Abstract
Free full text

The geometry of leptin action in the brain: More complicated than a simple ARC
Summary
Leptin signals the repletion of fat stores, acting in the CNS to permit energy utilization by a host of autonomic and neuroendocrine processes and to decrease feeding. While much recent research has focused on the leptin-regulated circuitry of the hypothalamic arcuate nucleus (ARC), the majority of brain leptin receptor (LepRb)-expressing neurons lie outside the ARC in other brain regions known to modulate energy balance. Each set of LepRb neurons throughout the brain presumably mediates unique aspects of leptin action, and understanding the function for LepRb-expressing neurons throughout the brain represents a crucial next step in the study of energy homeostasis.
The physiologic response to negative energy balance
The naturalist Marston Bates famously observed that if evolution is a play, then ecology is the stage upon which it is performed (Bates, 1960). Periodic inadequacy of food supplies likely represented an important component of this setting for mammalian evolution, selecting for the abilities to store energy for later use and to promote food seeking and conserve energy when food and energy stores are limiting. Presumably as a result of such selection, states of low or decreasing fat stores increase the drive to feed and attenuate energy utilization on a variety of endocrine and autonomic processes.
Although a variety of signals convey information regarding energy stores and nutritional status, the adipocyte-derived hormone, leptin, plays a central role in mediating the response to energy sufficiency or deficit (Ahima et al., 2000). Circulating leptin levels mirror body fat stores, and leptin deficiency not only increases feeding, but (like fasting) decreases activity, sympathetic tone, and thyroid function, causes hypothalamic infertility, and impairs lactation (Elmquist JK et al., 1999; Ahima et al., 2000; Farooqi et al., 1999; Oral et al., 2002; Chehab FF, 1997). Conversely, treatment with exogenous leptin during caloric restriction or in the face of low body fat (e.g. anorexia nervosa, lipodystrophy syndromes) attenuates hunger, defends thyroid tone and cold tolerance, and supports reproductive function (Oral et al., 2002; Rosenbaum et al., 2002; Welt et al., 2004).
Leptin acts via LepRb in the CNS
While alternative mRNA splicing produces multiple isoforms of the leptin receptor (LepR), the LepRb form mediates physiologic leptin action (Chua, Jr. et al., 1996; Lee et al., 1996). Tissue-specific deletion and transgenic reconstitution experiments in rodents revealed the crucial role for CNS LepRb for leptin effects on feeding and overall energy balance (de Luca et al., 2005; Cohen et al., 2001).
Numerous populations of LepRb-expressing neurons exist in the brain, and areas with particularly high LepRb expression include several regions of the hypothalamus, midbrain, and brainstem that play important roles in energy balance (Elmquist et al., 1998b; Leshan et al., 2006). For instance, LepRb is expressed in the LHA, and ablation of the lateral hypothalamic area (LHA) attenuates food intake, suggesting an important role in the promotion of feeding (Kelley et al., 2005; Elmquist JK et al., 1999). Also, while the surgical transection of forebrain projections to the brainstem impairs many behaviors, these decerebrate animals will consume food that is placed in their oral cavity and will modulate the amount of food that they ingest in response to a variety of hormonal and gut-derived neural cues- suggesting important roles for the hindbrain in the regulation of feeding (Grill, 2006).
Electrolytic lesions of structures in the medial basal hypothalamus (MBH, including the ARC and ventromedial hypothalamic nucleus (VMH)) results in hyperphagia and obesity, suggesting roles for these nuclei in limiting feeding (Elmquist JK et al., 1999). The more circumscribed chemical lesions produced by gold thioglucose (lateral ARC and VMH) and monosodium glutamate (medial basal ARC) also produce dramatic hyperphagia (Bergen et al., 1998). The restoration of LepRb expression in the MBH of LepRb-null animals diminishes the hyperphagia, obesity and hyperglycemia of these animals, consistent with an important role for MBH leptin action (Coppari et al., 2005; Morton et al., 2003).
The ARC POMC/AgRP circuit mediates a portion of leptin action
While other brain regions have received less attention over the past decade, the study of ARC neuropeptidergic systems has become a major focus for energy balance research. At least two distinct populations of ARC neurons express LepRb: a leptin-activated set of neurons that expresses pro-opiomelanocortin (POMC) and an opposing, leptin-inhibited population that co-expresses neuropeptide Y (NPY) and agouti related peptide (AgRP) (Elmquist et al., 2005; Morton et al., 2006). Leptin action on this “ARC POMC/AgRP circuit” attenuates feeding (Elmquist et al., 2005; Morton et al., 2006). Interference with POMC action in the brain (e.g. by deletion of POMC or the downstream melanocortin 4 receptor (MC4R)) or ablation of POMC neurons produces dramatic hyperphagia (albeit without many of the neuroendocrine sequelae of leptin deficiency, such as infertility and hypercortisolism) (Smart et al., 2006; Butler and Cone, 2002; Morton et al., 2006). Conversely, ablation of the AgRP/NPY neuron dramatically diminishes feeding (Gropp et al., 2005; Luquet et al., 2005). The ARC POMC/AgRP circuit thus represents an important and well-understood circuit; it is also genetically tractable (e.g., by transgenic manipulation) due to the essentially ARC-restricted pattern of POMC and AgRP expression within the CNS (van de et al., 2008; Butler and Cone, 2002; Elmquist et al., 2005; Kim et al., 2000).
Of course, not all of the actions of the ARC POMC/AgRP circuit are attributable to leptin: Indeed, not all POMC neurons express functional LepRb (Munzberg et al., 2003). Furthermore, many factors other than leptin, such as the gut peptide, ghrelin (which opposes leptin by activating AgRP/NPY neurons) also regulate these neurons (Morton et al., 2006; Butler and Cone, 2002; Chen et al., 2004; Tschop et al., 2006). Also, insulin-mediated phosphatidylinositol 3′-kinase signaling (PI3K, which is also modulated by leptin) regulates the membrane potential of POMC and AgRP neurons and PI3K in these neurons contributes importantly to the modulation of energy balance and glucose homeostasis (Niswender et al., 2004; Rother et al., 2008; Hill et al., 2008b). Glucose sensing by POMC neurons also contributes to the regulation of these neurons and to the control of glucose homeostasis (Parton et al., 2007).
Leptin action outside the ARC POMC/AgRP circuit
It is also clear that this POMC/AgRP circuit alone cannot account for the majority of leptin action in vivo. For instance, the disruption of Lepr in POMC or AgRP and POMC neurons results in modest obesity compared to that of Leprdb/db animals (Balthasar et al., 2004) (van de et al., 2008), and does not cause infertility or hypercortisolism, as is observed in Leprdb/db animals. Since ARC POMC and AgRP neurons account for only a portion of the overall leptin effect, other LepRb-expressing neurons must mediate crucial components of leptin action. Indeed, ARC LepRb neurons together comprise a minority of the total CNS LepRb neurons (Elmquist et al., 1998b; Leshan et al., 2006).
Given the pleiotrophic effects of leptin, it is unreasonable to expect that a single neural circuit (such as the POMC/AgRP circuit) or a single nucleus (like the ARC) could mediate all- or even the majority- of leptin action. Each region and subregion of the brain contains neurons with specific functional properties that dictate the physiological outputs they control. For instance, while the ARC operates together with other medial hypothalamic and brainstem sites to regulate satiety (the perception of fullness that terminates feeding) (Woods et al., 2000), the incentive (hedonic or rewarding) value of food is largely encoded by the ventral tegmental area (VTA) and striatum, which comprise the core of the mesolimbic dopamine (DA) system (Figlewicz et al., 2006; Kelley et al., 2005).
Leptin Regulation of the PVH
Although ARC neurons project to a number of other regions in the hypothalamus, they densely innervate the paraventricular hypothalamic nucleus (PVH), which regulates autonomic and neuroendocrine (e.g. thyroid, adrenal) function to modulate energy expenditure, as well as contributing to satiety (Elmquist et al., 2005; Butler and Cone, 2002). PVH-specific reactivation of the MC4R (which mediates crucial responses to the ARC POMC neurons) attenuates the hyperphagia of MC4R-null animals (Balthasar et al., 2005). Other MC4R-expressing neurons must contribute to the regulation of the autonomic nervous system, however, since PVH reactivation of MC4R fails to normalize autonomic control. Together with the known role for the PVH in autonomic regulation, these findings also hint that melanocortin-independent mechanisms likely contribute to the regulation of autonomic function via the PVH. Indeed, inputs from non-ARC areas that contain substantial populations of LepRb neurons (e.g. the VMH and the DMH) regulate the ARC and/or PVH, modifying the output of the circuit (Thompson and Swanson, 2003; Elmquist et al., 1998a). For instance, VMH neurons contribute excitatory projections onto ARC POMC neurons and the inhibitory/excitatory tone of these inputs varies with leptin levels and nutritional status (Sternson et al., 2005; Pinto et al., 2004). In addition to receiving copious projections from the ARC, the DMH densely innervates the PVH (as well as with several other regions of the hypothalamus), and DMH lesions alter feeding (Thompson and Swanson, 2003; Zaretskaia et al., 2008; Elmquist JK et al., 1999).
The PVH and its surrounding circuitry present a reasonable (if simplified) model of the integrated leptin-responsive neural circuitry (Figure 1). Here, the VMH, DMH and ARC function together with the PVH as an interconnected circuit, with leptin presumably acting via LepRb-expressing neurons in each region to modulate PVH output. Indeed, direct leptin action on SF-1-expressing VMH LepRb neurons contributes to the regulation of energy balance (Dhillon et al., 2006; Bingham et al., 2008).
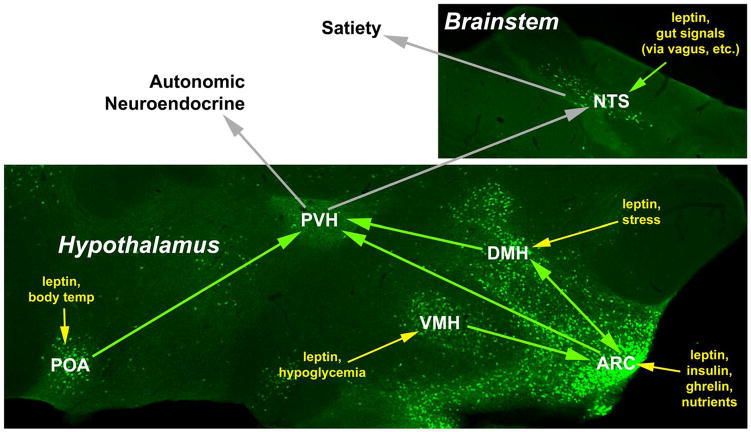
A simplified model “wiring” diagram of the medial hypothalamic circuitry as described in the text is shown superimposed upon an image of green fluorescent protein (GFP)-containing LepRb neurons (green) from sagittal sections of the medial hypothalamus and brainstem of mice that express GFP in LepRb neurons (Leshan et al., 2006). Each set of LepRb neurons responds not only to leptin, but also to additional stimuli. Thus, leptin acts at a number of points to enable the integration of signals of body energy status with other physiologic variables that dictate the strength of the final output signal. Note that while the PVH contains many GFP-reactive projections, few LepRb neurons reside in this output nucleus. Physiologic inputs to each nucleus are shown in yellow and putative projections from LepRb-containing neurons are denoted as green arrows.
Why should leptin act via so many different populations of LepRb neurons to regulate PVH output?
The central nervous system controls each aspect of energy intake and expenditure in the context of overall homeostatic need and external conditions (not just energy status); the repletion of fat stores must therefore be integrated with other information that reflects the requirement for the activity of a particular system in order to respond appropriately to the aggregate conditions. For instance, in addition to the permissive effect of leptin, the PVH output to the sympathetic nervous system must take into account temperature, cardiovascular status, stress, activity, and so forth, and must then generate the appropriate tone via specific branches of the autonomic nervous system. Hence, in the face of low energy stores, core body temperature may be allowed to decrease slightly to conserve energy, but a larger decrease in core body temperature must still promote a robust thermogenic response (while at the same time not altering cardiovascular homeostasis).
Each set of LepRb neurons within this ARC/VMH/DMH → PVH circuit presumably conveys specific information to the PVH. In this model of leptin action, each set of LepRb neurons (including those in the ARC) does not signal leptin levels alone, but rather transmits a signal that conveys information relevant to a specific set of inputs and outputs. For instance, the ARC senses rapid changes in insulin, ghrelin and circulating nutrients, as well as leptin, to signal acute peripheral nutritional status. The DMH, in contrast, is shielded from acute alterations in peripheral nutritional status by the blood-brain barrier (BBB) (Faouzi et al., 2007), but integrates (among other things) information about body temperature (from the preoptic area (POA)- where another set of LepRb neurons lie) and stress, along with leptin-encoded information about long-term energy status (Thompson and Swanson, 2003). The VMH is rich in glucose-sensing neurons and the LepRb neurons in this nucleus may also participate in the regulation of glucose homeostasis (Tong et al., 2007; King, 2006). Thus, each set of LepRb neurons encodes a distinct type of information, and the presence of LepRb on these neurons allows the integration of information regarding long-term energy stores into the aggregate signal mediated by each population of neurons.
Unfortunately, the current lack of markers (and thus neuron-specific cre-expressing mice) for LepRb neurons in the DMH and elsewhere limits the functional analysis of many components of this integrated leptin-regulated circuit. In addition to the ARC LepRb neurons, it will thus be important to define the neurotransmitter content, neuronal targets, and physiologic function of each set of LepRb neurons in the VMH, DMH, and ARC, as well as understanding the stimuli and mechanisms that regulate their activity and gene expression.
The regulation of brainstem satiety circuits
Satiety (the sensation of fullness that promotes meal termination) represents an important process by which leptin regulates feeding (Woods et al., 2000). Brainstem neural circuits (including the nucleus of the solitary tract (NTS)) encode a major component of satiety (Morton et al., 2006; Grill, 2006). The brainstem receives numerous inputs from gut peptides and the vagus, and can control meal size in the absence of descending projections (e.g., from the hypothalamus, including the PVH) (Grill, 2006). Leptin acts synergistically with the vagal actions of anorexigenic gut peptides like GLP-1 and cholecystokinin (CCK) to regulate the NTS and satiety (Huo et al., 2007; Morton et al., 2005; Williams et al., 2006). While the NTS contains LepRb neurons (Leshan et al., 2006; Elmquist et al., 1998b), it remains unclear whether these hindbrain LepRb neurons (as opposed to hypothalamic LepRb neurons that project directly or indirectly to the hindbrain) represent the major contributors to the regulation of hindbrain satiety circuits by leptin. Parsing this important issue will be crucial to understanding the regulation of satiety by leptin.
Leptin Regulation of the Mesolimbic Dopamine System
The incentive value of palatable food can override satiety signals to promote excess food ingestion (Figlewicz et al., 2006; Kelley et al., 2005). The most obvious analogy is that while one may be full after eating a meal, one often has dessert anyway, because eating tasty foods is pleasurable. Leptin regulates the incentive value of food (as well as of other addictive substances, such as drugs of abuse), and some of the neural mechanisms by which leptin may control food reward are beginning to be elucidated as investigators probe the interaction of leptin with the mesolimbic dopamine (DA) system (Carr, 2006; Fulton et al., 2006; Hommel et al., 2006; Figlewicz et al., 2006). At its core, the mesolimbic DA system contains of a set of DA neurons in the ventral tegmental area (VTA) that project forward to innervate the striatum (including the nucleus accumbens (NAc)), amygdala, and prefrontal cortex (PFC) (Figure 2). This system mediates the reinforcing effects of drugs of abuse, as well as the incentive salience of food and other natural rewards (Kelley et al., 2005; Nestler, 2005).
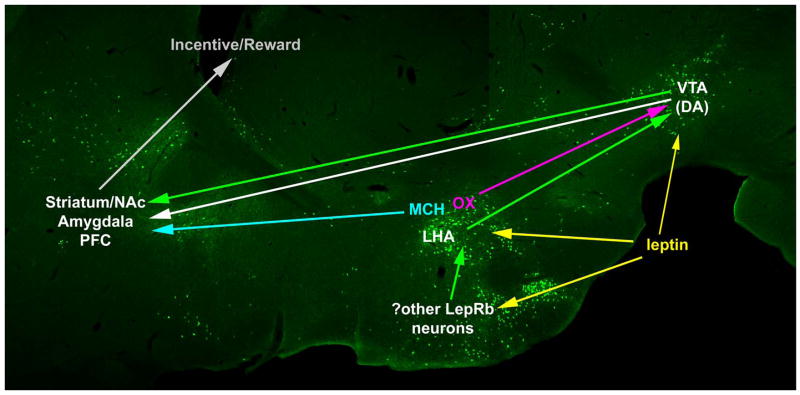
Components of the mesolimbic DA system are shown superimposed upon an image of GFP-containing LepRb neurons as in Figure 1. At its core, this system contains a set of DA neurons within the VTA and that project to the striatum (including the nucleus accumbens (NAc), amygdala, and PFC) to encode the incentive salience of natural and artificial rewards. Leptin may modify the activity of the mesolimbic DA system in a number of ways, including by the direct regulation of a LepRb-expressing population of VTA DA neruons. Additionally, leptin regulates the lateral hypothalamic area (LHA- a major upstream regulator of the mesolimbic DA system) via a number of mechanisms. In addition to containing a set of LepRb-expressing neurons, the LHA contains OX neurons (pink) that project to and regulate VTA DA neurons and MCH neurons (light blue) that project to the striatum. Putative projections from LepRb-containing neurons are denoted as green arrows.
ARC LepRb neurons do not project to the VTA, but systemic leptin administration modulates food reward and gene expression in the VTA (Figlewicz et al., 2006), suggesting a non-ARC locus for the regulation of DA signaling by leptin. A number of groups have reported the presence of LepRb-expressing VTA DA neurons, and have demonstrated the ability of leptin to modulate this system, (Fulton et al., 2006; Hommel et al., 2006; Figlewicz et al., 2006). Direct application of leptin to the VTA slightly inhibits VTA DA neurons in normal animals, suggesting that leptin might act directly on VTA LepRb neurons to block DA release (Hommel et al., 2006). In contrast, leptin-deficient Lepob/ob mice exhibit decreased DA and less VTA expression of the DA-producing enzyme, tyrosine hydroxylase (TH), and systemic leptin treatment restores TH and DA levels, suggesting that the chronic absence of leptin impairs the mesolimbic DA system (Fulton et al., 2006; Roseberry et al., 2007). Thus, leptin clearly promulgates a powerful signal to this system.
Several types of neurons in the LHA also respond to leptin and interact extensively with the mesolimbic DA system to regulate motivation and reward, including food reward (Fulton et al., 2000; Kelley et al., 2005; DiLeone et al., 2003). LHA orexin (OX) neurons project to the VTA and acute OX injection into the VTA promotes drug- and food-seeking (DiLeone et al., 2003; Harris et al., 2005). Leptin inhibits OX expression and the activity of OX neurons (presumably indirectly, via LepRb neurons residing elsewhere); this effect of leptin would presumably attenuate DA release by VTA neurons to decrease feeding (DiLeone et al., 2003; Harris et al., 2005). It is difficult to reconcile these data with the finding of mesolimbic DA suppression in Lepob/ob mice, however, since these animals display increased LHA OX expression and would therefore presumably exhibit enhanced mesolimbic DA signaling (Yamanaka et al., 2003).
Leptin also inhibits expression of the orexigenic LHA neuropeptide MCH (again, presumably indirectly), and may thus inhibit the MCH neurons that project to the NAc and promote feeding (Qu et al., 1996; Segal-Lieberman et al., 2003; Georgescu et al., 2005). Furthermore, we have identified a distinct population of LepRb-expressing neurons in the LHA that project to the VTA to regulate the mesolimbic DA system (our unpublished data). Thus, leptin acts via multiple pathways to control the mesolimbic DA system, and presumably to modulate the incentive salience of food. Furthermore, the bulk of these data suggest that the regulation of the mesolimbic DA system by leptin is more complex than the simple inhibition (or conversely, stimulation) of overall DA release. Rather, leptin appears to block DA signaling by some components of the VTA, while stimulating others. This presumably reflects the diverse functions of the various subpopulations of VTA DA neurons, themselves, along with the need for leptin to promote certain reward-directed behaviours (e.g. reproduction), while attenuating hedonic feeding. Part of the confusion regarding the action of leptin on the mesolimbic DA system probably derives from the complex relationship between DA release and behavior. While conventional wisdom suggests that DA correlates with reward-seeking behaviors, striatal dopamine release does not always promote increased feeding: Indeed, acutely increasing DA availability, as by cocaine or amphetamine, blunts feeding while promoting sexual behavior.
Going forward, it will be important to define the populations of LepRb neurons that directly or indirectly regulate the mesolimbic DA system, as well as the roles that each of these neural mediators of leptin action play in the regulation of distinct aspects of mesolimbic DA system function and in energy balance.
Leptin Action in Reproduction
The energetic cost of reproduction is very high, especially for females, who bear the nutritional burdens of fetal development and lactations, and energy stores must be adequate for reproduction. Leptin communicates the sufficiency of energy stores for reproduction by promoting the activity of gonadotropin-releasing hormone (GnRH)-secreting neurons; these neurons in turn stimulating the release of pituitary gonadotropins into the circulation. GnRH neurons do not express LepRb, however, indicating that leptin must act indirectly/trans-synaptically to regulate GnRH secretion (Nagatani S et al., 1998).
Kisspeptin-containing hypothalamic neurons have recently emerged as crucial regulators of GnRH neurons and the hypothalamic reproductive axis; indeed, the loss of kisspeptin action results in hypothalamic infertility (Gottsch et al., 2006; Tena-Sempere, 2006). One population of kisspeptin neurons express LepRb (Smith et al., 2006), but the large population of kisspeptin neurons that appears to be responsible for the ovulatory GnRH surge in females does not overlap with the distribution of LepRb neurons. Thus, while some potential regulation of kisspeptin could be direct, while other mechanisms would be indirect.
In order to fully reveal the mechanisms linking energy balance to fertility, it will be important to define the populations of LepRb neurons that directly or indirectly innervate the GnRH and kisspeptin-containing neurons. The ventral premammilary nucleus (PMv) in particular contains a large number of LepRb neurons and densely innervates GnRH- and kisspeptin-containing brain regions (Canteras et al., 1992). Additionally, sexual cues activate neurons in the PMv and lesions of the PMv interfere with the hypothalamic control of reproduction (Cavalcante et al., 2006; Simerly, 1998; Hill et al., 2008a). Thus, while multiple populations of LepRb neurons may modulate the reproductive axis, PMv LepRb neurons are likely candidates to contribute to this process.
Other substantial populations of LepRb neurons in the brain
In addition to the LepRb-expressing neurons noted above, other brain regions contain substantial populations of LepRb neurons. Included among these are the midbrain raphe nuclei, substantia nigra (SN), and periaqueductal grey matter (PAG), as well as other brainstem sites (Leshan et al., 2006; Elmquist et al., 1998b). While the LepRb neurons in these regions remain substantially uncharacterized in terms of phenotype and function, the expression of LepRb in each of these locations could at least make teleological sense in the context of energy balance. For instance, the SN controls the initiation of movement-a process one would expect to modulated by energy status. As importantly, LepRb expression is not ubiquitous: Few cortical or hippocampal neurons express LepRb (Leshan et al., 2006; Elmquist et al., 1998b). This restricted pattern of LepRb expression is consistent with the notion that leptin acts primarily at sites that integrate information to modulate processes that impact energy balance, as opposed to in higher cognitive centers or in areas that directly effect physiologic outputs (e.g. motor neurons).
The neural basis of leptin action
Overall, while the ARC POMC/AgRP circuit remains an important site of leptin action, it is unreasonable to ascribe the majority of leptin action to this single circuit. Other important sites of direct leptin action in the brain have emerged, and many more require additional study. Each population of LepRb-expressing neurons presumably functions to integrate the leptin signal with other inputs crucial to the regulation of specific physiologic processes and thus contributes uniquely to the overall leptin signal. Developing a comprehensive understanding of leptin action will require elucidating the neuronal phenotype, regulation, and physiologic functions for each of the diverse LepRb-expressing neural populations in the CNS.
Acknowledgments
Supported by NIH DK57768 and DK78056 (to MGM), and grants from the American Diabetes Association (to MGM) and American Heart Association (to RLL, HM and MGM).
Reference List
- Ahima RS, Saper CB, Flier JS, Elmquist JK. Leptin regulation of neuroendocrine systems. Front Neuroendocrinol. 2000;21:263–307. [Abstract] [Google Scholar]
- Balthasar N, Coppari R, McMinn J, Liu SM, Lee CE, Tang V, Kenny CD, McGovern RA, Chua SC, Jr, Elmquist JK, Lowell BB. Leptin Receptor Signaling in POMC Neurons Is Required for Normal Body Weight Homeostasis. Neuron. 2004;42:983–991. [Abstract] [Google Scholar]
- Balthasar N, Dalgaard LT, Lee CE, Yu J, Funahashi H, Williams T, Ferreira M, Tang V, McGovern RA, Kenny CD, Christiansen LM, Edelstein E, Choi B, Boss O, Aschkenasi C, Zhang CY, Mountjoy K, Kishi T, Elmquist JK, Lowell BB. Divergence of melanocortin pathways in the control of food intake and energy expenditure. Cell. 2005;123:493–505. [Abstract] [Google Scholar]
- Bates M. Forest & the Sea a Study of the Economy of Nature and the Ecology of Man. Random House 1960 [Google Scholar]
- Bergen HT, Mizuno TM, Taylor J, Mobbs CV. Hyperphagia and weight gain after gold-thioglucose: relation to hypothalamic neuropeptide Y and proopiomelanocortin. Endocrinology. 1998;139:4483–4488. [Abstract] [Google Scholar]
- Bingham NC, Anderson KK, Reuter AL, Stallings NR, Parker KL. Selective loss of leptin receptors in the ventromedial hypothalamic nucleus results in increased adiposity and a metabolic syndrome. Endocrinology. 2008;149:2138–2148. [Europe PMC free article] [Abstract] [Google Scholar]
- Butler AA, Cone RD. The melanocortin receptors: lessons from knockout models. Neuropeptides. 2002;36:77–84. [Abstract] [Google Scholar]
- Canteras NS, Simerly RB, Swanson LW. Projections of the ventral premammillary nucleus. J Comp Neurol. 1992;324:195–212. [Abstract] [Google Scholar]
- Carr KD. Chronic food restriction: Enhancing effects on drug reward and striatal cell signaling. Physiol Behav 2006 [Abstract] [Google Scholar]
- Cavalcante JC, Bittencourt JC, Elias CF. Female odors stimulate CART neurons in the ventral premammillary nucleus of male rats. Physiol Behav. 2006;88:160–166. [Abstract] [Google Scholar]
- Chehab FF. The reproductive side of leptin. Nature Medicine. 1997;3:952–953. [Abstract] [Google Scholar]
- Chen HY, Trumbauer ME, Chen AS, Weingarth DT, Adams JR, Frazier EG, Shen Z, Marsh DJ, Feighner SD, Guan XM, Ye Z, Nargund RP, Smith RG, Van Der Ploeg LH, Howard AD, MacNeil DJ, Qian S. Orexigenic action of peripheral ghrelin is mediated by neuropeptide Y and agouti-related protein. Endocrinology. 2004;145:2607–2612. [Abstract] [Google Scholar]
- Chua SC, Jr, Chung WK, Wu-Peng XS, Zhang Y, Liu SM, Tartaglia LA, Leibel RL. Phenotypes of mouse diabetes and rat fatty due to mutations in the OB (Leptin) receptor. Science. 1996;271:994–996. [Abstract] [Google Scholar]
- Cohen P, Zhao C, Cai X, Montez JM, Rohani SC, Feinstein P, Mombaerts P, Friedman JM. Selective deletion of leptin receptor in neurons leads to obesity. J Clin Invest. 2001;108:1113–1121. [Europe PMC free article] [Abstract] [Google Scholar]
- Coppari R, Ichinose M, Lee CE, Pullen AE, Kenny CD, McGovern RA, Tang V, Liu SM, Ludwig T, Chua SC, Jr, Lowell BB, Elmquist JK. The hypothalamic arcuate nucleus: a key site for mediating leptin’s effects on glucose homeostasis and locomotor activity. Cell Metab. 2005;1:63–72. [Abstract] [Google Scholar]
- de Luca C, Kowalski TJ, Zhang Y, Elmquist JK, Lee C, Kilimann MW, Ludwig T, Liu SM, Chua SC., Jr Complete rescue of obesity, diabetes, and infertility in db/db mice by neuron-specific LEPR-B transgenes. J Clin Invest. 2005;115:3484–3493. [Abstract] [Google Scholar]
- Dhillon H, Zigman JM, Ye C, Lee CE, McGovern RA, Tang V, Kenny CD, Christiansen LM, White RD, Edelstein EA, Coppari R, Balthasar N, Cowley MA, Chua S, Jr, Elmquist JK, Lowell BB. Leptin directly activates SF1 neurons in the VMH, and this action by leptin is required for normal body-weight homeostasis. Neuron. 2006;49:191–203. [Abstract] [Google Scholar]
- DiLeone RJ, Georgescu D, Nestler EJ. Lateral hypothalamic neuropeptides in reward and drug addiction. Life Sci. 2003;73:759–768. [Abstract] [Google Scholar]
- Elmquist JK, Elias CF, Saper CB. From lesions to leptin: hypothalamic control of food intake and body weight. Neuron. 1999;22:221–232. [Abstract] [Google Scholar]
- Elmquist JK, Ahima RS, Elia CF, Flier JS, Saper CB. Leptin activates distinct projections from the dorsomedial and ventromedial hypothalamic nuclei. Proc Natl Acad Sci U S A. 1988a;95:741–746. [Europe PMC free article] [Abstract] [Google Scholar]
- Elmquist JK, Bjorbaek C, Ahima RS, Flier JS, Saper CB. Distributions of leptin receptor mRNA isoforms in the rat brain. J Comp Neurol. 1998b;395:535–547. [Abstract] [Google Scholar]
- Elmquist JK, Coppari R, Balthasar N, Ichinose M, Lowell BB. Identifying hypothalamic pathways controlling food intake, body weight, and glucose homeostasis. J Comp Neurol. 2005;493:63–71. [Abstract] [Google Scholar]
- Faouzi M, Leshan R, Bjornholm M, Hennessey T, Jones J, Munzberg H. Differential Accessibility of Circulating Leptin to Individual Hypothalamic Sites. Endocrinology 2007 [Abstract] [Google Scholar]
- Farooqi IS, Jebb SA, Langmack G, Lawrence E, Cheetham CH, Prentice AM, Hughes IA, McCamish MA, O’Rahilly S. Effects of recombinant leptin therapy in a child with congenital leptin deficiency. N Engl J Med. 1999;341:879–884. [Abstract] [Google Scholar]
- Figlewicz DP, Naleid AM, Sipols AJ. Modulation of food reward by adiposity signals. Physiol Behav 2006 [Europe PMC free article] [Abstract] [Google Scholar]
- Fulton S, Pissios P, Manchon RP, Stiles L, Frank L, Pothos EN, Maratos-Flier E, Flier JS. Leptin regulation of the mesoaccumbens dopamine pathway. Neuron. 2006;51:811–822. [Abstract] [Google Scholar]
- Fulton S, Woodside B, Shizgal P. Modulation of brain reward circuitry by leptin. Science. 2000;287:125–128. [Abstract] [Google Scholar]
- Georgescu D, Sears RM, Hommel JD, Barrot M, Bolanos CA, Marsh DJ, Bednarek MA, Bibb JA, Maratos-Flier E, Nestler EJ, DiLeone RJ. The hypothalamic neuropeptide melanin-concentrating hormone acts in the nucleus accumbens to modulate feeding behavior and forced-swim performance. J Neurosci. 2005;25:2933–2940. [Abstract] [Google Scholar]
- Gottsch ML, Clifton DK, Steiner RA. Kisspepeptin-GPR54 signaling in the neuroendocrine reproductive axis. Mol Cell Endocrinol. 2006;254–255:91–96. [Abstract] [Google Scholar]
- Grill HJ. Distributed neural control of energy balance: contributions from hindbrain and hypothalamus. Obesity (Silver Spring) 2006;14(Suppl 5):216S–221S. [Abstract] [Google Scholar]
- Gropp E, Shanabrough M, Borok E, Xu AW, Janoschek R, Buch T, Plum L, Balthasar N, Hampel B, Waisman A, Barsh GS, Horvath TL, Bruning JC. Agouti-related peptide-expressing neurons are mandatory for feeding. Nat Neurosci. 2005;8:1289–1291. [Abstract] [Google Scholar]
- Harris GC, Wimmer M, Aston-Jones G. A role for lateral hypothalamic orexin neurons in reward seeking. Nature. 2005;437:556–559. [Abstract] [Google Scholar]
- Hill JW, Elmquist JK, Elias CF. Hypothalamic pathways linking energy balance and reproduction. Am J Physiol Endocrinol Metab. 2008a;294:E827–E832. [Abstract] [Google Scholar]
- Hill JW, Williams KW, Ye C, Luo J, Balthasar N, Coppari R, Cowley MA, Cantley LC, Lowell BB, Elmquist JK. Acute effects of leptin require PI3K signaling in hypothalamic proopiomelanocortin neurons in mice. J Clin Invest. 2008b;118:1796–1805. [Abstract] [Google Scholar]
- Hommel JD, Trinko R, Sears RM, Georgescu D, Liu ZW, Gao XB, Thurmon JJ, Marinelli M, DiLeone RJ. Leptin receptor signaling in midbrain dopamine neurons regulates feeding. Neuron. 2006;51:801–810. [Abstract] [Google Scholar]
- Huo L, Maeng L, Bjorbaek C, Grill HJ. Leptin and the Control of Food Intake: Neurons in the Nucleus of the Solitary Tract Are Activated by Both Gastric Distension and Leptin. Endocrinology. 2007;148:2189–2197. [Abstract] [Google Scholar]
- Kelley AE, Baldo BA, Pratt WE. A proposed hypothalamic-thalamic-striatal axis for the integration of energy balance, arousal, and food reward. J Comp Neurol. 2005;493:72–85. [Abstract] [Google Scholar]
- Kim YB, Uotani S, Pierroz DD, Flier JS, Kahn BB. In vivo administration of leptin activates signal transduction directly in insulin-sensitive tissues: overlapping but distinct pathways from insulin. Endocrinology. 2000;141:2328–2339. [Abstract] [Google Scholar]
- King BM. The rise, fall, and resurrection of the ventromedial hypothalamus in the regulation of feeding behavior and body weight. Physiol Behav. 2006;87:221–244. [Abstract] [Google Scholar]
- Lee GH, Proenca R, Montez JM, Carroll KM, Darvishzadeh JG, Lee JI, Friedman JM. Abnormal splicing of the leptin receptor in diabetic mice. Nature. 1996;379:632–635. [Abstract] [Google Scholar]
- Leshan RL, Bjornholm M, Munzberg H, Myers MG., Jr Leptin receptor signaling and action in the central nervous system. Obesity (Silver Spring) 2006;14(Suppl 5):208S–212S. [Abstract] [Google Scholar]
- Luquet S, Perez FA, Hnasko TS, Palmiter RD. NPY/AgRP neurons are essential for feeding in adult mice but can be ablated in neonates. Science. 2005;310:683–685. [Abstract] [Google Scholar]
- Morton GJ, Blevins JE, Williams DL, Niswender KD, Gelling RW, Rhodes CJ, Baskin DG, Schwartz MW. Leptin action in the forebrain regulates the hindbrain response to satiety signals. J Clin Invest. 2005;115:703–710. [Abstract] [Google Scholar]
- Morton GJ, Cummings DE, Baskin DG, Barsh GS, Schwartz MW. Central nervous system control of food intake and body weight. Nature. 2006;443:289–295. [Abstract] [Google Scholar]
- Morton GJ, Niswender KD, Rhodes CJ, Myers MG, Jr, Blevins JT, Baskin DG, Schwartz MW. Arcuate nucleus-specific leptin receptor gene therapy attenuates the obesity phenotype of Koletsky (fak/fak) rats. Endocrinology. 2003;144:2016–2024. [Abstract] [Google Scholar]
- Munzberg H, Huo L, Nillni EA, Hollenberg AN, Bjorbaek C. Role of signal transducer and activator of transcription 3 in regulation of hypothalamic proopiomelanocortin gene expression by leptin. Endocrinology. 2003;144:2121–2131. [Abstract] [Google Scholar]
- Nagatani S, Guthikonda P, Thompson RC, Tsukamura H, Maeda KI, Foster DL. Evidence for GnRH regulation by leptin: leptin administration prevents reduced pulsatile LH secretion during fasting. Neuroendocrinology. 1998;67:370–376. [Abstract] [Google Scholar]
- Nestler EJ. Is there a common molecular pathway for addiction? Nat Neurosci. 2005;8:1445–1449. [Abstract] [Google Scholar]
- Niswender KD, Baskin DG, Schwartz MW. Insulin and its evolving partnership with leptin in the hypothalamic control of energy homeostasis. Trends Endocrinol Metab. 2004;15:362–369. [Abstract] [Google Scholar]
- Oral EA, Simha V, Ruiz E, Andewelt A, Premkumar A, Snell P, Wagner AJ, Depaoli AM, Reitman ML, Taylor SI, Gorden P, Garg A. Leptin-replacement therapy for lipodystrophy. N Engl J Med. 2002;346:570–578. [Abstract] [Google Scholar]
- Parton LE, Ye CP, Coppari R, Enriori PJ, Choi B, Zhang CY, Xu C, Vianna CR, Balthasar N, Lee CE, Elmquist JK, Cowley MA, Lowell BB. Glucose sensing by POMC neurons regulates glucose homeostasis and is impaired in obesity. Nature. 2007;449:228–232. [Abstract] [Google Scholar]
- Pinto S, Roseberry AG, Liu H, Diano S, Shanabrough M, Cai X, Friedman JM, Horvath TL. Rapid rewiring of arcuate nucleus feeding circuits by leptin. Science. 2004;304:110–115. [Abstract] [Google Scholar]
- Qu D, Ludwig DS, Gammeltoft S, Piper M, Pelleymounter MA, Cullen MJ, Mathes WF, Przypek J, Kanarek R, Maratos-Flier E. A role for melanin-concentrating hormone in the central regulation of feeding behavior. Nature. 1996;380:243–247. [Abstract] [Google Scholar]
- Roseberry AG, Painter T, Mark GP, Williams JT. Decreased vesicular somatodendritic dopamine stores in leptin-deficient mice. J Neurosci. 2007;27:7021–7027. [Abstract] [Google Scholar]
- Rosenbaum M, Murphy EM, Heymsfield SB, Matthews DE, Leibel RL. Low dose leptin administration reverses effects of sustained weight-reduction on energy expenditure and circulating concentrations of thyroid hormones. J Clin Endocrinol Metab. 2002;87:2391–2394. [Abstract] [Google Scholar]
- Rother E, Konner AC, Bruning JC. Neurocircuits integrating hormone and nutrient signaling in control of glucose metabolism. Am J Physiol Endocrinol Metab. 2008;294:E810–E816. [Abstract] [Google Scholar]
- Segal-Lieberman G, Bradley RL, Kokkotou E, Carlson M, Trombly DJ, Wang X, Bates S, Myers MG, Jr, Flier JS, Maratos-Flier E. Melanin-concentrating hormone is a critical mediator of the leptin-deficient phenotype. Proc Natl Acad Sci U S A. 2003;100:10085–10090. [Europe PMC free article] [Abstract] [Google Scholar]
- Simerly RB. Organization and regulation of sexually dimorphic neuroendocrine pathways. Behav Brain Res. 1998;92:195–203. [Abstract] [Google Scholar]
- Smart JL, Tolle V, Low MJ. Glucocorticoids exacerbate obesity and insulin resistance in neuron-specific proopiomelanocortin-deficient mice. J Clin Invest. 2006;116:495–505. [Abstract] [Google Scholar]
- Smith JT, Acohido BV, Clifton DK, Steiner RA. KiSS-1 neurones are direct targets for leptin in the ob/ob mouse. J Neuroendocrinol. 2006;18:298–303. [Abstract] [Google Scholar]
- Sternson SM, Shepherd GM, Friedman JM. Topographic mapping of VMH --> arcuate nucleus microcircuits and their reorganization by fasting. Nat Neurosci. 2005;8:1356–1363. [Abstract] [Google Scholar]
- Tena-Sempere M. KiSS-1 and reproduction: focus on its role in the metabolic regulation of fertility. Neuroendocrinology. 2006;83:275–281. [Abstract] [Google Scholar]
- Thompson RH, Swanson LW. Structural characterization of a hypothalamic visceromotor pattern generator network. Brain Res Brain Res Rev. 2003;41:153–202. [Abstract] [Google Scholar]
- Tong Q, Ye C, McCrimmon RJ, Dhillon H, Choi B, Kramer MD, Yu J, Yang Z, Christiansen LM, Lee CE, Choi CS, Zigman JM, Shulman GI, Sherwin RS, Elmquist JK, Lowell BB. Synaptic glutamate release by ventromedial hypothalamic neurons is part of the neurocircuitry that prevents hypoglycemia. Cell Metab. 2007;5:383–393. [Europe PMC free article] [Abstract] [Google Scholar]
- Tschop MH, Castaneda TR, Woods SC. The brain is getting ready for dinner. Cell Metab. 2006;4:257–258. [Abstract] [Google Scholar]
- van de WE, Leshan R, Xu AW, Balthasar N, Coppari R, Liu SM, Jo YH, MacKenzie RG, Allison DB, Dun NJ, Elmquist J, Lowell BB, Barsh GS, de Luca C, Myers MG, Jr, Schwartz GJ, Chua SC., Jr Collective and individual functions of leptin receptor modulated neurons controlling metabolism and ingestion. Endocrinology. 2008;149:1773–1785. [Europe PMC free article] [Abstract] [Google Scholar]
- Welt CK, Chan JL, Bullen J, Murphy R, Smith P, Depaoli AM, Karalis A, Mantzoros CS. Recombinant human leptin in women with hypothalamic amenorrhea. N Engl J Med. 2004;351:987–997. [Abstract] [Google Scholar]
- Williams DL, Baskin DG, Schwartz MW. Leptin regulation of the anorexic response to glucagon-like peptide-1 receptor stimulation. Diabetes. 2006;55:3387–3393. [Abstract] [Google Scholar]
- Woods SC, Schwartz MW, Baskin DG, Seeley RJ. Food intake and the regulation of body weight. Annu Rev Psychol. 2000;51:255–277. [Abstract] [Google Scholar]
- Yamanaka A, Beuckmann CT, Willie JT, Hara J, Tsujino N, Mieda M, Tominaga M, Yagami K, Sugiyama F, Goto K, Yanagisawa M, Sakurai T. Hypothalamic orexin neurons regulate arousal according to energy balance in mice. Neuron. 2003;38:701–713. [Abstract] [Google Scholar]
- Zaretskaia MV, Zaretsky DV, Sarkar S, Shekhar A, DiMicco JA. Induction of Fos-immunoreactivity in the rat brain following disinhibition of the dorsomedial hypothalamus. Brain Res. 2008;1200:39–50. [Europe PMC free article] [Abstract] [Google Scholar]
Full text links
Read article at publisher's site: https://doi.org/10.1016/j.cmet.2008.12.001
Read article for free, from open access legal sources, via Unpaywall:
http://www.cell.com/article/S1550413108003860/pdf
Citations & impact
Impact metrics
Citations of article over time
Smart citations by scite.ai
Explore citation contexts and check if this article has been
supported or disputed.
https://scite.ai/reports/10.1016/j.cmet.2008.12.001
Article citations
A subset of dopamine receptor-expressing neurons in the nucleus accumbens controls feeding and energy homeostasis.
Nat Metab, 6(8):1616-1631, 15 Aug 2024
Cited by: 1 article | PMID: 39147933 | PMCID: PMC11349581
Leptin signaling and its central role in energy homeostasis.
Front Neurosci, 17:1238528, 31 Oct 2023
Cited by: 3 articles | PMID: 38027481 | PMCID: PMC10644276
Review Free full text in Europe PMC
Altered function of arcuate leptin receptor expressing neuropeptide Y neurons depending on energy balance.
Mol Metab, 76:101790, 09 Aug 2023
Cited by: 0 articles | PMID: 37562743 | PMCID: PMC10460992
Identification of a GABAergic neural circuit governing leptin signaling deficiency-induced obesity.
Elife, 12:e82649, 12 Apr 2023
Cited by: 4 articles | PMID: 37043384 | PMCID: PMC10097419
The neural addiction of cancer.
Nat Rev Cancer, 23(5):317-334, 11 Apr 2023
Cited by: 13 articles | PMID: 37041409
Review
Go to all (179) article citations
Similar Articles
To arrive at the top five similar articles we use a word-weighted algorithm to compare words from the Title and Abstract of each citation.
The Role of PVH Circuits in Leptin Action and Energy Balance.
Annu Rev Physiol, 78:207-221, 01 Jan 2016
Cited by: 60 articles | PMID: 26863324 | PMCID: PMC5087283
Review Free full text in Europe PMC
Minireview: CNS Mechanisms of Leptin Action.
Mol Endocrinol, 30(1):3-12, 20 Oct 2015
Cited by: 87 articles | PMID: 26484582 | PMCID: PMC4695630
Review Free full text in Europe PMC
A spontaneous leptin receptor point mutation causes obesity and differentially affects leptin signaling in hypothalamic nuclei resulting in metabolic dysfunctions distinct from db/db mice.
Mol Metab, 25:131-141, 25 Apr 2019
Cited by: 10 articles | PMID: 31076350 | PMCID: PMC6601129
The temporal and spatial pattern of leptin receptor-expressing cells in the developing mouse hypothalamus.
J Neuroendocrinol, 36(2):e13366, 27 Jan 2024
Cited by: 0 articles | PMID: 38279680
Funding
Funders who supported this work.
NIDDK NIH HHS (8)
Grant ID: DK78056
Grant ID: R01 DK078056-02
Grant ID: R37 DK056731
Grant ID: R01 DK057768-09
Grant ID: DK57768
Grant ID: R01 DK057768
Grant ID: R01 DK078056
Grant ID: R37 DK056731-10