Abstract
Free full text

Plasmodium falciparum: Rosettes do not protect merozoites from invasion-inhibitory antibodies
Abstract
Rosetting is a parasite adhesion phenotype associated with severe malaria in African children. Why parasites form rosettes is unknown, although enhanced invasion or immune evasion have been suggested as possible functions. Previous work showed that rosetting does not enhance parasite invasion under standard in vitro conditions. We hypothesised that rosetting might promote invasion in the presence of host invasion-inhibitory antibodies, by allowing merozoites direct entry into the erythrocytes in the rosette and so minimising exposure to plasma antibodies. We therefore investigated whether rosetting influences invasion in the presence of invasion-inhibitory antibodies to MSP-1. We found no difference in invasion rates between isogenic rosetting and non-rosetting lines from two parasite strains, R29 and TM284, in the presence of MSP-1 antibodies (P=0.62 and P=0.63, Student's t test, TM284 and R29, respectively). These results do not support the hypothesis that rosettes protect merozoites from inhibitory antibodies during invasion. The biological function of rosetting remains unknown.
1. Introduction
The binding of infected red blood cells (iRBC) to uninfected red blood cells (RBC) is a Plasmodium falciparum adhesion phenotype known as rosetting. P. falciparum field isolates vary in the extent to which they form rosettes, and high levels of rosette formation have been strongly associated with severe malaria in African children in numerous studies (e.g., Carlson and Wahlgren, 1992; Ringwald et al., 1993; Rowe et al., 1995, 2002b). A human polymorphism associated with deficiency of the RBC rosetting receptor Complement Receptor 1 that reduces rosetting (Rowe et al., 1997), has been shown to confer protection from severe malaria in a malaria endemic region (Cockburn et al., 2004), thus providing more evidence for an association between rosetting and life-threatening malaria.
How rosetting may contribute to the development of severe malaria is not understood. Other Plasmodium species that infect humans form rosettes (Angus et al., 1996; Lowe et al., 1998; Udomsanpetch et al., 1995) but do not typically cause the same severe malaria symptoms as P. falciparum. Obstruction of the microvasculature and impairment of blood flow by rosettes has been shown in an ex vivo model (Kaul et al., 1991), and it is likely that rosettes aggravate the pathologies caused by cytoadherence of iRBC by further blocking the microvasculature (Nash et al., 1992). Cytoadherence, where iRBC bind to the endothelium lining the blood vessels (reviewed in Newbold et al., 1999), is a parasite property unique to P. falciparum among the human Plasmodium species, which may explain why rosetting is not associated with severe malaria in P. ovale, P. malariae or P.vivax. Rosetting also occurs in several rodent and primate plasmodia such as Plasmodium chabaudi (Mackinnon et al., 2002), Plasmodium fragile (David et al., 1988), Plasmodium coatneyi (Kawai et al., 1995), and Plasmodium cynomolgi (Handunnetti et al., 1989). The widespread occurrence of rosetting in a variety of plasmodium species suggests that rosetting may have an important biological function, however this function is currently unknown.
Rosetting in P. falciparum field isolates has been positively associated with host parasitaemia in African children (Rowe et al., 2002a). It had been postulated that the function of rosetting could be to facilitate merozoite invasion (Wahlgren et al., 1989), which would promote high asexual blood stage parasitaemias in the host. However, experiments with a culture-adapted laboratory strain PA1 showed conclusively that there was no difference in invasion rates between the isogenic rosetting and non-rosetting parasites in this strain (Clough et al., 1998).
We hypothesised that increased invasion of rosetting parasites may only occur in the presence of host immunity, when rosettes could potentially act as an immune evasion mechanism by shielding the merozoites from host invasion-inhibitory antibodies. It is plausible that merozoites released from a rosetting iRBC may be able to reinvade the neighbouring RBC without prolonged exposure to host antibodies in the plasma, as indicated in Fig. 1A. Merozoites from non-rosetting parasites would be exposed to plasma antibodies that could inhibit their invasion (Fig. 1B). If this were the case, one would expect rosetting parasites to have higher invasion rates than isogenic non-rosetting parasites in the presence of invasion inhibiting antibodies. To test this hypothesis, we compared the invasion rates of isogenic rosetting and non-rosetting parasite lines of two culture-adapted P. falciparum strains in the presence of invasion-inhibitory antibodies to MSP-1(merozoite surface protein one) (Singh et al., 2003).
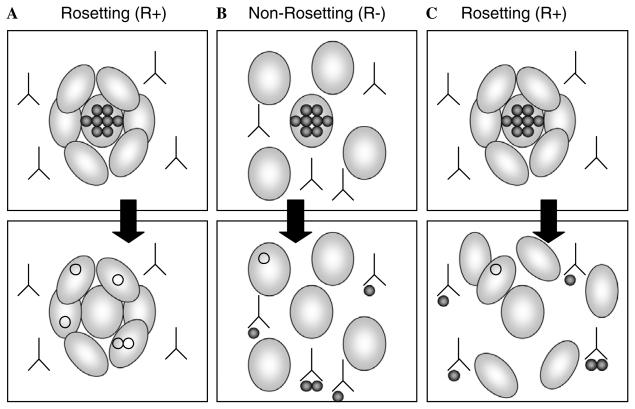
Diagram showing how merozoites released from rosetting and non-rosetting P. falciparum iRBC could be differentially inhibited by invasion-inhibitory antibodies. (A) Rosetting might reduce the effect of invasion-inhibitory antibodies if merozoites invade directly into the RBC in rosettes, thereby avoiding prolonged exposure to antibodies in the host's plasma. (B) Merozoites derived from non-rosetting parasites would be exposed to antibodies in the plasma and hence would show lower invasion rates than merozoites from rosetting parasites. (C) If merozoites from rosetting parasites do not preferentially invade the RBC in the rosette but are exposed to plasma antibodies in a similar way to merozoites from non-rosetting parasites, they would be expected to show reduced invasion rates in the presence of host antibodies. Our results indicate that merozoites released from rosetting parasites are inhibited to the same extent as merozoites released from non-rosetting parasites (B and C).
2. Methods
2.1. Parasite culture
The parasites studied were P. falciparum clone R29 (derived from the IT strain) and strain TM284 (Rowe et al., 1997). R29 and TM284 are genotypically distinct and express different PfEMP1 variants (Rowe et al., 1997 and unpublished data). Parasites were cultured in complete RPMI (cRPMI: RPMI 1640 plus 25 mM Hepes, 2 mM glutamine, 25 mM glucose, 25 μg/ml gentamicin, and 10% pooled human serum) at 1–2% haematocrit in O+ RBC at 37 °C with 3% CO2, 1% O2, and 96% N2. Parasites were synchronised by sorbitol lysis (Lambros and Vanderberg, 1979). To select for isogenic rosetting and non-rosetting parasite lines, the cultures were selected using plasmagel flotation (Pasvol et al., 1978; Rowe et al., 1997). Following suspension in plasmagel solution, the rosetting trophozoite-stage parasites settle to the bottom with the RBC pellet and the non-rosetting trophozoite-stage parasites float in the upper layer above the RBC pellet. The non-rosetting upper layer was carefully removed and both layers were washed and then returned to culture. Repeated plasmagel selections over 1–2 weeks result in rosetting lines with >65% rosette frequency and non-rosetting lines with <6% rosette frequency.
2.2. Invasion assays
Synchronous trophozoite-stage parasite cultures were diluted to approximately 1% parasitaemia with RBC and resuspended in cRPMI at 2% haematocrit. Fifty microlitre samples were set up in quadruplicate or triplicate wells in a 96-well flat-bottomed plate. The wells immediately surrounding the samples had been prepared to contain 100 μl incomplete RPMI to prevent significant evaporation from test wells during the assay. The plate was placed in a gas modulator chamber and gassed with 3% CO2, 1% O2, and 96% N2 for 3 min before being placed at 37 °C for 24h. From the remaining sample, pre-invasion smears were made to count the exact pre-invasion parasitaemia on a Giemsa-stained thin smear. After 24h, Giemsa-stained thin smears were made from all wells and the parasitaemia was assessed by counting 1000 RBC. The invasion rate is the post-invasion parasitaemia divided by the pre-invasion parasitaemia.
Invasion inhibition assays were carried out using rabbit polyclonal IgG to MSP-1–42 (Singh et al., 2003). We also tested a monoclonal antibody to MSP-1 (Blackman et al., 1990; McBride and Heidrich, 1987), two other rabbit polyclonal antibodies to MSP-1 (gift from Dr. Mike Blackman) and a rabbit polyclonal IgG to AMA-1 (gift from Dr. Allan Saul), however, none of these reagents gave significant inhibition of invasion with R29 or TM284. For the assays with the anti-MSP-1–42 antibody, the starting cultures were diluted to 0.9–1.3% parasitaemia with RBC and resuspended to 2% haematocrit with no added antibody (control) or a final concentration of 1.5 mg/ml of anti-MSP-1 IgG. Assays were set up and invasion rates were assessed as described above, with triplicate wells for each antibody and control. To ensure that the invasion-inhibitory antibody did not disrupt rosettes, samples of the rosetting parasites were incubated with the MSP-1–42 antibodies at the above concentration for 30 min at 37 °C and then the rosette frequency was assessed.
2.3. Assessment of rosette frequency
A 200 μl aliquot of culture suspension containing mature pigmented trophozoite-stage parasites was stained with 25 μg/ml ethidium bromide for 5 min at 37 °C. Ten microlitres of culture suspension was placed on a microscope slide and covered with a coverslip. The stained sample was viewed with a 40× objective using a Leica fluorescence microscope with both UV and brightfield light to allow visualisation of both iRBC and RBC simultaneously. Two hundred iRBC were counted and scored for rosetting, with a rosette being defined as an iRBC binding two or more RBC. The percentage of the 200 iRBC in rosettes is termed the rosette frequency (RF). For the invasion assays, rosetting cultures had a RF of above 65% and non-rosetting cultures had a RF of less than 6%.
2.4. Statistics
Statistical analysis was done with Statview 5.0.1 software. The invasion rates of isogenic rosetting and non-rosetting parasites were analysed with a paired Student's t test, paired by experiment to control for variations in invasion due to RBC donor, RBC age, and human sera. The percentage inhibition of invasion by the MSP-1–42 antibody of rosetting and non-rosetting parasites was analysed with a Student's t test.
3. Results and discussion
3.1. Invasion rates of isogenic rosetting and non-rosetting parasites
We first set out to investigate whether isogenic rosetting and non-rosetting parasite lines derived from R29 and TM284 differed in their invasion rates under standard in vitro culture conditions. Over seven (TM284) or eight (R29) experiments, there was no significant difference in the invasion rates of the isogenic rosetting and non-rosetting lines (Table 1, P=0.86 and P=0.21, paired Student's t test, TM284 and R29, respectively). We therefore confirmed the previous findings of Clough et al. that rosetting does not enhance invasion (Clough et al., 1998).
Table 1
Rosette frequency and invasion rate for two pairs of isogenic P. falciparum rosetting and non-rosetting parasites
Strain | Ra | N | RFb | Invasion ratec | Paired t testd |
---|---|---|---|---|---|
TM284 | R+ | 7 | 74 (4.8) | 6.7 (1.6) | P= 0.86 |
R− | 7 | 3 (2.4) | 6.6 (2.0) | ||
R29 | R+ | 8 | 77 (8.3) | 5.3 (1.8) | P= 0.21 |
R− | 8 | 2 (1.6) | 4.4 (1.6) |
3.2. Invasion rates of isogenic rosetting and non-rosetting parasites in the presence of antibodies to MSP-1
To examine the hypothesis that rosetting may enhance invasion in the presence of host invasion-inhibitory antibodies (Figs. 1A and B), we studied the invasion rates of isogenic rosetting and non-rosetting parasites in the presence of antibodies to MSP-1–42 (Singh et al., 2003). In two experiments with both TM284 and R29, we found that the isogenic rosetting and non-rosetting parasites did not differ significantly in the percentage inhibition of invasion in the presence of the MSP-1–42 antibodies (Table 2, P= 0.62 and P=0.63, Student's t test, TM284 and R29, respectively). The mean inhibition of invasion for rosetting parasites was 33.0% (95% confidence interval 25.5–40.5%) and for non-rosetting parasites was 31.8% (95% confidence interval 26.3–37.3%). Therefore, we found no evidence to support the hypothesis that rosettes protect merozoites from invasion-inhibitory antibodies as hypothesised in Fig. 1A. Instead, the data suggest that rosetting parasites are inhibited by invasion-inhibitory antibodies to the same extent as non-rosetting parasites (Figs. 1B and C).
Table 2
Invasion inhibition of two pairs of isogenic rosetting and non-rosetting P. falciparum parasites
Strain | Experiment | Ra | RFb | Invasion ratec | Inhibitiond | |
---|---|---|---|---|---|---|
Control | MSP-1 Ab | |||||
TM284 | 1 | R+ | 70 | 7.4 (1.2) | 4.9 (0.1) | 33.3 |
R− | 2 | 6.8 (1.4) | 4.7 (0.3) | 30.2 | ||
2 | R+ | 68 | 9.0 (1.2) | 6.2 (0.9) | 31.2 | |
R− | 0 | 8.7 (0.2) | 5.2 (0.7) | 40.2 | ||
R29 | 1 | R+ | 76 | 5.1 (1.2) | 3.8 (0.3) | 24.4 |
R− | 0 | 2.5 (0.3) | 1.8 (0.2) | 28.0 | ||
2 | R+ | 76 | 5.9 (0.3) | 3.4 (0.2) | 42.9 | |
R− | 0 | 4.3 (1.2) | 3.1 (0.3) | 28.9 |
Although we have found no evidence to support the hypothesis that rosettes protect merozoites from invasion-inhibitory antibodies, because of the variation in the experiments shown in Table 2, we cannot completely exclude the possibility of a minor difference in invasion inhibition between rosetting and non-rosetting parasites. To increase the sensitivity of these experiments, we attempted to identify a reagent that would provide greater inhibition of rosetting for repeated assays, however, none of the other reagents to MSP-1 and AMA-1 that were tested (see Section 2) showed any significant invasion inhibition in either R29 or TM284. We also attempted to examine the inhibitory effect of the MSP-1–42 antibodies on R29 and TM284 cultures under shaking conditions, however, no inhibition of invasion was seen when the cultures were shaken rather than static (data not shown).
To ensure that our results were not due to rosettes being disrupted by the invasion-inhibitory antibodies, we assessed the effect of the MSP-1–42 antibodies on rosetting. For both R29 and TM284, we found that the RF did not change in the presence of the MSP-1–42 antibodies (R29—control with no antibody 48% RF, 1.5mg/ml MSP-1–42 52% RF; TM284—control with no antibody 83% RF, 1.5mg/ml MSP-1–42 84% RF).
It is unclear whether rosettes are stable during schizogony or whether they break apart before the schizonts rupture. Our results point towards the latter being the most likely possibility. This suggestion is consistent with previous findings showing that the RBC forming the rosette are not preferentially targeted during invasion (Clough et al., 1998).
In the invasion inhibition experiments (Table 2), the data show a trend towards rosetting R29 parasites having higher overall invasion rates than R29 non-rosetting parasites in the control cultures without added antibodies. However, as shown in Table 1, an analysis of a larger number of experiments does not support a significant difference in invasion rates between these isogenic lines.
Although we have found no evidence to support the hypothesis that rosettes protect merozoites from invasion-inhibitory antibodies, it remains possible that rosettes may affect the interactions of iRBC with other components of the host's immune system. Contact-dependent activation of natural killer cells—an important first line in innate immunity against P. falciparum—has been reported (Artavanis-Tsakonas et al., 2003), and this activation may not occur if uninfected RBC surround the iRBC. Contrary to this, binding of iRBC to dendritic cells has been shown to modulate the function of antigen-presenting cells to possibly delay an immune reaction (Urban et al., 1999), so rosetting may prevent the binding of iRBC to receptors on the surface of dendritic cells and may promote dendritic cell activity. Furthermore, rosettes may shield the iRBC from phagocytosis by macrophages and neutrophils. Future experiments will compare natural killer cell activation, dendritic cell modulation, and phagocytosis of rosetting and non-rosetting parasites in an attempt to understand the biological function of rosetting.
Acknowledgments
We are grateful to Mike Blackman, Tony Holder, Carole Long, Siddhartha Mahanty, and Allan Saul for the gift of invasion-inhibitory antibodies. This work was funded by the Wellcome Trust (4 year PhD studentship to A.M.D. and Senior Research Fellowship Grant No. 067431 to J.A.R.).
Abbreviations
RBC | red blood cells |
iRBC | infected red blood cells |
cRPMI | complete RPMI |
RF | rosetting frequency |
MSP-1 | merozoite surface protein 1 |
AMA1 | apical membrane antigen 1 |
References
- Angus BJ, Thanikkul K, Silamut K, White NJ, Udomsangpetch R. Short report: Rosette formation in Plasmodium ovale infection. American Journal of Tropical Medicine and Hygiene. 1996;55:560–561. [Abstract] [Google Scholar]
- Artavanis-Tsakonas K, Eleme K, McQueen KL, Cheng NW, Parham P, Davis DM, Riley EM. Activation of a subset of human NK cells upon contact with Plasmodium falciparum-infected erythrocytes. Journal of Immunology. 2003;171:5396–5405. [Abstract] [Google Scholar]
- Blackman MJ, Heidrich HG, Donachie S, McBride JS, Holder AA. A single fragment of a malaria merozoite surface protein remains on the parasite during red cell invasion and is the target of invasion-inhibiting antibodies. Journal of Experimental Medicine. 1990;172:379–382. [Europe PMC free article] [Abstract] [Google Scholar]
- Carlson J, Wahlgren M. Plasmodium falciparum erythrocyte rosetting is mediated by promiscuous lectin-like interactions. Journal of Experimental Medicine. 1992;176:1311–1317. [Europe PMC free article] [Abstract] [Google Scholar]
- Clough B, Atilola FA, Pasvol G. The role of rosetting in the multiplication of Plasmodium falciparum: rosette formation neither enhances nor targets parasite invasion into uninfected red cells. British Journal of Haematology. 1998;100:99–104. [Abstract] [Google Scholar]
- Cockburn IA, Mackinnon MJ, O'Donnell A, Allen SJ, Moulds JM, Baisor M, Bockarie M, Reeder JC, Rowe JA. A human complement receptor 1 polymorphism that reduces Plasmodium falciparum rosetting confers protection against severe malaria. Proceedings of the National Academy of Sciences of the United States of America. 2004;101:272–277. [Europe PMC free article] [Abstract] [Google Scholar]
- David PH, Handunnetti SM, Leech JH, Gamage P, Mendis KN. Rosetting: a new cytoadherence property of malaria-infected erythrocytes. American Journal of Tropical Medicine and Hygiene. 1988;38:289–297. [Abstract] [Google Scholar]
- Handunnetti SM, David PH, Perera KL, Mendis KN. Uninfected erythrocytes form “rosettes” around Plasmodium falciparum infected erythrocytes. American Journal of Tropical Medicine and Hygiene. 1989;40:115–118. [Abstract] [Google Scholar]
- Kaul DK, Roth EF, Nagel RL, Howard RJ, Handunnetti SM. Rosetting of Plasmodium falciparum-infected red blood cells with uninfected red blood cells enhances microvascular obstruction under flow conditions. Blood. 1991;78:812–819. [Abstract] [Google Scholar]
- Kawai S, Kano S, Suzuki M. Rosette formation by Plasmodium coatneyi-infected erythrocytes of the Japanese macaque (Macaca fuscata) American Journal of Tropical Medicine and Hygiene. 1995;53:295–299. [Abstract] [Google Scholar]
- Lambros C, Vanderberg JP. Synchronization of Plasmodium falciparum erythrocytic stages in culture. Journal of Parasitology. 1979;65:418–420. [Abstract] [Google Scholar]
- Lowe BS, Mosobo M, Bull PC. All four species of human malaria parasites form rosettes. Transactions of the Royal Society of Tropical Medicine and Hygiene. 1998;92:526. [Abstract] [Google Scholar]
- Mackinnon MJ, Walker PR, Rowe JA. Plasmodium chabaudi: rosetting in a rodent malaria model. Experimental Parasitology. 2002;101:121–128. [Abstract] [Google Scholar]
- McBride JS, Heidrich HG. Fragments of the polymorphic Mr 185,000 glycoprotein from the surface of isolated Plasmodium falciparum merozoites form an antigenic complex. Molecular and Biochemical Parasitology. 1987;23:71–84. [Abstract] [Google Scholar]
- Nash GB, Cooke BM, Carlson J, Wahlgren M. Rheological properties of rosettes formed by red blood cells parasitized by Plasmodium falciparum. British Journal of Haematology. 1992;82:757–763. [Abstract] [Google Scholar]
- Newbold C, Craig A, Kyes S, Rowe A, Fernandez-Reyes D, Fagan T. Cytoadherence, pathogenesis and the infected red cell surface in Plasmodium falciparum. International Journal for Parasitology. 1999;29:927–937. [Abstract] [Google Scholar]
- Pasvol G, Wilson RJ, Smalley ME, Brown J. Separation of viable schizont-infected red cells of Plasmodium falciparum from human blood. Annals of Tropical Medicine and Parasitology. 1978;72:87–88. [Abstract] [Google Scholar]
- Ringwald P, Peyron F, Lepers JP, Rabarison P, Rakotomalala C, Razanamparany M, Rabodonirina M, Roux J, Le Bras J. Parasite virulence factors during falciparum malaria: rosetting, cytoadherence, and modulation of cytoadherence by cytokines. Infection and Immunity. 1993;61:5198–5204. [Europe PMC free article] [Abstract] [Google Scholar]
- Rowe A, Obeiro J, Newbold CI, Marsh K. Plasmodium falciparum rosetting is associated with malaria severity in Kenya. Infection and Immunity. 1995;63:2323–2326. [Europe PMC free article] [Abstract] [Google Scholar]
- Rowe JA, Moulds JM, Newbold CI, Miller LH. P. falciparum rosetting mediated by a parasite-variant erythrocyte membrane protein and complement-receptor 1. Nature. 1997;388:292–295. [Abstract] [Google Scholar]
- Rowe JA, Obiero J, Marsh K, Raza A. Short report: Positive correlation between rosetting and parasitemia in Plasmodium falciparum clinical isolates. American Journal of Tropical Medicine and Hygiene. 2002a;66:458–460. [Abstract] [Google Scholar]
- Rowe JA, Shafi J, Kai O, Marsh K, Raza A. Nonimmune IgM but not IgG binds to the surface of Plasmodium falciparum infected erythrocytes and correlates with rosetting and severe malaria. American Journal of Tropical Medicine and Hygiene. 2002b;66:692–699. [Abstract] [Google Scholar]
- Singh S, Kennedy MC, Long CA, Saul AJ, Miller LH, Stowers AW. Biochemical and immunological characterization of bacterially expressed and refolded Plasmodium falciparum 42-kilodalton C-terminal merozoite surface protein 1. Infection and Immunity. 2003;71:6766–6774. [Europe PMC free article] [Abstract] [Google Scholar]
- Udomsanpetch R, Thanikkul K, Pukrittayakamee S, White NJ. Rosette formation by Plasmodium vivax. Transactions of the Royal Society of Tropical Medicine and Hygiene. 1995;89:635–637. [Abstract] [Google Scholar]
- Urban BC, Ferguson DJ, Pain A, Willcox N, Plebanski M, Austyn JM, Roberts DJ. Plasmodium falciparum-infected erythrocytes modulate the maturation of dendritic cells. Nature. 1999;400:73–77. [Abstract] [Google Scholar]
- Wahlgren M, Carlson J, Udomsangpetch R, Perlmann P. Why do Plasmodium falciparum-infected erythrocytes form spontaneous erythrocyte rosettes? Parasitology Today. 1989;5:183–185. [Abstract] [Google Scholar]
Full text links
Read article at publisher's site: https://doi.org/10.1016/j.exppara.2005.11.007
Read article for free, from open access legal sources, via Unpaywall:
https://www.pure.ed.ac.uk/ws/files/7616266/EPMCFG_2006_3_Rowe.pdf
Citations & impact
Impact metrics
Citations of article over time
Alternative metrics
Smart citations by scite.ai
Explore citation contexts and check if this article has been
supported or disputed.
https://scite.ai/reports/10.1016/j.exppara.2005.11.007
Article citations
Mechanism of Immune Evasion in Mosquito-Borne Diseases.
Pathogens, 12(5):635, 23 Apr 2023
Cited by: 3 articles | PMID: 37242305 | PMCID: PMC10222277
Review Free full text in Europe PMC
Measuring Rosetting Inhibition in Plasmodium falciparum Parasites Using a Flow Cytometry-Based Assay.
Methods Mol Biol, 2470:493-503, 01 Jan 2022
Cited by: 0 articles | PMID: 35881369
Plasmodium-infected erythrocytes induce secretion of IGFBP7 to form type II rosettes and escape phagocytosis.
Elife, 9:e51546, 18 Feb 2020
Cited by: 12 articles | PMID: 32066522 | PMCID: PMC7048393
Rosetting revisited: a critical look at the evidence for host erythrocyte receptors in Plasmodium falciparum rosetting.
Parasitology, 147(1):1-11, 16 Sep 2019
Cited by: 17 articles | PMID: 31455446 | PMCID: PMC7050047
Review Free full text in Europe PMC
'2TM proteins': an antigenically diverse superfamily with variable functions and export pathways.
PeerJ, 6:e4757, 11 May 2018
Cited by: 5 articles | PMID: 29770278 | PMCID: PMC5951124
Go to all (14) article citations
Similar Articles
To arrive at the top five similar articles we use a word-weighted algorithm to compare words from the Title and Abstract of each citation.
Short report: Positive correlation between rosetting and parasitemia in Plasmodium falciparum clinical isolates.
Am J Trop Med Hyg, 66(5):458-460, 01 May 2002
Cited by: 49 articles | PMID: 12201576
Detection of naturally acquired, strain-transcending antibodies against rosetting Plasmodium falciparum strains in humans.
Infect Immun, 92(7):e0001524, 06 Jun 2024
Cited by: 0 articles | PMID: 38842304 | PMCID: PMC11238554
Immunisation with recombinant PfEMP1 domains elicits functional rosette-inhibiting and phagocytosis-inducing antibodies to Plasmodium falciparum.
PLoS One, 6(1):e16414, 31 Jan 2011
Cited by: 31 articles | PMID: 21305024 | PMCID: PMC3031562
Three Is a Crowd - New Insights into Rosetting in Plasmodium falciparum.
Trends Parasitol, 33(4):309-320, 18 Jan 2017
Cited by: 27 articles | PMID: 28109696
Review
Funding
Funders who supported this work.
Wellcome Trust (1)
Investigation into the potential for a Plasmodium falciparum erythrocyte membrane protein one (PfEMP1) vaccine to inhibit rosetting and prevent severe malaria.
Dr Rowe, University of Edinburgh
Grant ID: 067431