Abstract
Free full text

CXCR3 directs antigen-specific effector CD4+ T cell migration to the lung during parainfluenza virus infection1
Abstract
Effector T cells are a crucial component of the adaptive immune response to respiratory virus infections. Although it was previously reported that the chemokine receptors CCR5 and CXCR3 are expressed on respiratory virus-specific effector CD8+ T cells, it is unclear whether these receptors govern effector CD4+ T cell migration to the lungs. To assess the role of CCR5 and CXCR3 in vivo, we directly compared the migration of antigen-specific wild-type and chemokine receptor-deficient effector T cells in mixed bone marrow chimeric mice during a parainfluenza virus infection. CXCR3-deficient effector CD4+ T cells were 5-10 fold less efficient at migrating to the lung compared with wild-type cells, whereas CCR5-deficient effector T cells were not impaired in their migration to the lung. In contrast to its role in trafficking, CXCR3 had no impact on effector CD4+ T cell proliferation, phenotype, or function in any of the tissues examined. These findings demonstrate that CXCR3 controls virus-specific effector CD4+ T cell migration in vivo, and suggest that blocking CXCR3-mediated recruitment may limit T cell-induced immunopathology during respiratory virus infections.
Introduction
The adaptive immune response to respiratory pathogens is characterized by the proliferation of antigen-specific T lymphocytes in the draining lymphoid tissues, their entry into the circulation via the thoracic duct, and their subsequent migration to various compartments of the lung (1). The arrival of effector CD4+ and CD8+ T cells in the lung has a dramatic impact on the viral load that is mediated by the expression of cytokines and the direct lysis of infected cells. Virus-specific effector CD4+ T cells in the lung produce the pro-inflammatory cytokines IFN-γ and TNF-α, and to a lesser extent also produce IL-2 and IL-10 (2-4). In addition, effector CD4+ T cells can directly lyse target cells via a perforin-mediated mechanism and contribute to antibody-independent protection (5, 6). In the case of parainfluenza virus infections, antigen-specific CD4+ T cells in the lung confer significant protection from a secondary virus infection (7), and effector CD4+ T cells in the lung play a substantial role in mediating virus clearance (8). Together, these studies demonstrate that in addition to providing help for CD8+ T cell and B cell responses, the antiviral effector functions of CD4+ T cells in the lungs play an important part in the control of virus replication.
Analysis of the inflammatory response following influenza and parainfluenza virus infection revealed that both CCR5- and CXCR3-binding chemokines are highly expressed in the lung, suggesting an important role for these molecules in directing the recruitment of virus-specific effector T cells to the site of infection (9, 10). In addition, it has been shown that CXCR3, and to a lesser extent CCR5, are expressed on effector or memory T cells specific for respiratory viruses (11-14), and that CXCR3 is important for the migration of effector CD8+ T cells to the lung during respiratory virus infections (15, 16). In contrast to CD8+ T cells, however, relatively little is known about chemokine receptor expression on virus-specific effector CD4+ T cells (17). Although both CXCR3 and CCR5, or their ligands, have been implicated in CD4+ T cell-mediated pulmonary disease models (18-21), there is no direct evidence that these chemokine receptors control the migration of antigen-specific effector CD4+ T cells in vivo during a respiratory virus infection.
One difficulty in studying CD4+ T cell responses to respiratory viruses is the lack of reagents capable of identifying polyclonal virus-specific cells that are generated from endogenous naïve precursors. In the present study, we have investigated the role of CCR5 and CXCR3 in effector CD4+ T cell recruitment to the lungs during a parainfluenza virus infection by employing mixed bone marrow (BM) chimeras and virus-specific multimers to directly compare the migration of polyclonal antigen-specific wild-type and chemokine receptor-deficient T cells under identical conditions in vivo.
Materials and Methods
Mice, viruses, and infection
C57BL/6, B6.SJL-Ptprca Pep3/BoyJ (CD45.1), and B6.Ccr5-/- (B6.129P2-Ccr5tm1Kuz /J) mice were purchased from The Jackson Laboratory. B6.Cxcr3-/- and B6.Ccr5-/- Cxcr3-/- double-deficient mice were previously described (22, 23). Mixed BM chimeras were generated as previously described (24). Mice (8-12 weeks) were anesthetized by intraperitoneal injection with 2,2,2-tribromoethanol (200 mg/kg) and infected intranasally with 250 50% egg infectious doses (EID50) of Sendai virus (Enders strain). All animal studies have been reviewed and approved by the Trudeau Institute Animal Care and Usage Committee.
Tissue harvest and flow cytometry
Mice were sacrificed on day 10 post-infection and single-cell suspensions were obtained from the lung airways (by bronchoalveolar lavage, BAL), lung parenchyma, mediastinal lymph node (MLN), and spleen as previously described (25). Cells were stained with SenHN419-433Ab-APC multimer and SenNP324-332Kb-PE tetramer and antibodies to CD4, CD8, CD27, CD43, CD44, CD45.2, CD49b, CD69, CCR5, PSGL1, NK1.1, TCRβ (BD Biosciences and eBbiosciences), KLRG1 (Southern Biotech), PD1 (BioLegend) or CXCR3 (R&D Systems). Samples were acquired on a FACSCanto II flow cytometer (BD Biosciences) and data were analyzed with FlowJo software (Tree Star).
Assessment of cytokine production
Single cell suspensions were incubated with SenHN419-433 or FluHA211-225 peptides as previously described (26). Cells were stained for surface markers, fixed and permeabilized (CytoFix/CytoPerm kit, BD Biosciences), and stained for intracellular cytokines with antibodies to IFN-γ, TNF-α, and IL-2.
Measurement of proliferation by 5-bromo-2-deoxyuridine (BrdU) incorporation
Mice were administered BrdU (200μl of a 4mg/ml solution in PBS) intraperitoneally and maintained on drinking water containing BrdU (0.8 mg/ml) for 24 hours prior to harvest. Single cell suspensions were stained with tetramers and antibodies to surface proteins as described above and BrdU incorporation was detected using the BrdU Flow Kit (BD Biosciences).
Calculation of relative recruitment
The standardized number of antigen-specific T cells in the BAL, lung, or MLN compared to the spleen for each donor population was calculated as: (the number of antigen-specific T cells in each tissue / the number of antigen-specific T cells in spleen) × 100. The relative recruitment comparing wild-type and chemokine receptor-deficient antigen-specific T cells to the BAL, lung, and MLN is calculated as: (the standardized number of wild-type (CD45.1+) antigen-specific T cells in each tissue / the standardized number of chemokine receptor-deficient (CD45.2+) antigen-specific T cells in each tissue).
Statistics
Statistical analysis was performed with Prism GraphPad software using a two-tailed student's t test. Differences with a p value <0.05 were considered significant and are denoted by an asterisk.
Results
The majority of virus-specific CD4+ T cells express CXCR3
Previous studies have documented the expression of CXCR3 and CCR5 on respiratory virus-specific effector CD8+ T cells, but there is little information regarding chemokine receptor expression by polyclonal virus-specific effector CD4+ T cells. Thus, we first assessed the surface expression of CXCR3 and CCR5 on SenHN419-433/Ab-specific CD4+ T cells after Sendai virus infection. Similar to CD8+ T cells, the majority of antigen-specific CD4+ T cells in the BAL, lung, MLN, and spleen expressed CXCR3, whereas only a small frequency expressed CCR5 (Figure 1A, 1B). However, it was unclear from these data whether one or both of these chemokine receptors were important for antigen-specific CD4+ T cell migration during a respiratory virus infection.
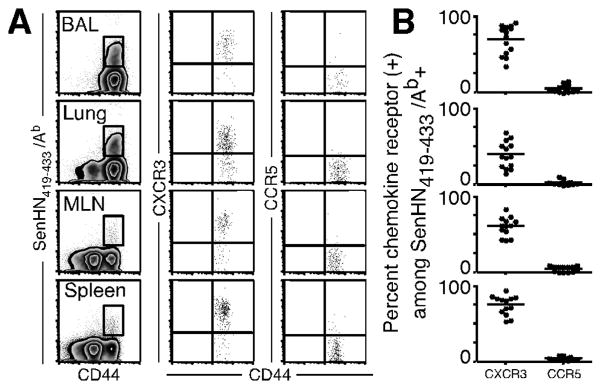
(A, B) C57BL/6 mice were infected with Sendai virus, and cells were obtained from the indicated tissues on day 10 post-infection. Antigen-specific CD4+ cells were identified by SenHN419-433Ab multimer, and representative staining for CXCR3 and CCR5 gated on antigen-specific cells is shown for each tissue. (B) The frequency of SenHN419-433Ab+ cells expressing CXCR3 and CCR5 in each tissue. Each symbol represents an individual mouse. The data are representative of 3 independent experiments.
Normal generation of polyclonal virus-specific effector CD4+ T cells in chemokine receptor-deficient mice
To begin assessing the impact of chemokine receptor deficiency on virus-specific effector CD4+ T cells, we infected wild-type and gene-deficient mice with Sendai virus and determined the number of SenHN419-433/Ab-specific CD4+ T cells in multiple tissues at the peak of the primary response. As shown in Figure 2A, antigen-specific CD4+ T cells were detected in the lungs of Ccr5-/-, Cxcr3-/-, and Ccr5-/- × Cxcr3-/- mice at day 10 post-infection. In terms of total numbers, Ccr5-/- mice had fewer SenHN419-433/Ab-specific CD4+ T cells in the BAL and lungs when compared to wild-type mice (Figure 2B). However, Ccr5-/- mice also had fewer SenHN419-433/Ab-specific CD4+ T cells in the MLN and spleen, making it difficult to determine whether the decreased number of antigen-specific cells at the site of infection was due to a defect in T cell migration, or simply because there were fewer total antigen-specific cells in the animal. Similarly, although the number of SenHN419-433Ab+ CD4+ T cells in the lungs of Ccr5-/- × Cxcr3-/- mice was similar to wild-type mice, Ccr5-/- × Cxcr3-/- mice had a larger number of SenHN419-433/Ab-specific CD4+ T cells in the spleen. An additional difficulty in studying the role of CCR5 and CXCR3 in lymphocyte migration during infection is that many different cell types involved in the response, including monocytes, dendritic cells, NK cells, and T cells, express these receptors (27-30). Thus, interpreting data regarding effector CD4+ T cell recruitment from experiments utilizing gene-deficient mice or blocking antibodies can be difficult due to indirect effects from these other cell types, and because the global inhibition of one or more chemokine receptors may alter the course of infection, even though each of these strains was able to clear Sendai virus and survive (data not shown). Therefore, although these data clearly demonstrate that chemokine receptor deficiency did not prohibit the generation of polyclonal virus-specific effector CD4+ T cells, it was not clear whether these chemokine receptors were impacting SenHN419-433/Ab-specific CD4+ T cell migration to the lung.
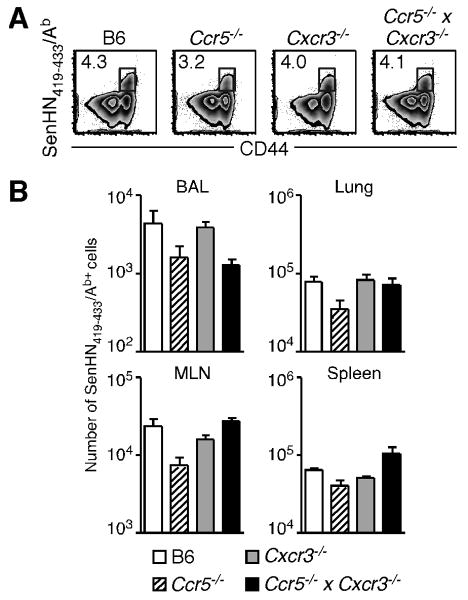
C57BL/6, Ccr5-/-, Cxcr3-/-, and Ccr5-/- × Cxcr3-/- mice were infected with 250 EID50 Sendai virus, and cells were obtained from the indicated tissues on day 10 post-infection. (A) Example staining of SenHN419-433Ab+ cells (gated on total CD4+ cells) from each mouse strain is shown. (B) The total number of SenHN419-433Ab+ cells from the BAL, lung, MLN, and spleen for each mouse strain is graphed as the mean ± s.d. The data are representative of 2 independent experiments with 5 mice per group.
The migration of virus-specific CD4+T cells to the lung is significantly impaired in the absence of CXCR3
To directly investigate the role of CCR5 and CXCR3 in T cell migration in vivo during respiratory virus infection, we generated mixed BM chimeras from wild-type congenic (CD45.1) and chemokine receptor-deficient mice (CD45.2). Chimeras were then infected with Sendai virus (Figure 3A) and antigen-specific donor CD4+ T cells identified by congenic marker and multimer staining (Figure 3B). As shown in Figure 3C, there was no significant difference in the number of wild-type and Ccr5-/- SenHN419-433/Ab-specific CD4+ T cells in the BAL, lung, MLN and spleen (left graph). Furthermore, the relative recruitment of wild-type and Ccr5-/- cells (which allows for the normalization of recruitment between donor populations in the same animal based on the number of antigen-specific cells at a non-inflamed site such as the spleen) showed that these cells migrated to the BAL and lung with equal efficiency (Figure 3D, left graph). In contrast, the number of Cxcr3-/- antigen-specific CD4+ T cells in the BAL and lung was significantly reduced compared to wild-type T cells in the same host (Figure 3C, middle graph). We confirmed that the difference in antigen-specific CD4+ T cell number was indeed due to defective migration of Cxcr3-deficient cells, as wild-type cells were approximately 5-fold more efficient than Cxcr3-deficient cells at migrating to the BAL and lung (Figure 3D, middle graph). However, both the number and relative recruitment of wild-type and Cxcr3-/- cells was similar in the MLN, demonstrating that these differences are specific for the lung. Notably, while migration of Cxcr3-deficient CD4+ T cells to these sites was severely impaired, migration was not completely abolished. We considered the possibility that CCR5 may be compensating for a lack of CXCR3 expression, allowing for low levels of T cell migration. To test this hypothesis, we investigated the recruitment of Ccr5 × Cxcr3-double deficient cells. However, there was no further impairment in recruitment to the lung compared to cells that lacked expression of CXCR3 only (Figure 3C, 3D, right graphs). Thus, CXCR3, but not CCR5, controls virus-specific effector CD4+ T cell recruitment to the lung airways and lung parenchyma during the peak of an adaptive immune response.
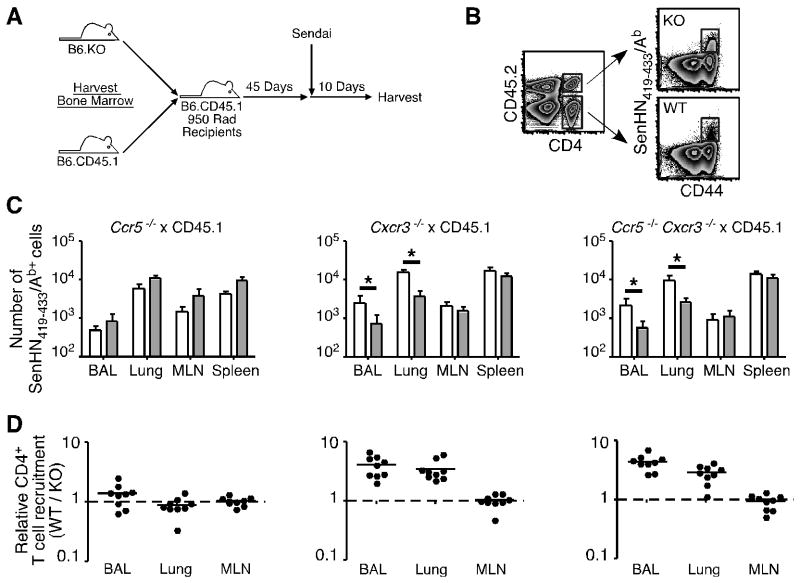
(A) Experimental design for the generation and infection of mixed BM chimeras. Chimeras were generated with BM from wild-type congenic (CD45.1+) and either Ccr5-/-, Cxcr3-/-, or Ccr5-/- × Cxcr3-/- (all CD45.2+) mice. (B) Donor CD4+ T cells were separated based on congenic marker expression, and antigen-specific chemokine receptor-deficient (KO) or wild-type (WT) cells were identified by SenHN419-433Ab multimer staining. Representative staining and gating strategy from the lung is shown. (C, D) Mixed BM chimeras with wild-type and Ccr5-/- (left graphs), Cxcr3-/- (middle graphs), or Ccr5-/- Cxcr3-/- (right graphs) donor cells were generated and infected with Sendai virus. (C) On day 10 post-infection, the number of SenHN419-433Ab+ wild-type (open bars) and chemokine receptor-deficient (shaded bars) cells in each tissue is graphed as the mean ± s.d. (D) The relative recruitment of wild-type versus chemokine receptor-deficient (WT / KO) antigen-specific CD4+ T cells is graphed for individual mice. The data are representative of 3 independent experiments for each set of chimeras.
To confirm the validity of the mixed BM chimera model and investigate whether CXCR3 controls the recruitment of other lymphocyte populations to the lung during a Sendai virus infection, we also examined wild-type and CXCR3-deficient antigen-specific CD8+ T cells, NK cells, and NKT cells. Similar to CD4+ T cells, both wild-type and Cxcr3-deficient CD8+ T cells were able to generate a robust response to the immunodominant SenNP324-332Kb epitope (Figure 4A). In agreement with published data in other respiratory virus models, we also observed significantly decreased numbers and impaired recruitment of virus-specific CD8+ T cells to the lung in the absence of CXCR3 (Figure 4B). To investigate whether the recruitment of other lymphocytes to the lung at the peak of the immune response was also controlled by CXCR3, we examined NK (NK1.1+ TCRβ-) and NKT (NK1.1+ TCRβ+) cells in the mixed BM chimera model (Figure 4C). In contrast to virus-specific T cells, there was no impairment in NK and NKT cell numbers or relative recruitment in the absence of CXCR3 (Figure 4D, 4E). Thus, both CD4+ and CD8+ effector T cells, but not NK and NKT cells, require CXCR3 for optimal recruitment to the lung during Sendai virus infection.
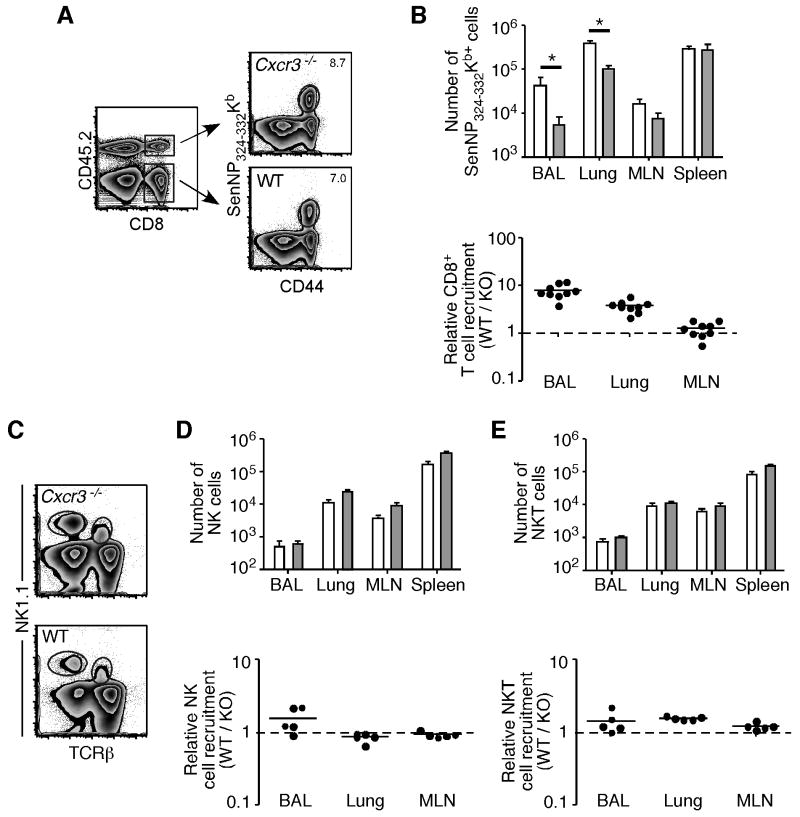
(A) Mixed BM chimeras (Cxcr3-/- × CD45.1) were sacrificed on day 10 post-infection and wild-type or Cxcr3-deficient antigen-specific CD8+ T cells were identified by SenNP324-332Kb tetramer staining. (B) The number (top graph) of wild-type (open bars) and Cxcr3-deficient (shaded bars) SenNP324-332Kb-specific CD8+ T cells is graphed as the mean ± s.d., and relative recruitment (bottom graph, WT / KO) is graphed for individual mice. (C) Representative staining for NK and NKT cells gated on total CD45.2+ (top plot, Cxcr3-/-) or CD45.2- (bottom plot, WT) lymphocytes. (D,E) The number (top graphs) of wild-type (open bars) and Cxcr3-deficient (shaded bars) NK cells (D) and NKTcells (E) is graphed as the mean ± s.d., and relative recruitment (bottom graph, WT / KO) is graphed for individual mice. The data in A and B are representative of 3 independent experiments, and the data in C-E are representative of 2 independent experiments.
Effector CD4+ T cell proliferation, phenotype, and cytokine production is not impacted by CXCR3
The quality of an adaptive immune response, whether generated by infection or vaccination, can be directly correlated to the ability of individual cells to elicit multiple effector functions (31). As it has been reported that CXCR3 signaling can influence the proliferation and activity of Th1-polarized CD4+ T cells the lung (32), we investigated the functional capacity of individual wild-type and Cxcr3-/- effector CD4+ T cells within the same host at the peak of the adaptive immune response. Mixed BM chimeras were administered BrdU on day 9 post-Sendai virus infection, and BrdU incorporation by antigen-specific CD4+ T cells was measured 24 hours later (Figure 5A). Within all tissues examined, antigen-specific CD4+ T cells lacking CXCR3 showed equivalent or slightly higher incorporation of BrdU than wild-type cells (Figure 5B). Similar data was obtained at day 8 post-Sendai virus infection (not shown), demonstrating that Cxcr3 deficiency does not impair the proliferation of virus-specific CD4+ T cells. We also examined the expression of cell surface markers associated with acute activation and cell adhesion in the lung (33-35). Similar to BrdU incorporation, there was no difference in expression of the activation markers CD27, CD43, CD69, and KLRG1 and the adhesion molecules CD49b and PSGL-1 between wild-type and Cxcr3-deficient antigen-specific CD4+ T cells (Figure 5C).
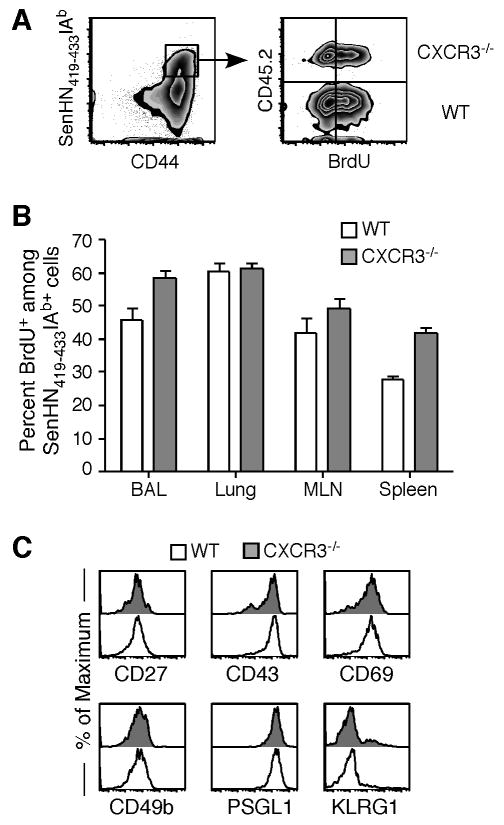
Mixed BM chimeras (Cxcr3-/- × CD45.1) were administered BrdU on day 9 post-infection and cells were harvested from the indicated tissues 24 hours later. (A) Representative multimer (gated on CD4+ cells) and BrdU staining (gated on SenHN419-433Ab+ cells) from the lung is shown. The quadrant gates were set based on isotype control antibody staining. (B) The frequency of BrdU+ cells among wild-type (open bars) or Cxcr3-/- (shaded bars) SenHN419-433Ab+ cells is graphed as the mean ± s.d. from 5 individual mice. (C) The expression of CD27, CD43, CD69, CD49b, PSGL1, and KLRG1 on wild-type (open histograms) and Cxcr3-/- (shaded histograms) cells was measured by flow cytometry. All plots are gated on SenHN419-433Ab+ cells. The data are representative of 2 independent experiments.
To investigate the effector functions of wild-type and Cxcr3-deficient cells in the lung, we measured the production of Th1 cytokines by CD4+ T cells following stimulation with SenHN419-433 peptide. Both wild-type and Cxcr3-/- antigen-specific cells in the lung parenchyma produced IFN-γ, TNF-α, and IL-2 in response to antigen stimulation (Figure 6A). Gating on IFN-γ+ wild-type or Cxcr3-/- cells, the distribution of cells producing additional cytokines was similar between wild-type and Cxcr3-/- populations (Figure 6B). In addition, wild-type and Cxcr3-/- cells from the lung produced only minimal amounts of IL-10, and no detectable IL-17, following peptide stimulation (data not shown). Thus, together with Figure 5, these data demonstrate that the absence of CXCR3 did not impact the functional capacity of antigen-specific CD4+ T cells in the lung.
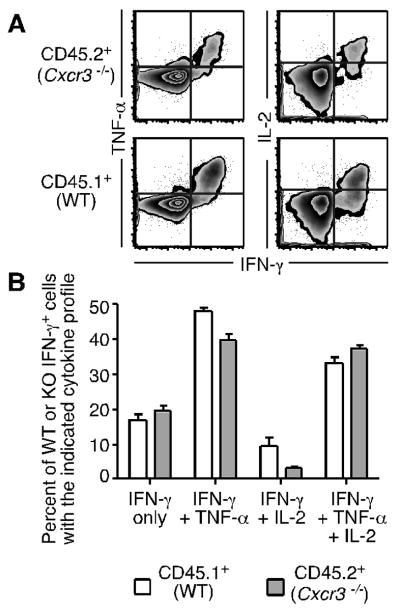
(A) Cells from the lungs of Cxcr3-/- × CD45.1 mixed BM chimeras on day 10 post-infection were stimulated with SenHN419-433 peptide and the expression of IFN-γ, TNF-α, and IL-2 was measured by flow cytometry. Representative staining gated on CD4+ CD44hi Cxcr3-/- or wild-type cells is shown. (B) After gating on IFN-γ+ cells, the frequency of wild-type (open bars) or Cxcr3-/- (shaded bars) cells expressing additional cytokines is graphed as the mean ± s.d. The data are representative of 3 independent experiments.
Discussion
Previous findings have shown that respiratory virus-specific CD4+ T cells have a diverse range of effector functions, and in the case of influenza and parainfluenza virus infections can directly contribute to pathogen clearance in the lung. In the present study, we demonstrate that polyclonal antigen-specific CD4+ T cells generated following Sendai virus infection express the chemokine receptor CXCR3. Utilizing mixed BM chimeras to directly compare wild-type and chemokine receptor-deficient cells in the same host, we show that the significant decrease in numbers of CXCR3-deficient virus-specific CD4+ T cells in the lung is due solely to a defect in recruitment. Furthermore, role of CXCR3 on lymphocyte recruitment to the lung at the peak of the adaptive immune response was restricted to virus-specific T cells, as neither NK cell nor NKT cell recruitment was decreased in the absence of CXCR3.
The importance of virus-specific T cell responses for protection from respiratory viruses was shown in seminal studies utilizing mice lacking B cells (36, 37). In both studies, virus-specific effector CD4+ T cells were able to confer at least partial protection even in the absence of effector CD8+ T cells. Building on these original observations, numerous studies have identified mechanisms that effector CD4+ T cells employ to limit viral replication. One common theme among the investigations of effector functions is that the accumulation of virus-specific CD4+ T cells in the lung is essential for their protective effect. We have identified an important factor controlling the accumulation of virus-specific CD4+ T cells in the lung by demonstrating that, when directly comparing wild-type and chemokine receptor-deficient cells under identical conditions in vivo, there is a 5-10 fold defect in the number of effector CD4+ T cells in the lung in the absence of CXCR3. One potential explanation for this observation was a difference in proliferation of wild-type and Cxcr3-deficient within the lung itself. Indeed, it was previously shown that lung-resident RSV-specific CD4+ T cells undergo rapid expansion following virus challenge (38). However, the methodology we employed required effector CD4+ T cells to first be generated from naïve T cell precursors in the lung-draining lymph nodes, and these cells were therefore not present in the lung at the time of infection. Furthermore, both wild-type and Cxcr3-deficient Sendai-specific CD4+ T cells showed similar BrdU incorporation in both the lung and lymphoid tissues at the peak of the adaptive response. Thus, our data support a model where, following initial antigen encounter and proliferation in the lymph nodes, virus-specific effector CD4+ T cells enter the circulation and then utilize CXCR3 to extravisate into the infected lung.
An interesting finding from these experiments is that CCR5 does not appear to be important for migration of effector CD4+ T cells to either the lung airways or lung parenchyma. Although this finding is supported by data from other pulmonary disease models such as asthma or COPD, it has been reported that CCR5 is important for the recruitment of CD4+ T cells to the lung during active tuberculosis (39). One possible explanation for this discrepancy is the unique inflammatory environment established in the lung during an active Mycobacterium tuberculosis infection (40). Indeed, purified protein derivative of Mycobacterium tuberculosis instilled in the lungs of subjects with no history of active tuberculosis resulted in the expression of CXCR3-, but not CCR5-, binding chemokines, suggesting that the subsequent recruitment of CD4+ T cells to the lung would depend on CXCR3 (41). In the case of acute respiratory virus infections, because ligands for both CCR5 and CXCR3 are highly induced, the lack of a role for CCR5 in the migration of effector CD4+ T cells to the lung may simply be attributed to the observation that very few antigen-specific T cells express this chemokine receptor.
In summary, we demonstrate that CXCR3, but not CCR5, is important for the migration of antigen-specific effector CD4+ T cells to both the lung airways and lung parenchyma during the acute phase of a respiratory virus infection. In addition, the influence of CXCR3 was limited to recruitment, as it did not impact the ability of effector T cells in the lung to produce multiple cytokines. Therefore, both CD8+ and CD4+ virus-specific effector T cells require CXCR3 for optimal migration to the lung.
Acknowledgments
We thank Drs. Marcy Blackman, Markus Mohrs, and Gary Winslow for critical review of the manuscript, and the Trudeau Institute Molecular Biology Core for production of tetramer and multimer reagents.
Abbreviations
- BM
- bone marrow
- EID50
- 50% egg infectious dose
- BAL
- bronchoalveolar lavage
- MLN
- mediastinal lymph node
Footnotes
1This work was supported by National Institutes of Health grants AI67967, AI76499, and T32 AI49823 to D.L.W., F32 AI71478 to J.E.K., and funds from the Trudeau Institute.
References
Full text links
Read article at publisher's site: https://doi.org/10.4049/jimmunol.0902022
Read article for free, from open access legal sources, via Unpaywall:
https://www.jimmunol.org/content/jimmunol/183/7/4378.full.pdf
Free after 12 months at www.jimmunol.org
http://www.jimmunol.org/cgi/reprint/183/7/4378
Free to read at www.jimmunol.org
http://www.jimmunol.org/cgi/content/abstract/183/7/4378
Free after 12 months at www.jimmunol.org
http://www.jimmunol.org/cgi/content/full/183/7/4378
Citations & impact
Impact metrics
Citations of article over time
Alternative metrics

Discover the attention surrounding your research
https://www.altmetric.com/details/162108713
Smart citations by scite.ai
Explore citation contexts and check if this article has been
supported or disputed.
https://scite.ai/reports/10.4049/jimmunol.0902022
Article citations
Principles and therapeutic applications of adaptive immunity.
Cell, 187(9):2052-2078, 01 Apr 2024
Cited by: 7 articles | PMID: 38670065 | PMCID: PMC11177542
Review Free full text in Europe PMC
Interferon lambda in respiratory viral infection: immunomodulatory functions and antiviral effects in epithelium.
Front Immunol, 15:1338096, 01 Mar 2024
Cited by: 1 article | PMID: 38495892 | PMCID: PMC10940417
Review Free full text in Europe PMC
The chemokine receptor CXCR3 promotes CD8<sup>+</sup> T cell-dependent lung pathology during influenza pathogenesis.
Sci Adv, 10(1):eadj1120, 03 Jan 2024
Cited by: 2 articles | PMID: 38170765 | PMCID: PMC10776024
CXCR3 Provides a Competitive Advantage for Retention of Mycobacterium tuberculosis-Specific Tissue-Resident Memory T Cells Following a Mucosal Tuberculosis Vaccine.
Vaccines (Basel), 11(10):1549, 29 Sep 2023
Cited by: 1 article | PMID: 37896952 | PMCID: PMC10611282
Quantitative analyses of T cell motion in tissue reveals factors driving T cell search in tissues.
Elife, 12:e84916, 23 Oct 2023
Cited by: 1 article | PMID: 37870221 | PMCID: PMC10672806
Go to all (90) article citations
Data
Similar Articles
To arrive at the top five similar articles we use a word-weighted algorithm to compare words from the Title and Abstract of each citation.
Opposing effects of CXCR3 and CCR5 deficiency on CD8+ T cell-mediated inflammation in the central nervous system of virus-infected mice.
J Immunol, 175(3):1767-1775, 01 Aug 2005
Cited by: 35 articles | PMID: 16034118
Pathogenesis of herpetic stromal keratitis in CCR5- and/or CXCR3-deficient mice.
Curr Eye Res, 33(9):736-749, 01 Sep 2008
Cited by: 16 articles | PMID: 18798077
Antibody-independent antiviral function of memory CD4+ T cells in vivo requires regulatory signals from CD8+ effector T cells.
J Immunol, 167(3):1379-1386, 01 Aug 2001
Cited by: 32 articles | PMID: 11466356
CD4+ T cell priming accelerates the clearance of Sendai virus in mice, but has a negative effect on CD8+ T cell memory.
J Immunol, 164(6):3274-3282, 01 Mar 2000
Cited by: 29 articles | PMID: 10706720
Funding
Funders who supported this work.
NIAID NIH HHS (12)
Grant ID: R01 AI076499-02
Grant ID: F32 AI071478
Grant ID: T32 AI049823
Grant ID: T32 AI049823-09
Grant ID: F32 AI071478-01A1
Grant ID: AI76499
Grant ID: R01 AI067967
Grant ID: R01 AI067967-04
Grant ID: AI67967
Grant ID: F32 AI71478
Grant ID: R01 AI076499
Grant ID: T32 AI49823