Abstract
Free full text

Breathtaking TRP Channels: TRPA1 and TRPV1 in Airway Chemosensation and Reflex Control
Abstract
New studies have revealed an essential role for TRPA1, a sensory neuronal TRP ion channel, in airway chemosensation and inflammation. TRPA1 is activated by chlorine, reactive oxygen species and noxious constituents of smoke and smog, initiating irritation and airway reflex responses. Together with TRPV1, the capsaicin receptor, TRPA1 may contribute to chemical hypersensitivity, chronic cough and airway inflammation in asthma, COPD and reactive airway dysfunction syndrome.
Airway Chemosensation and Reflex Responses
The airways are highly sensitive to damage by airborne reactive chemicals, toxic particulates and infectious agents. Airway reflex responses such as cough and sneezing are crucial for the protection of the airways from chemical and biological challenges (33). Cough and sneezing are triggered by activation of peripheral sensory nerve endings in the airway lining. The airways are innervated by both chemosensory nerves, detecting a large variety of noxious chemicals, and mechanosensory nerves that constantly measure the tension of airway tissue and participate in respiratory feedback control.
Trigeminal chemosensory nerve endings in the nasal mucosa are in the first line of defense against noxious chemical challenges. Trigeminal excitation initiates the sensation of irritation and pain and initiates sneezing (10). Sensory-autonomic parasympathetic efferent reflex pathways induce secretions from nasal, lacrimatory and salivary glands, and the dilation of vessels in the nasal mucosa and sinuses. Neuropeptides such as Substance P and CGRP, released from chemically stimulated nerve endings, promote neurogenic inflammatory vasodilation and leakage, contributing to narrowing or obstruction of the nasal passages (11, 102). Chemical stimulation of vagal sensory fibers terminating in the glottis and larynx trigger the cough reflex that, similar to sneezing, promotes the extrusion of air, particulates and mucus from the airways. When irritants reach the lower airways, sensory neuronal activity can trigger tracheal and bronchial constriction, bronchospasm, mucus secretion and neurogenic inflammation.
In animal models, respiratory depression, the acute drop in respiratory rates following chemical exposure, is considered to be a sign of chemical sensory irritation in the airways (2). The RD50, the airborne irritant concentration inducing a 50% decrease in respiratory rate in mice, is an important toxicological parameter for categorization of irritant potencies, used as a guideline for the establishment of human exposure limits (68). RD50 parameters have been established for a large number of environmental and industrial irritants, including acids (HCl, sulfur dioxide), bases (ammonia), oxidants (ozone, chlorine, nitrogen dioxide), electrophilic aldehydes (acrolein, formaldehyde, acetaldehyde), alcohols (methanol, ethanol), solvent fumes (acetone, toluene, xylene) and basic industrial chemicals (isocyanates, styrene) (68). Many of these agents are combined with other irritants in complex environmental exposures. For example, photochemical smog contains ozone, nitrogen oxides and volatile organic compounds (VOCs) such as acrolein, generated through oxidation of VOCs released from vegetation, or from human sources such as automobile exhaust. Tobacco smoke, smoke from structural fires or from burning vegetation contains mixtures of reactive carbonyl compounds (acrolein, methacrolein, croton aldehyde), oxidants, other reactive chemicals and particulates.
Chemical exposure studies in animals showed that reactive irritants can cross-desensitize each other's ability to initiate respiratory defensive responses. For example, airway challenges by formaldehyde vapors in rats reduced respiratory irritant responses to subsequent exposures to chlorine and acetaldehyde, and vice versa (6, 30). These observations suggest that sensory neurons contain a single reactive irritant receptor site. Once this site is saturated through exposure to a reactive irritant, it is rendered unresponsive to subsequent challenges by the same, or other, irritants. Alternatively, several different receptors may exist, cross-desensitizing each other through cellular signaling pathways.
In contrast to other sensory systems, little is known about the molecular nature of chemical receptor sites in airway sensory neurons. In the human olfactory system, hundreds of different G-protein coupled receptors, each restricted in expression to individual neurons, detect components of airborne chemical mixtures and represent their compositions as a particular smell (24). In contrast, a relatively small number of taste receptors expressed in the taste buds of the tongue discriminate between ingested chemicals and induce sweet, bitter and savory taste sensations (29). Whether airway chemosensory neurons use similar, or other, receptor systems for chemical detection and signal integration is a central question in airway physiology and chemosensory research.
Capsaicin, TRPV1 and Cough
Peripheral sensory neurons give rise to different neuronal fiber types categorized by size, degree of myelination and sensitivity to physical and chemical stimuli. Chemicals encountered in the airways are detected by C-fibers. C-fibers lack myelination, allowing rapid access for chemical stimuli along the fiber path through the tissue. The majority of C-fibers are sensitive to capsaicin, the pungent ingredient in chili peppers. Capsaicin is a potent respiratory irritant, inducing sneezing, cough, profuse mucus secretion and pain. Respiratory capsaicin exposures can lead to life-threatening complications, potentially leading to respiratory arrest and death (117). Inhalation challenge experiments in humans identified resiniferatoxin, an ultra-potent capsaicin analog, as one of the most potent tussive agents ever described (72). The capsaicin cough challenge test is widely used in clinical research to examine sensory hyperexcitability in the background of respiratory disease (37). Patients affected by cough variant asthma, a form of asthma that manifests itself through chronic cough, often show increased sensitivity to capsaicin challenges (96). Capsaicin cough hypersensitivity has also been observed in patients affected by chronic obstructive pulmonary disease (COPD), interstitial lung disease (ILD), gastroesophegal reflux disease (GARD), upper airway cough syndrome and bronchiectasis (49, 71, 99). Airway infections are also known to heighten capsaicin cough sensitivity (100, 104: Braman, 2006 #403).
Studies in animal models found that capsaicin effectively desensitizes acute and inflammatory airway responses to many different noxious chemical stimuli. For example, intraperitoneal injection of capsaicin prevented chlorine-induced respiratory depression in mice and reduced ozone-induced airway inflammation and hyperreactivity in guinea pigs and rats (60, 67, 93). Capsaicin injections also diminished airway responses to acrolein in guinea pigs and mice, and to formaldehyde and cigarette smoke in rats (73, 78, 79, 92). In guinea pigs, capsaicin abated sulfur dioxide-provoked bronchoconstriction (9). These observations suggested that capsaicin receptors are either directly involved in irritant sensing, or that capsaicin desensitizes chemical detection mechanisms. This hypothesis was further supported by the observation that capsazepine, a specific antagonist of capsaicin-induced C-fiber activation, effectively blocked acid-induced bronchoconstriction and cough in guinea pigs (14, 70).
The cloning of the capsaicin receptor, reported in 1997, launched a new era in chemosensory research (27). The capsaicin (or vanilloid) receptor, TRPV1, is a member of the Transient Receptor Potential (TRP) gene family of ion channel subunits (27). Immunohistochemical studies confirmed the presence of TRPV1 in airway sensory fibers lining the trachea, bronchi and alveoli, as well as the nasal mucosa (46, 114, 133). In recent years TRPV1 has been the focus of hundreds of studies investigating its pharmacology, regulation, structure-function relationships and physiological role. In addition to being activated by capsaicin, TRPV1 is activated and potentiated by extracellular protons generated during tissue acidosis and ischemia (59, 126). Moreover, TRPV1 was found to be thermosensitive, responding to painfully hot thermal stimuli exceeding 42°C (27). Genetic studies in mice revealed that TRPV1 is a major mediator of inflammatory thermal hyperalgesia, the inflammatory painful hypersensitivity to hot stimuli (26). TRPV1 is a tetrameric non-selective cation channel. Structure-function studies localized the vanilloid binding domain to a protein segment encompassing predicted transmembrane domains 3-4 (FIGURE 1) (56, 58, 77). The vanilloid binding domain is also the interaction site for endogenous agonists of TRPV1, including anandamide, a cannabinoid product (58). Potential proton interaction sites were identified in the vicinity of the channel pore domain (FIGURE 1) (59).

Structures of TRPA1 (left) and TRPV1 (right) monomers, modeled after the X-ray crystal structure of the potassium channel, Kv1.2, a channel of the same superfamily of P-loop cation channels (77). The intracellular N-terminal segment of TRPA1 has ~ 15 ankyrin repeats, containing several cysteine and lysine residues (grey) crucial for activation by reactive agonists, as well as a partial EF-hand domain implicated in calcium-dependent gating (39, 50, 80, 138). Sites in transmembrane domain S6 are essential for gating by antagonists and agonists (31). TRPV1 is gated by vanilloids and endogenous inflammatory fatty acid metabolites binding to sites close to the intracellular face of the membrane (58). Protons interact with sites at the extracellular end of S5 and within the channel pore loop (59). N- and C-termini have interaction sites for calmodulin (CaM) and ATP (127). Positively charge domains within the C-termini of TRPA1 and TRPV1 may serve as interaction sites for phosphatidylinositol-4,5-bisphosphate (PIP2), the substrate of phospholipase C, exerting an inhibitory effect on channel function (36, 62, 105, 127).
The cloning of TRPV1 sparked intensive drug discovery efforts, resulting in the development of novel selective TRPV1 antagonists with analgesic and anti-inflammatory properties in animal models of pain (121). A small number of studies examined the effects of these antagonists in the respiratory system. Iodo-resiniferatoxin, an iodinated derivative of resiniferatoxin with TRPV1 antagonist activity, was shown to block anandamide- and acid-induced cough in guinea pigs (55, 128). Two other newly designed TRPV1 antagonist inhibited acid-induced cough in guinea pigs with efficacies similar to that of codeine (17, 75). Taken together, these studies support an essential role of TRPV1 in acid-induced cough. Tissue acidification has been observed in a variety of conditions associated with airway hyperresponsiveness and cough, including acid reflux disease, asthma, interstitial lung disease and COPD (64). Electrophysiological studies on vagal sensory nerves detected acid-induced sustained inward currents with TRPV1-like characteristics, as well as transient acid-sensitive currents, probably mediated by sodium-selective acid sensitive ion channels (ASICs) (47, 66). The contribution of these ion channel types to acid sensing in the airways differs across species, and may differentially affect acute and inflammatory airway responses. Whereas the role of TRPV1 in airway acid sensing is firmly established in the guinea pig model, TRPV1 may only play a minor role in acute acid sensing in rodent airways (65, 120).
While capsaicin is capable of desensitizing airway irritant responses through activation of TRPV1, TRPV1 is unlikely to represent the major reactive irritant receptor. TRPV1-deficient mice showed normal respiratory sensitivity to electrophilic agents (acrolein) and solvents (styrene), responding with profound respiratory depression (120). In electrophysiological studies TRPV1 lacked any responsiveness to acrolein (38). Moreover, calcium imaging studies in cultured sensory neurons found that electrophilic irritants activated only a subset of capsaicin-sensitive neurons (52).
TRPA1 – More Than Just Spice
Following the discovery of TRPV1, additional TRP channel subunits were identified in sensory neurons. The newest addition to the family of sensory neuronal TRP channels is TRPA1 (Transient Receptor Potential Ankyrin 1) (119). TRPA1 is the only member of the TRPA sub-branch of the TRP gene family in mammals, characterized by a large number of N-terminal ankyrin repeats (~17) (FIGURE 1). TRPA1 cDNA was initially cloned from cultured human lung fibroblasts (54). In sensory neurons, TRPA1 was first identified as a cold-sensitive ion channel in a small subset (<4%) of peptidergic sensory neurons (119). However, later studies showed that TRPA1 expression is more widespread, encompassing 20-35% of sensory neurons (57, 95). Pharmacological experiments revealed that TRPA1 is the sensory neuronal receptor for mustard oil (allyl isothiocyanate), the pungent ingredient in mustard and wasabi (FIGURE 2)(7, 57). Similar to capsaicin, mustard oil activates peripheral C-fibers, causing acute pain, thermal and mechanical hyperalgesia and neurogenic inflammation (12, 53). Recent studies identified additional pungent natural products as TRPA1 agonists. These include cinnamic aldehyde, from cinnamon, allicin and diallyl sulfides, derived from garlic and onions, carvacrol, in oregano, as well as isovelleral, a fungal deterrent, and polygodial, the spicy ingredient in water pepper and Tasmanian pepper (FIGURE 2)(7, 13, 41, 81, 136).
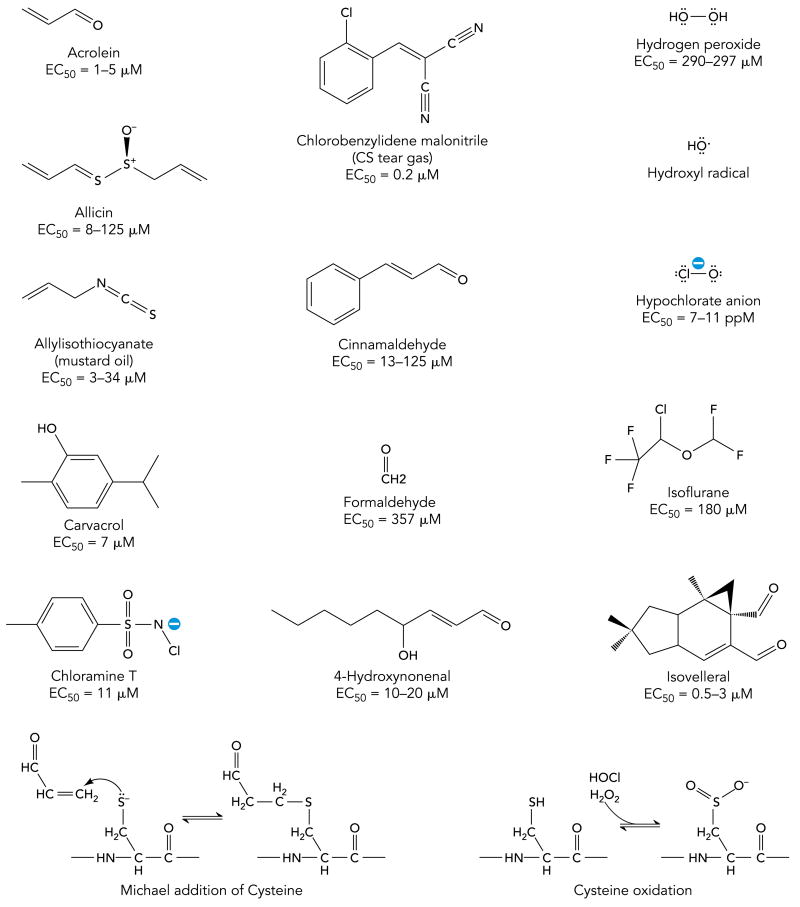
TRPA1 is activated by a large variety of reactive irritants, including acrolein, a major constituent of tobacco smoke, allicin, mustard oil and cinnamaldehyde, the pungent ingredients in garlic mustard and cinnamon, respectively, chlorobenzylidene malononitrile (CS tear gas), the reactive oxygen species (ROS) hydrogen peroxide and hydroxyl radical, and hypochlorite, the product of the potent irritant gas, chlorine. Formaldehyde is a potent industrial and environmental irritant. Isovelleral, a painful fungal dialdehyde sesquiterpene, activates TRPA1 to deter fungivores. TRPA1 is also activated by the disinfectant, chloramines-T, as well as by 4-hydroxy-nonenal (4-HNE), a potential endogenous agonist. Reactive irritants activate TRPA1 through covalent protein modification, as shown for the example of acrolein undergoing a Michael addition reaction with a cysteine residue. Oxidants such as hypochlorite or hydrogen peroxide oxidize cysteine residues to sulfinic or sulfonic acid groups.
The biophysical and pharmacological properties of TRPA1 show intriguing parallels with those of the proposed reactive airway irritant receptor. First, functional imaging and electrophysiological studies revealed that TRPA1 is expressed in a subpopulation of TRPV1-expressing C-fiber neurons and is permeable to calcium ions. This observation is in congruence with previous studies showing that reactive airway irritants activate influx of calcium ions in a subset of capsaicin-sensitive neurons (52). Second, TRPA1 is blocked by ruthenium red, known to antagonize irritant-induced bronchoconstriction (5, 18, 84). Third, capsaicin pre-treatment renders C-fibers insensitive to both mustard oil and chemical airway irritants (20, 53). Fourth, mustard oil itself is a potent upper airway irritant, as sushi connoisseurs can attest who take delight in the nose-clearing effects of wasabi (20). Taken together, these facts encouraged closer examination of the role of TRPA1 in airway chemical sensing.
An initial breakthrough was made when pharmacological experiments revealed that acrolein, the noxious α, β-unsaturated aldehyde enriched in photochemical smog and smoke, is a potent agonist of human and murine TRPA1 channels (FIGURE 2)(12). Acrolein robustly activated TRPA1-mediated influx of calcium ions into cultured sensory neurons and heterologous cells (12). Cultured sensory neurons from TRPA1-deficient mice lacked any responsiveness to this irritant, suggesting that TRPA1 is the sole chemosensory receptor for acrolein (12). Recent studies showed that TRPA1 is activated by additional noxious α, β-unsaturated aldehydes, including smoke constituents such as methacrolein, methyl vinyl ketone and croton aldehyde (4, 41).
TRPA1 is not only sensitive to electrophiles, but is also activated by oxidizing agents entering the airways. A recent study identified hypochlorite (OCl-), the oxidative mediator of the potent airway irritant, chlorine gas, as a potent agonist of TRPA1 (16). This study was the first to show that TRPA1 is essential for irritant-induced airway responses in conscious animals in vivo (16). Chlorine is a ubiquitous chemical, used in industrial processes and for disinfection. Airway exposures to chlorine frequently occur in industrial and transportation accidents, or as a consequence of mishandling of chlorine-based disinfectants in commercial, recreational or domestic settings (42). Chlorine was also used as warfare agent, specifically targeting the airways (111). Although highly reactive and cytotoxic, hypochlorite was found to activate influx of calcium ions into a specific subset of cultured murine sensory neurons, including neurons derived from trigeminal and nodose ganglia innervating the upper and lower airways, respectively (16). Pharmacological characterization of the hypochlorite-sensitive neuronal population revealed that it was identical to the mustard oil-sensitive population. Superfusion with hypochlorite-containing solution led to robust activation of cloned human and murine TRPA1 channels expressed in HEK293 cells. Strikingly, responses to hypochlorite were absent in neurons dissociated from TRPA1-deficient mice (16). Whereas wild-type mice responded to hypochlorite exposures with a typical decline in respiratory rates, TRPA1-deficient mice seemed unmoved by the noxious stimulus, displaying normal respiratory rates throughout extended exposures at concentrations near the RD50 for chlorine (16). Similar differences were observed following exposures to hydrogen peroxide, another oxidizing agent known to excite airway nerve fibers and to induce respiratory depression (16, 109, 110).
A recent study by Andre et al. provided additional support for the essential role of TRPA1 in airway chemosensation of noxious tobacco smoke constituents (4). The authors found that a TRPA1-specific antagonist, HC-030031, abolished neuropeptide-mediated constriction of isolated guinea pig bronchial segments following superfusion with α, β-unsaturated aldehydes or aqueous cigarette smoke extract. In TRPA1-deficient mice, instillation of cigarette smoke extract into the trachea failed to initiate neurogenic plasma extravasation. Chemical depletion of electrophilic compounds from cigarette smoke extract abolished neurogenic inflammation, suggesting that the interaction of electrophilic constituents of cigarette smoke with TRPA1 is the major mechanism by which cigarette smoke induces acute neurogenic inflammation in the airways (4).
Studies using specific antibodies to detect TRPA1 immunoreactivity in airway nerve endings have not been published, probably due to the lack of high quality antibodies. TRPA1 mRNA was detected together with TRPV1 mRNA in a significant percentage of airway-innervating neurons identified through retrograde fluorescent labeling (97). Similar to other electrophilic irritants, the TRPA1 agonist cinnamic aldehyde excited these neurons and induced respiratory depression upon airway exposure (97).
In summary, these recent studies have shown that TRPA1 is essential for chemical sensing of oxidants and electrophilic agents in the airways, initiating physiological responses such as respiratory depression and neurogenic inflammation. This important role of TRPA1 may not be restricted to chemical sensing of oxidants and unsaturated aldehydes. Recent pharmacological studies examining cellular responses and chemically induced pain behavior found that TRPA1 is mediating sensory neuronal responses to formaldehyde, acetaldehyde, tear gas agents and industrial isocyanates, all potent airway irritants (FIGURE 2)(8, 15, 21, 89). TRPA1 activation may also underlie the irritant effects of common inhalation anesthetics. Anesthetics such as isoflurane or desflurane are known to reduce respiratory function and can cause severe, sometimes lethal, respiratory complications in pediatric and adult patients with unexpected bronchial hyperreactivity (FIGURE 2)(83, 86). Both anesthetics activate TRPA1 in native neurons and in heterologous cells, and promote TRPA1-dependent neurogenic edema (86). Whether TRPA1 is the major specific target for these agents in airway sensory nerve endings remains to be established in tests of airway function.
TRPA1 Activation – A Mechanism that Sticks
Reactivity recognition by TRPA1
Since TRPA1 was identified as the sensory neuronal receptor for mustard oil, a plethora of additional TRPA1 agonists has been discovered. These include structurally diverse pungent natural products, environmental toxic irritants, industrial chemicals and pharmaceuticals, as well as endogenous reactive mediators (FIGURE 2). How can a single receptor cover such broad chemical space ? Recent structure-function studies provided a potential explanation for the diversity of TRPA1 agonists (50, 80). These studies suggest that TRPA1 is activated through covalent modification of the channel protein. Most TRPA1 agonists are chemically unstable in biological environments and can undergo chemical reactions with proteins, lipids, nucleic acids and metabolic products. Isothiocyanates such as mustard oil (allyl isothiocyanate) form thiourea adducts with cysteine residues (50). Unsaturated aldehydes such as acrolein are strong electrophiles and react with cysteine residues via the Michael Addition mechanism, and with lysine and histidine residues through Schiff base formation (FIGURE 2). Hypochlorite, hydrogen peroxide and other reactive oxygen species directly oxidize cysteine thiols to sulfinic or sulfonic acid groups (FIGURE 2) (101). Hypochlorite also chlorinates lysine residues, leading to formation of chloramines that undergo further chemical reactions (101). By probing TRPA1 responsiveness with the irreversible cysteine-reactive agent, N-methyl maleimide, Hinman et al. found that TRPA1 can be locked into a constitutively active state, indicating saturation of a reactive site (50). Through systematic mutation of candidate acceptor sites these authors identified an essential cluster of cysteine and lysine residues in the cytosolic N-terminus of TRPA1 (FIGURE 1). When non-reactive residues were introduced at these sites, TRPA1 became unresponsive to mustard oil (50). The same sites were also found to be essential for activation of TRPA1 by chlorine, hydrogen peroxide and unsaturated aldehydes (16, 41, 129). A separate study discovered that, while almost all cytosolic cysteine residues in TRPA1 were modified following chemical treatment with cysteine reactive agents, three cysteine residues were crucial for channel activation (80).
The studies summarized above provide strong support for a mechanism of activation of TRPA1 by covalent modification through reactive irritants. This mechanism implies that dose-response relationships and activation kinetics of TRPA1 do not conform to standard pharmacological paradigms and are highly dependent on the chemical status of the cellular and tissue environment. Indeed, EC50 parameters for mustard oil and other TRPA1 agonists show wide divergence, sometimes 1-2 orders of magnitude, when compared between heterologous expression systems and native sensory neurons, and in physiological and behavioral experiments in intact animals. For any given chemical, TRPA1 agonist activity will depend on the reversible or irreversible nature of the chemical bonds formed and on agonist membrane permeability. Since most TRPA1 agonist can react with thiols, cellular and extracellular reduced glutathione levels will affect the reach and potency of inhaled airway irritants. Once glutathione is depleted, either as a consequence of disease or during extended exposures, TRPA1 may respond much more strongly. With each breath more reactive agonist is delivered, leading to an increase in covalent modifications and heightened TRPA1 activity. This cumulative effect may result in robust TRPA1-induced irritation even at low sub-acute exposure levels, for example during periods of increased photochemical smog exposures, or low level indoor air pollution. Once irreversibly modified, channels may remain active for extended periods of time even when the irritant stimulus is removed. Continued, stimulus-independent, TRPA1 activity may explain the extended respiratory depression observed in mice following removal of chlorine exposures, and the continued sense of irritation in individuals exposed to smoke or tear gases. (16).
Activation by endogenous mediators of oxidative stress
New reactive TRPA1 agonists have been discovered at a breathtaking pace suggesting that, given appropriate conditions, almost all oxidizing or electrophilic chemicals will affect TRPA1 function. In addition to exogenous chemicals, several groups of reactive biological products were identified that, when applied exogenously, activated TRPA1 in vitro and in vivo. These potential endogenous agonists include reactive oxygen species (ROS), hypochlorite, lipid peroxidation products, cyclopentenone prostaglandins and isoprostanes.
Oxidative stress is a hallmark of most acute and chronic inflammatory airway conditions, including viral infections, asthma, rhinitis and COPD (19, 51, 107). ROS are also produced during oxidant exposures and through catalysis by inhaled toxic particulates (94). During inflammation, ROS are generated by infiltrating macrophages and neutrophils (108, 135). Similar to the oxidant gas chlorine, ROS such as hydrogen peroxide excite airway sensory nerve fibers, resulting in respiratory depression (109, 110). TRPA1-deficient mice showed largely reduced airway responsiveness to hydrogen peroxide given as an aerosol (16). TRPA1 is activated by hydrogen peroxide in heterologous systems and cultured sensory neurons, and is essential for pain behavior triggered by injections of hydrogen peroxide into the mouse paw (3, 16, 112). Andersson et al. provide evidence that the effects of hydrogen peroxide are partially mediated through hydroxyl radicals, suggesting that TRPA1 is activated by multiple ROS (3).
Hypochlorite, shown to cause respiratory depression through activation of TRPA1 in mice, is also generated endogenously, through myeloperoxidase-mediated catalysis in inflammatory neutrophils (16). Hypochlorite is a highly reactive oxidant and contributes to chemical tissue damage in chronic inflammatory conditions (43). During interstitial airway inflammation neutrophil-generated hypochlorite concentrations can rise to millimolar levels, by far exceeding the measured EC50 for TRPA1 activation (16, 134).
Exposure of cellular membranes to inflammatory ROS, hypochlorite or exogenous oxidants causes membrane lipid peroxidation, producing electrophilic reactive mediators such as 4-hydroxy-2-nonenal (HNE), 4-oxo-2-nonenal (ONE), as well as cyclopentenone prostaglandins and isoprostanes (FIGURE 2) (76, 113). These compounds are α, β-unsaturated carbonyls, with their reactive moieties resembling the previously identified TRPA1 agonist, acrolein (12). In fact, acrolein itself is produced endogenously by lipid peroxidation and other oxidative processes and can be considered both an exogenous and endogenous TRPA1 agonist (12, 113, 118, 130). Similar to acrolein, exogenously applied HNE, ONE and cyclopentenone prostaglandins activated TRPA1 in heterologous cells, neurons and nerve fibers, and induced TRPA1-dependent nocifensive behavior in mice upon hindpaw injections of synthetic compounds (3, 34, 82, 85, 122, 124, 125, 129). The potencies of these compounds correlated with their reactivity towards thiols. ONE, carrying two reactive carbonyl moieties, was found to be the most potent TRPA1 agonist among the lipid peroxidation products tested (3, 124). Difficult technical challenges remain to prove whether any of the above compounds is among the predominant endogenous agonists of TRPA1 in vivo. Information about tissue concentrations, stability and reach of lipid peroxidation products is scarce, estimated mostly through measurements of their chemical conjugates in tissue extracts (113). TRPA1 agonist activity of a given endogenous compound depends on its site of origin, its reactivity, membrane permeability and reach, on tissue antioxidant levels and many other factors. TRPA1 is likely to be activated or sensitized by a diverse cocktail of simultaneously present oxidants and electrophiles than by a single predominant agonist. This idea relates to the concept of the “inflammatory soup”, describing the diverse mix of chemical and biological mediators promoting sensory neuronal sensitization and activation during tissue injury and inflammation (90). It appears that endogenous oxidants and electrophiles need to be added to this particular mix.
Calcium, adding fuel to the fire
Calcium ions are essential co-activators of TRPA1 (28, 39, 57, 95, 132, 138). Removal of extracellular and intracellular Ca2+ dramatically reduced the potency of mustard oil to activate TRPA1 and slowed channel activation kinetics (57, 95). Patch-clamp studies showed that Ca2+, when applied to the intracellular face of the membrane, induced TRPA1 channel activity (39, 138). Structural prediction algorithms identified a partial EF-hand loop as a potential Ca2+-binding domain, localized within the cytosolic N-terminus of TRPA1, overlapping with one of the multiple N-terminal ankyrin repeats (FIGURE 1). Mutations at putative Ca2+-coordination sites within this domain diminished or abolished activation of TRPA1 by Ca2+ (39, 138). However, the essential role of these residues in Ca2+-dependent gating has recently been disputed (132).
The dependence of TRPA1 on Ca2+ is likely to contribute to the variability of channel responses to reactive agonists. In the absence of Ca2+ reactive irritants such as mustard oil are in fact rather weak TRPA1 agonists (57). However, in the presence of Ca2+, even weak channel activation may allow sufficient influx of Ca2+ through the TRPA1 channel pore, increasing intracellular Ca2+ levels to a point where Ca2+ becomes the major activating stimulus. This positive feedback mechanism may result in uncoupling of TRPA1 activity from the initial irritant stimulus. TRPA1 channels in the vicinity of activated channels may be activated through spillover of Ca2+, without ever being modified by a reactive ligand. TRPA1 may also act as an amplifier of other Ca2+ mobilizing pathways including activation of TRPV1, or Ca2+ release from intracellular stores, leading to neuronal excitation and local inflammatory release of neuropeptides. TRPA1 activity may be highly dependent on local cellular Ca2+ buffering and extrusion mechanisms, as well as on the number of TRPA1 channels expressed at the site of exposure. Besides Ca2+, other factors add to the complexity of TRPA1 activation and modulation. For example, intracellular polyphosphate (PPPi) ions were recently identified as essential co-factors for irritant- and Ca2+-induced TRPA1 activation (28, 63).
TRPV1 and TRPA1 in Airway Disease - Too Much Protection ?
The same signaling pathways responsible for inflammatory sensitization in cutaneous and visceral sensory neurons are also present in trigeminal and vagal fibers innervating the upper and lower airways (74, 123). While inflammatory sensitization of somatosensory neurons causes hyperalgesia and allodynia, sensitization of airway neurons increases nasal and cough sensitivity, heightens the sense of irritation, and promotes fluid secretion, airway narrowing and bronchoconstriction. Transient sensitization of airway neurons is thought to contribute to airway protection, eliminating chemical irritants and infectious agents and promoting tissue healing and recovery. In contrast, persistent inflammatory neuronal sensitization adds to the complications experienced by patients suffering from chronic airway conditions. Inflammatory airway diseases such as asthma, rhinitis or COPD are accompanied by the production of a wide range of pro-inflammatory mediators known to sensitize airway sensory fibers. These include histamine, prostaglandins, cysteinyl leukotrienes, proteases and peptides such as nerve growth factor and bradykinin. Bradykinin is a potent tussive agent and bronchoconstrictor in asthmatics and is elevated in the serum and airway fluid of asthma and rhinitis patients (1, 40). Block of angiotensin-converting enzyme (ACE), an enzyme involved in degradation of bradykinin, results in heightened bradykinin levels, causing chronic cough as a side effect in patients treated with ACE inhibitors for hypertension (44). Nerve growth factor is produced during allergic inflammation in salivary glands, airway epithelium, smooth muscle other airway tissue types (98).
Activation and sensitization by inflammatory receptors pathways
TRPV1 is sensitized following activation of neuronal receptors for bradykinin, prostaglandins, histamine, purines, proteases, NGF, chemokines and many other pro-inflammatory mediators (FIGURE 3)(32, 45, 48, 116). TRPV1 is crucial for bradykinin-induced excitation of vagal airway fibers. In TRPV1-deficient mice, bradykinin-induced fiber excitation is diminished, but not completely abrogated (25, 65). Similar to TRPV1, TRPA1 is both activated and sensitized through inflammatory receptor pathways (7, 12, 36, 57, 131). Activation of bradykinin or histamine receptors induces TRPA1 activity, both in heterologous cells and native sensory neurons cultured from trigeminal ganglia (7, 12, 131). TRPA1-deficient mice failed to develop thermal and mechanical hyperalgesia following injections of bradykinin into the hindpaw, indicating that TRPA1 is an important target of inflammatory signaling pathways in vivo (12, 69). A newly developed TRPA1-antagonist attenuated bradykinin-induced mechanical hyperalgesia (103). Activation of neuronal bradykinin receptors or protease-activated receptors (PAR-2) in dorsal root ganglia heightened the sensitivity of TRPA1 to mustard oil and cinnamaldehyde (36, 131). Pharmacological studies revealed that both phospholipase C (PLC) - and protein kinase A (PKA) -dependent mechanisms contribute to activation and sensitization of TRPA1 downstream of bradykinin receptor activation (7, 12, 131). Neuronal B2 bradykinin and PAR-2 receptors predominantly signal through phospholipase C. PLC catalyzes the hydrolysis of phosphatidylinositol 4,5-biphosphate (PIP2), stimulating intracellular Ca2+-release. PIP2 was shown to inhibit TRPA1, whereas sequestration of PIP2 was found to increase TRPA1 activity (36, 61, 62). These data suggest that two PLC-dependent mechanisms, PIP2 hydrolysis and intracellular release of Ca2+, promote TRPA1 activity downstream of inflammatory receptors.
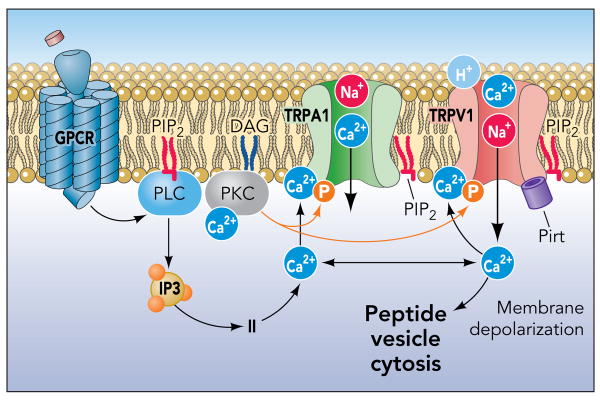
TRPA1 and TRPV1 are sensitized or activated through multiple phospholipase C-coupled receptor pathways active during inflammation, including receptors for histamine, bradykinin, prostaglandins, proteases, nerve growth factor. Intracellular Ca2+ is crucial for activation of TRPA1, mobilized either through release from intracellular stores, permeation through TRPA1, or, possibly, TRPV1. Phosphorylation through PKC and other kinases also affect TRP channel activity during inflammation. Heightened Ca2+ levels trigger release of pro-inflammatory neuropeptides such as substance p or CGRP.
Asthma, COPD, RADS, MCS & Co. – Diseases of Chemical Sensing ?
The recent experimental work summarized above shows that almost all major neuronal inflammatory signaling pathways converge on TRPV1 and TRPA1 to increase C-fiber excitability during airway inflammation. Inflammatory oxidants, lipid products and protons further promote activity of these ion channels through direct interactions or covalent modification. Due to their central role TRPV1 and TRPA1 are considered as promising targets for the development of novel anti-inflammatory and anti-tussive drugs (22, 87). This idea is supported by the recent proof of efficacy of a TRPV1 antagonist in an animal model of allergen-induced chronic cough (88).
Modulation of TRP channel activity is not restricted to acute regulatory mechanisms. Chronic inflammation in a mouse asthma model was shown to lead to changes in transcriptional patterns, inducing expression of TRPV1 in myelinated airway fibers (137). Recruitment of additional fibers may lead to long term changes in neuronal excitability reminiscent of chronic inflammatory or neuropathic pain states, causing chronic increased chemical and mechanical sensitivity and neuronal excitation even in the absence of any chemical stimuli. Neuronal remodeling should be considered as a potential additional disease mechanism, occurring in parallel with inflammatory airway tissue remodeling in airway conditions such as asthma and COPD.
Exposures to high levels of TRPA1 agonists, including chlorine and aldehydes, often induce reactive airways dysfunction syndrome (RADS) (23, 106, 115). RADS is characterized by by asthma-like symptoms such as cough, wheezing, chest tightness and dyspnoea and heightened sensitivity to chemical and physical stimuli, including the initial sensitizing stimulus (23). The multiple chemical sensitivity of TRPA1 can readily explain the broad chemical sensitivity observed in RADS patients. An initial chemical sensory challenge and tissue injury may sensitize TRPA1 channels through inflammatory signaling pathways, thereby establishing prolonged hypersensitivity to multiple reactive chemicals (7, 12, 36, 57). The role of TRPA1 in chemical hypersensitivity may extend to other, less clearly defined, conditions, including sensory hyperreactivity (SHR) and multiple chemical sensitivity (MCS) (35, 91). RADS and related conditions are only partially responsive to the therapeutic interventions developed for the treatment of asthma. TRPA1 antagonists may be useful for blocking the exaggerated chemosensory responses accompanying these conditions. Post-exposure treatment with TRP channel antagonists may reduce sensory irritation and, potentially, prevent adverse long-term health effects elicited by neurogenic inflammatory mechanisms.
Acknowledgments
This work was funded by the National Institute of Environmental Health Sciences grants ES015056 and ES015674, and by funds from the Connecticut Department of Public Health (2007-0161 BIOMED).
References
Full text links
Read article at publisher's site: https://doi.org/10.1152/physiol.00026.2008
Read article for free, from open access legal sources, via Unpaywall:
https://europepmc.org/articles/pmc2735846?pdf=render
Free after 12 months at intl-nips.physiology.org
http://intl-nips.physiology.org/cgi/pmidlookup?view=long&pmid=19074743
Citations & impact
Impact metrics
Citations of article over time
Alternative metrics
Smart citations by scite.ai
Explore citation contexts and check if this article has been
supported or disputed.
https://scite.ai/reports/10.1152/physiol.00026.2008
Article citations
Functions of TRPs in retinal tissue in physiological and pathological conditions.
Front Mol Neurosci, 17:1459083, 25 Sep 2024
Cited by: 0 articles | PMID: 39386050 | PMCID: PMC11461470
Review Free full text in Europe PMC
Formaldehyde and the transient receptor potential ankyrin-1 contribute to electronic cigarette aerosol-induced endothelial dysfunction in mice.
Toxicol Sci, 201(2):331-347, 01 Oct 2024
Cited by: 0 articles | PMID: 39067042
The Flavoring Agent Ethyl Vanillin Induces Cellular Stress Responses in HK-2 Cells.
Toxics, 12(7):472, 29 Jun 2024
Cited by: 0 articles | PMID: 39058124 | PMCID: PMC11280803
TRPV1: The key bridge in neuroimmune interactions.
J Intensive Med, 4(4):442-452, 01 Apr 2024
Cited by: 1 article | PMID: 39310069 | PMCID: PMC11411435
Review Free full text in Europe PMC
Pharmacologic Inhibition of Transient Receptor Potential Ion Channel Ankyrin 1 Counteracts 2-Chlorobenzalmalononitrile Tear Gas Agent-Induced Cutaneous Injuries.
J Pharmacol Exp Ther, 388(2):613-623, 17 Jan 2024
Cited by: 1 article | PMID: 38050077 | PMCID: PMC10801748
Go to all (239) article citations
Other citations
Wikipedia
Data
Similar Articles
To arrive at the top five similar articles we use a word-weighted algorithm to compare words from the Title and Abstract of each citation.
Effect of cigarette smoking on cough reflex induced by TRPV1 and TRPA1 stimulations.
Respir Med, 106(3):406-412, 29 Dec 2011
Cited by: 31 articles | PMID: 22209625
Cough: The Emerging Role of the TRPA1 Channel.
Lung, 188 Suppl 1:S63-8, 30 Nov 2009
Cited by: 41 articles | PMID: 20091046
Review
Interaction between TRPA1 and TRPV1: Synergy on pulmonary sensory nerves.
Pulm Pharmacol Ther, 35:87-93, 14 Aug 2015
Cited by: 22 articles | PMID: 26283426 | PMCID: PMC4690745
Review Free full text in Europe PMC
Funding
Funders who supported this work.
NIEHS NIH HHS (7)
Grant ID: R01 ES015056
Grant ID: R01 ES015056-03
Grant ID: U01 ES015674
Grant ID: ES-015674
Grant ID: U01 ES015674-03
Grant ID: ES-015056
Grant ID: U54 ES017218