Abstract
Free full text

Lipotoxicity in obese pregnancy and its potential role in adverse pregnancy outcome and obesity in the offspring
Abstract
Increasing maternal obesity is a challenge that has an impact on all aspects of female reproduction. Lean and obese pregnant women gain similar fat mass, but lean women store fat in the lower-body compartment and obese women in central compartments. In the non-pregnant, central storage of fat is associated with adipocyte hypertrophy and represents a failure to adequately store excess fatty acids, resulting in metabolic dysregulation and ectopic fat accumulation (lipotoxicity). Obese pregnancy is associated with exaggerated metabolic adaptation, endothelial dysfunction and increased risk of adverse pregnancy outcome. We hypothesize that the preferential storage of fat in central rather than ‘safer’ lower-body depots in obese pregnancy leads to lipotoxicity. The combination of excess fatty acids and oxidative stress leads to the production of oxidized lipids, which can be cytotoxic and influence gene expression by acting as ligands for nuclear receptors. Lipid excess and oxidative stress provoke endothelial dysfunction. Oxidized lipids can inhibit trophoblast invasion and influence placental development, lipid metabolism and transport and can also affect fetal developmental pathways. As lipotoxicity has the capability of influencing both maternal endothelial function and placental function, it may link maternal obesity and placentally related adverse pregnancy outcomes such as miscarriage and pre-eclampsia. The combination of excess/altered lipid nutrient supply, suboptimal in utero metabolic environment and alterations in placental gene expression, inflammation and metabolism may also induce obesity in the offspring.
HYPOTHESIS
The 70–100% increase in maternal obesity over the last decade has had ramifications for all aspects of female reproduction, with maternal adiposity strongly associated with an increased risk of essentially all maternal and fetal complications [1]. Our hypothesis is that, in lean pregnant women, fatty acids required for fetal growth are efficiently stored and mobilized from lower-body fat depots. In obese pregnant women, lower-body fat depots are replete and/or there is a preference to store fat centrally with the potential of fatty acid spillover and lipotoxicity. Lipotoxicity leads to maternal endothelial dysfunction, decreases trophoblast invasion and influences placental metabolism and function. This is the pathological link between maternal obesity and adverse ‘metabolic’ pregnancy outcomes such as GDM (gestational diabetes mellitus), pregnancy-induced hypertension and pre-eclampsia, and may have consequences for the programming of obesity in the offspring.
BODY FAT DISTRIBUTION, METABOLISM AND LIPOTOXICITY IN THE NON-PREGNANT
Human body fat compartments can be classified pragmatically as lower-body subcutaneous fat, upper-body subcutaneous fat and intra-abdominal/visceral fat [2]. The roles of these different depots in producing and storing fatty acids has been reviewed recently [2]. The accepted view is that visceral fat is associated with an abnormal metabolic and adipokine profile. However, upper-body subcutaneous and visceral fat stores are often not differentiated and the relative contributions of each depot to metabolic dysregulation is unclear [2]. Upper-body subcutaneous fat is relatively resistant to insulin suppression of lipolysis and is estimated to be the source of approx. 60% of circulating NEFAs (non-esterified fatty acids). Conversely, lower-body fat is much more sensitive to insulin suppression of lipolysis, contributing only 15–20% of circulating NEFAs. Consistent with these site-specific functional differences, lower-body fat (assessed by computed tomography) is independently associated with a reduced risk of lipid and carbohydrate metabolic dysregulation [3], and larger depots of lower-body adipose tissue are associated with more efficient storage of dietary fat [2]. Large population studies in women over 40 years of age have demonstrated that central adiposity (i.e. waist circumference) increases with age and, independently of age, with parity [4].
Obesity is associated with upper-body adipose cell enlargement in adults (hypertrophic obesity) and adipose cell size is correlated with whole-body insulin resistance. Hypertrophic obesity results in reduced uptake and storage of fatty acids along with increases in lipolysis, inflammatory cell infiltration and adipokine secretion [2]. Furthermore, the number of pre-adipocytes in abdominal subcutaneous adipose tissue capable of undergoing differentiation to adipose cells is reduced in hypertrophic obesity [5]. Thus this large capacity ‘sink’ for fatty acids is effectively replete and there is NEFA spillover into the circulation, promoting ectopic fat accumulation in extra-adipose tissues such as liver, muscle and heart [5]. This phenomenon of NEFA spillover is termed lipotoxicity. When the intracellular accumulation of fatty acids in these ectopic sites exceeds the ability of the cells to utilize the fatty acids then cellular dysfunction occurs. Lipotoxic consequences involve not only fatty acids, but also metabolites of cholesterol. In the liver, for example, the abnormal or excessive oxidation of fatty acids leads to ROS (reactive oxygen species) production, disturbances in cellular membrane fatty acids and phospholipid composition, alterations in cholesterol content and changes in ceramide signalling [6].
OXIDATIVE STRESS AND HIGH LIPID CONCENTRATIONS LEAD TO ENDOTHELIAL DYSFUNCTION
Obesity is associated with oxidative stress and high levels of ROS [7]. Oxidative stress may arise from intracellular accumulation of triacylglycerols (triglycerides) having an impact on mitochondrial efficiency, resulting in accumulation of electrons within the electron transport chain which react with oxygen to form superoxide radicals. The combination of high lipid levels and oxidative stress leads to the production of three types of oxidized lipid product with harmful effects: lipid peroxides, oxidized lipoproteins and oxysterols. Elevated plasma NEFA levels can induce nitroxide radical formation in smooth muscle and endothelial cells, induce respiratory burst in white cells and serve as substrates for oxidation themselves, leading to the propagation of lipid peroxides. High plasma triacylglycerol concentrations lead to formation of small-dense LDL (low-density lipoprotein), a highly atherogenic lipoprotein particle readily susceptible to oxidation forming OxLDL (oxidized LDL). In addition to oxidized fatty acids, OxLDL contains oxysterols. Direct attack on cholesterol by ROS can lead to formation of the relatively stable 7α- and 7β-hydroxycholesterol, 7-ketocholesterol and 5,6-epoxycholesterol [8,9]. High intracellular levels of cholesterol can lead to tissue oxysterol production [e.g. 22(R)-hydroxycholesterol and 24(S)-hydroxycholesterol] via specific enzymes in liver, brain and macrophages, and these oxysterols are detectable in plasma [8]. Plasma oxysterol concentrations are raised in the metabolic syndrome, diabetes and hypercholesterolaemia [10]. In adolescent females, oxysterols correlate with BMI (body mass index), waist circumference and fasting insulin, and were suggested to be early markers of oxidative-stress-mediated metabolic dysregulation [11]. Intracellular oxysterols may have both beneficial and detrimental effects [8]. They can decrease cholesterol biosynthesis via interaction with SREBP-1c (sterol-regulatory-element-binding protein-1c) and are also potent transactivators of the nuclear receptors LXR (liver X receptor) α and β [12]. LXRα is involved in the regulation of steroid hormone biosynthesis, bile acid synthesis, conversion of lanosterol into cholesterol, cellular cholesterol efflux and lipid-mobilizing proteins such as CETP (cholesteryl ester transfer protein) [8]. Oxysterols, however, can be toxic to cells inducing inflammation, oxidative stress and apoptosis [8,12]. Thus there is ample evidence to link oxidative lipid products to metabolic dysregulation.
There is evidence that triacylglycerol-rich lipoproteins play a role in endothelial dysfunction [13]. OxLDL induces PAI-1 (plasminogen-activator inhibitor-1) expression in endothelial cells [14]. Long-term NEFA elevation results in reduced insulin-mediated vasodilation [2]. Oxysterols can induce expression of inflammatory markers in endothelial cells via LXR-independent mechanisms [15] and may be damaging to vascular endothelium via both oxidative and inflammatory means [9,16]. Lipid effects on vascular endothelium may be direct or via ROS generation. NEFAs can directly inhibit NO bioavailability [17], and activate NADPH oxidase and the mitochondrial electron transport chain to generate superoxide [18]. Effects of OxLDL on endothelial cells may also be via NADPH oxidase ROS production [14]. There is substantial evidence supporting the presence of endothelial dysfunction in obesity [17], including increased NADPH oxidase expression in vascular endothelial cells, and evidence of endothelial oxidative stress [19]. There is a specific relationship with upper-body obesity, with higher waist/hip ratio an important independent positive predictor of flow-mediated dilatation [20] and arterial stiffness [21]. Lower-body fat has no association (or a weak inverse association) with arterial stiffness [21]. These results indicate that lower-body fat may be protective against vascular dysfunction.
CHANGES IN FAT MASS AND DISTRIBUTION DURING PREGNANCY
In pregnancy, total body fat increases from the first trimester to parturition with a peak in the second trimester [22], and there is an increase in the intra-abdominal visceral fat compartment [23]. Obese women show similar or smaller increases in total weight, fat mass, percentage fat and total body fat than lean women [24–27]. Studies of skinfold thicknesses [26,27] show that obese women put on more fat in the upper-body compartment (suprailiac and subscapular skinfold), but that lean women put on more fat in the lower-body compartment (mid-thigh skinfold). Non-pregnant women with lower-body obesity are more efficient at storing systemic NEFAs in lower-body adipose depots than women with upper-body obesity [28], and this could be interpreted as ‘pear-shaped’ women being efficient at storing fat in lower-body depots that provide protection against metabolic risk and endothelial dysfunction.
METABOLIC DYSREGULATION, OXIDATIVE STRESS AND ENDOTHELIAL DYSFUNCTION IN OBESE PREGNANCY
Healthy pregnancy is associated with lipid mobilization (increases in plasma cholesterol and triacylglycerols), changes in insulin resistance (a gestational increase in insulin resistance) and improvement in endothelial function [29]. In maternal obesity, there is an exaggerated lipid response [30], a greater degree of insulin resistance [30] and less improvement in endothelium-dependent microvascular function [31]. The relative endothelial dysfunction in obese pregnancy is in part explained by altered levels of plasma cytokines [IL (interleukin)-6 and IL-10] with obesity [31]. Lipid peroxides are increased in healthy pregnancy [32], but few findings are available on oxidative stress in obese pregnancy. An association between maternal BMI and also maternal cholesterol levels and the oxidizability of LDL has been observed [33]. In non-pregnant Type 2 diabetics, high plasma oxysterol levels are particularly observed when plasma LDL levels are above approx. 3.6 mmol/l [10], a level that is exceeded in overweight and obese women in later pregnancy. Although the literature on oxysterols during pregnancy is extremely limited, gestational increases and further increases with maternal diabetes have been reported [34].
CONSEQUENCES OF LIPOTOXICITY IN PREGNANCY
Pregnant women with familial hypercholesterolaemia have evidence of endothelial activation [35] and increased uteroplacental vascular resistance [36]. In pregnancies complicated by GDM, women are insulin-resistant [37], have hyperlipidaemia [30], increased oxidative stress [38], increased LDL susceptibility to oxidation [33] and poorer endothelial function than control women [39]. These two ‘extreme’ metabolic conditions (GDM and familial hypercholesterolaemia), in conjunction with the results in the non-pregnant population relating high lipid levels to oxidative stress and endothelial dysfunction, suggest that lipid abnormalities arising from NEFA or cholesterol excess may be responsible for the observed endothelial dysfunction of obese pregnancy. The generation of oxysterols under lipotoxic conditions also has potential implications for placental development and function. Oxysterols are ligands for LXRα and β which are expressed by human placenta and may have an impact on lipid transport and metabolism. OxLDL inhibits trophoblast invasion potentially via oxysterol activation of LXRβ [40]. Collectively, these pathways may contribute to the observed increase in placental complications in obese pregnancy.
POTENTIAL IMPACT ON OFFSPRING
There are also immediate [1] and long-term consequences of obese pregnancy for the offspring, including potential programming of future obesity [41,42]. Oxysterols are involved in Hedgehog signalling pathways, disruption of which are associated with structural defects [43]. LXRs have been proposed to be important in placental cholesterol transport from mother to fetus [44], and synthetic LXR agonists increase fatty acid synthesis in trophoblast cell lines [45]. In the hyperlipidaemic states of Type 1 diabetes and GDM, placental expression of genes coding for the transport and activation of fatty acids are up-regulated and, in GDM placenta, there is also a selective up-regulation of genes involved in pathways for intracellular lipid biosynthesis [46]. In primary trophoblasts, insulin and NEFAs enhance the formation of fatty acid droplets [46,47]. Placentae from obese pregnancy have macrophage accumulation and inflammation in an analogous way to adipose tissue [48]. Thus the placenta is another likely site of ectopic fat accumulation in obese pregnancy but with a finite capacity, which, if exceeded, e.g. due to a maternal high-fat diet, may induce lipotoxicity in the fetus [42,49].
SUMMARY (Figure 1)
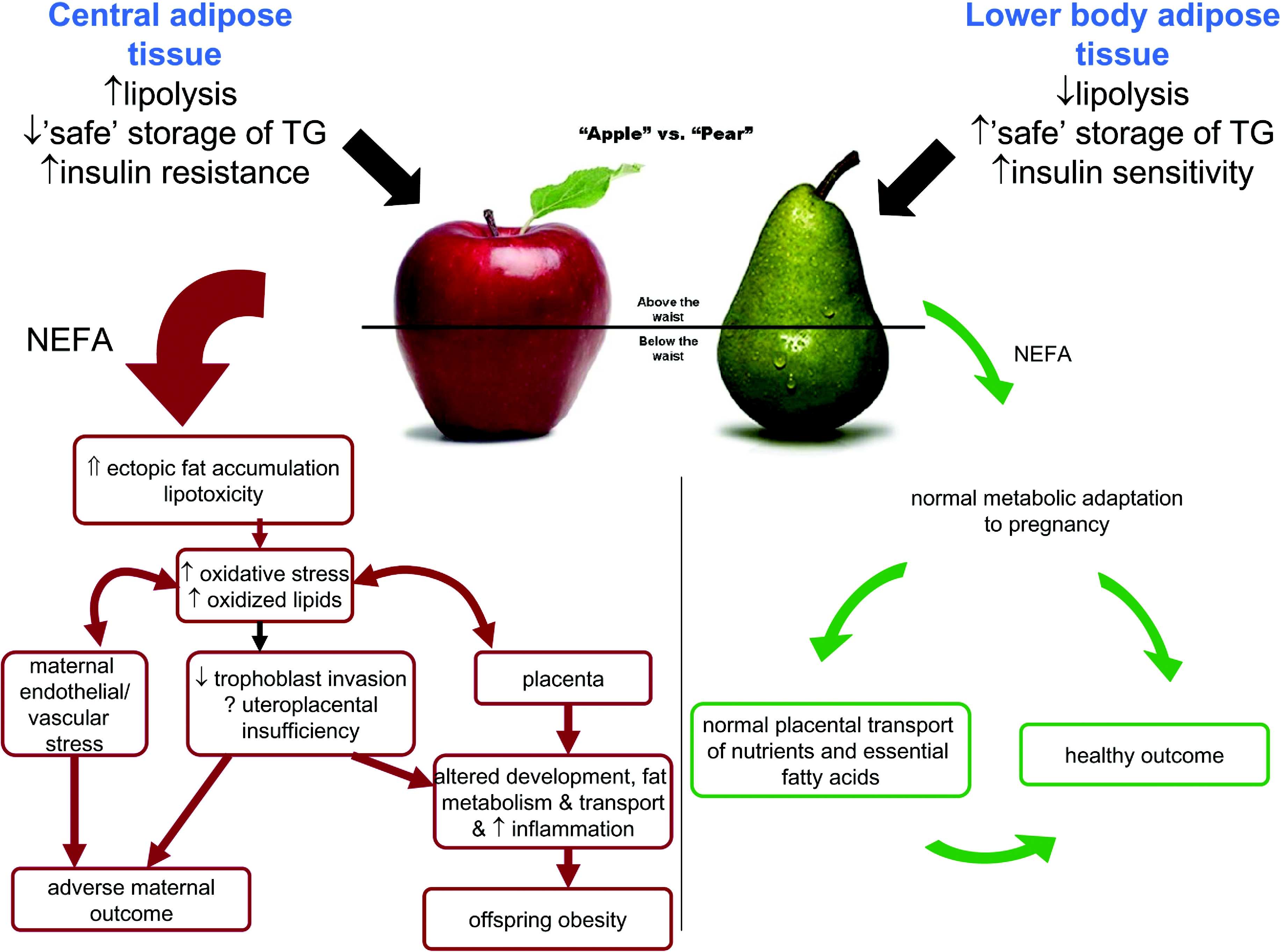
A central fat accumulation during pregnancy leads to fatty acid overspill from adipose depots and lipotoxicity. Lipotoxic effects include maternal endothelial dysfunction, decreased trophoblast invasion and altered placental metabolism. These may result in adverse pregnancy outcome (such as pre-eclampsia or miscarriage) and programming of obesity in the offspring. A lower-body fat accumulation allows ‘safe’ storage of fatty acids and a normal metabolic and physiological adaptation to pregnancy with appropriate nutrient transfer to the offspring. TG, triacylglycerol.
Lower-body adipose tissue depots are insulin-sensitive and they can efficiently store fat. Healthy pregnant women store fat accumulated during pregnancy predominately in lower-body depots, and this is associated with a benign maternal adaptation of metabolism and physiology to pregnancy and a preservation of endothelial function. In contrast, upper-body fat contains hypertrophic adipocytes and is relatively insulin-resistant and in excess (i.e. in ‘apple-shaped’ women) results in increased lipolysis of upper-body triacylglycerol stores and increased adipokine secretion. Central obesity is also associated with fatty acid excess and ectopic fat accumulation in liver, pancreas and placenta. Obese women therefore have a predisposition to lipotoxicity, metabolic dysregulation and inflammation, which may be exaggerated by the accumulation of further fat during pregnancy. Under conditions of oxidative stress, excess lipids become oxidized to form lipid peroxides, oxidized lipoproteins and oxysterols. These oxidized lipid mediators are cytotoxic and can act as ligands for nuclear receptors involved in key metabolic and developmental pathways, which may adversely affect maternal endothelial function, trophoblast invasion and placental function. Thus oxidized lipid products may be the link between maternal obesity and placentally related adverse pregnancy outcomes such as miscarriage and pre-eclampsia. The combination of excess lipid nutrient supply, suboptimal in utero metabolic environment and the incapacity of the placenta to effectively buffer excessive fatty acids may trigger adaptive pathways which ultimately result in increased percentage body fat and propensity to future obesity in the offspring of obese mothers.
TESTING THE HYPOTHESIS
As the consequences of lipotoxicity in pregnancy have both potential impact on pregnancy outcome and have far-reaching consequences for offspring health, a study of the degree, causes and impact of lipotoxicity in obese mothers is warranted.
An understanding of the functionality of visceral, upper-body subcutaneous and lower-body subcutaneous adipose tissue in pregnancy with respect to lipolysis (basal and stimulated), insulin resistance and adipokine production is required. There are few data on the measurement of oxidative stress in obese pregnancy and assessment of lipid peroxides, oxidized lipoproteins and oxysterols. In order to link lipotoxic mediators to adverse pregnancy outcome, studies which test the relationship between circulating markers of lipotoxicity and endothelial function should be undertaken. To test whether altered fat distribution underlies or contributes to lipotoxicity in pregnancy a comparison of metabolic response to pregnancy, endothelial function and placental function in women matched for BMI but with different fat distributions (‘apples’ compared with ‘pears’) would be informative. An epidemiological assessment of the impact of lower-body compared with upper-body fat distribution on risk of adverse pregnancy outcome should be undertaken. Maternal age is increasing and, at least in women over 40 years of age, is positively associated with central adiposity. Thus a higher proportion of women may be starting pregnancy with higher reserves of upper-body fat and, linked to this, lower reserves of safe fat depots. An investigation into fat distribution associated with parity and age in women of child-bearing age would highlight its potential impact on pregnancy outcomes. Interesting new findings show that a high-fat meal induces low-grade endotoxaemia and results in increased expression of markers of endothelial dysfunction potentially via TLR (Toll-like receptor) signalling pathways [50]. In view of the macrophage infiltration of adipose tissue and placenta in obese pregnancy, this new link between fatty acid handling, inflammation and endothelial dysfunction is worth pursuing. Finally, there is very little information on the impact of maternal diet on maternal metabolism in pregnancy and diets may vary greatly between the non-obese and obese. Diet has huge potential to affect the maternal response to pregnancy that may have downstream effects in the offspring such as those observed in animal studies [42,49]. More detailed studies of potential dietary determinants of maternal response to pregnancy, their interaction with the innate immune system and their consequences are required.
CLINICAL IMPACT
Up to one in five women of reproductive age are obese with significant socioeconomic costs directly attributable to the increased risk of maternal and neonatal complications [51]. Direct evidence for lipotoxicity in obese pregnancy, which may link maternal obesity and placentally related adverse pregnancy outcome, is lacking. The placenta may transmit metabolic abnormalities resulting from lipotoxicity to the offspring via in utero programming and hence there could be far-reaching consequences for offspring health. Because of the potential for harm to both mother and baby, a study of the degree, causes and impact of lipotoxicity in obese pregnancy is timely. Research in the fields of cardiovascular and diabetic medicine suggest ways of manipulating lipid metabolism using both lifestyle and pharmacological interventions that may have potential utility in obese pregnancy. Finally, if our hypotheses are proven correct, the work would give additional incentives for mothers to improve their metabolic status (i.e. reduce their waist circumferences) in advance of becoming pregnant for benefits both to themselves and their offspring.
FUNDING
E. J. is the recipient of a University of Glasgow Medical Faculty Entry Level Clinical Research Fellowship.
References
Full text links
Read article at publisher's site: https://doi.org/10.1042/cs20090640
Read article for free, from open access legal sources, via Unpaywall:
http://www.clinsci.org/content/119/3/123.full.pdf
Citations & impact
Impact metrics
Citations of article over time
Alternative metrics
Smart citations by scite.ai
Explore citation contexts and check if this article has been
supported or disputed.
https://scite.ai/reports/10.1042/cs20090640
Article citations
Association of gastrointestinal microbiome and obesity with gestational diabetes mellitus-an updated globally based review of the high-quality literatures.
Nutr Diabetes, 14(1):31, 21 May 2024
Cited by: 0 articles | PMID: 38773069 | PMCID: PMC11109140
Review Free full text in Europe PMC
Maternal obesity and placental function: impaired maternal-fetal axis.
Arch Gynecol Obstet, 309(6):2279-2288, 18 Mar 2024
Cited by: 1 article | PMID: 38494514 | PMCID: PMC11147848
Review Free full text in Europe PMC
Visceral Adipose Tissue Depth as a Novel Predictor for Gestational Diabetes Mellitus: A Comprehensive Meta-Analysis and Systematic Review.
Medicina (Kaunas), 60(4):557, 29 Mar 2024
Cited by: 0 articles | PMID: 38674203 | PMCID: PMC11052462
Review Free full text in Europe PMC
Maternal pre-pregnancy overweight or obesity and risk of birth defects in offspring: Population-based cohort study.
Acta Obstet Gynecol Scand, 103(5):862-872, 28 Jan 2024
Cited by: 1 article | PMID: 38282287 | PMCID: PMC11019515
The association between dyslipidaemia in the first trimester and adverse pregnancy outcomes in pregnant women with subclinical hypothyroidism: a cohort study.
Lipids Health Dis, 23(1):13, 11 Jan 2024
Cited by: 2 articles | PMID: 38212787 | PMCID: PMC10782788
Go to all (135) article citations
Similar Articles
To arrive at the top five similar articles we use a word-weighted algorithm to compare words from the Title and Abstract of each citation.
Maternal obesity modulates intracellular lipid turnover in the human term placenta.
Int J Obes (Lond), 41(2):317-323, 26 Oct 2016
Cited by: 44 articles | PMID: 27780978 | PMCID: PMC5309341
Maternal obesity, metabolism, and pregnancy outcomes.
Annu Rev Nutr, 26:271-291, 01 Jan 2006
Cited by: 193 articles | PMID: 16704347
Review
The Impact of Maternal Body Composition and Dietary Fat Consumption upon Placental Lipid Processing and Offspring Metabolic Health.
Nutrients, 12(10):E3031, 03 Oct 2020
Cited by: 7 articles | PMID: 33022934 | PMCID: PMC7601624
Review Free full text in Europe PMC
Maternal obesity is associated with a lipotoxic placental environment.
Placenta, 35(3):171-177, 11 Jan 2014
Cited by: 165 articles | PMID: 24484739 | PMCID: PMC3978121