Abstract
Free full text

Macrophages Directly Contribute to the Exaggerated Inflammatory Response in Cystic Fibrosis Transmembrane Conductance Regulator−/− Mice
Abstract
Pulmonary infection with an exaggerated inflammatory response is the major cause of morbidity and mortality in cystic fibrosis (CF). The objective of this study was to determine whether differences in the innate immune system underlie the exaggerated immune response in CF. We established a model that recapitulates the exaggerated immune response in a CF mouse model by exposure to Pseudomonas aeruginosa LPS and assessed the pulmonary cellular and cytokine responses of wild-type (WT) and CF mice. Compared with WT mice, CF mice had increased numbers of neutrophils and increased proinflammatory cytokines in their bronchoalveolar lavage fluid after LPS exposure. Based on the increased levels of IL-1α, IL-6, granulocyte colony-stimulating factor (G-CSF), and keratinocyte chemoattractant, all of which are known to be produced by macrophages, we tested whether two populations of macrophages, bone marrow–derived macrophages and alveolar macrophages, directly contribute to the elevated cytokine response of CF mice to LPS. After in vitro stimulation of bone marrow–derived macrophages and alveolar macrophages with LPS, IL-1α, IL-6, G-CSF, and monocyte chemoattractant protein-1 were higher in CF compared with WT cell supernatants. Quantitative analyses for IL-6 and keratinocyte chemoattractant revealed that LPS-stimulated CF macrophages have higher mRNA and intracellular protein levels compared with WT macrophages. Our data support the hypothesis that macrophages play a role in the exuberant cytokine production and secretion that characterizes CF, suggesting that the macrophage response may be an important therapeutic target for decreasing the morbidity of CF lung disease.
Cystic fibrosis (CF) is caused by mutations of the CF transmembrane conductance regulator (CFTR) gene, which encodes a chloride channel that is expressed on the apical membrane of secretory epithelia. The absence of functional CFTR in airway epithelia of patients with CF leads to abnormal airway surface liquid, which favors chronic infection with Pseudomonas aeruginosa (PA) and other bacteria. This chronic infection in CF lungs is associated with an exaggerated inflammatory response characterized by abnormal cytokine secretion and neutrophil infiltration in the lung. Although it is clear that the proximal cause of disease in CF is lack of normal levels of functional CFTR protein, the mechanisms by which this leads to chronic lung infection have not been fully elucidated (1). It has been postulated that the lack of CFTR activity impacts the immune response in the respiratory tract at a number of levels. The airway epithelium, which has been studied extensively, is known to contribute to this response. However, little is known about the role of CFTR−/− immune cells in the exaggerated response to bacterial infection. Neither is it known if immune cells have a direct contribution to the lung phenotype, or if the abnormal response is a consequence of the primary epithelial defect. Although somewhat controversial (2), recent data suggest that CFTR may have a significant role in the normal function of immune cells. For instance, Di and colleagues (3) demonstrated that CFTR is expressed in phagolysosomes of macrophages and has a critical role in phagosome acidification, which is fundamental for efficient bactericidal activity. Others have shown that a similar defect in CF-affected neutrophils leads to a decreased ability to kill organisms (4, 5). Also, CFTR activity may be necessary for the physiological apoptosis of neutrophils, which is required to end an inflammatory response (6).
Taken together, these data suggest that there are multiple steps in the innate immune response that are potentially affected by CFTR dysfunction, and provide the rationale for our studies.
MATERIALS AND METHODS
Mouse Breeding
Transgenic CFTR−/− (B6.129P2-Cftrtm1Unc) (7) mice were purchased from the Jackson Laboratory (Bar Harbor, ME), and bred in the Yale University Animal Facility. CFTR−/− mice were fed with a liquid diet (Peptamen; Nestle, Deerfield, IL), as previously described (8). Non-CF mice were maintained on the identical diet to the CFTR−/− mice to eliminate nutritional status as a potential confounder. All procedures were performed in compliance with relevant laws and institutional guidelines, and were approved by the Yale University Institutional Animal Care and Use Committee.
In Vivo LPS Administration
Wild-type (WT), heterozygous (HET), and CF mice received three doses of LPS (no. L8643; Sigma, St. Louis, MO) over 3 days. LPS (12.5 mg) was administered to the mice with a nebulizer (Pulmo-Aide Compressor; Natallergy, Duluth, GA). A 5-ml aliquot of solution was nebulized over 15 minutes. WT, HET, and CF mice were randomized to three different groups that were treated at different times. Table E1 in the online supplement summarizes the age, sex, and weight of the mice used in all three experiments.
Bronchoalveolar Lavage Fluid Collection Analysis and Lung Histology
Mice were anesthetized and 200 μl of blood was recovered by retroorbital puncture. Bronchoalveolar lavage (BAL) fluid was collected using standard methods. The dilution of epithelial lining fluid recovered with BAL was calculated based on the blood urea nitrogen concentration in the plasma, as previously described (9). Lung tissues were paraffin embedded and then stained with H&E for morphological analysis (see online supplement).
Isolation and Culture of Alveolar Macrophages and Differentiation of Bone Marrow–Derived Macrophages
Bone marrow–derived macrophages.
Bone marrow (BM) collection was performed as previously described (10). After overnight culture, the nonadherent cells were differentiated for 7 days in 20 ng/ml recombinant macrophage colony-stimulating factor (M-CSF) (315–02 PeproTech Inc., Rocky Hill, NJ). After 7 days, cells were detached, characterized by flow cytometry (F4-80+/MAC-1+ population), and morphology analyzed on cytospin (Figure E1). We obtained ~ 1–3 × 107 mature macrophages from each mouse. Cells were plated in six-well plates at a concentration of 1 × 106 cells/well overnight, challenged with LPS (10 ng/ml) for the time indicated and the supernatant, harvested for analysis. For time 0 hours, fresh media without LPS were harvested after 12 hours of incubation.
Alveolar macrophages.
BAL fluid was recovered from untreated mice (a total of 1 × 104 to 1 × 105 macrophages were recovered from each mouse, depending on genotypes). After culturing BAL cells for 24 hours, fresh media containing 10 ng/ml LPS was added and cells and supernatants were analyzed. Figure E1 shows validation of alveolar macrophages (AMs).
Cytokine Quantification
The cytokine concentration in the BAL or in the media of cultured macrophages was assessed either by ELISA (Quantikine Mouse MIP-2; R&D Systems, Inc., Minneapolis, MN) or by LINCO-plex following the manufacturer's instructions (LINCO, MCYTO-70K-PMX22; St. Charles, MO). Real-time PCR analysis was performed with an iCycler (Bio-Rad, Hercules, CA) using TaqMan technology. Copy number was normalized to 18S levels. Intracellular IL-6 and keratinocyte chemoattractant (KC) quantification was performed by flow cytometry (see online supplement) and confirmed by Western Blot.
BM Transplantation Method
Adult BM transplantation was performed as previously described (10). Bone marrow–derived cells (BMDC) were suspended in serum-containing medium at 5 × 106 cells/100 μl. Recipients were irradiated with 900 rads using a cesium irradiator. BMDC were injected intravenously 4 hours after irradiation, as previously described (11).
Statistical Analysis
All graphical presentations show data on the raw scale of measurement. Because of concerns over skewed distributions, all data were log-transformed prior to statistical analysis. In addition, we present the results of both parametric and nonparametric analyses, wherever feasible, to address concerns about potential outliers.
We used ANOVA to compare the in vivo BAL cytokine concentrations in WT, HET, and CF mice. For each cytokine, ANOVA was used to model the log-transformed data as a function of time (treated as a factor with three levels: 3, 6, and 24 h after LPS stimulation) and type (CF, HET, and WT). When the analyses of variance uncovered interesting differences between types, paired t tests were used to study differences between WT, HET, and CF. Statistical analysis of the in vitro results was carried out using one-sided, two-sample t tests (allowing for unequal variances) and Wilcoxon rank-sum tests. We present all P values rather highlighting statistical significance at an arbitrary level.
RESULTS
Increased Inflammatory Cells in the Lungs of CF Mice before and after LPS Administration In Vivo
We first confirmed that repeated administration of PA LPS recapitulates the abnormal inflammatory response observed in CF mice in response to live PA bacteria (12). CFTR+/+ (n = 6 for each time point), CFTR+/− (n = 9 for each time point), and CFTR−/− (n = 9 for each time point) mice were nebulized with LPS as described in Materials and methods, and killed 3, 6, 24, and 72 hours after the last LPS dose (see Table E1 for a detailed description of mice used). At 0 hours (before LPS treatment), CF and HET mice had a significantly higher number of total BAL cells, and of macrophages in the BAL compared with WT mice (Figure 1A; P values from the statistical analyses are summarized in Table E2), supporting the hypothesis that, although CF mice do not display overt lung pathology, they have an abnormal phenotype, not only in their transepithelial electrophysiologic response, but also in their immune profile (13).
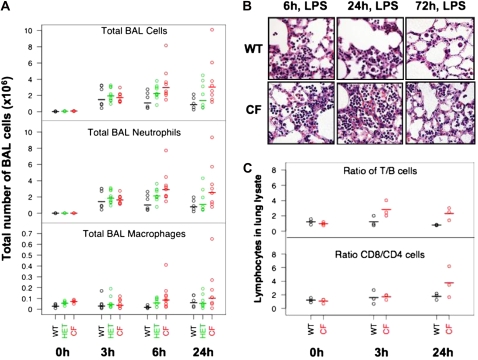
Cystic fibrosis (CF) and HET mice treated with LPS have higher numbers of cells in the BAL. (A) Total and differential cell counting in the bronchoalveolar lavage (BAL) fluid of control (0 h) and LPS-treated (3, 6, and 24 h) wild-type (WT) (black), HET (green), and CF (red) mice. Note different scales for neutrophils and macrophages. (B) H&E staining of lung tissues, confirming that the clearance of neutrophils in the lung of CF mice is slower than in WT mice. (C) Top: ratio of T (CD3+) to B (B220+) lymphocyte; bottom: ratio of CD8 to CD4 lymphocytes, as assessed by flow cytometry of whole-lung digests. Line segments denote means (calculated on the log scale).
In all three groups of mice, the total cell number in the BAL 3 hours after the third dose of LPS was higher than untreated mice (0 h), and the BAL cells were mostly polymorphonuclear leukocyte neutrophils (PMN) (Figure 1A). The number of PMNs and macrophages in the BAL 6 hours after the last dose of LPS was significantly higher in CF and HET mice than in WT mice. Furthermore, HET mice had a cell count that was intermediate to that of WT and CF mice. The higher number of PMNs observed in the CF mice persisted for over 24 hours (Figure 1A, Table E2). However, by 72 hours, the BAL cell number returned to baseline, which, in the CF mice, was still elevated when compared with WT mice (data not shown). Note that these statistical analyses were performed using both parametric (t tests) and nonparametric (Wilcoxon rank-sum tests) approaches, and, in most cases, the results were very similar (Table E2). Lung histology reflected the PMN infiltration in all three groups of mice treated with LPS, and confirmed the increased numbers and slower clearance of PMNs in CF mice (Figure 1B and Figure E2).
Using flow cytometry on single-cell preparations (n = 3, WT and CF mice), we examined the ratio of B to T lymphocytes in untreated (0 h) and LPS-treated lungs (3 and 24 h) (Figure 1C). Although the total number of cells cannot be calculated given that this is a lung lysate, and the cell number varies depending on the efficiency of the digest, one can determine the ratio of T to B cells before and after LPS treatment in CF and WT mice. Using this comparison, the T:B ratio was higher in CF lungs than WT lungs after LPS exposure (two-sided t test at 3 h, P = 0.04, and at 24 h, P = 0.011). Within the T-cell population, CD8:CD4 ratio was greater than 1 in both WT and CF lungs (Figure 1C). Taken together, these data show that CF mice have higher numbers of immune cells in the lung than WT mice under naive as well as LPS-stimulated conditions, and, interestingly, HET mice have an intermediate cell number. After LPS stimulation, the high numbers of neutrophils that migrate to the lung are cleared more slowly in CF mice. Finally, other immune cells, including macrophages and perhaps T cells, are also elevated in CF lungs.
Increased Inflammatory Cytokines in BAL In Vivo after LPS Stimulation
We analyzed the concentration of 22 cytokines in the BAL fluid of treated mice using a microsphere-based multiplex immunoassay (Luminex; Millipore, Billerica, MA). Based on ANOVA, the levels of nine cytokines (IL-1α, IL-6, IL-12p70, IL-17, IL-2, IL-4, IL-7, granulocyte colony-stimulating factor [G-CSF], and KC) were different between CF and WT BAL after LPS stimulation. There were significant increases in CF BAL of IL-1α, IL-6, IL-12p70, IL-17, IL-7, G-CSF, and KC and decreases in IL-2 and IL-4 (Figures 2A and 2B). The levels of all other cytokines assessed are presented in Figure E3. Although the differences between WT and CF for the remaining 13 cytokines were not significantly different, 5 of the 13 cytokines (e.g., monocyte chemoattractant protein [MCP]-1, macrophage inhibitory protein [MIP]-1α, TNF-α, IFN-γ, and IL-β) showed increased concentrations in CF BAL. The concentration of MIP-2, assessed by ELISA immunoassay, was also elevated in CF BAL (data not shown). Of note, the concentration of IL-10 was lower in CF BAL (Figure E3). Interestingly, the cytokines that were present at higher concentrations in BAL from CF mice (IL-1α, IL-6, G-CSF and KC) are produced predominantly by macrophages, with lesser contributions from epithelial and endothelial cells. In contrast, the cytokines that were present in lower concentrations are mainly secreted by lymphocytes, such as IL-2 and IL-4. However, IL-17, the proinflammatory cytokine that regulates both granulopoiesis and recruitment of neutrophils, is elevated in CF when compared with WT, which is similar to the findings of Dubin and Kolls (14) in both murine models and human CF lung disease.

Comparison of BAL cytokine concentrations in LPS-treated WT, HET, and CF mice. (A) BAL cytokine concentration before (0 h) and after (3, 6, 24 h) LPS treatment in WT (black), HET (green), and CF (red) mice. The cytokines represented here show notable differences between WT and CF. Cytokines that did not exhibit significant differences between WT and CF are shown in Figure E2. (B) P values for the paired genotype comparisons for the nine cytokines represented in (A). The first column of P values (Type) shows the statistical significance of the genotypes in the ANOVA. The other columns of P values show the subsequent paired comparisons between WT and HET (WTvHET), HET and CF (HETvCF). and WT and CF (WTvCF).
Cytokine concentrations in HET mice were generally intermediate between WT and CF values (Figure 2 and Figure E3). Furthermore, HET BAL was significantly different from WT BAL for IL-6 (P = 0.0060) and KC (P = 0.0009), suggesting that these cytokines may be directly affected by the amount of CFTR expressed. Mice nebulized with saline alone showed no alteration in the BAL cellularity or cytokine secretion (Figure E3).
Although the secretion of almost all of the cytokines changed over time, with a general increase at 3 and 6 hours and a drop at 24 hours after LPS stimulation (Figure 2 and Figure E3), there were no genotype-dependent alterations in the time course of secretion, except for IL-17 and G-CSF. IL-17 was higher in CF BAL at the early time points (3 and 6 h), and was lower at 24 hours when compared with WT and HET BAL. In contrast, G-CSF increased similarly in WT, HET, and CF media at 3 and 6 hours; however, the concentration of this cytokine remained high in CF, whereas it dropped in HET and WT BAL by 24 hours after LPS stimulation (Figure 2). Taken together, it appears that LPS treatment of mice that lack CFTR leads to broad alterations in the levels of cytokines that are associated with macrophages. Furthermore, these changes may be directly affected by the amount of CFTR activity for a subset of the cytokines.
CF Macrophages Have Higher LPS-Stimulated Cytokine Secretion than WT Macrophages In Vitro
We wanted to determine whether the hyperresponsiveness demonstrated in vivo could be due, at least in part, to CF macrophages. If the hyperresponsiveness demonstrated in the in vivo model is a primary property of all CFTR−/− macrophages, then CF macrophages derived in vitro from BM precursors should respond to LPS stimulation differently from WT macrophages. Conversely, if this hyperresponsiveness is only observed in vivo, then either the observed response is not macrophage driven, and only driven by the epithelia, or the subset of macrophages from the alveolar microenvironment may be different from their BM derived counterparts. Such a result would suggest that this increased cytokine secretion may be influenced by the CF lung microenvironment.
To test whether macrophages may directly contribute to the elevated secretion of cytokines by CFTR−/− mice in response to LPS, macrophages from WT, HET, and CF mice (n = 6 mice for each genotype) were differentiated from BM in the presence of M-CSF. First, we confirmed CFTR expression in WT macrophages differentiated from BM cells, and were able to demonstrate that they express low but detectable levels of CFTR mRNA after two cycles of amplification, as assessed by RT-PCR (data not shown). The cells were then cultured in the presence or absence of LPS, and the cytokine profiles in the supernatants were assessed from both WT and CF macrophages using Luminex analysis. Nine cytokines that were elevated in vivo were examined (IL-1α, IL-12p70, IL-17, IL-2, IL-6, IL-9, MCP-1, G-CSF, MIP-1α, and KC) as were granulocyte macrophage colony-stimulating factor (GM-CSF), IL-1β, and TNF-α. Supernatants were harvested at 0 (no LPS), 3, 6, 24, and 48 hours after LPS treatment. All values were normalized to 1 × 106 cells. In the absence of LPS exposure, cultured BM-derived macrophages (BMDM) had low levels of secretion of all cytokines except for MCP-1, which was high (3,000 pg/ml) in both WT and CF.
After LPS stimulation, CF BMDM had robust levels of secretion of IL-1α, IL-6, KC, MCP-1, and MIP-1α at all time points analyzed (3, 6, 24, and 48 h) when compared with WT. In addition, G-CSF secretion was higher in CF compared with WT at the earliest time points (3 and 6 h), and was equal to WT cells at later time points (Figures 3A and 3B). The cytokine levels secreted by HET macrophages were between those of CF and WT for KC, IL-1α, and MCP-1. The concentration of IL-9 was notably increased after LPS stimulation; however, no difference was observed between WT and CF cells. As expected, IL-12p70, IL-17, and IL-2 were not detected in LPS-stimulated macrophages, as these cytokines are primarily produced by lymphocytes. Consistent with the in vivo data, GM-CSF, IL-β, and TNF-α were not significantly different between CF and WT.

Kinetics of cytokine secretion by bone marrow (BM)–derived macrophages (BMDM) after LPS stimulation. (A) Supernatants from BMDM from WT (black), HET (green), and CF (red) mice (n = 6 mice for each genotype) were analyzed before (0 h) and after (3, 6, 24, and 48 h) stimulation with LPS for the cytokine concentration (pg/ml) by Luminex. The concentration of each cytokine was normalized to 1 × 106 BMDC. The plots represent means of cytokine secretions for the six cytokines showing the most robust differences between WT and CF. Note that for G-CSF, only the 3 and 6 hours time points are graphed. At 0 hours G-CSF was not detected and at 24 and 48 hours G-CSF concentration was much higher than 3 and 6 hours, but showed no differences between the genotypes. (B) P values from one-sided, two-sample t tests (unequal variances) and Wilcoxon rank-sum tests for the cytokine concentrations in the supernatant of WT, HET, and CF BMDMs cultured in the absence (0 h) or presence of LPS. Values less than 0.05 are in boldface.
To test whether the differences in cytokine secretion between WT and CF macrophages were due to a different rate of cell division or cell death, we counted the total live cells per well at 12, 24, and 48 hours after LPS stimulation. There was no difference in survival or cell number between WT and CF macrophages in response to LPS (data not shown). In conclusion, these data show that, consistent with in vivo observations, CF macrophages have altered cytokine secretion upon LPS stimulation, and, as observed in vivo, the amount of expression of functional CFTR protein may influence the production/secretion of some cytokines, such as KC.
Elevated Cytokine Secretion Is Associated with an Increase in Both mRNA and Intracellular Protein Levels
To determine whether the increased cytokine levels observed in the supernatant of CF macrophages were due to alterations in transcriptional and/or translational processes, we performed quantitative RT-PCR as well as intracellular flow cytometry analysis (Figure 4). For this evaluation, we focused on IL-6 and KC, and found that mRNA levels of both cytokines increased within hours after the addition of LPS. After LPS stimulation, WT and CF macrophages had a clear difference in the degree of increase of both KC and IL-6 transcripts (Figure 4A). IL-6 mRNA in CF macrophages was 3.7-fold higher than WT 1 hour after LPS, and was ~ twofold higher at 3, 6, and 24 hours. For both WT and CF macrophages, the highest concentration of IL-6 mRNA was detected 6 hours after LPS treatment. KC mRNA in CF macrophages was twofold higher than WT 1 and 3 hours after LPS stimulation. Quantification of IL-6 protein was performed after 6 and 24 hours of LPS exposure. CF macrophages had significantly more IL-6 protein than WT macrophages at 24 hours, as determined by flow cytometry (Figure 4B) and confirmed by Western blot analysis. Similar results were observed for KC (data not shown). These data show that LPS exposure leads to greater increases in mRNA expression and protein production of proinflammatory cytokines in CF macrophages compared with WT.

mRNA and intracellular protein quantification of IL-6 and KC. (A) The relative amount of specific mRNA expression in macrophages was determined by quantitative RT-PCR using a standard curve method. The copy number of IL-6 mRNA (top) and KC mRNA (bottom) was normalized to a ubiquitously expressed mRNA (18S) level. The data are expressed as the mRNA expression at different time points after LPS treatment, normalized to mRNA expression in untreated cells. The data represent the average of two independent experiments and two RT-PCR repeats for WT (open bars) and CF (closed bars). (B) Intracellular staining for IL-6 before (0 h) and after (24 h) LPS treatment. Error bars: standard error of the mean (SEM).
Characterization of WT, HET, and CF AMs Challenged with LPS In Vitro
Next, we examined whether WT, HET, and CF AM isolated from BAL and grown in culture would respond to LPS stimulation in a similar manner to BMDMs. As expected, WT AM expressed CFTR, whereas CFTR−/− macrophages did not (Figure 5A). In the absence of LPS exposure (time 0), the concentrations of IL-6, KC, and TNF-α were detectable in the AM, unlike in the BMDMs. This difference may be due to prior exposure of the AM to antigens in vivo, whereas the BMDMs were a truly naive population, as they were differentiated in vitro. Even before LPS exposure, the concentrations of IL-6 (P < 0.003), G-CSF (P = 0.019), and TNF-α (P = 0.0009) were much higher in CF supernatant compared with WT (Figure 5B). Also, in AMs, KC is secreted in the absence of LPS stimulation (Figure 5B), unlike in BMDMs. However, this higher concentration of KC was not different between WT and CF AM supernatants, suggesting that AMs may be already in an activated state.
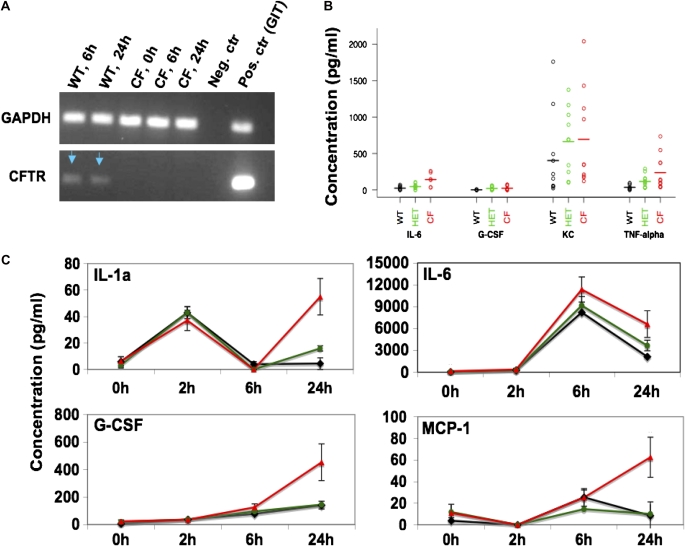
Alveolar macrophage (AM) characterization. (A) RT-PCR for GAPDH (upper panel) and CF transmembrane conductance regulator (CFTR) (lower panel) from mRNA inverted transcribed in cDNA with random primers and amplified with GAPDH- and CFTR-specific primers. Positive control (Pos. ctr): cDNA from WT gastrointestinal tract (GIT). (B) Cytokine concentration in the supernatant of WT (black), HET (green), and CF (red) untreated AM. G-CSF and IL-6 were higher in CF compared with WT (based on t tests and nonparametric tests of log-transformed data; P < 0.05). (C) Mean cytokine concentration after treatment with LPS for different times (2, 6, and 24 h). Concentration (pg/ml) was normalized to 1 × 105. Each of these cytokines showed robust differences between WT and CF at 24 hours (based on t tests and nonparametric tests of log-transformed data; P < 0.05). Error bars: standard error of the mean (SEM).
After the AMs were exposed to LPS in vitro, we noted differences in cytokine secretion between WT and CF cells. There were elevated levels of IL-1α, IL-6, G-CSF, and MCP-1, which were consistent with our in vivo observations. The most drastic differences between the cytokine concentration in WT and CF AM supernatant were observed at 24 hours after LPS treatment (Figure 5C). At this time point, all of the cytokines shown in Figure 5C were higher in CF compared with WT (P < 0.05). Moreover, the IL-6 mRNA in CF AMs was two- and threefold higher than WT at these time points, respectively (data not shown). Lastly, after LPS exposure, the supernatant concentration of TNF-α was not different between WT and CF, which is consistent with what was observed in vivo. Although the differences in cytokine secretion noted in CFTR−/− AMs were also noted in CFTR−/− BMDMs, the pattern of cytokine secretion in AMs was different from BMDMs for many cytokines.
Transplantation of CFTR+/+ BM Corrects a Significant Portion of the Exaggerated Inflammatory Response to LPS in CFTR−/− Mice
To evaluate whether replacement of CFTR−/− BM with WT cells could prevent the observed exaggerated inflammatory response and, thereby, confirm the contribution of the immune cells to the exaggerated inflammatory response to LPS, CFTR+/+ (W) and CFTR−/− (C) mice were total-body irradiated and transplanted with either WT BMs (W-W, n = 5; W-C, n = 6) or CF BM (C-W, n = 3; C-C, n = 3). Three months after BM transplantation, when peripheral blood engraftment had occurred and the majority of BAL cells were donor derived (80 ± 16%, data not shown), mice were nebulized with LPS for 3 days, and the BAL cytokine concentration was evaluated using a microsphere-based multiplex immunoassay.
For 12 of the proinflammatory cytokines (IL-6, IL-12p70, IL-17, IL-9, IL-15, INF-γ, KC, G-CSF, TNF-α, MIP-1α, MCP-1, and regulated upon activation, normal T-cell expressed and secreted), the W-W and W-C groups had lower cytokine concentrations than the C-W and C-C groups, suggesting that their secretion was most affected by the BM cell genotype (Figure 6). In contrast, the concentration of just three cytokines (IL-1β, GM-CSF, and interferon-inducible protein [IP]-10) appeared to be dependent on the host genotype. Their concentrations in the W-W and C-W groups were the same as were their concentrations in the W-C and C-C groups. Additionally, the concentrations of these three cytokines were higher in the W-W and C-W groups than in the W-C and C-C groups. Finally, IL-1α, IL-5, and IL-10 showed a trend across the four groups, with the lowest BAL concentration in the W-W group and the highest in the C-C group (W-W > W-C > C-W > C-C).

Cytokine concentrations in the BAL of transplanted animals after LPS stimulation. BAL cytokine concentrations 6 hours after LPS treatment in WT into WT (W-W), WT into CF (W-C), CF into WT (C-W), and CF into CF (C-C) mice. (A) Nine cytokines, the concentrations of which appear to be dependent on BM genotype. Concentrations of IL-6, IL-17, IL-15, INF-γ, KC, G-CSF, TNF-α, and regulated upon activation, normal T-cell expressed and secreted (RANTES) were significantly different in C-C versus W-C groups and W-W versus C-W (P < 0.05). (B) Three cytokines that appear to be dependent on epithelial (host) genotype. Concentration of IL-1β was significantly different in C-W versus C-C and the W-W versus W-C (P < 0.05). Y axes = concentration (pg/ml). Error bars: standard error of the mean (SEM).
DISCUSSION
The chronic bacterial infection in CF lungs is associated with an excessive inflammatory response, characterized by abnormal cytokine secretion and massive neutrophil infiltration, leading to irreversible lung damage. CF airway epithelia are known to contribute greatly to the abnormal pulmonary inflammatory response (15); however, in the last year, two groups reported that CFTR is also expressed in immune cells, such macrophages and neutrophils. In both of these cell types, lack of CFTR may lead to impaired maturation of the phagolysosome and impaired ingestion of bacteria. Di and colleagues (3) reported that CFTR-null macrophages were unable to acidify the late phagosomes to a pH less than 5, which compromised the ability of these cells to kill bacteria. The investigators hypothesized that the lack of CFTR resulted in alterations in intracellular compartmental chloride concentrations, which then impeded H+ accumulation, thus inhibiting phagolysosomal acidification. Painter and colleagues (4) reached a similar conclusion regarding a function for CFTR in neutrophils. Again, CFTR was expressed on phagolysosomes, and lack of expression was associated with a deficiency in killing of ingested bacteria due to defective hypochlorous acid production within phagolysosomes.
Previously, van Heeckeeren and colleagues have looked at the inflammatory response to PA in CF mice transplanted with WT or CF BM. CF mice that received WT BM displayed a milder response to PA, characterized by lower neutrophilic infiltration in the lung and lower proinflammatory cytokine concentrations in their BAL when compared with CF mice that received CF BM (32). It should be noted that there are a number of other investigators who have examined the inflammatory response to PA in CF mice and have failed to observe a hyperinflammatory response in CF animals (16, 17). Our current transplant data support and extend the van Heeckeeren findings. Furthermore, an elegant study by Oceandy and colleagues (18) supports the hypothesis that deficient CFTR function in both epithelial cells and macrophages contributes to the persistent PA infection in CF. They created transgenic mice expressing CFTR under either an epithelial-specific (CK18) or a macrophage-specific promoter (c-fms) on a CFTR−/− background. Although the mice with epithelial-specific expression of CFTR had a more dramatic amelioration of the response to PA, there was also a significant decrease in the inflammatory response of mice with macrophage-specific expression of CFTR compared with CFTR−/− mice. Specifically, there was a decrease in the bacterial burden in the lung and a decrease in some inflammatory cytokines, including MIP-2 (18). Taken together, these data suggest that macrophages and other immune cells play a role in the exaggerated immune response associated with CF, but the mechanisms by which this occurs have not yet been well defined.
Here, we investigated the contribution of pure populations of macrophages (BMDMs and AMs) in the abnormal immune response to LPS in a murine model of CF. In this model, the responses observed are limited to those triggered by repeated exposure to LPS, instead of the full cascade that is observed when CF mice are infected with PA-impregnated beads. This allows us to investigate whether there are differences in the initial response to Toll-like receptors (TLRs) in CF versus wild-type mice. It should be noted that CFTR−/− mouse models have a variable pulmonary phenotype, but do mirror humans with regard to the exaggerated inflammatory response to bacterial pathogens (13, 19–21). Unlike the differences observed between murine and human neutrophilic responses, which occur in part due to differences in IL-8/CXCR1 system, macrophage responses are reported to be much more homologous, and, therefore, may serve as a good surrogate for the exaggerated inflammatory response observed in CF (22).
First, we assessed the cellularity and cytokine levels in the BAL of WT, HET, and CF mice after LPS stimulation using the murine in vivo model of inflammation previously described by Freedman and colleagues (12). As expected, CF mice had more robust neutrophil infiltration, and neutrophil clearance from the lungs was slower when compared with WT lungs. Also, there were more macrophages in CF lungs, which were observed both before and after LPS stimulation. Lastly, the ratio of T to B lymphocytes (mostly CD8+ cells) was higher in the lungs of CF mice. The BAL fluid of CF mice had higher concentrations of seven cytokines (IL-1α, IL-6, IL-12p70, IL-17, IL-7, G-CSF, and KC), and half of them are produced predominantly by macrophages. Lower levels of IL-2 and IL-4 were observed in the CF mice. Although not statistically significant, the cytokine multiplex analysis revealed a broad cytokine secretion alteration in CF BAL. For instance, as expected, IL-10, an antiinflammatory cytokine involved in CF lung disease, was decreased in the BAL fluid from CF mice (23–26), as were IL-1β and TNF-α, which are well documented to be higher in CF epithelia stimulated with live bacteria (20). The modest differences for these cytokines may be due to our use of aerosolized LPS rather than whole bacteria, which allows for isolation of TLR4-mediated effects. Furthermore, we focused on the in vivo response to LPS and not the direct in vitro response of CF-affected epithelia. Signal transduction in response to LPS is mediated by TLR4, which binds to LPS specifically, in concert with accessory LPS-binding proteins, including MD-2 and CD14 (27). In addition, TLR4 signaling plays a major role in the activation of the innate immune response to PA (28). Airway epithelial cells express TLR4, but it is localized to the basolateral membrane (29), which likely explains the limited involvement of epithelial cells in mediating responses to LPS. In airways, myeloid cells are far more responsive to LPS than epithelial cells (30, 31).
Given that the chemokines and cytokines that were elevated in the BAL of CF mice are either potent activators of acute inflammatory responses (IL-6 and IL-1α), involved in neutrophil migration (e.g., KC) and survival (G-CSF), or involved in the “crosstalk” and stimulation of innate immunity (e.g., IL-12), we hypothesized that, during the acute phase of PA infection, macrophages directly contribute to the abnormal proinflammatory response observed in CF. We therefore looked at the cytokine secretion pattern in CF macrophages under LPS stimulation. The data clearly show that CF macrophages secrete higher levels of the cytokines normally produced during the acute phase of inflammation (IL-1α, IL-6, G-CSF, KC, MCP-1, and MIP-1α). In addition, CF macrophages have higher levels of mRNA and protein for IL-6 and KC. These data suggest that the cytokine secretion alterations observed in CF macrophages may be due to an increased transduction of the inflammatory stimuli, as observed in epithelial cells via the NF-κB pathway. This phenomenon appears to be CFTR dependent, as suggested by our data in which mice and cells heterozygous for the CFTR-null allele have a phenotype between WT and CF. One might speculate that this intermediate phenotype could be due to allele-specific silencing, resulting in half of the macrophages having the knockout phenotype. In addition, our transplant data clearly demonstrate CFTR+/+ hematopoietic cells, including macrophages, significantly dampen the proinflammatory response to LPS in CFTR−/− animals.
In conclusion, our data support the hypothesis that, after LPS stimulation, CF mice have an elevated production of proinflammatory cytokines. Furthermore, CFTR-null macrophages contribute to the exaggerated immune response in CF during the acute phase of infection. Our data demonstrate that the expression of functional CFTR in macrophages influences the secretion of a number of cytokines similar to what has been shown for airway epithelial cells (15). Because macrophages are early responders in the innate immune system, and the subsequent adaptive immune response is very much dependent on the initial response of macrophages, abnormal macrophage activity due to the lack of CFTR may be one of the upstream causes of the long-term chronic inflammation observed in CF. Although this study was done with a limited number of mice, we believe that it identifies many differences in the immune response to LPS between WT and CF that, at the very least, deserves further investigation.
Acknowledgments
The authors thank Naiqian Niu, Lesley Devine, Erica Herzog, Paul Clark, Stephanie Donaldson, Lauren Cohn, Ruslan Medzhitov, Anna van Heeckeren, Dominik Hartl, and Ee-chun Cheng for their significant contributions to these studies.
Notes
This work was supported by National Institutes of Health grants DK 53428 (M.E.E.), DK 61846 (D.S.K.), HL 73742 (D.S.K.), DK 72442 (D.S.K.), and by Cystic Fibrosis Foundation (CFF) grant EGAN06G0 (M.E.E.).
This article has an online supplement, which is accessible from this issue's table of contents at www.atsjournals.org
Originally Published in Press as DOI: 10.1165/rcmb.2008-0170OC on September 5, 2008
Conflict of Interest Statement: None of the authors has a financial relationship with a commercial entity that has an interest in the subject of this manuscript.
References
Articles from American Journal of Respiratory Cell and Molecular Biology are provided here courtesy of American Thoracic Society
Full text links
Read article at publisher's site: https://doi.org/10.1165/rcmb.2008-0170oc
Read article for free, from open access legal sources, via Unpaywall:
https://europepmc.org/articles/pmc2645527?pdf=render
Free after 12 months at intl-ajrcmb.atsjournals.org
http://intl-ajrcmb.atsjournals.org/cgi/content/full/40/3/295
Free to read at intl-ajrcmb.atsjournals.org
http://intl-ajrcmb.atsjournals.org/cgi/content/abstract/40/3/295
Free after 12 months at intl-ajrcmb.atsjournals.org
http://intl-ajrcmb.atsjournals.org/cgi/reprint/40/3/295.pdf
Citations & impact
Impact metrics
Citations of article over time
Alternative metrics
Article citations
Pseudomonas aeruginosa Lipid A Structural Variants Induce Altered Immune Responses.
Am J Respir Cell Mol Biol, 71(2):207-218, 01 Aug 2024
Cited by: 1 article | PMID: 38656811
Cystic fibrosis.
Nat Rev Dis Primers, 10(1):53, 08 Aug 2024
Cited by: 1 article | PMID: 39117676
Review
Analysis of CFTR mRNA and Protein in Peripheral Blood Mononuclear Cells via Quantitative Real-Time PCR and Western Blot.
Int J Mol Sci, 25(12):6367, 08 Jun 2024
Cited by: 0 articles | PMID: 38928073
MEK1/2 inhibition decreases pro-inflammatory responses in macrophages from people with cystic fibrosis and mitigates severity of illness in experimental murine methicillin-resistant Staphylococcus aureus infection.
Front Cell Infect Microbiol, 14:1275940, 30 Jan 2024
Cited by: 2 articles | PMID: 38352056 | PMCID: PMC10861668
Extracellular vesicles secreted by primary human bronchial epithelial cells reduce Pseudomonas aeruginosa burden and inflammation in cystic fibrosis mouse lung.
Am J Physiol Lung Cell Mol Physiol, 326(2):L164-L174, 12 Dec 2023
Cited by: 0 articles | PMID: 38084406 | PMCID: PMC11279747
Go to all (148) article citations
Data
Data behind the article
This data has been text mined from the article, or deposited into data resources.
BioStudies: supplemental material and supporting data
Similar Articles
To arrive at the top five similar articles we use a word-weighted algorithm to compare words from the Title and Abstract of each citation.
Azithromycin reduces exaggerated cytokine production by M1 alveolar macrophages in cystic fibrosis.
Am J Respir Cell Mol Biol, 41(5):590-602, 24 Feb 2009
Cited by: 75 articles | PMID: 19244203
Acute Pseudomonas challenge in cystic fibrosis mice causes prolonged nuclear factor-kappa B activation, cytokine secretion, and persistent lung inflammation.
J Allergy Clin Immunol, 117(5):1163-1169, 01 May 2006
Cited by: 24 articles | PMID: 16675347
Defective innate immunity and hyperinflammation in newborn cystic fibrosis transmembrane conductance regulator-knockout ferret lungs.
Am J Respir Cell Mol Biol, 52(6):683-694, 01 Jun 2015
Cited by: 71 articles | PMID: 25317669 | PMCID: PMC4491130
Harnessing Neutrophil Survival Mechanisms during Chronic Infection by Pseudomonas aeruginosa: Novel Therapeutic Targets to Dampen Inflammation in Cystic Fibrosis.
Front Cell Infect Microbiol, 7:243, 30 Jun 2017
Cited by: 11 articles | PMID: 28713772 | PMCID: PMC5492487
Review Free full text in Europe PMC
Funding
Funders who supported this work.
NHLBI NIH HHS (2)
Grant ID: R01 HL093004
Grant ID: HL 73742
NIDDK NIH HHS (4)
Grant ID: DK 72442
Grant ID: P30 DK072442
Grant ID: DK 61846
Grant ID: DK 53428