Abstract
Free full text

Plasma tissue factor may be predictive of venous thromboembolism in pancreatic cancer
Pancreatic cancer is the fourth leading cause of cancer death in the US [1]. It is frequently complicated by venous thromboembolism (VTE), with published incidence rates varying from 17% to 57% [2]. VTE can directly contribute to mortality, and has been associated with poor outcomes in pancreatic cancer [3].
Tissue factor (TF) is the principal physiologic initiator of coagulation and is commonly expressed in a variety of cancers. In pancreatic cancer, high grade TF expression is an adverse prognostic factor [4]. We recently published findings showing that TF expression occurs early in malignant transformation of the pancreas, is associated with angiogenesis and may be predictive of subsequent VTE [5]. Patients with high-grade TF expression had a symptomatic VTE rate of 26.3% compared with 4.5% in patients with low expression (P = 0.04). Similar findings have been reported in ovarian cancer [6]. Patients with metastatic pancreatic cancer have been shown to have increased levels of microparticle (MP)-associated TF activity compared with healthy controls [7].
We hypothesized that elevated circulating levels of TF would be associated with development of VTE in pancreatic cancer. We, therefore, measured TF in samples obtained from patients with locally advanced or metastatic pancreatic cancer enrolled in a prospective trial conducted by the University of Rochester Community Clinical Oncology Program. Selected sites participating in the trial also offered participation in a laboratory companion study from 2 October 2002 to 29 December 2006. The study was approved by an Institutional Review Board. All patients provided written informed consent. All patients were randomized to single-agent gemcitabine alone or with dalteparin 5000 anti-Xa units until progression. Blood collection was planned at baseline (prior to initiating chemotherapy) and every 4 weeks while on the study. Citrated plasma was processed within 1 h of collection by centrifuging at 2000 × g for 15 min at 8 °C to produce platelet-poor plasma (PPP; <10 000 platelets μL−1) and then stored at −80 °C.
TF antigen (Ag) levels were determined by an in-house ELISA from citrated plasma stored at −80 °C. Briefly, 100 μL of the mouse anti-human TF antibody, hTF1 (5 μg mL−1 in PBS), was coated onto 96-well ELISA plates (Nunc No. 439454) by overnight incubation at 4 °C. All subsequent washes and incubations were done at 25 °C. Plates were washed with PBS-Tween 20 and blocked with casein (Vector Labs No. SP-5020) for 15 min. Stored plasma samples were thawed, mixed, centrifuged at 350 × g for 1 min, and diluted 1:1 in casein. Serial dilutions of a recombinant soluble TF1–219 (sTF) standard were added to the plates in duplicate. One hundred microliters of the diluted plasma samples were added to the plates in triplicate, incubated at room temperature for 2 h and then washed. One hundred microliters of an sTF immuno-purified, biotinylated, F(ab′)2-fragmented rabbit anti-sTF anti-body (0.12 μg mL−1 in casein) was added to each well. The plates were incubated for 2 h and then washed. Streptavidin (Pierce No. 21126) (Pierce, Rockford, IL, USA) was diluted 1:10 000 and 100 μL was added to each well. The plates were incubated at room temperature for 30 min and then washed. One hundred microliters of the 1-Step Ultra TMB-ELISA reagent (Pierce No. 34028) was added to each well. The plates were incubated for 8 min and the reaction was stopped with 100 μL of 2 M sulfuric acid before reading the optical density (OD) at 450 nm in a 96-well reader (Spectramax190, Molecular Devices, Sunnyvale, CA, USA) from Molecular Devices using SoftmaxPro software (Molecular Devices, Sunnyvale, CA). TF concentrations were determined from a standard plot of the sTF protein concentrations and reported as pg mL−1 of sTF. To determine a normal level for TF Ag, we measured TF in plasma from 15 healthy volunteers and from a commercially available pooled plasma control (Innovative Research, Inc., Southfield, MI, USA) repeated six times in separate assays. Mean plasma TF was 27.0 pg mL−1 (SD 12.9) in healthy volunteers, and 26.9 pg mL−1 (SD 3.94) in pooled plasma control, with an interassay coefficient of variability (CV) of 14.7%.
TF was also measured using a procoagulant activity (PCA) assay in a similar manner to a recent study [7]. MPs were pelleted from 200 μL of PPP by centrifugation at 20 000 × g for 15 min at 4 °C, washed twice with HBSA (137 mM NaCl, 5.38 mM KCl, 5.55 mM glucose, 10 mM HEPES, 0.1% bovine serum albumin, pH 7.5), and re-suspended in 100 μL of HBSA. Samples were incubated with either hTF1 (4 μg mL−1; 1 μL) or a control antibody (mouse IgG: 4 μg mL−1; 1 μL) for 15 min at 25 °C and then 50 μL aliquots were added to duplicate wells of a 96-well plate. Next, 50 μL of HBSA containing 10 nM FVIIa, 300 nM FX and 10 mM CaCl2 was added to each sample and the mixture incubated for 2 h at 37 °C. FXa generation was stopped by the addition of 25 μL of 25 mM EDTA buffer and 25 μL of the chromogenic substrate S2765 (4 mM) was added and incubated at 37 °C for 15 min. Finally, absorbance at 405 nm was measured using a VERSAmax microplate reader (Molecular Devices). TF activity was calculated by reference to a standard curve generated using relipidated recombinant human TF (0–55 pg mL−1). The TF-dependent FXa generation (pg mL−1) was determined by subtracting the amount of FXa generated in the presence of hTF1 from the amount of FXa generated in the presence of the control antibody. Mean TF PCA in healthy controls was 0.21 pg mL−1 (SD 0.11) with an interassay CV of 21%.
Eleven patients provided at least one blood specimen (range, 1–8; median five samples per patient). TF Ag was measured in all 11 patients and TF MP PCA in 10 patients with available specimens. The mean TF Ag level (± SD) for all timepoints was 30.9 pg mL−1 (±15.4) and mean TF PCA was 0.85 pg mL−1 (±1.26). Mean TF Ag levels and MP-PCA at baseline were 56.6 and 0.95 pg mL−1, respectively. However, there was a wide variation, with baseline TF Ag levels ranging from 0 to 269 pg mL−1 (Fig. 1A) and TF MP PCA from 0 to 3.1 pg mL−1. Nine patients had levels of plasma TF Ag (32 ± 19 pg mL−1 SD) that were similar to those seen in healthy volunteers although TF MP PCA was elevated in these patients compared with controls (0.46 ± 1.26 pg mL−1, P = 0.01). In these patients, both TF levels and PCA remained stable throughout treatment. None of these patients developed VTE. Four of these nine patients had been randomized to dalteparin. In contrast, patient 5, randomized to dalteparin, had a rapid increase in TF Ag and PCA after visit 2, and patient 10 had TF Ag and MP PCA that were high at baseline and increased progressively through 3 months of chemotherapy (Fig. 1A). Both of these patients developed VTE. Patient 5 developed an occlusive popliteal deep venous thrombosis (DVT), confirmed by ultrasound, 15 days after discontinuing both gemcitabine and dalteparin due to progression of cancer. The patient subsequently presented 12 days later with sudden-onset shortness of breath while on anticoagulation with warfarin, was admitted and died during the hospital admission. An autopsy confirmed the presence of a massive pulmonary embolism (PE). The last TF Ag and MP PCA for patient 5, taken 15 days prior to the initial episode of DVT, were 377 and 4.4 pg mL−1, respectively. Patient 10, who was randomized to no prophylaxis, developed an ultrasound-confirmed peroneal vein DVT 56 days after starting chemotherapy. The patient then had extension into the femoral vein, confirmed by ultrasound, 26 days later while on therapeutic anticoagulation with warfarin. The last TF Ag and MP PCA for patient 10 taken on the day of diagnosis of DVT were 504 and 5.5 pg mL−1, respectively. When evaluating the entire study population, there was a significant association between TF Ag levels (P = 0.036) as well as TF MP PCA levels and development of VTE (P = 0.044). There was also a significant correlation between TF Ag and MP PCA assays (linear correlation coefficient 0.89, P < 0.0001; Fig. 1B).
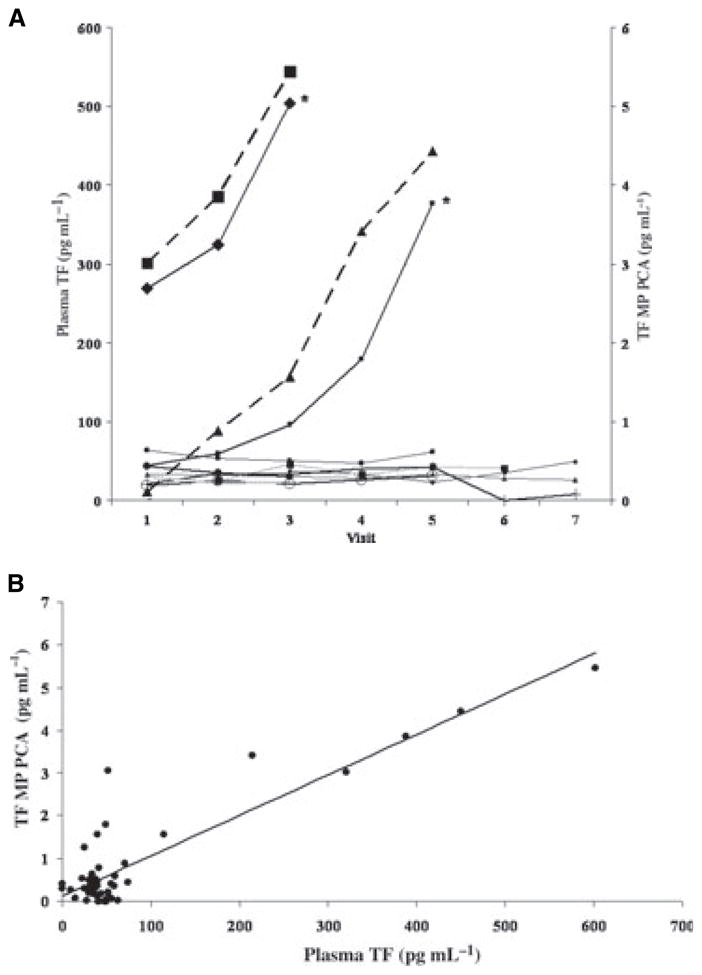
(A) Serial plasma TF Ag levels in 11 patients with locally advanced or metastatic pancreatic cancer receiving systemic chemotherapy. Patients 5 and 10, marked with an asterisk, developed VTE. TF MP PCA levels for these two patients are displayed as dashed lines. Visit refers to serial 4-weekly blood draws. (B) Correlation between TF Ag and MP PCA assays (linear correlation coefficient 0.89, P < 0.0001).
This analysis suggests a role for TF in the pathogenesis of VTE in pancreatic cancer. Furthermore, these data suggest that a rise in plasma TF measured either by TF Ag or MP PCA during the course of chemotherapy may be predictive of subsequent symptomatic VTE events in patients with pancreatic cancer. The source of circulating TF is an area of controversy. Studies have identified TF-containing microparticles that also possess endothelial cell-, platelet- and macrophage-derived surface antigens [8–10]. In patients with cancer, tumor cells may be a potential source of TF as well. The findings reported here are in agreement with previous reports, including ours, suggesting an association between TF expression by tumor cells and subsequent VTE in pancreatic and ovarian cancer [5,6]. Although our findings are of statistical significance, given the small sample size and small number of events, we consider this to be a preliminary report requiring confirmation. This study was also inadequately powered to evaluate the impact of thromboprophylaxis and response to chemotherapy. Our findings suggest, however, that future prospective studies should investigate the potential role of TF as a biomarker for VTE in pancreatic and other cancers known to have high levels of TF expression by tumor cells. Both TF Ag and MP PCA assays deserve further investigation in this regard.
Acknowledgments
A. A. Khorana is supported by a grant from the National Cancer Institute K23 CA120587. Funding for this study was provided by Pfizer, Inc and the University of North Carolina Cancer Research Fund. The authors would like to acknowledge B. H. Smith for technical expertise.
Footnotes
Disclosure of Conflict of Interests
The authors state that they have no conflict of interest.
References
Full text links
Read article at publisher's site: https://doi.org/10.1111/j.1538-7836.2008.03156.x
Read article for free, from open access legal sources, via Unpaywall:
https://onlinelibrary.wiley.com/doi/pdfdirect/10.1111/j.1538-7836.2008.03156.x
Citations & impact
Impact metrics
Citations of article over time
Article citations
Cancer progression and tumor hypercoagulability: a platelet perspective.
J Thromb Thrombolysis, 57(6):959-972, 17 May 2024
Cited by: 0 articles | PMID: 38760535
Review
The pathogenesis of cancer-associated thrombosis.
Int J Hematol, 119(5):495-504, 29 Feb 2024
Cited by: 5 articles | PMID: 38421488
Review
Mechanism of Hirudin-Mediated Inhibition of Proliferation in Ovarian Cancer Cells.
Mol Biotechnol, 66(5):1062-1070, 07 Jan 2024
Cited by: 1 article | PMID: 38184808
Cancer-Associated Thrombosis: Epidemiology, Pathophysiological Mechanisms, Treatment, and Risk Assessment.
Clin Med Insights Oncol, 17:11795549231220297, 26 Dec 2023
Cited by: 2 articles | PMID: 38152726 | PMCID: PMC10752082
Review Free full text in Europe PMC
Incidence of venous thromboembolism and predictive ability of age-adjusted international prognostic index for prediction of venous thromboembolism in Asian patients with diffuse large B-cell lymphoma.
J Thromb Thrombolysis, 57(3):473-482, 13 Dec 2023
Cited by: 0 articles | PMID: 38091158
Go to all (182) article citations
Similar Articles
To arrive at the top five similar articles we use a word-weighted algorithm to compare words from the Title and Abstract of each citation.
Tissue factor and its procoagulant activity on cancer-associated thromboembolism in pancreatic cancer.
Cancer Sci, 112(11):4679-4691, 30 Aug 2021
Cited by: 19 articles | PMID: 34382298 | PMCID: PMC8586686
Venous thromboembolism in patients with pancreatic cancer: implications of circulating tissue factor.
Blood Coagul Fibrinolysis, 22(4):295-300, 01 Jun 2011
Cited by: 13 articles | PMID: 21372691
Increased microparticle tissue factor activity in cancer patients with Venous Thromboembolism.
Thromb Res, 125(6):511-512, 24 Oct 2009
Cited by: 129 articles | PMID: 19854471 | PMCID: PMC2878897
Predictive value of tissue factor bearing microparticles in cancer associated thrombosis.
Thromb Res, 125 Suppl 2:S89-91, 01 Apr 2010
Cited by: 37 articles | PMID: 20434015
Review
Funding
Funders who supported this work.
NCI NIH HHS (2)
Grant ID: K23 CA120587-03
Grant ID: K23 CA120587
NHLBI NIH HHS (4)
Grant ID: R01 HL084087
Grant ID: R01 HL095096
Grant ID: R01 HL084087-02
Grant ID: R01 HL095096-01