Abstract
Free full text

p53-mediated hematopoietic stem and progenitor cell competition
Abstract
Cell competition was originally described in Drosophila as a process for selection of the fittest cells. It is likely to play an important role in tissue homeostasis in all metazoans, but little is known about its role and regulation in mammals. Using genetic mosaic mouse models and bone marrow chimeras, we describe here a form of cell competition that selects for the least damaged cells. This competition is controlled by p53 but is distinct from the classical p53-mediated DNA damage response: it persists for months, is specific to the hematopoietic stem and progenitor cells, and depends on the relative rather than absolute level of p53 in competing cells. The competition appears to be mediated by a non-cell autonomous induction of growth arrest and senescence-related gene expression in outcompeted cells with higher p53 activity. p53-mediated cell competition of this type could potentially contribute to the clonal expansion of incipient cancer cells.
Introduction
Despite being genetically identical, cells in multicellular organisms are unequal in many ways. Even developmentally equivalent cells within a given tissue are heterogeneous due to stochastic variation in gene expression (Bahar et al., 2006; Chang et al., 2008; Spencer et al., 2009), or unequal exposure to nutrients, oxygen, growth factors and stress. Elimination of stressed, damaged, or otherwise suboptimal cells is important for tissue function and homeostasis. It can occur through well characterized cell-autonomous mechanisms that are triggered based on the absolute degree of cell stress or damage. For example, DNA damage that exceeds a certain level induces p53-mediated apoptosis or cell cycle arrest. However, cell-autonomous mechanisms that are based on the absolute degree of damage may not be sufficient to ensure that the healthiest cells available are those that are maintained for ongoing tissue homeostasis. Thus, under certain conditions, cell fate may depend on the relative status of other cells in the same tissue, and cell extrinsic mechanisms that depend on relative cell fitness may exist to complement cell-autonomous mechanisms that are based on the absolute level of fitness (Figure 1A).
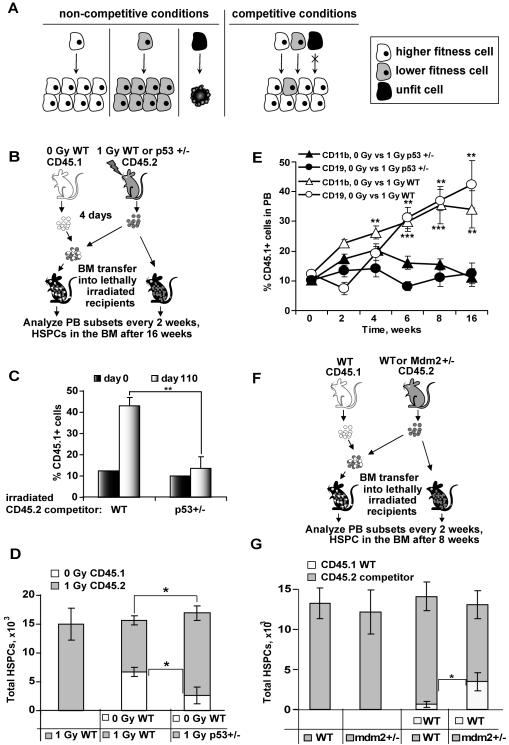
A, model of stress-induced cell competition. Cells with intermediate level of stress (grey) have growth potential in non-competitive conditions, but are underrepresented in the tissue in presence of undamaged cells (white). Cells with high level of damage (black) may undergo senescence, apoptosis, or necrosis, regardless of the presence of competitors. B, design of the experiment shown in panels C, D and E. Total BM was isolated from CD45.2 WT or p53+/− mice 4 days after exposure to 1 Gy of IR, and either used as is or mixed with BM from untreated mice carrying the CD45.1 congenic marker roughly at a ratio of (9:1). 15×106 cells were injected into lethally irradiated recipients, and the rest was stained with antibodies against CD45.1 and HSPC markers and analyzed by FACS to determine the initial contribution of HSPCs from the donors. Total HSPC numbers (C) and percent chimerism (D) were determined in the BM of the recipient mice 16 weeks later by staining for CD45.1 and HSPC markers. E, chimerism in the PB was measured every 2 weeks after transfer. Error bars represent s.e.m., n ≥ 4; * p ≤ 0.05. F, design of the experiment shown in panel G and Figure S1C-G. Untreated mdm2+/− or WT CD45.2 donor BM was used as is or mixed with BM from untreated WT CD45.1 mice, and BM transfer and analysis performed as in (B) 8 weeks after transfer. Error bars represent s.e.m., n ≥ 4; *, p≤0.05; **, p≤0.01; ***, p≤0.001. See also Figure S1.
Active elimination of suboptimal cells by cell competition was identified and subsequently well characterized in developing Drosophila wing (Adachi-Yamada and O’Connor, 2004; de la Cova et al., 2004; Morata and Ripoll, 1975; Moreno and Basler, 2004; Simpson and Morata, 1981). Cell competition has also been described in a diverse range of model organisms and cell types, such as Drosophila germline stem cells (Jin et al., 2008; Rhiner et al., 2009; Sheng et al., 2009); somatic and germline stem cells of different colonies of Botryllus (Laird et al., 2005); and pre-stalk and pre-spore cells in Dictyostelium (Santorelli et al., 2008). Cell competition is also likely to play an important role in mammalian biology (Baker and Li, 2008; Khare and Shaulsky, 2006; Moreno, 2008; Raff, 1992), and several phenomena described in mammals may potentially be the result of cell competition (Oertel et al., 2006; Oliver et al., 2004; Rajewsky, 1996; Zhao and Reed, 2001).
It is not yet known whether the examples of cell competition described so far share similar underlying mechanisms, or whether multiple types of cell competition exist. Regardless of mechanism, the defining feature of cell competition is that the cell fate is dependent on the presence, within the same tissue compartment, of developmentally equivalent cells with different levels of fitness sharing a common limiting resource (such as nutrients, growth or survival factors, or space). At the cell population level, the outcome of cell competition is a greater contribution of cells with higher fitness to overall tissue composition.
A number of tumor suppressor pathways have been implicated in cell competition in Drosophila (Tyler et al, 2007). To investigate whether tumor suppressor-based competition mechanisms exist in mammals, we have created a mosaic mouse model with different levels of p53 activity. Using this model, we characterize a form of cell competition that is induced by stress and is mediated by p53. Interestingly, the function of p53 in stress-induced competition is distinct from the classical p53-mediated acute DNA damage response. Finally, we find that p53-mediated competition in the hematopoietic system occurs primarily in the stem and progenitor cell compartment.
Results
Hematopoietic stem and progenitor cell competition is induced by DNA damage
To examine the effect of stress on the competitive status of cells in the hematopoietic system, we used bone marrow (BM) chimeras in competitive repopulation assays. Ionizing radiation (IR) was used as a sourse of stress, because of its quantitative nature. To exclude direct pro-apoptotic effects of IR on competing cells, mice were treated with a low dose of IR (1Gy) and their BM was harvested on day 4 after the treatment. At this time, no more IR-induced apoptosis is observed in the BM (Christophorou et al., 2005), and expression of DDR genes in total BM as well as in progenitor cells returns to baseline (Figure S1A, B). The BM from 1 Gy-treated mice was transferred into lethally irradiated recipient mice, either alone, or with mixed with untreated competitor BM, with 90% of BM cells being from irradiated donors and 10% from untreated donors (Figure 1B). 16 weeks later, the percentage of hematopoietic stem and progenitor cells (HSPCs; defined as lineage- c-kit+ Sca1+ CD48− CD150+ population) from the untreated donors increased approximately 4-fold relative to their initial 10% in the injected cell mixture (Figure 1C, WT, day 0 versus 110). The absolute number of HSPCs at the time of analysis was similar in chimeras with and without untreated competitors (Figure 1D, left and central bars), so any differences in the contribution of one donor must have occurred at the expense of the other. In addition, the untreated donors contributed more that the expected 10% to the lymphoid and myeloid lineages in the peripheral blood (PB), mirroring the changes observed with HSPCs (Figure 1E, open symbols). These results suggested that hematopoietic cells from untreated donors outcompeted cells from irradiated donors. This IR-induced competition presumably involved long-term repopulating stem cells, because it affected multiple lineages as well as phenotypic HSCs as late as16 weeks post-transplantation.
Relative level of damage in HSPCs is sensed by p53
We next asked whether IR-induced competition is caused by a general loss of fitness in irradiated cells due to accumulated damage, or is mediated by a dedicated sensor of stress. p53 is a central conductor of cellular stress responses (Chumakov, 2007; Levine and Oren, 2009; Vousden and Prives, 2009). We hypothesized, therefore, that p53 activity may be a measure of the stress-related competitive status of HSPCs. If this is the case, then lowering p53 activity in irradiated HSPCs should make them more resistant to being outcompeted. We tested this possibility using BM from irradiated p53 +/− mice as competitors (Figure 1B). p53 +/− rather than p53 −/− cells were used because chimeras made using p53 −/− cells showed signs of lymphoma and/or died by 7 wks after transplantation. 16 weeks after transfer, HSPCs from the untreated donor replaced a significant number of HSPCs of the irradiated (1 Gy) wild type (WT) donor, but not from irradiated p53 +/− donor mice (Figure 1C; Figure 1D, right bar). Similar changes occurred in the PB (Figure 1E, compare open versus closed symbols). This result suggested that IR-induced competition depends on the p53 level in the competing cells.
In contrast to the strong competitive advantage of irradiated donor p53 +/− cells (Figure 1C, D, E), p53-deficient cells have only modest competitive advantage in conventional BM repopulation assays when donors are not irradiated (see below; (TeKippe et al., 2003)). Thus, it is possible that p53-mediated cell competition occurs only when p53 activity reaches certain threshold level. To test this, and to distinguish p53-mediated cell competition from other effects of IR, we sought to use cells that have elevated p53 activity in the absence of external genetoxic stress. The activity of p53 is highly sensitive to the level of Mdm2 (Marine et al., 2006). Mdm2 +/− mice display enhanced p53 responses to stress, but have normal hematopoiesis (Mendrysa et al., 2006). We made BM chimeras using unirradiated WT and mdm2 +/− (Montes de Oca Luna et al., 1995) donors (Figure 1F). Mdm2 +/− BM showed no defect in reconstituting the hematopoietic system of lethally irradiated recipients when transplanted alone (Figures 1G 2nd bar, and Figure S1C). However, WT donor contribution to the HSPC pool (Figure 1G 3rd versus 4th bar, and Figure S1D) and peripheral organs (Figure S1C, E, F, G) was significantly higher than expected in the presence of mdm2 +/− competitor BM, but not in the presence of a littermate WT competitor BM. Thus mdm2 +/− HSPCs are outcompeted in vivo in the absence of external stress. This could be due to either increased background p53 activity in mdm2 +/− HSPCs, or increased p53 activation by endogenous sources of stress, or both.
Dominant-negative oncogenic mutation of p53 is insufficient for clonal expansion in vivo
To further characterize p53-mediated cell competition, we created an inducible genetic mosaic mouse model. A targeting construct driving expression of the oncogenic p53 mutant R172H (mp53) together with GFP was knocked into the Rosa26 locus, creating a mouse strain that we will refer to as R26-mp53 (Figure 2A). The R172H mutant suppresses endogenous p53 activity in a dominant-negative fashion, by forming mixed tetramers with wild type p53, and thus reducing p53 binding to the p53 responsive element in its target genes (de Vries et al., 2002; Willis et al., 2004). Transcription of the mp53 and GFP in the transgene is controlled by the ubiqitous β-actin and Rosa26 promoters, but is blocked by a transcription stop signal (Figure 2A). Using Cre with either inducible activation or variegated expression, the stop signal can be removed from a fraction of cells in every tissue, producing mosaics of otherwise equivalent cells in which GFP expression marks cells with lower p53 activity due to coexpression of the dominant-negative mp53 (for more details see Supplemental Methods and Figure S2).
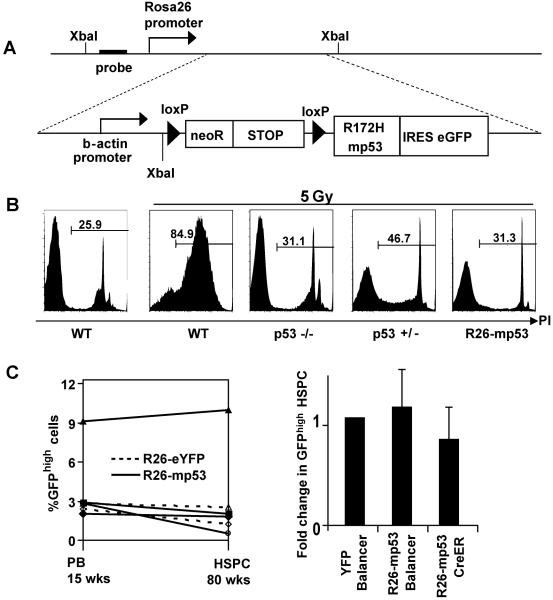
A, schematic of the inducible mp53 knock-in allele integrated into Rosa26 locus. B, Thymocyte apoptosis was assayed in mice of the indicated genotypes 20 hrs after exposure to 5 Gy by propidium iodide (PI) exclusion assay. The dye can only penetrate damaged cell membranes; percentage of PI+ (dead) cells is shown. The data is representative of two independent experiments, n =2 in each. C, mp53 cell population size does not change in steady-state conditions. Left panel, mosaicism in the PBL of the R26-mp53 CreER (solid lines) and R26-eYFP CreER (dotted lines) at 15 weeks (left Y-axis) versus HSPCs in the BM at 80 weeks (right Y-axis). Lines represent individual animals. Right panel, comparison of mosaicism levels in the PBLs at 5 weeks of age (prebleed) and in the HSPC gate at 8 to 20 weeks of age. Here and throughout the paper, fold change in GFPhigh HSPCs is calculated as follows: the percent of GFPhigh HSPCs (analysed at the end of the experiment) is divided by percent of GFPhigh PBLs in the prebleed (analysed at 5 weeks of age) of each mouse. See also Figure S2.
Correct targeting of the construct was confirmed by Southern blotting (Figure S2A). In R26-mp53 mice that had the stop cassette removed in all cells by germline recombination (R26-mp53Δ mice), mp53 was moderately overexpressed (Figure S2B), with further accumulation upon DNA damage. Thymocytes in R26-mp53Δ mice were protected from apoptosis similar to p53 −/− controls (Figure 2B), consistent with data from other mp53 mouse models (de Vries et al., 2002; Olive et al., 2004), confirming that mp53 inhibited the endogenous p53 activity in these mice in the expected manner.
Primary cells expressing oncogenic p53 mutants in culture divide faster and survive better than the WT controls (de Vries et al., 2002; Lang et al., 2004; Olive et al., 2004). To test if mp53 cells have a growth advantage in vivo, we crossed R26-mp53 mice to Cre-ER mice, in which Cre can be inducibly activated by tamoxifen injection (Badea et al., 2003). Because of the low efficiency of Cre-mediated recombination in Cre-ER mice, tamoxifen injection results in the generation of genetic mosaics where only a fraction of cells has undergone recombination event. Adminstration of a single dose of tamoxifen to R26-mp53; Cre-ER mice resulted in mosaic expression of mp53 in the blood and other tissues, which could be monitored through GFP expression (Figure S2C and data not shown). As a control, we crossed Cre-ER mice to R26-eYFP reporter mice (Srinivas et al., 2001) that do not express mp53. Also, to generate mp53 mosaicism by different means, we crossed the R26-mp53 mice to the Balancer-Cre strain, which expresses Cre in all tissues and organs in a mosaic manner during early development, due to stochastic activity of the Cre integration site (Betz et al., 1996). We monitored the relative abundance of GFPhigh cells in the PB and HSPC compartment of the resulting R26-mp53; CreER, R26-eYFP; CreER and R26-mp53; Balancer-Cre strains. The percentage of GFPhigh cells was nearly identical in PB and HSPCs in all three strains (Figure 2C), and, due to the stochastic nature of Cre activation in these strains, was different in each mouse. The level of mosaicism was similar in all hematopoietic lineages and progenitors of each individual mouse (Figure (Figure2C,2C, S2D) and remained constant up to 80 weeks (Figure 2C, left panel). Thus, in contrast to the in vitro observations (Olive et al., 2004; Willis et al., 2004), expression of mp53 under homeostatic conditions did not confer a selective advantage in vivo, suggesting that the basal level of stress in vivo does not create sufficient selective pressure (Marusyk and DeGregori, 2008) to promote expansion of mp53 cells, at least in the hematopoietic system.
DNA damage triggers p53-dependent cell competition in HSCs
We next examined the effect of DNA damage on p53-mediated cell competition in the R26-mp53 mosaic mice. The mosaicism was induced by tamoxifen injection into R26-mp53; CreER mice. Five days later, initial levels of GFPhigh cells were measured in the PB (“prebleed”), and mice were treated with a mild dose of IR (2.5 Gy) (Figure 3A, B). Following IR, the percentage of GFPhigh cells increased in myeloid and lymphoid lineages and in the HSPC compartment (Figure 3B). The expansion of GFPhigh cells appeared to involve the long-term HSC pool, because a 20-fold increase in GFPhigh cells persisted in the lymphoid and myeloid PB lineages and lin- c-kit+ Sca1+ CD48-CD150+ BM compartment for at least 6 months after a single 2.5 Gy treatment (Figure 3B). Importantly, there was no significant difference in total HSPC numbers between WT and mosaic mice 3 weeks (Figure S3A) and 6 months (Figure S3B) after IR. Thus, rather than simply expanding, the mp53 cells seemed to be replacing WT cells. This suggests that competition between mp53 and WT HSPCs occurrs within the constraints of the regulatory circuits that normally control compartment size.
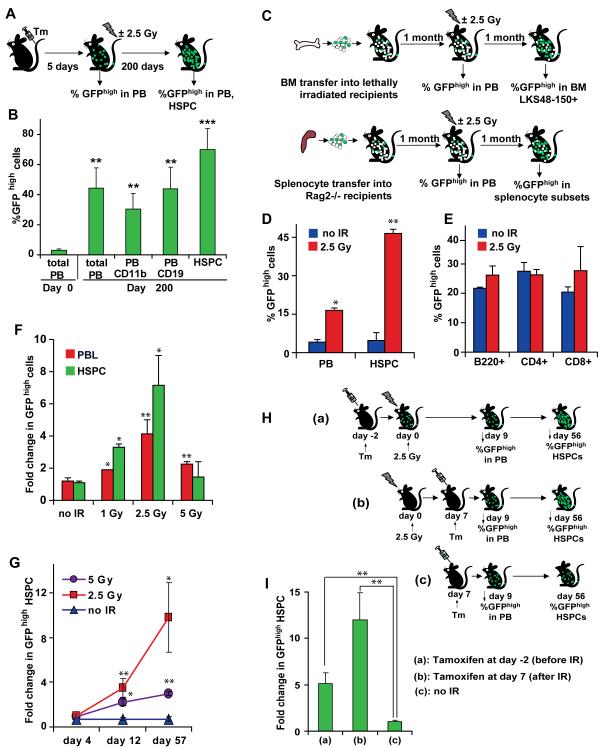
A, B, Long-term effects of p53-mediated competition. Mosaicism was induced in R26-mp53; CreER mice by tamoxifen injection. 5 days later, percentage GFPhigh cells was determined in the PB, and mice were treated with 2.5 Gy. Percentage of GFPhigh cells in PB subsets and in HSPCs in the BM was assayed 200 days later. C, D, E, Cell type specificity of p53-mediated competition. For (D), total BM from R26-mp53 Mx1Cre (please see Materials and Methods for details) mosaic mice was transferred into lethally irradiated recipients (C, upper schematic); for (E), total splenocytes from R26-mp53 Mx1Cre mosaic donors were transfered into Rag2 −/− recipients (C, lower schematic). After one month, half of the chimeras in each group was subjected to 2.5 Gy. Percentage of GFPhigh cells was measured one month after the 2.5 Gy treatment in HSPC compartment of the BM chimeras (D) or in splenocyte subsets of the lymphocyte-only chimeras (E). F, effect of the IR dose on mp53 cell expansion. Percentage of GFPhigh cells was determined one month after exposure of R26-mp53 Balancer mosaic mice to the indicated doses, and normalized to the prebleed. G, kinetics of the mp53 cell expansion. R26-mp53; CreER mosaic mice were analyzed at indicated time points after exposure to 2.5 Gy. H,I, IR-induced HSPC competition can occur after the acute DDR phase. R26-mp53; CreER mice were injected with tamoxifen either 2 days before (a) or 7 days after (b) 2.5 Gy exposure. Unirradiated control mice (c) were injected with tamoxifen at the same day as group (b). Shown is ratio of GFPhigh HSPCs (gated on lin-c-Kit+ Sca-1+ CD48-CD150+ CD34− cells) at 8 weeks after IR to the percent of GFPhigh cells in the PB at day 9 after IR. All data is representative of at least 2 independent experiments, each with n≥3. Error bars represent s.e.m. *, p≤0.05; **, p≤0.01; ***, p≤0.001. Experiments in F, G were done with both CreER and Balancer strains. See also Figure S3.
An increase in GFPhigh differentiated cell subsets could reflect either direct effects of p53 in differentiated cells, or an increased contribution of GFPhigh HSPC pool to the differentiated cell compartment. To discriminate between these possibilities, we made mice chimeric either in the entire hematopoietic system (“total” chimeras) or only within the lymphocyte compartment (Figure 3C). For “total” chimeras, we transferred BM from mosaic R26-mp53 donors into lethally irradiated recipients. To achive chimerism restricted to the lymphocyte compartment, we transferred splenocytes from mosaic R26-mp53 donors into Rag2 −/− hosts (Figure 3C). T and B cells transferred into Rag2 −/− mice, which lack their own mature lymphocytes, can stably reconstitute those populations (Sprent et al., 1991), while other hematopoietic lineages and lymphocyte precursors remain host-derived.
Upon IR, the percent of GFPhigh cells increased dramatically both in the lymphocyte and HSPC compartments of the “total”chimeras (Figure 3D), whereas the numbers of GFPhigh cells in “lymphocyte-only” chimeras did not change significantly (Figure 3E). Thus, our data suggest that IR-induced, p53-mediated cell competition in the hematopoietic system occurs predominantly in the HSPC compartment.
p53-mediated cell competition is distinct from acute p53-mediated DNA damage response
In assays that measure the immediate consequences of IR, such as growth arrest and apoptosis, lymphocytes are significantly more radiosensitive than HSCs (Anderson et al., 1977; Down et al., 1995; Fujikawa et al., 2000; Kataoka and Sado, 1975; Meijne et al., 1991; Ploemacher et al., 1992; van Bekkum, 1991) (Figure S3C). At 2.5 Gy, these responses are strictly p53-dependent (Christophorou et al., 2005; Christophorou et al., 2006). Strong competition among HSPCs but not lymphocytes (Figure 3D,E) suggested that cell competition is distinct from the acute p53-mediated DNA damage response (p53-DDR). Consistent with that, the kinetics and dose response of cell competition differed significantly from the classical p53-DDR. Thus, in contrast to the p53-DDR, which has linear dose dependence up to 5 Gy (Down et al., 1995; Ploemacher et al., 1992) (Figure S3C), expansion of mp53 cells peaked at 2.5 Gy, and was inhibited at doses of 5 Gy (Figure 3F) and higher (data not shown). In addition, the kinetics of the cell competition was inconsistent with the p53-DDR. By day 4 after IR, the time when the acute p53 response has already ended (Christophorou et al., 2005; Christophorou et al., 2006) (Figure S1A, B), there were still no significant change in the mp53:WT ratio within the HSPC compartment (Figure (Figure3G,3G, S3D). The increase in mp53 HSPCs was detectable on day 12, and continued progressively for at least 2 months (Figure 3G).
To confirm that events relevant to cell competition occur after the p53-DDR, we administered tamoxifen to induce recombination in R26-mp53; CreER mice either 2 days before, or 7 days after they had been irradiated with 2.5 Gy (Figure 3H). By 8 weeks after IR, the percent of HSPCs significantly increased in both groups (in comparison to day 9 after IR, and to untreated group) (Figure 3I). Thus, IR-induced HSPC competition appears to be mediated by long-lasting changes in p53 activity distinct from the acute DDR response.
p53-mediated cell competition depends on p53 status of the competitors
If the increase in mp53 HSPCs were caused purely by protection from apoptosis or growth arrest that are dependent on absolute, not relative, levels of p53 activity, these cells would be expected to always have the same advantage, regardless of what percentage of the mp53 cells comprise of the total HSPC pool. As a result, mp53 cell numbers would always increase to the same extent upon IR. Instead, we found that the extent of mp53 cell expansion was inversely proportional to the initial number of mp53 cells (Figure 4A,B): that is, mp53 cells expanded much more when they initially constituted only a small minority. Our interpretation is that when mp53 cells are in minority they mostly compete with WT cells rather than with each other. A similar phenomenon in population genetics, called frequency-dependent selection, is mediated by competition (Fitzpatrick et al., 2007).
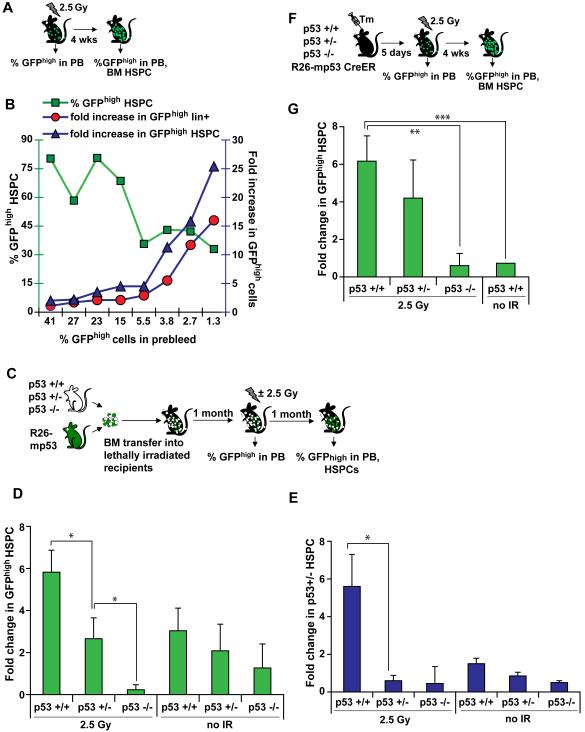
A, B, mp53 cell expansion is frequency-dependent. Percentage of GFPhigh cells was determined in R26-mp53 Balancer mice one month after 2.5 Gy exposure. Right Y-axis: percent of GFPhigh HSPCs (green squares). Left Y-axis: fold increase in GFPhigh HSPCs (blue triangles) and in GFPhigh lineage-positive cells of the BM (red circles). Each number on the X axis corresponds to percent GFPhigh cells in the prebleed of an individual mouse. C, D, relative p53 status determines the outcome of competition. p53 +/+, p53 +/− or p53 −/− BM was mixed with mosaic R26- mp53;Mx1Cre BM. The mixtures were transfered into lethally irradiated WT recipients. 1 month after the transfer, percent of chimerism was determined in the PB (prebleed), and half of the mice were treated with 2.5 Gy. Percentage of GFPhigh HSPCs was assayed 1 month after the IR treatment, and normalized to the prebleed. E, the experiment was done as in (C) except for p53 +/− CD45.1 donor BM was used instead of R26-mp53. F,G, endogenous p53 is required for the effects of mp53 on cell competition. Percentage of GFPhigh HSPCs were determined in R26-mp53 CreER mosaic mice crossed onto p53 +/+, p53 +/−, or p53 −/− background, one month after exposure to 2.5 Gy, and normalized to prebleed. All data is representative of at least 2 independent experiments, each with n≥3. Error bars represent s.e.m. *, p≤0.05; **, p≤0.01, ***, p≤0.001.
We also found that the outcome of competition depended on the relative p53 status of the competing cells. We created (mp53: p53+/+), (mp53: p53+/−), or (mp53: p53−/−) mixed BM chimeras, and after reconstitution half of the animals were irradiated with 2.5 Gy. Four weeks later, contributions of the mp53 cells to HSPC pool were compared to their pre-irradiation levels (Figure 4C, D). mp53 HSPCs expanded in the presence of p53+/+ (WT) competitors, did not change in presence of p53 +/− competitors, and contracted in presence of p53 −/− competitors (Figure 4D). This effect was only marginal in unirradiated chimeras (Figure 4D).
As an independent means to probe the role of p53 in cell competition, we performed a similar experiment but using p53+/− competitors carrying the congenic marker CD45.1 instead of R26-mp53. CD45.1+; p53 +/− HSPCs expanded in the presence of the p53 +/+ but not p53 −/− BM (Figure 4E), confirming the observations obtained with the R26-mp53 mouse model.
Selective advantage of mp53 does not require gain-of-function properties
In addition to its dominant-negative effects, the R172H mutant p53 used in this study can also contribute to tumorigenesis by gain-of-function mechanisms (Lang et al., 2004; Weisz et al., 2007). The effects of this mutant on cell competition could, therefore, be mediated either by suppressing endogenous p53, or (at least in part) by interfering with the related proteins p63 and p73 (Lang et al., 2004; Weisz et al., 2007). If a gain-of-function mechanism contributes to mp53-mediated cell competition in our mouse model, mp53-expressing cells should have a selective advantage even in the absence of the endogenous WT p53. However, when R26-mp53; CreER mice were crossed to a p53-heterozygous background, the advantage of the mp53 cells was decreased compared to the p53+/+ background; and on the p53−/− background, IR did not stimulate expansion of the mp53 HSPCs at all (Figure 4F, G). Thus, the extent of HSPC competition was proportional to the ratio of the mutant and endogenous p53.
Collectively, these data indicate that in our experimental system mp53 increases cell’s competitive status through a dominant-negative rather than a gain-of-function mechanism.
Apoptosis does not contribute to p53-mediated HSPC competition
In Drosophila, cells with higher levels of Myc induce apoptosis of the neighboring cells (de la Cova et al., 2004; Moreno and Basler, 2004). “Loser” cells, otherwise lost from the tissue, can be retained by increased dosage of the anti-apoptotic gene dIAP1 (Moreno and Basler, 2004), or decreased dosage of the pro-apoptotic gene hid (de la Cova et al., 2004). To test whether blocking apoptosis can protect “loser” HSPCs from p53-dependent stress-induced competition, we crossed R26-mp53; CreER mice to Hk-Bcl-2 transgenic mice, that overexpress Bcl2 in the entire hematopoietic system (Domen et al., 1998). Bcl-2 expression in these mice protects HSCs from the cytotoxic effects of IR (Domen et al., 1998). Nevertheless, mp53-expressing HSPCs were still able to expand in the Bcl-2 transgenic mosaic mice to the same extent as in the transgene-negative littermates (Figure 5A,B), suggesting that p53-mediated competition is not dependent on killing of outcompeted cells.
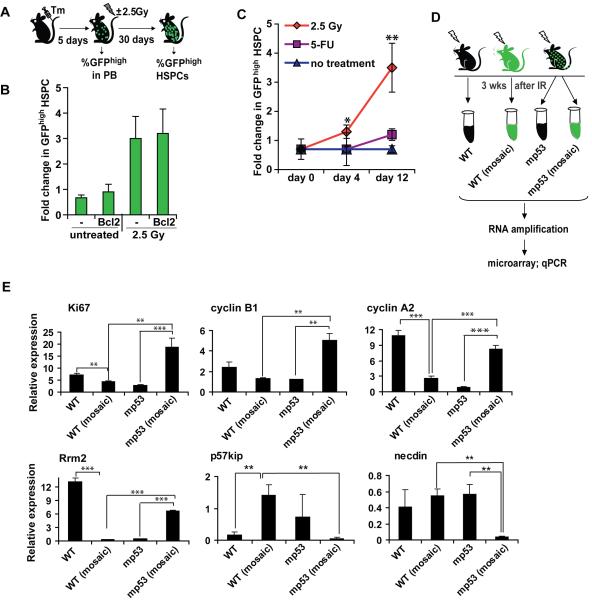
A, B, Protection from IR-induced apoptosis by Bcl2 does not inhibit p53-mediated competition. R26-mp53 CreER mice were crossed to Hk-Bcl-2 transgene (Bcl2) and compared to non-transgenic R26-mp53; CreER littermates (-). Mice were treated and analyzed as in Figure 4F. C, HSPC proliferation is insufficient to induce p53-mediated competition. Percentage of GFPhigh HSPCs were assayed in R26-mp53; CreER mosaic mice at indicated times after treatment with 2.5 Gy IR or 3 mg 5-fluorouracil (5-FU), and normalized to prebleed. D, E, HSPCs were sorted from WT, R26-mp53Δ or R26-mp53 mosaic mice 3 weeks after 2.5 Gy exposure. mRNA was amplified and analysed by microarray (not shown) and qPCR (E). All data is representative of at least 2 independent experiments, each with n≥3. Error bars represent s.e.m. *, p≤0.05; **, p≤0.01; ***, p≤0.001. See also Figure S4.
To obtain further insight into the mechanism of p53-mediated competition, we performed a microarray analysis comparing genes expressed in WT and mp53 HSPCs under competitive (mosaic mice) or non-competitive (non-mosaic mice) conditions, either untreated or 3 weeks after 2.5 Gy exposure (Figure S4A). Selected genes were further analyzed by qRT-PCR. We found that expression of apoptosis-related genes did not correlate with the cells’ competitive status (Figure S4B; and data not shown), providing additional evidence that apoptosis is unlikely to play a major role in IR-induced p53-mediated HSPC competition.
IR triggers differential proliferation of “winner” and “loser” HSPCs
IR affects HSPC proliferation in two opposite ways: first, it directly induces DNA damage-dependent transient cell cycle arrest or long-term senescence; and, second, by killing peripheral cells it indirectly promotes HSPC compensatory proliferation.
We first investigated the possibility that a massive entry of normally quiescent HSCs into the cell cycle could bring about intrinsic differences in proliferation rates between WT and mp53 HSPCs. To test this, we treated R26-mp53; CreER mosaic mice with 5-fluorouracil (5-FU) and measured changes in GFPhigh HSPC proportion. Unlike IR, which affects both PB leukocytes and HSCs, 5-FU selectively kills proliferating leukocytes and progenitor cells but has no direct toxic effects on HSCs (Hodgson and Bradley, 1984; Randall and Weissman, 1997). Instead, HSCs undergo robust proliferation in order to replenish the killed cells, which peaks at day 5 and decreases to baseline by day 12 after 5-FU treatment (Randall and Weissman, 1997). The percentage of GFPhigh HSPCs did not increase in R26-mp53;Cre-ER mosaic mice by day 12 of 5-FU treatment (Figure 5C), whereas it increased 3-fold by the same time point after IR (Figure (Figure5C,5C, ,3G).3G). Thus, even though overall recovery of hematopoiesis from 5-FU treatment is affected by p53 status (Wlodarski et al., 1998), our data indicate that stimulation of HSPC proliferation is insufficient to promote p53-mediated competition.
Consistent with this, p53-deficient cells show only a modest advantage in mixed BM chimeras in the absence of IR (Figure 4D, E) and (TeKippe et al., 2003), and mp53 cells in R26-mp53;Cre-ER mosaic mice did not expand for up to 80 weeks (Figure 3F). Repopulation of BM chimeras and long-term homeostatic maintenance of hematopoiesis in mosaic mice both require many HSC divisions, so any differences in proliferation rates should have been apparent. Together, these data suggest that, unlike in cultures of MEFs (Olive et al., 2004) and cell lines (Willis et al., 2004), expression of mp53 does not alter proliferation of HSPCs in vivo in the absence of DNA damage.
However, we observed a proliferation-related “signature” when comparing the gene expression profiles of WT and mp53 HSPCs after IR using microarrays (data not shown) followed by qPCR (Figure 5D, E). Several positive markers of cell proliferation, such as Ki67, cyclin B1, cyclin A2, and ribonucleotide reductase subunit Rrm2, were all expressed at higher levels in mp53 than in WT cells sorted by flow cytometry from irradiated mosaic mice (Figure 5E, compare columns 2 versus 4). Genes encoding negative regulators of HSC proliferation, p57kip and necdin, displayed the opposite pattern of expression (Figure 5E). Importantly, these changes in gene expression were dependent on the presence of competitor cells: expression of these genes differed between cells of the same genotype but isolated from mosaic versus non-mosaic irradiated mice (Figure 5E, compare columns 1 versus 2 and 3 versus 4). These data suggest that one of the mechanisms underlying p53-mediated HSPC competition is a non-cell-autonomous effect of p53 on cell proliferation.
Senescence-like phenotype in outcompeted HSPCs
The slow kinetics of p53-mediated competition (Figure 3G) suggested that a single dose of IR induced long-term changes in HSPCs that affected their competitive status. Competitor-dependent changes in the expression of cell cycle-related genes observed 3 weeks after IR (Figure 5E) provided further evidence that DNA damage had a prolonged, p53-dependent effect on HSPC proliferation reminiscent of senescence. p53 has been implicated in stress-induced senescence (Itahana et al., 2001), and IR selectively induces senescence in HSCs (Wang et al., 2006). We therefore analysed expression of senescence markers in competing HSPCs.
p16INK4a is a marker and mediator of HSC senescence (Janzen et al., 2006; Sharpless and DePinho, 2007). Based on qRT-PCR analysis, expression of p16 was changed during IR-induced competition in a way that reflected differences in p53 activity (Figure 6A,B). Furthermore, expression of Ezh2 (Tang et al., 2004), a Polycomb group gene implicated in senescence (Bracken et al., 2007; Kamminga et al., 2006), control of cdkn2a locus (Bracken et al., 2007) and HSC exhaustion (Kamminga et al., 2006), was increased in “winner” and decreased in “loser” HSPCs. In addition, P-selectin and Sdpr, which are upregulated in aged HSCs (Chambers et al., 2007; Rossi et al., 2005), were also upregulated in “loser” and downregulated in “winner” HSPC cells (Figure 6B). IRF8 is downregulated both in human and mouse HSCs with age (Stirewalt et al., 2009); it was upregulated in “winner” and downregulated in “loser” HSPCs (Figure 6B).
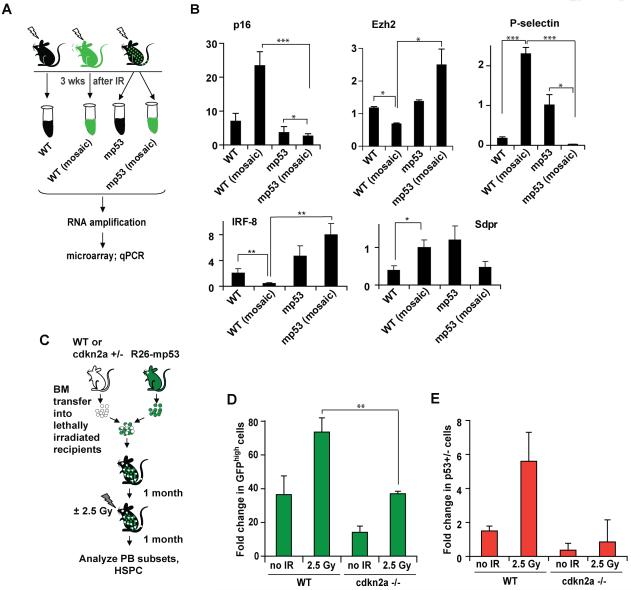
A, B, expression of senescence marker genes was measured by qPCR as in Figure 5D. C, D, E, WT or cdkn2a−/− BM was mixed with either p53 +/− BM (D) or R26-mp53 mosaic BM (E) and transfered into lethally irradiated WT recipients. Mice were treated and analyzed as in Figure 4C, D, E. All data is representative of 2 independent experiments, each with n≥3. Error bars represent s.e.m. *, p≤0.05; **, p≤0.01; ***, p≤0.001. See also Figure S5.
Importantly, similar to proliferation-related genes discussed above, these changes occured in cells of the same genotype in a way that dependend on the presence of the competitor cells (Figure 6B, compare column 1 versus 2; 3 versus 4).
Senescence of HSCs is, at least in part, dependent on p16 (Janzen et al., 2006). We therefore used cdkn2a knockout mice (lacking both p16INK4a and p19Arf) as a “senescent-deficient” mouse model, to test if IR-induced HSPC competition depends on p16-mediated senescence. To this end, we made BM chimeras using R26-mp53 cells mixed with either cdkn2a+/+ or cdkn2a −/− cells (Figure 6C), and compared the ability of mp53 HSPCs to expand after IR. p16 −/− HSCs from young mice (Janzen et al., 2006) as well as cdkn2a−/− mice (Figure S5A) do not have an advantage in competitive reconstitution assays. Nonetheless, in the presence of cdkn2a−/− competitors, the contribution of mp53 donor cells to the HSPC pool was decreased, both with and without IR (Figure 6D). These changes did not affect the frequency or total HSPC number in the BM (Figure S5B and data not shown). A similar experiment using cdkn2a −/− and p53+/− mixed bone marrow chimeras produced analogous results (Figure 6E). Collectively, these data suggest that p53-mediated IR-induced competition proceeds through induction of senescence-like state in the outcompeted HSPCs.
Genes involved in cell interactions with the environment are differentially expressed in competing HSPCs
A large group of genes differentially expressed in competitive versus non-competitive conditions encoded proteins that mediate cell interactions with the environment. This group included proteins involved in HSC adhesion and migration (P-selectin, Robo4, L-selectin) (Figures (Figures6B,6B, ,7A)7A) and in modulation of receptor signaling (CD44, TGFbRIII (betaglycan) and SOCS2) (Figure 7A).
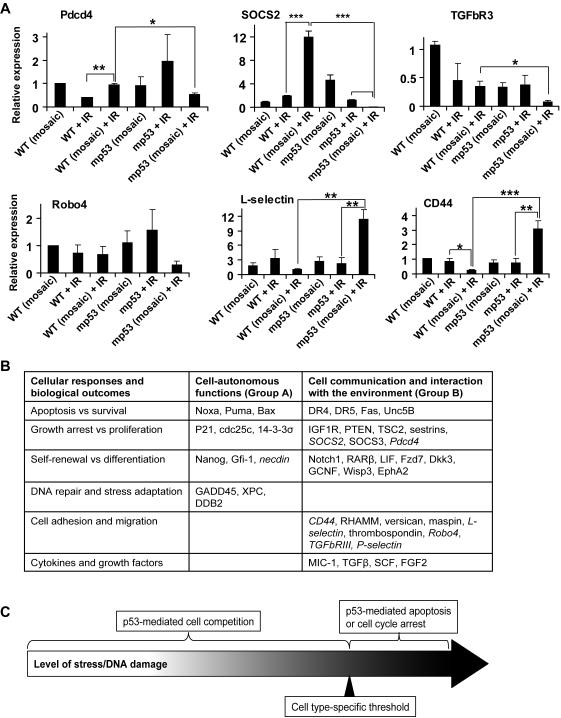
A, Adhesion and signal transduction-related genes differentially expressed in HSPCs under competitive versus non-competitive conditions were assayed as described in Figure 5D, with the addition of WT and mp53 HSPC sorted from un-irradiated mosaic R26-mp53 mice. All data is representative of 2 independent experiments, each with n≥3. Error bars represent s.e.m. *, p≤0.05; **, p≤0.01; ***, p≤0.001. B, p53 regulates expression of two classes of genes: Group A genes are targets of the canonical p53-DDR, which include DNA repair, cell cycle arrest and cell intrinsic apoptosis. This group of genes affects cell fates such as proliferation versus cell cycle arrest, survival versus apoptosis, based on absolute levels of p53 activity and regardless of the presence of competitor cells. Group B genes are involved in cell communication with the environment. Arrows indicate up- or down-regulation by p53; genes that were expressed in HSPCs in p53- and competition-dependent manner (in this study) are italicized. C, p53 can have two types of activities: when the level of damage or stress reaches a certain threshold, the cell can undergo p53 dependent apoptosis or cell cycle arrest regardless of the status of other cells in the population. This effect is mediated by p53 target genes from the group A. If the level of damage or stress is below the threshold, the cells with lower level of p53 activity would outcompete cells with higher level of p53 activity. These competitive interactions are likely mediated by p53 target genes from group B. See also Figure S6.
Mobilization of HSCs to PB and spleen is induced by variety of treatments, including cytotoxic drugs. It is possible that IR induced mp53 HSC expansion by increasing their “affinity” for some of the several currently recognized sites of HSC residence (Papayannopoulou and Scadden, 2008). To investigate if the expansion occured preferentially at one site, we followed changes in GFPhigh HSPCs in BM and spleen after IR. Although with different kinetics, mp53 HSPCs increased at both sites at 4 and 21 days after IR (Figure S6). Thus, although differential homing may contribute to competitive advantage of p53 HSPCs (for example, the availability of mitogens or self-renewal factors may vary across different niches), relocation to the spleen cannot account for overall mp53 cell expansion.
Discussion
Cell competition has been implicated in tissue development and homeostasis in several model organisms (Johnston, 2009). Here, we describe a form of cell competition in the mammalian hematopoietic system that appears to be based on the different levels of stress accrued in individual cells within a population. This stress-induced competition is mediated by p53, and at least in the hematopoietic system, it is restricted to the HSPC compartment where outcompeted cells appear to acquire a senescence-like phenotype. p53 is known to function as a sensor for multiple forms of cell stress and DNA damage and because p53 activity is a graded function, it is well suited for reporting on different relative levels of cellular stress or damage.
Although both DDR and stress-induced competition are mediated by p53, our data suggest that they are separate phenomena. Classical p53-mediated DDR is a cell-autonomous process that is triggered based on the absolute level of DNA damage. In contrast, p53-mediated cell competition is based on the relative level of cell stress or DNA damage, and on the presence of competitor cells. In addition, the p53-DDR and stress-induced competition differ in other ways: the dose sensitivity and timing of stress-induced competition are distinct from the p53-DDR (for timing, compare Figure 3G,I to Figure S1A,B; for dose sensitivity, compare Figure 3F to Figure S3C). Furthermore, p53 function in stress-induced competition is predominant in HSPCs (Figure 3D, E), whereas p53-DDR (cell cycle arrest and apoptosis) in the hematopoietic system is much more pronounced in differentiated cells such as lymphocytes (Figure S3C); (Anderson et al., 1977; Down et al., 1995; Fujikawa et al., 2000; Kataoka and Sado, 1975; Meijne et al., 1991; Ploemacher et al., 1992). Stress-based cell competition may be particularly relevant for stem cells, which contribute to differentiated cell pool and are long-lived to experience multiple insults. Recent evidence suggests that hematopoietic stem cells are inherently heterogeneous in terms of their life span, proliferation, and bias towards particular lineage, even when identified by the most up-to-date assays (Schroeder, 2010). Our conclusion that p53-mediated competition involves stem cells is based not only on stringent surface phenotype but also on the long-term persistence (up to 6 months after IR; Figure 3B) of the “winners” within multiple lineages and phenotypic HSC pool. In addition, it is possible that competition affects less primitive populations as well.
DNA damage is a type of stress that has important implications for HSPC activity, as it is heritable, often irreversible, and plays a central role in the decline of stem cell function over time (Marusyk and DeGregori, 2008; Nijnik et al., 2007; Reese et al., 2003; Rossi et al., 2007a; Sharpless and DePinho, 2007), likely due to both accumulation of mutations (Marusyk and DeGregori, 2008) and constraints on cell proliferation (Rossi et al., 2007b).
How does p53 mediate stress-induced competition? In the best characterized case, in Drosophila wing development, cell competition proceeds by killing of the neighboring cells (de la Cova et al., 2004; Moreno and Basler, 2004). Based on this evidence, it has been suggested that induction of death in the neighboring cells is a definitive feature of cell competition (Adachi-Yamada and O’Connor, 2004; Baker and Li, 2008; Morata and Martin, 2007). However, in other systems where cell competition occurs, other outcomes may be more physiologically relevant than apoptosis, as is illustrated in recently found cases of stem cell competition in the Drosophila germline (Issigonis et al., 2009; Jin et al., 2008; Rhiner et al., 2009; Sheng et al., 2009).
Consistent with this notion, during stress-induced competition, “loser” HSPCs underwent long-lasting, senescence-like changes, rather than apoptosis. Senescence induced in vitro is characterized by permanent growth arrest and unresponsiveness to growth factors. However this may be an extreme case, whereas in vivo cells may experience different degrees of senescence characterized by long-term growth inhibition. For example, naturally aged HSCs display signs of senescence while retaining the ability to proliferate (Rossi et al., 2007a). We propose that p53-mediated stem cell-specific, senescence-like response to DNA damage operates as a “memory of past damage”: while still compatible with proliferation, it permanently marks HSPCs that have experienced DNA damage and thus promotes their gradual replacement by undamaged cells over time, if such cells are available.
HSPCs with a given level of p53 activity either “win” or “lose”, depending on p53 status of the competitor cells (Figure 4B, C, D, E). Moreover, the extent of “winning” depends on the ratio of the competitors to one another (Figure 4B), which does not affect the p53-DDR. This function of p53 is non-cell autonomous in a sense that it depends on the relative p53 activity in competing cells. It is likely to be based on p53-induced changes in expression of genes that control interactions of the cell with its environment (Figure 7B, “Group B” genes). Indeed, p53 regulates expression of a number of growth factors, cytokines, and genes involved in inflammation, cell adhesion, migration and angiogenesis (Levine et al., 2006; Menendez et al., 2009; Vousden and Prives, 2009), which can impact the outcome of p53 activation. For example, regulation of expression of PAI-1 by p53 is essential for p53-mediated senescence (Kortlever et al., 2006). More generally, non-autonomous role of p53 has been demonstrated in tumor-stroma interactions (Bar et al.). However in most cases, the role of p53-mediated control of “Group B” genes in normal physiology is not well understood. We found that many genes in this category are expressed in a p53- and competitor-dependent manner in HSPCs (Figure 7A), implicating them in competitive interactions during stress response. Interestingly, these genes were expressed much later than the classical p53-DDR targets. Their regulation by p53 thus is likely to have distinct characteristics in terms of the dose and type of stress, cell-type specificity and kinetics, compared to the classical p53-DDR targets. Since many p53-DDR target genes encode basal components of apoptosis or cell cycle arrest, p53-DDR is more relevant immediately upon exposure to high levels of stress, as it will dominate over other events (Figure 7C). If the level of stress is insufficient to trigger irreversible cell cycle arrest or apoptosis, however, graded activities of the “Group B” p53-regulated genes (Figure 7B) may influence cell fate through competitive interactions with other cells.
While selective expansion of HSCs with relatively lower p53 activity is beneficial for optimal tissue composition and therefore fitness, cells with p53 mutations can gain selective advantage using the same mechanism. Since p53-mediated competition occurs in long-lived HSCs, it may play an important role in the early stages of cancer development. Furthermore, as DNA damage levels increase with age (Sharpless and DePinho, 2007), particularly in HSCs (Rossi et al., 2007a), p53-mediated competition might also contribute to a sharp increase in human cancer incidence with age (Armitage and Doll, 1954). If so, minimizing exposure to conditions that create selective pressure, increasing competitive status of normal cells, or otherwise blocking unwanted cancer cell competition, might provide new possibilities for tumor suppression.
Multiple steps of acquisition of mutations in tumor suppressors and oncogenes, each followed by clonal expansion, is a widely accepted model of tumorigenesis, which is thought to explain how rare and independent mutations can accumulate in a single clone (Rangarajan et al., 2004). A physiological counterpart of clonal expansion, if there is one, has not been characterized. It is possible that both physiological and pathological clonal expansion is mediated by cell competition. Thus, understanding mechanisms of cell competition in mammals may provide new insights into cancer initiation.
Note added in proof
While this Article was in press, a related paper (Marusyk et al. 2010) was published. The authors report irradiation induced long-term expansion of p53-deficient cells in BM chimeras, which can be blocked by addition of unirradiated WT competitors. The selection within HSPC compartment occurs in the absence of direct and immediate cytotoxicity.
EXPERIMENTAL PROCEDURES
Mouse colony
Animals were maintained at the Yale Animal Resources Center. All animal experiments were done with approval by the Institutional Animal Care and Use Committee of Yale University. Rosa26-CreER, Balancer1, Mx1Cre, p53 −/−, and CD45.1 were from Jackson Laboratory; Rosa26-eYFP mice were a kind gift of Dr. Frank Costantini; Hk-Bcl2 mice were a kind gift of Dr. Irving Weissman; Mdm2+/− mice were a kind gift of Dr. Jean-Christoph Marine. p53 −/− mice were crossed to CD45.1 strain for BM transfer experiments. All mice used for BM transfers were on C57BL/6 background.
Generation of R26-mp53 mice
Mouse R172H p53 cDNA was cloned into Rosa26 CAGGs vector (Sasaki et al., 2006). Please see Supplementary methods for details.
Mouse treatments
Mice were irradiated using an X-Rad 320 at 3 Gy/min, or a at 137Cs sourse at 1.8 Gy/min. Mosaicism was induced in R26-mp53 Rosa26-CreER mice at 4 weeks of age by a single intraperitoneal injection of 2 mg tamoxifen in peanut oil. For BM transfers involving R26-mp53, interferon-inducible Mx1Cre mice (Kuhn et al., 1995) crossed to R26-mp53 were used. Depending on the desired degree of mosaicism, mice were injected 1-3 times with 250 μg poly I:C (to induce interferon response, and therefore activate Cre) at least one week before use in BM transfers.
BM transfer experiments
One day before BM transfer, C57BL/6 recipients were irradiated with 10 Gy. BM from donor mice was harvested, mixed, and 5 × 106 total cells was transferred into the irradaited recipients by retroorbital vein injection. In parallel, an aliquot of the mixed donor BM was analyzed by FACS to determine the ratio of the donors’ HSPCs. 4 weeks after the transfer, PB was stained with CD11b, CD45.1 and CD45.2 antibodies to determine the donors’ contribution before treatment (“prebleed”). At this time point, it correlated well the original ratio of donors BM, and also allowed to take into account variations of individual mice. Where appropriate, mice where irradiated with 2.5 Gy the same day. Donor contribution was determined by gating on GFPhigh cells (for experiments involving R26-mp53 donors) or CD45.1 and CD45.2 cells (for all other expeiments). For experiments on Figures Figures3,3, ,44 and and6,6, the competition outcome (“fold change”) was determined based on the prebleed ratio. For experiments on Figure 1 (since they did not involve irradiation of the chimeras, there was no prebleed), “fold change” was calculated based on the initial ratio of the donor’s HSPCs in the injected BM mix.
Quantification of cell competition
For R26-mp53 mosaic mice, the quantification was done as following. Since at steady-state the percent of the GFPhigh LKS48-150+ cells was less than 1.5-fold different from the percent of GFPhigh cells in the blood of an individual mouse over several months, we used PB mosaicism at 5 weeks of age (“prebleed”) as a predictive value of the stem cell mosaicism in untreated mice. For BM chimera experiments, “prebleed” was determined at 4 weeks after BM transfer. The outcome of cell competition (“fold change in HSPCs”) was calculated as %GFPhigh HSPCs (or %CD45.1+ cells in BM chimeras) at the time of harvest divided by the %GFPhigh in the prebleed.
Flow cytometry
For HSPC staining, total BM cells were stained with antibodies against: B220, Gr1, CD3, CD4, CD8, Ter119, CD48, Sca1, c-kit (all from eBioscience or BD Pharmingen) and CD150 (BioLegend). Cells were analyzed by LSRII flow cytometer. Unless otherwise indicated, HSPC cells were identified by gating on lineage- c-kit+ Sca-1+ CD48− CD150+ cells. At least 300 cells were analyzed per mouse. For FACS sorting, cells were stained as above, with the following modifications: after staining with the lineage- and CD48-specific antibodies, negative fraction was purified by autoMACS sorting, stained with the other antibodies, and sorted on FACS Aria, FACS Vantage or MoFlow into the lysis buffer. Data were analyzed using FACS Diva software. For thymocyte apoptosis, freshly isolated unfixed thymocytes were analyzed in PBS containing propidium iodide.
Real-time PCR
Total RNA was isolated from cells using Trizol (Invitrogen) and (in case of HSPCs) amplified using RiboAmpHS kit from Arcturus. cDNA was synthesized by SuperScriptIII (Roche) and random primers. Real-time PCR analysis was performed using an Mx3000p (Stratagene) and SYBR green mix (Quantitect from Quiagen). Relative amounts of mRNA were normalized to GADPH RNA levels within each sample.
Data analysis
All the statistical analyses were done by Student’s t-test.
Acknowledgements
We thank Dr. Klaus Rajewsky for Rosa26 targeting vector and Bruce4 ES cells; Dr. Frank Costantini for providing Rosa26-LSL-eYFP mice; Dr. Irving Weissman for providing Hk-Bcl2 mice; Dr. Jean-Christophe Marine for providing Mdm2 +/− mice; Timothy Nottoli for technical assistance in generating R26-mp53 knock-in mice; Charles Anicelli and Sophie Cronin for expert mouse work; Gouzel Tokmulina, Tom Taylor and Xiao Xiao for FACS sorting; Bohwoo Kim and Sasha Stone for technical assistance, and all Medzhitov lab members for insightful discussions, and Maya Kotas, Oscar Colegio, Arun Unni and Dan Stetson for critical reading of the manuscript. T.B. is supported by Leukemia and Lymphoma Society postdoctoral fellowship. Supported by the Howard Hughes Medical Institute and grants from NIH.
Footnotes
Publisher's Disclaimer: This is a PDF file of an unedited manuscript that has been accepted for publication. As a service to our customers we are providing this early version of the manuscript. The manuscript will undergo copyediting, typesetting, and review of the resulting proof before it is published in its final citable form. Please note that during the production process errors may be discovered which could affect the content, and all legal disclaimers that apply to the journal pertain.
Accession numbers.
Microarray data is accessible at the GEO database under accession number GSExxxxx.
References
- Anderson RE, Olson GB, Autry JR, Howarth JL, Troup GM, Bartels PH. Radiosensitivity of T and B lymphocytes. IV. Effect of whole body irradiation upon various lymphoid tissues and numbers of recirculating lymphocytes. J Immunol. 1977;118:1191–1200. [Abstract] [Google Scholar]
- Armitage P, Doll R. The age distribution of cancer and a multi-stage theory of carcinogenesis. Br J Cancer. 1954;8:1–12. [Europe PMC free article] [Abstract] [Google Scholar]
- Baker NE, Li W. Cell competition and its possible relation to cancer. Cancer Res. 2008;68:5505–5507. [Abstract] [Google Scholar]
- Bar J, Moskovits N, Oren M. Involvement of stromal p53 in tumor-stroma interactions. Semin Cell Dev Biol. 21:47–54. [Europe PMC free article] [Abstract] [Google Scholar]
- Bracken AP, Kleine-Kohlbrecher D, Dietrich N, Pasini D, Gargiulo G, Beekman C, Theilgaard-Monch K, Minucci S, Porse BT, Marine JC, et al. The Polycomb group proteins bind throughout the INK4A-ARF locus and are disassociated in senescent cells. Genes Dev. 2007;21:525–530. [Europe PMC free article] [Abstract] [Google Scholar]
- Chambers SM, Shaw CA, Gatza C, Fisk CJ, Donehower LA, Goodell MA. Aging hematopoietic stem cells decline in function and exhibit epigenetic dysregulation. PLoS Biol. 2007;5:e201. [Europe PMC free article] [Abstract] [Google Scholar]
- Christophorou MA, Martin-Zanca D, Soucek L, Lawlor ER, Brown-Swigart L, Verschuren EW, Evan GI. Temporal dissection of p53 function in vitro and in vivo. Nat Genet. 2005;37:718–726. [Abstract] [Google Scholar]
- Christophorou MA, Ringshausen I, Finch AJ, Swigart LB, Evan GI. The pathological response to DNA damage does not contribute to p53-mediated tumour suppression. Nature. 2006;443:214–217. [Abstract] [Google Scholar]
- Chumakov PM. Versatile functions of p53 protein in multicellular organisms. Biochemistry (Mosc) 2007;72:1399–1421. [Europe PMC free article] [Abstract] [Google Scholar]
- de la Cova C, Abril M, Bellosta P, Gallant P, Johnston LA. Drosophila myc regulates organ size by inducing cell competition. Cell. 2004;117:107–116. [Abstract] [Google Scholar]
- de Vries A, Flores ER, Miranda B, Hsieh HM, van Oostrom CT, Sage J, Jacks T. Targeted point mutations of p53 lead to dominant-negative inhibition of wild-type p53 function. Proc Natl Acad Sci U S A. 2002;99:2948–2953. [Europe PMC free article] [Abstract] [Google Scholar]
- Domen J, Gandy KL, Weissman IL. Systemic overexpression of BCL-2 in the hematopoietic system protects transgenic mice from the consequences of lethal irradiation. Blood. 1998;91:2272–2282. [Abstract] [Google Scholar]
- Down JD, Boudewijn A, van Os R, Thames HD, Ploemacher RE. Variations in radiation sensitivity and repair among different hematopoietic stem cell subsets following fractionated irradiation. Blood. 1995;86:122–127. [Abstract] [Google Scholar]
- Fitzpatrick MJ, Feder E, Rowe L, Sokolowski MB. Maintaining a behaviour polymorphism by frequency-dependent selection on a single gene. Nature. 2007;447:210–212. [Abstract] [Google Scholar]
- Fujikawa K, Hasegawa Y, Matsuzawa S, Fukunaga A, Itoh T, Kondo S. Dose and dose-rate effects of X rays and fission neutrons on lymphocyte apoptosis in p53(+/+) and p53(−/−) mice. J Radiat Res (Tokyo) 2000;41:113–127. [Abstract] [Google Scholar]
- Itahana K, Dimri G, Campisi J. Regulation of cellular senescence by p53. Eur J Biochem. 2001;268:2784–2791. [Abstract] [Google Scholar]
- Janzen V, Forkert R, Fleming HE, Saito Y, Waring MT, Dombkowski DM, Cheng T, DePinho RA, Sharpless NE, Scadden DT. Stem-cell ageing modified by the cyclin-dependent kinase inhibitor p16INK4a. Nature. 2006;443:421–426. [Abstract] [Google Scholar]
- Johnston LA. Competitive interactions between cells: death, growth, and geography. Science. 2009;324:1679–1682. [Europe PMC free article] [Abstract] [Google Scholar]
- Kamminga LM, Bystrykh LV, de Boer A, Houwer S, Douma J, Weersing E, Dontje B, de Haan G. The Polycomb group gene Ezh2 prevents hematopoietic stem cell exhaustion. Blood. 2006;107:2170–2179. [Europe PMC free article] [Abstract] [Google Scholar]
- Kataoka Y, Sado T. The radiosensitivity of T and B lymphocytes in mice. Immunology. 1975;29:121–130. [Abstract] [Google Scholar]
- Khare A, Shaulsky G. First among equals: competition between genetically identical cells. Nat Rev Genet. 2006;7:577–583. [Abstract] [Google Scholar]
- Kortlever RM, Higgins PJ, Bernards R. Plasminogen activator inhibitor-1 is a critical downstream target of p53 in the induction of replicative senescence. Nat Cell Biol. 2006;8:877–884. [Europe PMC free article] [Abstract] [Google Scholar]
- Kuhn R, Schwenk F, Aguet M, Rajewsky K. Inducible gene targeting in mice. Science. 1995;269:1427–1429. [Abstract] [Google Scholar]
- Laird DJ, De Tomaso AW, Weissman IL. Stem cells are units of natural selection in a colonial ascidian. Cell. 2005;123:1351–1360. [Abstract] [Google Scholar]
- Lang GA, Iwakuma T, Suh YA, Liu G, Rao VA, Parant JM, Valentin-Vega YA, Terzian T, Caldwell LC, Strong LC, et al. Gain of function of a p53 hot spot mutation in a mouse model of Li-Fraumeni syndrome. Cell. 2004;119:861–872. [Abstract] [Google Scholar]
- Levine AJ, Hu W, Feng Z. The P53 pathway: what questions remain to be explored? Cell Death Differ. 2006;13:1027–1036. [Abstract] [Google Scholar]
- Levine AJ, Oren M. The first 30 years of p53: growing ever more complex. Nat Rev Cancer. 2009;9:749–758. [Europe PMC free article] [Abstract] [Google Scholar]
- Marine JC, Francoz S, Maetens M, Wahl G, Toledo F, Lozano G. Keeping p53 in check: essential and synergistic functions of Mdm2 and Mdm4. Cell Death Differ. 2006;13:927–934. [Abstract] [Google Scholar]
- Marusyk A, DeGregori J. Declining cellular fitness with age promotes cancer initiation by selecting for adaptive oncogenic mutations. Biochim Biophys Acta. 2008;1785:1–11. [Europe PMC free article] [Abstract] [Google Scholar]
- Marusyk A, Porter CC, Zaberezhnyy V, DeGregori J. Irradiation Selects for p53-Deficient Hematopoietic Progenitors. PLoS Biol. 2010;8:e1000324. [Europe PMC free article] [Abstract] [Google Scholar]
- Meijne EI, van der Winden-van Groenewegen RJ, Ploemacher RE, Vos O, David JA, Huiskamp R. The effects of x-irradiation on hematopoietic stem cell compartments in the mouse. Exp Hematol. 1991;19:617–623. [Abstract] [Google Scholar]
- Mendrysa SM, O’Leary KA, McElwee MK, Michalowski J, Eisenman RN, Powell DA, Perry ME. Tumor suppression and normal aging in mice with constitutively high p53 activity. Genes Dev. 2006;20:16–21. [Europe PMC free article] [Abstract] [Google Scholar]
- Menendez D, Inga A, Resnick MA. The expanding universe of p53 targets. Nat Rev Cancer. 2009;9:724–737. [Abstract] [Google Scholar]
- Montes de Oca Luna R, Wagner DS, Lozano G. Rescue of early embryonic lethality in mdm2-deficient mice by deletion of p53. Nature. 1995;378:203–206. [Abstract] [Google Scholar]
- Moreno E. Is cell competition relevant to cancer? Nat Rev Cancer. 2008;8:141–147. [Abstract] [Google Scholar]
- Moreno E, Basler K. dMyc transforms cells into super-competitors. Cell. 2004;117:117–129. [Abstract] [Google Scholar]
- Nijnik A, Woodbine L, Marchetti C, Dawson S, Lambe T, Liu C, Rodrigues NP, Crockford TL, Cabuy E, Vindigni A, et al. DNA repair is limiting for haematopoietic stem cells during ageing. Nature. 2007;447:686–690. [Abstract] [Google Scholar]
- Oertel M, Menthena A, Dabeva MD, Shafritz DA. Cell competition leads to a high level of normal liver reconstitution by transplanted fetal liver stem/progenitor cells. Gastroenterology. 2006;130:507–520. quiz 590. [Abstract] [Google Scholar]
- Olive KP, Tuveson DA, Ruhe ZC, Yin B, Willis NA, Bronson RT, Crowley D, Jacks T. Mutant p53 gain of function in two mouse models of Li-Fraumeni syndrome. Cell. 2004;119:847–860. [Abstract] [Google Scholar]
- Oliver ER, Saunders TL, Tarle SA, Glaser T. Ribosomal protein L24 defect in belly spot and tail (Bst), a mouse Minute. Development. 2004;131:3907–3920. [Europe PMC free article] [Abstract] [Google Scholar]
- Papayannopoulou T, Scadden DT. Stem-cell ecology and stem cells in motion. Blood. 2008;111:3923–3930. [Europe PMC free article] [Abstract] [Google Scholar]
- Ploemacher RE, van Os R, van Beurden CA, Down JD. Murine haemopoietic stem cells with long-term engraftment and marrow repopulating ability are more resistant to gamma-radiation than are spleen colony forming cells. Int J Radiat Biol. 1992;61:489–499. [Abstract] [Google Scholar]
- Raff MC. Social controls on cell survival and cell death. Nature. 1992;356:397–400. [Abstract] [Google Scholar]
- Rajewsky K. Clonal selection and learning in the antibody system. Nature. 1996;381:751–758. [Abstract] [Google Scholar]
- Randall TD, Weissman IL. Phenotypic and functional changes induced at the clonal level in hematopoietic stem cells after 5-fluorouracil treatment. Blood. 1997;89:3596–3606. [Abstract] [Google Scholar]
- Rangarajan A, Hong SJ, Gifford A, Weinberg RA. Species- and cell type-specific requirements for cellular transformation. Cancer Cell. 2004;6:171–183. [Abstract] [Google Scholar]
- Reese JS, Liu L, Gerson SL. Repopulating defect of mismatch repair-deficient hematopoietic stem cells. Blood. 2003;102:1626–1633. [Abstract] [Google Scholar]
- Rossi DJ, Bryder D, Seita J, Nussenzweig A, Hoeijmakers J, Weissman IL. Deficiencies in DNA damage repair limit the function of haematopoietic stem cells with age. Nature. 2007a;447:725–729. [Abstract] [Google Scholar]
- Rossi DJ, Bryder D, Zahn JM, Ahlenius H, Sonu R, Wagers AJ, Weissman IL. Cell intrinsic alterations underlie hematopoietic stem cell aging. Proc Natl Acad Sci U S A. 2005;102:9194–9199. [Europe PMC free article] [Abstract] [Google Scholar]
- Rossi DJ, Seita J, Czechowicz A, Bhattacharya D, Bryder D, Weissman IL. Hematopoietic stem cell quiescence attenuates DNA damage response and permits DNA damage accumulation during aging. Cell Cycle. 2007b;6:2371–2376. [Abstract] [Google Scholar]
- Santorelli LA, Thompson CR, Villegas E, Svetz J, Dinh C, Parikh A, Sucgang R, Kuspa A, Strassmann JE, Queller DC, et al. Facultative cheater mutants reveal the genetic complexity of cooperation in social amoebae. Nature. 2008;451:1107–1110. [Abstract] [Google Scholar]
- Sasaki Y, Derudder E, Hobeika E, Pelanda R, Reth M, Rajewsky K, Schmidt-Supprian M. Canonical NF-kappaB activity, dispensable for B cell development, replaces BAFF-receptor signals and promotes B cell proliferation upon activation. Immunity. 2006;24:729–739. [Abstract] [Google Scholar]
- Schroeder T. Hematopoietic Stem Cell Heterogeneity: Subtypes, Not Unpredictable Behavior. Cell Stem Cell. 2010;6:203–207. [Abstract] [Google Scholar]
- Sharpless NE, DePinho RA. How stem cells age and why this makes us grow old. Nat Rev Mol Cell Biol. 2007;8:703–713. [Abstract] [Google Scholar]
- Sprent J, Schaefer M, Hurd M, Surh CD, Ron Y. Mature murine B and T cells transferred to SCID mice can survive indefinitely and many maintain a virgin phenotype. J Exp Med. 1991;174:717–728. [Europe PMC free article] [Abstract] [Google Scholar]
- Srinivas S, Watanabe T, Lin CS, William CM, Tanabe Y, Jessell TM, Costantini F. Cre reporter strains produced by targeted insertion of EYFP and ECFP into the ROSA26 locus. BMC Dev Biol. 2001;1:4. [Europe PMC free article] [Abstract] [Google Scholar]
- Stirewalt DL, Choi YE, Sharpless NE, Pogosova-Agadjanyan EL, Cronk MR, Yukawa M, Larson EB, Wood BL, Appelbaum FR, Radich JP, et al. Decreased IRF8 expression found in aging hematopoietic progenitor/stem cells. Leukemia. 2009;23:391–393. [Europe PMC free article] [Abstract] [Google Scholar]
- Tang X, Milyavsky M, Shats I, Erez N, Goldfinger N, Rotter V. Activated p53 suppresses the histone methyltransferase EZH2 gene. Oncogene. 2004;23:5759–5769. [Abstract] [Google Scholar]
- TeKippe M, Harrison DE, Chen J. Expansion of hematopoietic stem cell phenotype and activity in Trp53-null mice. Exp Hematol. 2003;31:521–527. [Abstract] [Google Scholar]
- Vousden KH, Prives C. Blinded by the Light: The Growing Complexity of p53. Cell. 2009;137:413–431. [Abstract] [Google Scholar]
- Wang Y, Schulte BA, LaRue AC, Ogawa M, Zhou D. Total body irradiation selectively induces murine hematopoietic stem cell senescence. Blood. 2006;107:358–366. [Europe PMC free article] [Abstract] [Google Scholar]
- Weisz L, Oren M, Rotter V. Transcription regulation by mutant p53. Oncogene. 2007;26:2202–2211. [Abstract] [Google Scholar]
- Willis A, Jung EJ, Wakefield T, Chen X. Mutant p53 exerts a dominant negative effect by preventing wild-type p53 from binding to the promoter of its target genes. Oncogene. 2004;23:2330–2338. [Abstract] [Google Scholar]
- Wlodarski P, Wasik M, Ratajczak MZ, Sevignani C, Hoser G, Kawiak J, Gewirtz AM, Calabretta B, Skorski T. Role of p53 in hematopoietic recovery after cytotoxic treatment. Blood. 1998;91:2998–3006. [Abstract] [Google Scholar]
- Zhao H, Reed RR. X inactivation of the OCNC1 channel gene reveals a role for activity-dependent competition in the olfactory system. Cell. 2001;104:651–660. [Abstract] [Google Scholar]
Full text links
Read article at publisher's site: https://doi.org/10.1016/j.stem.2010.03.002
Read article for free, from open access legal sources, via Unpaywall:
http://www.cell.com/article/S1934590910000998/pdf
Citations & impact
Impact metrics
Citations of article over time
Alternative metrics

Discover the attention surrounding your research
https://www.altmetric.com/details/102472238
Article citations
Therapy-Related Myeloid Neoplasms: Complex Interactions among Cytotoxic Therapies, Genetic Factors, and Aberrant Microenvironment.
Blood Cancer Discov, 5(6):400-416, 01 Nov 2024
Cited by: 0 articles | PMID: 39422544 | PMCID: PMC11528189
Review Free full text in Europe PMC
Distinct landscape and clinical implications of therapy-related clonal hematopoiesis.
J Clin Invest, 134(19):e180069, 01 Oct 2024
Cited by: 1 article | PMID: 39352380 | PMCID: PMC11444158
Review Free full text in Europe PMC
Mechanisms of Germline Stem Cell Competition across Species.
Life (Basel), 14(10):1251, 01 Oct 2024
Cited by: 0 articles | PMID: 39459551 | PMCID: PMC11509876
Review Free full text in Europe PMC
Bone marrow niches for hematopoietic stem cells.
Hemasphere, 8(8):e133, 31 Jul 2024
Cited by: 1 article | PMID: 39086665 | PMCID: PMC11289431
Review Free full text in Europe PMC
Decoding Clonal Hematopoiesis: Emerging Themes and Novel Mechanistic Insights.
Cancers (Basel), 16(15):2634, 24 Jul 2024
Cited by: 0 articles | PMID: 39123361 | PMCID: PMC11311828
Review Free full text in Europe PMC
Go to all (217) article citations
Data
Data behind the article
This data has been text mined from the article, or deposited into data resources.
BioStudies: supplemental material and supporting data
Similar Articles
To arrive at the top five similar articles we use a word-weighted algorithm to compare words from the Title and Abstract of each citation.
Irradiation selects for p53-deficient hematopoietic progenitors.
PLoS Biol, 8(3):e1000324, 02 Mar 2010
Cited by: 102 articles | PMID: 20208998 | PMCID: PMC2830447
A distinctive DNA damage response in human hematopoietic stem cells reveals an apoptosis-independent role for p53 in self-renewal.
Cell Stem Cell, 7(2):186-197, 08 Jul 2010
Cited by: 187 articles | PMID: 20619763
[Mechanism of hematopoietic stem/progenitor cell aging induced by radiation damage].
Xi Bao Yu Fen Zi Mian Yi Xue Za Zhi, 29(3):233-236, 01 Mar 2013
Cited by: 0 articles | PMID: 23643076
The p53 tumor suppressor protein regulates hematopoietic stem cell fate.
J Cell Physiol, 226(9):2215-2221, 01 Sep 2011
Cited by: 60 articles | PMID: 21660944 | PMCID: PMC3081536
Review Free full text in Europe PMC
Funding
Funders who supported this work.
Howard Hughes Medical Institute
NIAID NIH HHS (4)
Grant ID: R01 AI046688-05
Grant ID: R01 AI055502-05
Grant ID: R01 AI046688
Grant ID: R01 AI055502
NIDDK NIH HHS (3)
Grant ID: R01 DK071754
Grant ID: R01 DK071754-04
Grant ID: P30 DK072442