Abstract
Free full text

Gli2 trafficking links Hedgehog-dependent activation of Smoothened in the primary cilium to transcriptional activation in the nucleus
Abstract
Stimulation by the extracellular Hedgehog (Hh) protein signal has been shown to alter ciliary localization of the mammalian Hh receptor components Smoothened (Smo) and Patched (Ptc), and mutations that disrupt the structure and function of the cilium also disrupt Hh-induced changes in gene expression. But how ciliary events affect gene expression in the nucleus is not known, and to address this question we have characterized the cellular trafficking of Gli2, the principal mediator of Hh-dependent transcriptional activation. From a combination of pharmacological and genetic manipulations we find in resting cells that both Gli2 and Smo appear to shuttle in and out of the cilium, with Gli2 but not Smo requiring intact cytoplasmic microtubules for ciliary entry and both requiring the ciliary retrograde motor, cytoplasmic dynein 2, for ciliary exit. We also find that changes in ciliary and nuclear trafficking of Gli2 are triggered by the Hh-dependent accumulation of activated Smo in the cilium, resulting in a shift from primarily cytoplasmic localization to accumulation at the distal tip of the cilium and within the nucleus. Gli2 thus functions as a dynamic monitor of Smo activity in the cilium and thereby links Hh pathway activation in the cilium to transcriptional activation in the nucleus.
Hh signaling organizes pattern formation in the embryonic limb, neural tube, and other structures (1–4), and also functions postembryonically in homeostatic processes such as tissue maintenance and regeneration (5, 6). Hh pathway dysfunction thus can lead to birth defects such as holoprosencephaly (HPE) (7) or proliferative disorders such as the growth of malignant tumors (8). Given its fundamental importance in development, physiology, and disease, this signaling pathway has been the object of intensive study over many years; surprising new findings regarding the biology and mechanism of signaling nevertheless continue to emerge. Recent studies, for example, have highlighted the importance of the primary cilium in transduction of mammalian Hh signals, despite no prior hint of such involvement in Drosophila (9, 10).
Hh ligand activates the pathway by binding to Ptc and relieving its suppression of Smo, which in turn leads to a block of repressor formation and activation of the full-length Gli/Ci proteins. In Drosophila all Hh-dependent transcriptional activation and repression activities are carried out by Ci, but in mammals these functions are subdivided among the Gli proteins. Thus, although Gli2 and Gli3 each can be found in full-length or proteolytically processed forms (11–14), transcriptional activation is primarily executed by Gli2, and transcriptional repression by Gli3 (14–16); Gli1 is nonessential and functions as an amplifier of the activated state (17). Pathway activation ultimately results in Gli-mediated activation of transcriptional targets of the Hh pathway, including Ptc and Gli1 (18).
The first indication as to the potential role of cilia in mammalian Hh signaling emerged from genetic screens in the mouse, which identified mutations affecting intraflagellar transport proteins (IFTs) as producing patterning defects like those of mutations in genes encoding Hh pathway components (9, 19). These genetic studies also identified Hh-related phenotypes associated with mutations that affect motors that function in ciliary transport, and other mutations that affect ciliary structure. A role for cilia is also consistent with a demonstrated requirement for high cell density or serum starvation for Hh response in cultured cells (20), as these conditions promote cell cycle arrest and outgrowth of the primary cilium (21).
Several studies have now confirmed that Smo appears to concentrate within the primary cilium upon Hh stimulation or upon treatment with pharmacological agents such as cyclopamine or SAG1 that bind Smo and either antagonize or stimulate its activity (22–26). A prominent concentration of Gli1, Gli2, and Gli3 at the tip of the cilium regardless of treatment with ShhN has been noted in cells virally transduced for expression of these proteins, and endogenous Gli3 has been detected at the ciliary tip in cultured primary limb bud cells in the absence of exogenous Hedgehog stimulation (27). Sufu, a critical regulator of Gli activity in mammalian transduction, similarly is concentrated at the ciliary tip of limb bud cells (27).
Among aspects of ciliary function in mammalian transduction not yet understood, a fundamental question is how Smo activity in the cilium might affect gene expression in the nucleus. Is the presence of activated Smo in the cilium required for modulation of Gli activity? If so, how does activated Smo transmit information to the Gli proteins, and how do Gli proteins respond? Antibody reagents capable of monitoring endogenous Gli proteins, particularly the major positive transcriptional effector of the pathway, Gli2, have only recently been reported. We therefore approached this issue by introducing an epitope-tagged Gli2 protein at a level of expression that retains physiological regulation, including Hh-sensitive proteolytic processing and Hh-dependent target gene activation, and have examined the effects of Hh stimulation on the ciliary and nuclear trafficking of this protein.
Results
High-Level Gli2 Expression Overwhelms Pathway Regulation.
As reported previously in limb bud mesenchymal cells (27), we noted in NIH 3T3 cells that exogenously introduced Gli2 was concentrated in cilia; we also noted the presence of Gli2 throughout the cytoplasm and in the nucleus (Fig. 1A). In these experiments, however, luciferase expression from a cotransfected Hh-dependent reporter occurred at maximal levels in the absence of Hh stimulation, with no increase in expression upon addition of ShhN protein (Fig. 1B, 50%). This high basal level of reporter activity associated with overexpression of Gli2 also is not affected by the Smo antagonist cyclopamine. This is in sharp contrast to ShhN-induced reporter activity observed in the absence of exogenous Gli2 (Fig. 1B, EGFP) and suggests that normal regulation of Gli2 is circumvented by overexpression of the protein. Indeed, decreasing levels of transfected Gli2 construct restored Hh-dependent reporter activation, and the induced reporter activity exceeded that achieved without Gli2 transfection (Fig. 1B), suggesting that at least some exogenously introduced Gli2 is regulated by normal Hh pathway mechanisms. Consistent with regulation by Hh signaling, all reporter activity above basal unstimulated levels was suppressed by addition of cyclopamine.
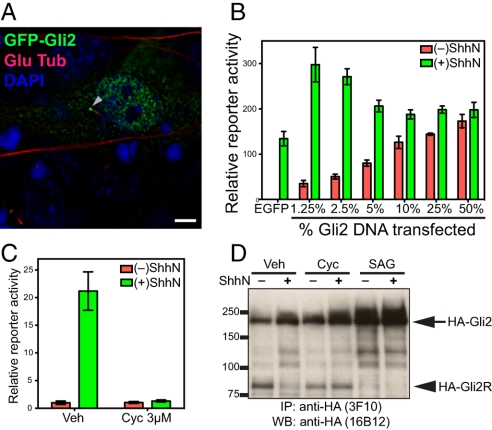
Physiological regulation of exogenously introduced Gli2. (A) NIH 3T3 cells were transiently transfected for expression of GFP-Gli2 and stained 48 h later. Overexpressed Gli2 is localized in the cytoplasm, at one end of the primary cilium (visualized with anti-detyrosinated tubulin), and in the nucleus (visualized with DAPI). (Scale bar, 5 μm.) (B) NIH 3T3 cells transfected for expression of Gli2 show that high levels produce unregulated reporter expression irrespective of ShhN stimulation, whereas lower levels can contribute to increased reporter expression with regulation by ShhN. Gli-luciferase reporter and control SV40-Renilla luciferase were cotransfected with the indicated proportion of Gli2 DNA (% of total DNA, wt/wt). Following transfection, cells were grown to confluency, incubated with Shhn, and assayed for reporter activity. Error bars indicate SD. (C) A Hh-responsive NIH 3T3/HA-Gli2 clone. An NIH 3T3 cell clone with a stably integrated construct for expression of HA-Gli2 was established and chosen for its low level of HA-Gli2 expression and assayed with Gli-luciferase reporter for response to ShhN stimulation. This clone displays Hh-responsive, cyclopamine-suppressible pathway activity. Error bars indicate SD. (D) 3T3/HA-Gli2 cells were incubated in the absence or presence of ShhN with vehicle (Veh), cyclopamine (Cyc), or SAG1, and then lysed and analyzed by IP-Western with anti-HA antibodies. Markers of molecular mass are indicated on the Left, and migration of full-length Gli2 (HA-Gli2, arrow) and its repressor form (HA-Gli2R, arrowhead) on the Right.
Given that exogenously introduced Gli2 expressed at lower levels is likely to be regulated by Hh pathway signaling, we constructed NIH 3T3 cell clones with stably integrated HA-Gli2 expression constructs and selected for further study one with low HA-Gli2 expression. This line lacked constitutive activity of a transfected Hh-sensitive reporter, but such reporter activity was induced by ShhN and fully inhibited by cyclopamine (Fig. 1C). Upon addition of ShhN or Hh agonist SAG1 the level of the HA-Gli2 full-length protein increased and that of HA-Gli2R significantly decreased (Fig. 1D), the latter largely disappearing within a few hours of stimulation (Fig. S1). The disappearance of HA-Gli2R in the presence of ShhN was prevented by cyclopamine (Fig. 1D). The kinetics of the shift between full-length and repressor forms are consistent with previously reported response to Hh stimulation (28), and along with data described above, further reinforce the conclusion that HA-Gli2 in this cell line comes under normal physiological regulation.
ShhN-Induced Accumulation of Gli2 in the Primary Cilium and in the Nucleus.
By indirect immunofluorescence staining, we noted that HA-Gli2 in this cell line was localized in faint cytoplasmic puncta, often in association with microtubules (see also ref. 29), but not in the primary cilium or the nucleus (Fig. S2A). As this differs from previously reported localization of overexpressed Gli2 and our own experiments with overexpressed HA-Gli2, we examined the effects of ShhN stimulation on HA-Gli2 localization. We noted a dramatic accumulation of HA-Gli2 in the primary cilium, mostly in a single aggregate at one end (Fig. 2A; Fig. S2A). This aggregate consistently was located at the opposite end of the cilium from the site of staining for γ-tubulin, which appears as a doublet at the base (30), thus indicating accumulation of HA-Gli2 at the distal tip (Fig. S2A; quantification in Fig. 2D; and Fig. S2 D and E). By immunostaining NIH 3T3 cells with a recently generated antibody (29), we were able to verify that endogenous Gli2 accumulates at the tip of the primary cilium upon Hh stimulation (Fig. S3).
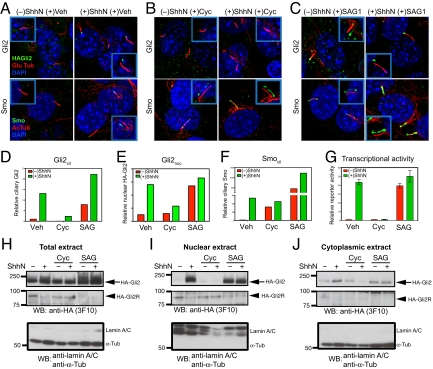
Gli2 accumulate at the primary cilium and the nucleus upon Hh stimulation, and pharmacological manipulation of Smo activity in cilia affects ciliary and nuclear accumulation and transcriptional activity of Gli2. (A–F) NIH 3T3/HA-Gli2 cells were incubated with or without ShhN in the presence of vehicle control (Veh), cyclopamine (3 μM) or SAG1 (400 nM) for 24 h. Cells were then stained to visualize Gli2, Smo, the primary cilium, and the nucleus as indicated. The Insets show shifted overlays. (A) ShhN-induced accumulation of Gli2 and Smo at the primary cilium. NIH 3T3/HA-Gli2 cells were grown to confluency and incubated in the presence or absence of ShhN; cells were stained to visualize HA-Gli2, the primary cilium (Glu tubulin), and the nucleus (DAPI) in Upper panels, or Smo, the primary cilium (acetylated tubulin), and the nucleus (DAPI) in Lower panels. (B) Cyclopamine induces Smo accumulation in the primary cilium but inhibits that of Gli2. (C) Smo agonist SAG1 induces accumulation of Gli2 and Smo in the cilium. (D–F) The amount of Gli2 in cilia (Gli2cil) and Gli2 in nuclei (Gli2nuc), Smo in cilia (Smocil) were quantified from complete Z-series of immunofluorescence images. (G) Gli-luciferase reporter activity was assayed in parallel. Error bars indicate SD. (H–J) Total extract, nuclear and cytoplasmic fractions were prepared from cells grown in parallel with A–F and analyzed by immunoblotting with anti-HA antibody. The Upper and Middle panels show full-length HA-Gli2 (HA-Gli2, arrow) and its repressor form (HA-Gli2R, arrowhead), respectively. Note the reciprocal accumulation of HA-Gli2 and HA-Gli2R in the absence or presence of ShhN in the nuclear extract. In the Lower panel, immunoblotting of the same extracts with a mixture of antibodies specific to lamin A/C and α-tubulin shows that only the nuclear extracts contain lamin A/C, and only the cytoplasmic extracts contain α-tubulin. Note that the total extract shows a strong α-tubulin band and also a faint lamin A/C band.
HA-Gli2 also accumulated in the nucleus, appearing there as small puncta (Fig. S2C; quantification in Fig. 2E; and Fig. S2F). To confirm these observations biochemically, we carried out subcellular fractionation of NIH 3T3/HA-Gli2 cells. We noted in total cell extracts that the full-length Gli2 protein, HA-Gli2, increased upon treatment with ShhN, whereas the processed form of HA-Gli2, HA-Gli2R, decreased to nearly undetectable levels (Fig. 2H). In nuclear and cytoplasmic extracts from cells treated in parallel, the HA-Gli2R protein was predominantly localized in the nuclei of unstimulated cells, which contained little or no detectable HA-Gli2. This situation was dramatically reversed in nuclear extracts from ShhN-stimulated cells, as HA-Gli2 dramatically increased and HA-Gli2R was much reduced (Fig. 2I; Fig. S2G). Stimulation by ShhN caused little change in the cytoplasmic fraction, as HA-Gli2 was present whereas HA-Gli2R was scarcely detectable, regardless of stimulation (Fig. 2J).
Pharmacological or Mutational Activation of Smo Triggers Changes in Gli2 Trafficking.
Hh stimulation alters Gli2 trafficking to cause accumulation at the ciliary tip and in the nucleus. These changes in Gli2 trafficking coincide with Hh-induced ciliary accumulation of Smo, suggesting the possibility of a causal link, and to explore this possibility we tested various pharmacological or mutational manipulations of Smo for their effects on Gli2 trafficking and transcriptional activity.
SAG1, a pathway agonist that activates the pathway without Hh stimulation by binding directly to Smo and stabilizing its active conformation (31, 32), causes Smo to accumulate in the cilium (Fig. 2 C and F); SAG1 treatment also changes Gli2 trafficking so that it accumulates at the tip of the cilium and in the nucleus (Fig. 2 C–E). The nuclear extracts from SAG-treated cells contained predominantly the full-length active form, HA-Gli2 (Fig. 2I). The amount of nuclear full-length Gli2 correlated very well with the extent of reporter activation (Fig. 2G; Table S1). Purmorphamine, a second Smo agonist, also causes ciliary accumulation of Smo, Gli2 accumulation at the ciliary tip, and activation of a Gli transcriptional reporter (Table S1).
Similar effects on Gli2 trafficking were produced by transfection of SmoA1, a mutationally altered, constitutively activated murine form of oncogenic Smo that is resistant to inhibition by Ptc and therefore activates the pathway without Hh stimulation. The SmoA1 variant expressed from a transfected construct accumulates in the cilium irrespective of ShhN stimulation and alters Gli2 trafficking to produce ciliary accumulation of Gli2 and activation of the Gli transcriptional reporter (Fig. 3; Table S1).
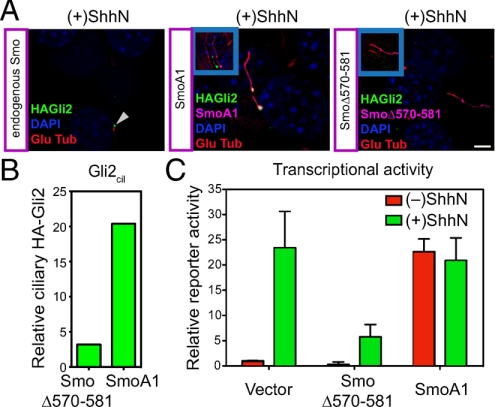
Mutational manipulation of Smo activity in cilia affects ciliary accumulation and transcriptional activity of Gli2. (A) NIH 3T3/HA-Gli2 cells were transfected with constructs for expression of mutant activated Smo (SmoA1) or a mutant dominant-negative Smo (SmoΔ570–581) (see Fig. S4). Cells were incubated with ShhN and stained for Smo and Gli2. As an internal control, the Left panel shows a cilium (arrowhead) from an untransfected cell (containing only endogenous Smo) in a SmoΔ570–581 transfection experiment. The Insets show shifted overlays. (B) The levels of ciliary Gli2 (Gli2cil) in complete Z-series of immunofluorescence images for cells transfected either with dominant-negative Smo (SmoΔ570–581) or activated Smo (SmoA1) in the presence of ShhN are shown. (C) Gli-luciferase reporter activity was assayed in parallel. Error bars indicate SD.
Ciliary Accumulation of Inactive Smo Fails to Trigger Changes in Gli2 Trafficking.
We also tested the effects of pharmacological inactivation of Smo. We confirmed, as reported previously (23), that the pathway antagonist SANT-1 (31) blocks ShhN-induced Smo accumulation at the primary cilium (Table S1); SANT-1 treatment also prevented ShhN-induced ciliary accumulation of Gli2. Cyclopamine is also a Smo antagonist, which surprisingly, despite a negative effect on Smo activity, has been reported not only to permit Hh-dependent Smo ciliary accumulation, but also to cause ciliary accumulation in the absence of Hh stimulation (23, 24).
We have confirmed that Smo indeed accumulates in the cilia of cyclopamine-treated cells under conditions of Hh stimulation, and to a significant level without Hh stimulation (Fig. 2 B and F; Table S1). Interestingly, however, we found that Gli2 in cyclopamine-treated cells with or without ShhN stimulation failed to accumulate at the ciliary tip and in the nucleus (Fig. 2 B, E, and I; Table S1). In contrast, the level of nuclear Gli2 repressor was maintained in cyclopamine-treated cells even in the presence of ShhN stimulation (Fig. 2I). These results suggest that the changes in Gli2 trafficking and transcriptional activity are dependent not only on ciliary accumulation of Smo, but also on its activity state in the cilium, and further suggest that the determinants required for Smo ciliary trafficking and accumulation may be distinct from those required for its activity in influencing Gli2 trafficking.
We also examined the behavior of SmoΔ570–581, an altered form of the Smo protein from which 12 residues of the cytoplasmic tail are deleted. The deleted portion of this variant encompasses six residues that when individually altered significantly disrupt the ability of Smo to rescue Hh responsiveness upon introduction into Smo−/− cells (33). The 12-residue deletion in SmoΔ570–581 also renders this variant unable to rescue Hh responsiveness in Smo−/− cells (Fig. S4A). Interestingly, this Smo variant when overexpressed in NIH 3T3 cells actually blocks ShhN-induced Gli reporter activation (Fig. 3C; Fig. S4B), suggesting that it may interfere with activity of the endogenous wild-type Smo. Although this Smo variant accumulates in the cilium of transfected cells, no changes in trafficking of HA-Gli2 (Fig. 3 A and B) can be produced by ShhN stimulation. This behavior reinforces the conclusion from the cyclopamine experiments that Hh-induced changes in Gli2 trafficking and activation of transcriptional targets requires critical input from Smo; although ciliary accumulation of Smo appears to be necessary for this input (see above), it is not sufficient if Smo is not activated.
Gli2 Appears to Shuttle in and out of Cilia.
To understand the dynamics of ciliary and nuclear Gli2 accumulation and their relationship to Smo, we analyzed the effects of manipulating the activities of motors involved in intraflagellar transport. In a mutant mouse lacking function of the cytoplasmic dynein 2 heavy chain (Dync2h1), a critical component of the motor required for retrograde transport of cargo out of cilia, it was previously reported that both activator and repressor functions of Gli were impaired and that Smo accumulation in cilia was defective (34). However, another study using mutant fibroblasts lacking function of Dync2h1 reported that Smo accumulated in the primary cilium even without Hh stimulation (35). We reproduced this ciliary accumulation of Smo in the absence of Hh stimulation by retroviral transduction of an shRNA targeting Dync2h1 into NIH 3T3/HA-Gli2 cells (Fig. 4). Our result is consistent with the hypothesis that Smo continuously shuttles in and out of cilium in the absence of Hh stimulation.
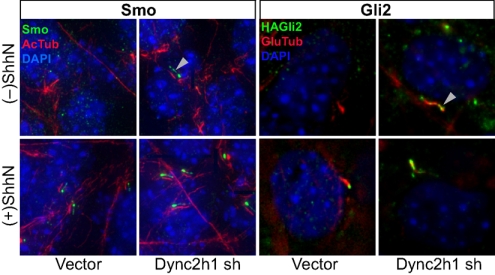
Gli2 shuttles in and out of cilia. NIH 3T3/HA-Gli2 cells infected with viruses from the LMP shRNA control vector or the same vector carrying cytoplasmic dynein 2 heavy chain (Dync2h1) shRNA were selected for puromycin resistance. Cells grown to confluency were incubated in the presence or absence of ShhN and stained for Smo or Gli2 as indicated. For greater clarity, Smo staining (green) in the Left four panels is shown as a shifted overlay with acetylated tubulin (red). Note the accumulation of both Smo and Gli2 in the primary cilium (white arrowheads) of cells infected with Dync2h1 shRNA viruses, even in the absence of ShhN, showing that impairment of the retrograde motor cytoplasmic dynein 2 causes ciliary accumulation of Smo and Gli2 in the primary cilium.
In these transduced NIH 3T3/HA-Gli2 cells we also observed Hh-independent accumulation of HA-Gli2 at the tip of the cilium (Fig. 4), often in a ball-shaped aggregate ranging in diameter from 1–3 μm (Fig. S5C), somewhat larger than that typically produced by ShhN stimulation. We note that despite Smo and Gli2 accumulation in the cilium in Dync2h1 shRNA-transduced cells in the absence of ShhN, there was little increase in expression of the Gli-luciferase reporter; these cells also showed significantly reduced Gli-luciferase reporter activity upon ShhN treatment (Fig. S6B). These results suggest that: (i) Smo and Gli2 are constitutively transported into the cilium; (ii) Smo and Gli2 are both dependent on the retrograde motor cytoplasmic dynein 2 for export, which prevents their accumulation in the cilium; and (iii) disruption of cytoplasmic dynein 2-based retrograde transport permits or enhances ShhN-stimulated ciliary accumulation of Smo and Gli2, but reduces nuclear accumulation of Gli2 and reporter activation.
Distinct Mechanisms for Gli2 and Smo Transport into Cilia.
We also investigated the effects of disrupting the anterograde ciliary motor Kif3 with the use of a Kif3a expression construct lacking the motor domain, which acts as a dominant-negative molecule (36). We found that transfection of this molecule reduced Hh-dependent reporter activation (Fig. S5B), consistent with a requirement for the Kif3a motor for Hh response in embryos (37, 38). Also consistent with a role in assembly of the primary cilium (39), cells with Kif3aDN overexpression were largely devoid of primary cilia (Fig. S5A), and we were therefore unable to systematically assess the role of Kif3a in ciliary transport of Smo or Gli2. We note that absence of cilia, as seen in cells at low density, also blocks Hh pathway activity (Fig. S7; see also refs. 20, 21).
Given the apparent association of at least some HA-Gli2 with cytoplasmic microtubules (29), we considered the possibility that disruption of cytoplasmic microtubules might affect processes required for transport of Gli2 into cilia. We found by testing increasing concentrations of vinblastine for periods up to 6 h that we were able to disrupt cytoplasmic microtubules while leaving primary cilia intact (Fig. 5), as previously reported (40). These increasing doses of vinblastine correspondingly blocked HA-Gli2 accumulation in cilia, culminating in a complete block at 100 nM vinblastine (Fig. 5B), which also efficiently inhibited pathway activation as measured with the Gli-luciferase reporter (Fig. 5D). These results confirm the importance of ciliary transport for Gli2 activation and further suggest that cytoplasmic microtubules are required for movement of Gli2 into cilia.
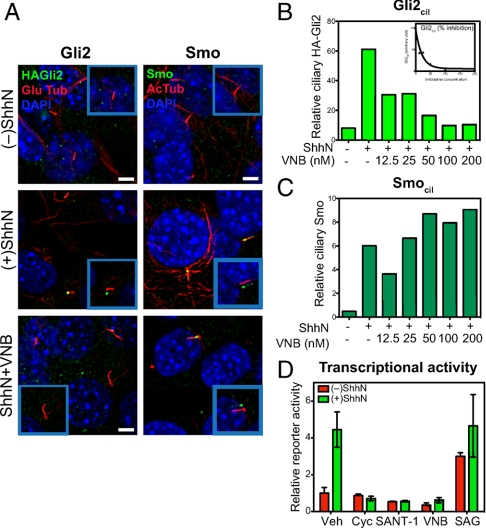
Microtubules are required for Gli2 but not Smo entry into cilia. (A) The microtubule inhibitor vinblastine (VNB) blocks ShhN-induced Gli2 accumulation in the primary cilium, but does not inhibit ciliary Smo accumulation. Cells incubated in the absence or presence of ShhN, or with 100 nM of VNB in the presence of ShhN for 6 h were stained for HA-Gli2 (Left panels) and Smo (Right panels). The Insets show shifted overlays. (Scale bar, 5 μm.) (B) The levels of Gli2 in cilia (Gli2cil) quantified from complete Z-series of immunofluorescence images are shown for cells incubated for 6 h with ShhN and increasing concentrations of VNB from 12.5 nM to 200 nM. (C) The levels of Smo in cilia (Smocil) in cells treated with ShhN and vinblastine were similarly determined. (D) Gli-luciferase reporter activity after 6 h of ShhN treatment in the presence of known pathway inhibitors (Cyc 3 μM, SANT-1 100 nM), VNB (100 nM), and an agonist SAG1 (400 nM). Note that 6 h of ShhN stimulation produces reduced but nevertheless measurable reporter activity, which is completely inhibited by VNB treatment at 100 nM. Error bars indicate SD.
Interestingly, microtubule disruption did not block Shh-induced Smo accumulation along the shaft, even at the high vinblastine concentrations that completely block Gli2 accumulation and Gli-luciferase reporter response (Fig. 5 A, C, and D; Fig. S2B). Our observations thus suggest that movement of Smo into cilia occurs by a distinct mechanism from that of Gli2, one which apparently does not require microtubule-dependent motors in the cytoplasm. Our results are consistent with a recent report that Gli2 localization in the primary cilium is somewhat reduced in embryonic fibroblasts lacking the kinesin family member, Kif7, whereas Smo appears to act upstream of Kif7 and its accumulation was not affected (41, 42). All of these data thus suggest that Smo and Gli2 have distinct requirements for ciliary trafficking and accumulation upon Hh stimulation.
Discussion
Ciliary Trafficking in the Gli2 Activity Cycle.
Although the events leading to Gli repressor formation are known to include specific site phosphorylation by several kinases, ubiquitinylation, and limited proteasomal processing, little is known of the molecular events leading to Gli protein activation. Gli activation is made even more intriguing by the apparent involvement of the primary cilium, an organelle not often associated with transcriptional modulation. In an effort to link ciliary events to transcriptional activation in the nucleus, we have focused here on the behavior of the major transcriptional activator of Hedgehog signaling in mammals, the Gli2 protein.
Our observations lead to the following view of mammalian Hh signal response. In the resting state Gli2, Smo, and perhaps other Hh pathway components appear to shuttle continuously in and out of the primary cilium. The presence of Gli2 and Smo in the primary cilia of resting cells is not apparent however, as the retrograde ciliary motor, cytoplasmic dynein 2, actively exports these proteins and prevents their accumulation. Gli2 thus appears to reside primarily within the cytoplasm in resting cells. Upon Hh stimulation the ciliary trafficking of both Smo and Gli2 is altered such that Smo accumulates within the cilium, and Gli2 accumulates primarily at the ciliary tip. We note that another group recently reported increased Gli2 localization in the cilium upon Hh stimulation (43).
Smo Activity State in Cilia Predicts Gli2 Nuclear Activity State.
The most critical aspect of Gli2 trafficking from the standpoint of target gene activation is its Hh-dependent accumulation within the nucleus, where it is responsible for Hh-induced changes in target gene transcription. We have shown biochemically that the Gli2 repressor dissipates and the Gli2 activator dramatically accumulates in the nuclei of Hh-stimulated cells. Our observations strongly suggest that a critical requirement for nuclear Gli2 accumulation is that ciliary trafficking must bring Gli2 into the cilium together with an activated form of Smo. The supporting evidence is as follows: first, all instances of nuclear Gli2 accumulation or Gli reporter activation that we have examined involve ciliary accumulation of activated Smo and ciliary accumulation of Gli2 (summarized in Table S1).
Second, manipulations that reduce formation of primary cilia, such as disruption of Kif3a function or cell growth at low density, reduce Hh-dependent transcriptional activation, even in the presence of pharmacologically or mutationally activated Smo or in the absence of the negative Smo regulator, Ptc. Thus, for example, transfection of SmoA1 failed to activate a Gli transcriptional reporter in cells plated at low density (20), and Ptc−/− cells do not show the typical constitutively high basal pathway activity at low density (Fig. S7B).
Third, Gli2 that accumulates in the nucleus appears likely to first pass through the cilium, as manipulations that trap Gli2 in the cilium by impairing its exit (shRNA against Dync2h1) or that prevent Gli2 ciliary entry (treatment with vinblastine) reduce Hh-induced transcriptional activation in the nucleus.
Finally, a number of pharmacological or genetic manipulations that prevent colocalization of Gli2 with activated Smo in the cilium all result in failure of Hh-dependent reporter activation. For example, in cells treated with ShhN and 100 nM vinblastine (Fig. 5), which permits ciliary accumulation of activated Smo but blocks ciliary entry of Gli2, reporter activation is blocked. In addition, Smo that accumulates in the cilium but is inactivated by cyclopamine treatment fails to cause nuclear accumulation of Gli2. Similarly, inactivation of Smo by a mutation that permits Hh-induced ciliary accumulation (SmoΔ570–581) fails to change Gli2 trafficking and activate Gli reporter expression. Taken together, these observations make a strong case that a key event in transcriptional activation of Hh pathway targets is for Gli2 to receive input from activated Smo in the cilium.
A Model: Gli2 as a Dynamic Monitor of the Ciliary Environment.
Our current evidence suggests that Hh stimulation imposes its effects on a dynamic background of active ciliary entry and exit by Smo, Gli2, and perhaps other pathway components. The Gli2 protein thus appears to continuously sample the ciliary compartment; upon Hh stimulation and consequent exposure to activated Smo in the primary cilium, Gli2 trafficking is altered such that it accumulates in the cilium and ultimately in the nucleus in active form. We do not yet know how directly activated Smo in the cilium affects Gli2, nor whether the effect is a covalent change or a noncovalent change in Gli2 association with other components. The consequence of this effect, however, appears to be an increased residence time at the ciliary tip and subsequent accumulation within the nucleus. In this model (Fig. S8), the unique feature of Gli2 relative to other signal-modulated transcription factors is its ability to dynamically monitor the signaling environment in the primary cilium. Under conditions of Hh stimulation, ciliary accumulation of activated Smo thus is read out as a Gli2 protein altered in its covalent structure or in its protein associations such that upon egress from the cilium it accumulates in the nucleus and there activates transcription of target genes. Further work will be required to define these changes and how they affect nuclear transport of Gli2.
Materials and Methods
Gli Reporter Assays.
NIH 3T3 cells were plated at 5–9 × 104 cells/well of 24-well plates and transfected the next day using FuGene6 (Roche) with Gli-luciferase reporter, control pRL-SV40 renilla luciferase, and other DNA constructs as indicated. After cells reached confluency in about 2 days, they were shifted to 0.5% serum medium and incubated 24 h with ShhN combined with additional treatments as indicated.
Immunofluorescence and Quantification of Microscopic Images.
Cells were plated onto 22-mm diameter circular coverslips in 6-well plates, and fixed either in cold methanol at −20 °C for 5 min or in 4% formaldehyde for 20 min. Cells were permeabilized with PBS/0.5% Triton X-100 for 3 min and nonspecific binding sites were blocked with 2% BSA in PBST (PBS with 0.5% Tween-20). Cells were stained with primary antibodies diluted in 2% BSA/PBST for 1 h at room temperature. After washing four times in PBST, cells were incubated for 1 h with appropriate Alexa 488-, Alexa 594-, or Alexa 680-conjugated secondary antibodies together with 1 μg/mL of 4′,6-diamidino-2-phenylindole (DAPI) for nuclear staining in 2% BSA/PBST. The amounts of ciliary Smo and Gli2 were determined by measuring Smo or Gli2 fluorescence intensity that colocalized with modified tubulin (acetylated or detyrosinated tubulin) and the amount of nuclear Gli2 by measuring Gli2 fluorescence intensity that colocalized with DAPI.
Further information is available in SI Materials and Methods.
Acknowledgments.
We thank Baolin Wang (Weil Medical College of Cornell University) for anti-Gli2 antibody that detects endogenous Gli2 proteins, Dale Gardner (U.S. Department of Agriculture) for cyclopamine, Trina Schroer (Johns Hopkins University) for motorless Kif3a dominant-negative (Kif3a-DN) construct. P.A.B. is an investigator of the Howard Hughes Medical Institute.
Footnotes
The authors declare no conflict of interest.
This article contains supporting information online at www.pnas.org/cgi/content/full/0912180106/DCSupplemental.
References
Articles from Proceedings of the National Academy of Sciences of the United States of America are provided here courtesy of National Academy of Sciences
Full text links
Read article at publisher's site: https://doi.org/10.1073/pnas.0912180106
Read article for free, from open access legal sources, via Unpaywall:
https://www.pnas.org/content/pnas/106/51/21666.full.pdf
Free after 6 months at www.pnas.org
http://www.pnas.org/cgi/content/abstract/106/51/21666
Free after 6 months at www.pnas.org
http://www.pnas.org/cgi/reprint/106/51/21666.pdf
Free after 6 months at www.pnas.org
http://www.pnas.org/cgi/content/full/106/51/21666
Citations & impact
Impact metrics
Citations of article over time
Article citations
Pleiotropy in FOXC1-attributable phenotypes involves altered ciliation and cilia-dependent signaling.
Sci Rep, 14(1):20278, 31 Aug 2024
Cited by: 0 articles | PMID: 39217245 | PMCID: PMC11365983
The senescence-associated secretome of Hedgehog-deficient hepatocytes drives MASLD progression.
J Clin Invest, 134(19):e180310, 27 Aug 2024
Cited by: 0 articles | PMID: 39190624 | PMCID: PMC11444248
mTORC1 hampers Hedgehog signaling in Tsc2 deficient cells.
Life Sci Alliance, 7(11):e202302419, 26 Aug 2024
Cited by: 0 articles | PMID: 39187374 | PMCID: PMC11349048
GRK2 kinases in the primary cilium initiate SMOOTHENED-PKA signaling in the Hedgehog cascade.
PLoS Biol, 22(8):e3002685, 13 Aug 2024
Cited by: 1 article | PMID: 39138140 | PMCID: PMC11322411
MI-181 Modulates Cilia Length and Restores Cilia Length in Cells with Defective Shortened Cilia.
ACS Chem Biol, 19(8):1733-1742, 06 Aug 2024
Cited by: 0 articles | PMID: 39106364 | PMCID: PMC11334112
Go to all (210) article citations
Data
Data behind the article
This data has been text mined from the article, or deposited into data resources.
BioStudies: supplemental material and supporting data
Similar Articles
To arrive at the top five similar articles we use a word-weighted algorithm to compare words from the Title and Abstract of each citation.
Bifurcating action of Smoothened in Hedgehog signaling is mediated by Dlg5.
Genes Dev, 29(3):262-276, 01 Feb 2015
Cited by: 21 articles | PMID: 25644602 | PMCID: PMC4318143
A central region of Gli2 regulates its localization to the primary cilium and transcriptional activity.
J Cell Sci, 127(pt 7):1500-1510, 24 Jan 2014
Cited by: 37 articles | PMID: 24463817 | PMCID: PMC3970560
Cilium-independent regulation of Gli protein function by Sufu in Hedgehog signaling is evolutionarily conserved.
Genes Dev, 23(16):1910-1928, 01 Aug 2009
Cited by: 252 articles | PMID: 19684112 | PMCID: PMC2725943
Hedgehog signaling from the primary cilium to the nucleus: an emerging picture of ciliary localization, trafficking and transduction.
Curr Opin Genet Dev, 23(4):429-437, 29 May 2013
Cited by: 91 articles | PMID: 23725801 | PMCID: PMC3913210
Review Free full text in Europe PMC
Funding
Funders who supported this work.