Bacterial Analysis
For Gram staining, undiluted or 10-fold diluted fecal material from the indicated organ was mounted on a glass slide, heat-fixed and stained consecutively for 30-60 sec with crystal violet, Gram’s iodine, isopropyl/methyl alcohol, and safranin.
For FISH and SYBR green DNA staining, distal half of small intestines as well as ceca and colons were collected and the whole organ homogenized with Mixer Mill. A 1:10 dilution of each organ homogenate was stored in 3.7% formalin at 4 °C until use. SYBR green DNA staining and FISH hybridization were performed as described before (Lupp et al., 2007). The percent composition of CFB and γ-Proteobacteria phyla was determined by dividing the numbers obtained for the specific probe to all Eubacteria (EUB338 probe). The percent composition of “Firmicutes/others” group was determined by subtracting the result obtained for CFB and γ-Proteobacteria from 100%.
Acknowledgements
We thank Maureen Bower for her help with the gnotobiotic experiments and Bo Liu for assistance with the colonization experiments. We also thank Edgardo Sanabria-Valentin for help with the microbiology and Roger Orcutt for advice and discussions. I.I.I., R.L.F., N.M., and K.Y. were supported by fellowships from the Crohn’s and Colitis Foundation of America, the Howard Hughes Medical Institute, the Cancer Research Institute, and the Uehara Foundation, respectively. The work was supported by the Howard Hughes Medical Institute (D.R.L.), the Helen and Martin Kimmel Center for Biology and Medicine (D.R.L.) and by grants from the Sandler Program for Asthma Research (D.R.L.), the National Gnotobiotic Rodent Resource Center (P40RR018603 and P30DK34987) (R.B.S.), the National Institutes of Health (CA034282 to D.B.R. and RO1 DK53347 to R.B.S., RO1 AI033856 to D.R.L.), Phillip Morris Foundation (D.B.R.), and the Canadian Institutes of Health Research (B.B.F).
Footnotes
Publisher's Disclaimer: This is a PDF file of an unedited manuscript that has been accepted for publication. As a service to our customers we are providing this early version of the manuscript. The manuscript will undergo copyediting, typesetting, and review of the resulting proof before it is published in its final citable form. Please note that during the production process errors may be discovered which could affect the content, and all legal disclaimers that apply to the journal pertain.
REFERENCES
- Atarashi K, Nishimura J, Shima T, Umesaki Y, Yamamoto M, Onoue M, Yagita H, Ishii N, Evans R, Honda K, et al. ATP drives lamina propria T(H)17 cell differentiation. Nature. 2008 [Abstract] [Google Scholar]
- Aujla SJ, Chan YR, Zheng M, Fei M, Askew DJ, Pociask DA, Reinhart TA, McAllister F, Edeal J, Gaus K, et al. IL-22 mediates mucosal host defense against Gram-negative bacterial pneumonia. Nat Med. 2008;14:275–281. [Europe PMC free article] [Abstract] [Google Scholar]
- Aujla SJ, Dubin PJ, Kolls JK. Th17 cells and mucosal host defense. Semin Immunol. 2007;19:377–382. [Europe PMC free article] [Abstract] [Google Scholar]
- Backhed F, Ley RE, Sonnenburg JL, Peterson DA, Gordon JI. Host-bacterial mutualism in the human intestine. Science. 2005;307:1915–1920. [Abstract] [Google Scholar]
- Bettelli E, Carrier Y, Gao W, Korn T, Strom TB, Oukka M, Weiner HL, Kuchroo VK. Reciprocal developmental pathways for the generation of pathogenic effector TH17 and regulatory T cells. Nature. 2006;441:235–238. [Abstract] [Google Scholar]
- Bettelli E, Oukka M, Kuchroo VK. T(H)-17 cells in the circle of immunity and autoimmunity. Nat Immunol. 2007;8:345–350. [Abstract] [Google Scholar]
- Coombes JL, Robinson NJ, Maloy KJ, Uhlig HH, Powrie F. Regulatory T cells and intestinal homeostasis. Immunol Rev. 2005;204:184–194. [Abstract] [Google Scholar]
- Coquet JM, Chakravarti S, Smyth MJ, Godfrey DI. Cutting edge: IL-21 is not essential for Th17 differentiation or experimental autoimmune encephalomyelitis. J Immunol. 2008;180:7097–7101. [Abstract] [Google Scholar]
- Denning TL, Wang YC, Patel SR, Williams IR, Pulendran B. Lamina propria macrophages and dendritic cells differentially induce regulatory and interleukin 17-producing T cell responses. Nat Immunol. 2007;8:1086–1094. [Abstract] [Google Scholar]
- Dewhirst FE, Chien CC, Paster BJ, Ericson RL, Orcutt RP, Schauer DB, Fox JG. Phylogeny of the defined murine microbiota: altered Schaedler flora. Appl Environ Microbiol. 1999;65:3287–3292. [Europe PMC free article] [Abstract] [Google Scholar]
- Eberl G, Marmon S, Sunshine MJ, Rennert PD, Choi Y, Littman DR. An essential function for the nuclear receptor RORgamma(t) in the generation of fetal lymphoid tissue inducer cells. Nat Immunol. 2004;5:64–73. [Abstract] [Google Scholar]
- Elson CO, Cong Y, McCracken VJ, Dimmitt RA, Lorenz RG, Weaver CT. Experimental models of inflammatory bowel disease reveal innate, adaptive, and regulatory mechanisms of host dialogue with the microbiota. Immunol Rev. 2005;206:260–276. [Abstract] [Google Scholar]
- Fujiwara D, Wei B, Presley LL, Brewer S, McPherson M, Lewinski MA, Borneman J, Braun J. Systemic control of plasmacytoid dendritic cells by CD8+ T cells and commensal microbiota. J Immunol. 2008;180:5843–5852. [Europe PMC free article] [Abstract] [Google Scholar]
- He B, Xu W, Santini PA, Polydorides AD, Chiu A, Estrella J, Shan M, Chadburn A, Villanacci V, Plebani A, et al. Intestinal bacteria trigger T cell-independent immunoglobulin A(2) class switching by inducing epithelial-cell secretion of the cytokine APRIL. Immunity. 2007;26:812–826. [Abstract] [Google Scholar]
- McKenzie Ivanov B.S., II, Zhou L, Tadokoro CE, Lepelley A, Lafaille JJ, Cua DJ, Littman DR. The orphan nuclear receptor RORgammat directs the differentiation program of proinflammatory IL-17+ T helper cells. Cell. 2006;126:1121–1133. [Abstract] [Google Scholar]
- Zhou Ivanov L., II, Littman DR. Transcriptional regulation of Th17 cell differentiation. Semin Immunol. 2007 [Europe PMC free article] [Abstract] [Google Scholar]
- Jensen KD, Su X, Shin S, Li L, Youssef S, Yamasaki S, Steinman L, Saito T, Locksley RM, Davis MM, et al. Thymic Selection Determines gammadelta T Cell Effector Fate: Antigen-Naive Cells Make Interleukin-17 and Antigen-Experienced Cells Make Interferon gamma. Immunity. 2008 [Europe PMC free article] [Abstract] [Google Scholar]
- Kelsall BL, Leon F. Involvement of intestinal dendritic cells in oral tolerance, immunity to pathogens, and inflammatory bowel disease. Immunol Rev. 2005;206:132–148. [Abstract] [Google Scholar]
- Khader SA, Bell GK, Pearl JE, Fountain JJ, Rangel-Moreno J, Cilley GE, Shen F, Eaton SM, Gaffen SL, Swain SL, et al. IL-23 and IL-17 in the establishment of protective pulmonary CD4+ T cell responses after vaccination and during Mycobacterium tuberculosis challenge. Nat Immunol. 2007;8:369–377. [Abstract] [Google Scholar]
- Korn T, Bettelli E, Gao W, Awasthi A, Jager A, Strom TB, Oukka M, Kuchroo VK. IL-21 initiates an alternative pathway to induce proinflammatory T(H)17 cells. Nature. 2007;448:484–487. [Europe PMC free article] [Abstract] [Google Scholar]
- Langrish CL, Chen Y, Blumenschein WM, Mattson J, Basham B, Sedgwick JD, McClanahan T, Kastelein RA, Cua DJ. IL-23 drives a pathogenic T cell population that induces autoimmune inflammation. J Exp Med. 2005;201:233–240. [Europe PMC free article] [Abstract] [Google Scholar]
- Ley RE, Hamady M, Lozupone C, Turnbaugh PJ, Ramey RR, Bircher JS, Schlegel ML, Tucker TA, Schrenzel MD, Knight R, et al. Evolution of Mammals and Their Gut Microbes. Science. 2008 [Europe PMC free article] [Abstract] [Google Scholar]
- Lupp C, Robertson ML, Wickham ME, Sekirov I, Champion OL, Gaynor EC, Finlay BB. Host-mediated inflammation disrupts the intestinal microbiota and promotes the overgrowth of Enterobacteriaceae. Cell Host Microbe. 2007;2:119–129. [Abstract] [Google Scholar]
- Macpherson AJ, Harris NL. Interactions between commensal intestinal bacteria and the immune system. Nat Rev Immunol. 2004;4:478–485. [Abstract] [Google Scholar]
- Mangan PR, Harrington LE, O’Quinn DB, Helms WS, Bullard DC, Elson CO, Hatton RD, Wahl SM, Schoeb TR, Weaver CT. Transforming growth factor-beta induces development of the T(H)17 lineage. Nature. 2006;441:231–234. [Abstract] [Google Scholar]
- Mazmanian SK, Liu CH, Tzianabos AO, Kasper DL. An immunomodulatory molecule of symbiotic bacteria directs maturation of the host immune system. Cell. 2005;122:107–118. [Abstract] [Google Scholar]
- Mazmanian SK, Round JL, Kasper DL. A microbial symbiosis factor prevents intestinal inflammatory disease. Nature. 2008;453:620–625. [Abstract] [Google Scholar]
- McKenzie BS, Kastelein RA, Cua DJ. Understanding the IL-23-IL-17 immune pathway. Trends Immunol. 2006;27:17–23. [Abstract] [Google Scholar]
- Mebius RE. Organogenesis of lymphoid tissues. Nat Rev Immunol. 2003;3:292–303. [Abstract] [Google Scholar]
- Mucida D, Park Y, Kim G, Turovskaya O, Scott I, Kronenberg M, Cheroutre H. Reciprocal TH17 and regulatory T cell differentiation mediated by retinoic acid. Science. 2007;317:256–260. [Abstract] [Google Scholar]
- Nurieva R, Yang XO, Martinez G, Zhang Y, Panopoulos AD, Ma L, Schluns K, Tian Q, Watowich SS, Jetten AM, et al. Essential autocrine regulation by IL-21 in the generation of inflammatory T cells. Nature. 2007;448:480–483. [Abstract] [Google Scholar]
- Ouyang W, Kolls JK, Zheng Y. The biological functions of T helper 17 cell effector cytokines in inflammation. Immunity. 2008;28:454–467. [Europe PMC free article] [Abstract] [Google Scholar]
- Rakoff-Nahoum S, Paglino J, Eslami-Varzaneh F, Edberg S, Medzhitov R. Recognition of commensal microflora by toll-like receptors is required for intestinal homeostasis. Cell. 2004;118:229–241. [Abstract] [Google Scholar]
- Sartor RB. Microbial influences in inflammatory bowel diseases. Gastroenterology. 2008;134:577–594. [Abstract] [Google Scholar]
- Sonderegger I, Kisielow J, Meier R, King C, Kopf M. IL-21 and IL-21R are not required for development of Th17 cells and autoimmunity in vivo. Eur J Immunol. 2008;38:1833–1838. [Abstract] [Google Scholar]
- Stockinger B, Veldhoen M. Differentiation and function of Th17 T cells. Curr Opin Immunol. 2007;19:281–286. [Abstract] [Google Scholar]
- Strober W, Fuss I, Mannon P. The fundamental basis of inflammatory bowel disease. J Clin Invest. 2007;117:514–521. [Europe PMC free article] [Abstract] [Google Scholar]
- Talham GL, Jiang HQ, Bos NA, Cebra JJ. Segmented filamentous bacteria are potent stimuli of a physiologically normal state of the murine gut mucosal immune system. Infect Immun. 1999;67:1992–2000. [Europe PMC free article] [Abstract] [Google Scholar]
- Travis MA, Reizis B, Melton AC, Masteller E, Tang Q, Proctor JM, Wang Y, Bernstein X, Huang X, Reichardt LF, et al. Loss of integrin alpha(v)beta8 on dendritic cells causes autoimmunity and colitis in mice. Nature. 2007;449:361–365. [Europe PMC free article] [Abstract] [Google Scholar]
- Uematsu S, Fujimoto K, Jang MH, Yang BG, Jung YJ, Nishiyama M, Sato S, Tsujimura T, Yamamoto M, Yokota Y, et al. Regulation of humoral and cellular gut immunity by lamina propria dendritic cells expressing Toll-like receptor 5. Nat Immunol. 2008;9:769–776. [Abstract] [Google Scholar]
- Umesaki Y, Setoyama H, Matsumoto S, Imaoka A, Itoh K. Differential roles of segmented filamentous bacteria and clostridia in development of the intestinal immune system. Infect Immun. 1999;67:3504–3511. [Europe PMC free article] [Abstract] [Google Scholar]
- Veldhoen M, Hocking RJ, Atkins CJ, Locksley RM, Stockinger B. TGFbeta in the context of an inflammatory cytokine milieu supports de novo differentiation of IL-17-producing T cells. Immunity. 2006;24:179–189. [Abstract] [Google Scholar]
- Weaver CT, Harrington LE, Mangan PR, Gavrieli M, Murphy KM. Th17: an effector CD4 T cell lineage with regulatory T cell ties. Immunity. 2006;24:677–688. [Abstract] [Google Scholar]
- Weaver CT, Hatton RD, Mangan PR, Harrington LE. IL-17 family cytokines and the expanding diversity of effector T cell lineages. Annu Rev Immunol. 2007;25:821–852. [Abstract] [Google Scholar]
- Yang XO, Panopoulos AD, Nurieva R, Chang SH, Wang D, Watowich SS, Dong C. STAT3 regulates cytokine-mediated generation of inflammatory helper T cells. J Biol Chem. 2007;282:9358–9363. [Abstract] [Google Scholar]
- Zheng Y, Valdez PA, Danilenko DM, Hu Y, Sa SM, Gong Q, Abbas AR, Modrusan Z, Ghilardi N, de Sauvage FJ, et al. Interleukin-22 mediates early host defense against attaching and effacing bacterial pathogens. Nat Med. 2008;14:282–289. [Abstract] [Google Scholar]
- Zhou L, Spolski Ivanov R., II, Min R, Shenderov K, Egawa T, Levy DE, Leonard WJ, Littman DR. IL-6 programs T(H)-17 cell differentiation by promoting sequential engagement of the IL-21 and IL-23 pathways. Nat Immunol. 2007;8:967–974. [Abstract] [Google Scholar]
- Zhou L, Lopes JE, Chong MM, Min Ivanov R., II, Victora GD, Shen Y, Du J, Rubtsov YP, Rudensky AY, et al. TGF-beta-induced Foxp3 inhibits T(H)17 cell differentiation by antagonizing RORgammat function. Nature. 2008;453:236–240. [Europe PMC free article] [Abstract] [Google Scholar]
Full text links
Read article at publisher's site: https://doi.org/10.1016/j.chom.2008.09.009
Read article for free, from open access legal sources, via Unpaywall:
http://www.cell.com/article/S1931312808003053/pdf
Citations & impact
Impact metrics
Citations of article over time
Alternative metrics

Discover the attention surrounding your research
https://www.altmetric.com/details/101817038
Article citations
Protocol for intracellular immunofluorescence measurements of latent HIV reactivation in a primary CD4<sup>+</sup> T cell model.
STAR Protoc, 5(4):103398, 18 Oct 2024
Cited by: 0 articles | PMID: 39425934 | PMCID: PMC11513541
A review of the pathogenesis of epilepsy based on the microbiota-gut-brain-axis theory.
Front Mol Neurosci, 17:1454780, 03 Oct 2024
Cited by: 0 articles | PMID: 39421261 | PMCID: PMC11484502
Review Free full text in Europe PMC
From bench to bedside: an interdisciplinary journey through the gut-lung axis with insights into lung cancer and immunotherapy.
Front Immunol, 15:1434804, 05 Sep 2024
Cited by: 0 articles | PMID: 39301033 | PMCID: PMC11410641
Review Free full text in Europe PMC
Role and functional mechanisms of IL‑17/IL‑17R signaling in pancreatic cancer (Review).
Oncol Rep, 52(5):144, 02 Sep 2024
Cited by: 0 articles | PMID: 39219271 | PMCID: PMC11378154
Review Free full text in Europe PMC
Microbiome-Stealth Regulator of Breast Homeostasis and Cancer Metastasis.
Cancers (Basel), 16(17):3040, 31 Aug 2024
Cited by: 0 articles | PMID: 39272898 | PMCID: PMC11394247
Review Free full text in Europe PMC
Go to all (1,114) article citations
Data
Data behind the article
This data has been text mined from the article, or deposited into data resources.
BioStudies: supplemental material and supporting data
Similar Articles
To arrive at the top five similar articles we use a word-weighted algorithm to compare words from the Title and Abstract of each citation.
Aberrant intestinal microbiota due to IL-1 receptor antagonist deficiency promotes IL-17- and TLR4-dependent arthritis.
Microbiome, 5(1):63, 23 Jun 2017
Cited by: 53 articles | PMID: 28645307 | PMCID: PMC5481968
Epithelial-derived IL-18 regulates Th17 cell differentiation and Foxp3⁺ Treg cell function in the intestine.
Mucosal Immunol, 8(6):1226-1236, 04 Mar 2015
Cited by: 119 articles | PMID: 25736457 | PMCID: PMC4368110
Reciprocal TH17 and regulatory T cell differentiation mediated by retinoic acid.
Science, 317(5835):256-260, 14 Jun 2007
Cited by: 1321 articles | PMID: 17569825
Synergy of IL-23 and Th17 cytokines: new light on inflammatory bowel disease.
Neurochem Res, 35(6):940-946, 14 Nov 2009
Cited by: 57 articles | PMID: 19915978 | PMCID: PMC7241863
Review Free full text in Europe PMC
Funding
Funders who supported this work.
Howard Hughes Medical Institute
NCI NIH HHS (3)
Grant ID: R01 CA034282-23
Grant ID: CA034282
Grant ID: R01 CA034282
NCRR NIH HHS (3)
Grant ID: P40RR018603
Grant ID: P40 RR018603-05
Grant ID: P40 RR018603
NIAID NIH HHS (1)
Grant ID: R01 AI033856
NIDDK NIH HHS (5)
Grant ID: R01 DK053347-09
Grant ID: P30 DK034987
Grant ID: R01 DK53347
Grant ID: R01 DK053347
Grant ID: P30DK34987