Abstract
Free full text

The Role of Stem Cells in Liver Repair and Fibrosis
Abstract
In response to liver injury or loss of liver mass, proliferation of mature liver cells is the first line defense to restore liver homeostasis. In the setting of chronic liver disease, however, the ability of hepatocytes and cholangiocytes to proliferate is blocked and small bipotential progenitor cells are activated. Recent studies have established the role of these facultative progenitor cells in injury repair and fibrosis in patients with chronic liver disease and in experimental models. Several signaling pathways linking progenitor cell activation and fibrosis have been identified, and there is increasing evidence that cross-talk (both physical and via soluble factors) between progenitor cells and myofibroblasts is essential for both fibrosis and parenchymal regeneration. Even more exciting are new data examining the cellular components of the progenitor cell niche, demonstrating that both resident liver cells and circulating cells from the bone marrow can function as stem cells, and suggesting that there is a surprising degree of phenotypic plasticity, such that progenitor cells can contribute to the myofibroblast population and vice versa. We highlight here recent findings from the literature demonstrating the cellular and functional complexity of the progenitor cell niche, and emphasize some of the important questions that remain to drive future research.
1. Introduction
The liver is a highly regenerative organ with the ability to restore its mass even in the face of massive parenchymal cell loss. Although often thought of in the context of acute liver injury, liver regeneration and reconstitution are also associated with fibrosis in chronic liver injury. The recent literature has provided new insights establishing a role for stem cells and progenitor cells in fibrosis and repair. We highlight here studies detailing the relationship between progenitor cells and myofibroblasts, and introducing the concept of phenotypic plasticity with a particular focus on evidence suggesting that stem cells contribute to the myofibroblast population and vice versa.
1.1 Hepatocytes and progenitor cells both contribute to liver repair
Hepatocytes and biliary epithelial cells (cholangiocytes) in the normal liver are quiescent. In response to liver injury or loss of liver mass, mature, differentiated liver cells proliferate as a first-line defense to restore homeostasis. Despite the low rate of hepatocyte turnover in the healthy liver, serial transplantation studies have demonstrated that differentiated hepatocytes are able to proliferate through many divisions in hosts in which the transplanted hepatocytes have a survival advantage (Overturf et al., 1999). In patients or in animal models of chronic liver disease, however, hepatocytes and cholangiocytes are blocked from proliferating, and a second mechanism of regeneration comes into play as small bipotential progenitor cells with the ability to differentiate into hepatocytes or cholangiocytes become activated (Thorgeirsson, 1996, Alison et al., 1998, Bisgaard et al., 2002, Tan et al., 2002, Fausto, 2004). These facultative stem cells, described in rodent models as oval cells or intermediate hepatobiliary cells, and referred to here as liver progenitor cells, have the appearance of small hepatocytes with scant cytoplasm. Whether or not small numbers of pluripotent stem cells also persist in the adult liver in addition to these bipotential progenitor cells is still unresolved ((Zhang et al., 2008) and reviewed in (Bird et al., 2008, Duncan et al., 2009)).
In addition to their role in liver repair, liver progenitor cells have received significant attention because they may contribute to a subset of hepatocellular cancers exhibiting stem cell characteristics. Progenitor cells are also of great interest to the biomedical community as a potential alternative to organ transplantation. These topics are beyond the scope of this article, and we direct readers to several recent reviews on the role of liver progenitor cells in stem cell cancers (Sell and Leffert, 2008, Mishra et al., 2009) and cell transplantation therapy (Oertel and Shafritz, 2008, Dalgetty et al., 2009).
1.2 The hepatic stem cell niche
A stem cell niche has been defined as a specialized microenvironment that “directly promotes the maintenance of stem cells” (Morrison and Spradling, 2008). In the niche, direct contact between stem cells and supporting cells in their local microenvironment is thought to be responsible for preserving the balance between stem cell self-renewal and differentiation (see section 4) (Nusse, 2008). Multiple groups of investigators have now clearly demonstrated in vivo and in vitro that the adult liver contains bipotential progenitor cells capable of self-renewal and differentiation towards either hepatocyte or cholangiocyte lineages ((Kamiya et al., 2009a); reviewed in (Duncan et al., 2009)); the precise location of the progenitor cell niche and the cellular components of this niche, however, have not been definitively established and results in the literature are conflicting (Petersen and Shupe, 2008). Cell populations that participate in the progenitor cell niche potentially include Kupffer cells (reviewed in (Holt et al., 2008)) and lymphocytes (T cells, NK and NKT cells) (Strick-Marchand et al., 2008). The recent study from Strick-Marchand and colleagues investigating CDE-diet induced progenitor cell proliferation in lymphocyte deficient animals highlights the concept that a critical balance between progenitor cell expansion and inflammation is necessary for liver repair and survival (Strick-Marchand et al., 2008). It may be that there are multiple niches, and potentially heterogeneous populations of progenitor and support cells. Evidence in support of this possibility was published by Kuwahara and colleagues, who used a label retention assay in acetaminophen-treated mice to identify four stem cell niches: the canal of Hering, intralobular bile ducts, periductal “null” mononuclear cells, and peribiliary hepatocytes (Kuwahara et al., 2008). The existence of multiple hepatic stem cell niches suggests that the activation of cells in a particular niche might depend on the mechanism and location of injury (Kuwahara et al., 2008, Petersen and Shupe, 2008).
The definitive characterization of hepatic progenitor cell niche(s) in experimental models and in humans will require the identification of specific stem cell markers. The winged helix factor, Foxl1 represents a candidate marker for bipotential hepatic progenitor cells, as it co-labels cells with either the cholangiocyte marker cytokeratin 19 or the hepatocyte-specific transcription factor HNF-4α following liver injury (Sackett et al., 2009). In a complementary approach, Kamiya and colleagues recently showed that single cell cultures of isolated hepatic progenitors were capable of self-renewal and gave rise to both hepatocyte and cholangiocyte lineages in culture (Kamiya et al., 2009b). Because these progenitors gave rise only to hepatocytes in vivo, the identification of a specific marker of bipotential progenitor cells awaits further investigation.
2. The role of hepatic progenitor cells in parenchymal and biliary repair in fibrosis and cirrhosis
Recent experiments have clearly demonstrated that there is an association between fibrosis and progenitor cell activation. Although the mechanisms that link liver progenitor cells and fibrogenic cells are as yet unknown, there are now several signaling pathways in common operating during their differentiation in liver repair and regeneration. Whether these signaling pathways are activated in the different cell types independently in parallel or whether there is cross-talk remains unclear.
2.1 The ductular reaction and fibrosis
“The ductular reaction” is the term given to a duct-like proliferation of a population of liver progenitor cells in the setting of fibrosis. The extent of the ductular reaction appears to correlate directly with impaired hepatocyte replication, consistent with the notion that progenitor cells are only recruited when replication of mature liver cells is inhibited. Multiple investigators have shown a compelling direct association between the extent of the ductular reaction and the severity of fibrosis in patients with chronic liver diseases from such diverse etiologies as hepatitis C (Clouston et al., 2005), non-alcoholic fatty liver disease (Richardson et al., 2007), and the pediatric cholangiopathies Alagille syndrome and biliary atresia (Fabris et al., 2007), suggesting that progenitor cell activation plays an important if undefined role in fibrosis.
A detailed marker analysis of ductular reactions in livers from cirrhotic patients demonstrated that these ductular reactions had a bipolar structure, with bidirectional differentiation to either hepatocytic or biliary lineages (Zhou et al., 2007). Other groups have not observed mutually exclusive expression of hepatocytic and biliary markers during progenitor cell proliferation but instead describe “intermediate hepatocytes” that express both sets of markers (Zhang et al., 2008, Fabris et al., 2007). These intermediate hepatocytes appear in contiguity with ductular progenitor cells when liver inflammation and necrosis is moderate or severe, suggesting that differentiation of progenitor cells towards the hepatocytic lineage is directly related to the extent of parenchymal injury (Lowes et al., 1999, Libbrecht et al., 2000, Roskams et al., 2003, Katoonizadeh et al., 2006). Regardless of the details of progenitor cell activation and marker expression, these observations strongly support the concept that hepatic progenitor cells of the ductular reaction contribute directly to the fibrogenic process, most likely through cell-cell cross talk between the progenitor cells and nearby mesenchymal populations and via recruitment of inflammatory cells (Strick-Marchand et al., 2008, Ruddell et al., 2009). The signals that mediate progenitor cell quiescence and activation, however, are still unclear. Nguyen and colleagues made the seminal observation that rat liver progenitor cells (oval cells), unlike hepatocytes, are resistant to the growth inhibitory effects of the growth factor TGF-β, providing at least a partial mechanistic basis for their differential activation in the TGF-β-rich environment of fibrosis and cirrhosis (Nguyen et al., 2007).
2.2 Shared signaling pathways in progenitor cell expansion and fibrogenic cell activation
The complexity of signaling pathways that regulate progenitor cell proliferation is underscored by the large number of ligands and receptors identified on either the progenitor cells themselves or adjacent cells that may be present within the progenitor cell niche (reviewed in (Michalopoulos, 2009)). Recently, the finding that hedgehog, wnt, and Notch pathways function in concert to regulate stem cell proliferation and cell fate decisions in other tissues has led investigators to explore the contribution of these signaling pathways to progenitor cell expansion, differentiation and fibrogenesis in the liver. Work by Omenetti and colleagues in both experimental models and patient livers has established that hedgehog signals are activated in various types of liver injury in adults (Omenetti and Diehl, 2008). Using a co-culture system of immature cholangiocytes and hepatic stellate cells (fibrogenic cell precursors), this group demonstrated that both cell populations are able to both respond to and produce hedgehog ligands and that the presence of these ligands is critical for survival, proliferation and migration of hepatic progenitor cells in culture (Omenetti et al., 2008). In a gain-of-function experiment of hedgehog activation, mice heterozygous for Patched (Ptc+/−), an inhibitory subunit of the hedgehog receptor complex, spontaneously develop expanded ductular reactions and increased fibrosis relative to littermate controls (Omenetti et al., 2007). While this study indicates that constitutive activation of hedgehog signals in the liver is associated with progenitor and/or cholangiocyte proliferation and fibrosis, understanding the contribution of hedgehog signals in stimulating progenitor cell proliferation and activating matrix-producing cells will require the use of additional genetic models.
Several recent gain- and loss-of-function studies in rodents have shown that wnt/β-catenin signals also play an important role in hepatic progenitor cell self-renewal and expansion. Oval cell expansion is decreased when β-catenin signaling is inhibited in hepatocytes and cholangiocytes (Apte et al., 2008) and forced expression of a constitutively active β-catenin mutant in isolated hepatic progenitor cells confers increased self-renewal behavior in vitro and results in the development of hepatic stem cell tumors in a xenotransplantation model (Chiba et al., 2007). Similarly, canonical wnt signaling is required for hepatic stellate cell activation, one of the major events in liver fibrosis (Cheng et al., 2008, Myung et al., 2007). While these studies provide evidence for the importance of wnt/β-catenin signaling in hepatic progenitor cells and in one of the most important profibrogenic populations in the liver, many questions remain to be addressed including identification of the upstream activator of the wnt/β-catenin pathway during liver injury and a detailed knowledge of autocrine and paracrine signals that regulate progenitor cell expansion. In particular, whether similar wnt activators stimulate both liver progenitor cells and hepatic stellate cells has not been examined. The expression of a large number of wnts, receptors and co-ligands in multiple liver cell populations poses a significant challenge to understanding wnt signaling pathways in vivo (Zeng et al., 2007).
The Notch pathway has well-established roles in biliary differentiation and morphogenesis during development and in duct remodeling during the postnatal period (Geisler et al., 2008, Lozier et al., 2008, Zong et al., 2009, Loomes et al., 2007). Recent observations from the livers of patients with Alagille syndrome, a genetic disease associated with impaired Notch signaling, suggest that this pathway may also be essential for biliary repair (Fabris et al., 2007, Loomes et al., 2007, Ryan et al., 2008). Fabris and colleagues have shown that ductular cells in livers from patients with Alagille syndrome fail to express markers of differentiated cholangiocytes, implicating Notch signaling in the regulation of hepatic progenitor cell differentiation to mature cholangiocytes during postnatal liver repair (Fabris et al., 2007). They provide evidence from lineage marker analysis in Alagille syndrome livers to suggest that, in this setting where hepatic progenitor cell differentiation towards the biliary lineage is blocked, hepatocytes undergo an incomplete transdifferentiation towards the biliary lineage. Alagille syndrome livers exhibited significantly reduced fibrosis when compared to livers from patients with biliary atresia, suggesting that fibrosis was dependent upon the presence of reactive ductular cells which are present in biliary atresia livers but absent from the livers from the Alagille syndrome patients. Additional support for the concept that reactive ductular proliferation and fibrosis are dependent on Notch signals in the postnatal period comes from observations in mice haploinsufficient for the Notch ligand Jagged and for members of the Fringe family of Notch receptor modifiers (Ryan et al., 2008). These mice exhibited diffuse bile duct proliferation that was not accompanied by increased fibrosis. It would be interesting to know whether ductular cells in these mice express increased numbers of intermediate hepatobiliary cells as observed in Alagille syndrome livers.
3. The source of progenitor cells in fibrosis
Our understanding of the complex origins of progenitor cells and fibrogenic cells is still rudimentary. On the one hand, there is data in the literature demonstrating that progenitor cells from both the liver and the bone marrow can become hepatic stellate cells and myofibroblasts in fibrosis. On the other hand, some groups suggest that stellate cells are themselves progenitor cells and play an important role in parenchymal and non-parenchymal cell reconstitution in the injured liver. Both concepts remain controversial, and questions about the quantitative importance of each and the relevant stimuli and signaling pathways have not yet been investigated.
3.1 Stem cells as a source of myofibroblasts
Myofibroblasts, defined operationally as fibrogenic cells expressing α-smooth muscle actin (α-SMA), are the primary source of fibrillar collagens and other abnormal extracellular matrix (ECM) proteins in fibrosis (Hinz et al., 2007). Hepatic stellate cells, a heterogeneous population of vitamin A-storing cells normally located in the space of Disse, have long been considered the major source of myofibroblasts in fibrosis, although other resident myofibroblast precursor cells including portal fibroblasts and mesenchymal cells are increasingly recognized as providing equal if not more significant contributions (Guyot et al., 2006, Beaussier et al., 2007, Tuchweber et al., 1996, Li et al., 2007). The embryonic origin of hepatic stellate cells and portal fibroblasts is debated, although studies using transgenic mice suggest that hepatic stellate cells as well as certain perivascular mesenchymal cells originate from the mesoderm of the septum transversum (Loo and Wu, 2008, Asahina et al., 2009, Villeneuve et al., 2009, Kalinichenko et al., 2003, Friedman, 2008). Portal fibroblasts and other mesenchymal cells appear during early development (Loo and Wu, 2008, Villeneuve et al., 2009), during which time stellate cells and portal fibroblasts share at least one specific marker (p75NTR) (Suzuki et al., 2008). Whether progenitor cells also contribute to the population of stellate cells, portal fibroblasts, and other myofibroblasts in the setting of significant or chronic injury in the adult liver is less clear.
Bone marrow transplants have been touted as a potential therapy for chronic liver disease (Houlihan and Newsome, 2008); thus, understanding the relationship between bone marrow stem cells and matrix producing cells in fibrosis is particularly important. There are now multiple reports suggesting that progenitor cells from the bone marrow can migrate to the injured liver and differentiate into stellate cells and myofibroblasts. In the most quantitative study, Russo et al. carried out male to female bone marrow transplants and found that 70% of fibrogenic myofibroblasts were derived from transplanted marrow cells. Transplantation of cells from mice with a mutant, degradation-resistant collagen I (col 1a1rr) resulted in increased fibrosis (Russo et al., 2006). A variety of other studies, most involving whole bone marrow transplantation but at least one in which the clonal progeny of a single hematopoietic stem cell were transplanted, yielded similar evidence that marrow-derived progenitor cells could generate fibrogenic myofibroblasts (Miyata et al., 2008, Kisseleva et al., 2006, Baba et al., 2004). A more recent study by Higashiyama et al., however, showed no evidence that any collagen expression in the injured liver was from bone marrow-derived cells (Higashiyama et al., 2009). In an elegant review, Kallis and Forbes summarized the existing studies, suggesting that heterogeneity in the population of bone marrow-derived stem cells explains these divergent results (Kallis and Forbes, 2009). Mesenchymal stem cells, which are resistant to radiation, and hematopoietic stem cells, which are radiosensitive, appear to have different abilities to repopulate the liver, and differences in experimental techniques may select for one versus the other. Interestingly, mesenchymal stem cells may also have indirect effects on fibrosis via the modulation of hepatic stellate cell phenotype (Parekkadan et al., 2007). Ultimately, improved marker analysis for bone marrow stem cells may be required in clinical applications in order to carry out selective transplantation of cells with minimal fibrogenic potential.
3.2 The relationship between stellate cells and resident liver stem cells
Several groups have demonstrated that hepatic stellate cells express epithelial markers, including E-cadherin and cytokeratins, and have raised the possibility that stellate cells are derived from epithelial cells or are themselves progenitor cells giving rise to epithelial cells (Sicklick et al., 2006, Lim et al., 2007, Cassiman and Roskams, 2002, Suskind and Muench, 2004, Yang et al., 2008). Haussinger and colleagues have demonstrated that primary hepatic stellate cells express the stem cell marker CD133 and possess the ability to differentiate into endothelial cells and hepatocytes, suggesting that they are progenitor cells (Kordes et al., 2007); this group also argues that the space of Disse in which stellate cells reside has the characteristics of a stem cell niche (Sawitza et al., 2009, Kordes et al., 2009). Diehl and colleagues have hypothesized more specifically that hepatic stellate cells are a form of oval cell and can differentiate into hepatocytes during injury. This group used glial fibrillary acidic protein (GFAP)-Cre mice crossed with different LoxP reporter mice to show that large numbers of hepatocyte-like, albumin-expressing cells carrying the reporter tag appeared after mice were treated with a methionine-choline-deficient, ethionine-supplemented diet (Yang et al., 2008). The starting population of tagged cells included both perisinusoidal cells, thought to be hepatic stellate cells, and cholangiocytes; however, the epithelial cells did not proliferate in the context of injury and so were not thought to have contributed to parenchymal reconstitution. Unfortunately, GFAP is expressed by oval cells as well as stellate cells; the definitive demonstration that stellate cells differentiate into hepatocytes will require use of a stellate cell-specific marker and single cell culture techniques.
Thus, there is now intriguing evidence to suggest that hepatic stellate cells, one of the most important myofibroblast precursor populations, may function as stem or progenitor cells. Notably, neither self-renewal nor transplantability has been demonstrated; additionally, single cells were not analyzed, so contamination of primary stellate cell cultures by another progenitor population cannot be excluded. The use of stellate cell-specific markers and inducible transgenic animals may enable the stem cell nature of stellate cells to be definitively addressed in the future. One of the most interesting questions to be answered will be the relationship between the myofibroblastic and epithelial differentiation pathways of stellate cells in fibrosis: what determines the pathway of a given stellate cell, which pathway predominates in different forms of injury, and how important is the myofibroblastic function of stellate cells compared to their potential role as progenitor cells?
4. Myofibroblasts as a key component of the stem cell niche
The concept of a stem cell niche has gained in importance as it has been shown for multiple progenitor cell populations that the local microenvironment - including neighboring cells, extracellular matrix, and soluble factors - is critical for localizing and maintaining the unique characteristics of stem cells (Fuchs et al., 2004). In the liver, it has recently been suggested that the space of Disse functions as a stem cell niche for hepatic stellate cells, with sympathetic innervation, matrix proteins, and signaling pathways that are key for the maintenance of “stemness” (Kordes et al., 2009, Sawitza et al., 2009, Roskams, 2008). In this context it is also plausible that the space of Disse is a niche for other resident stem cells or plays an important role in the retention and maintenance of circulating stem cells from the bone marrow. The niche for hepatic progenitor cells has not been well characterized, but new data suggest that stellate cells, portal fibroblasts, and myofibroblasts may play an important role.
4.1 Activated hepatic stellate cells and other myofibroblasts may mediate progenitor cell differentiation
As noted above (section 1), there is evidence in support of at least four stem cell niche locations in the injured liver (Kuwahara et al., 2008). As these are better defined, their relationship to myofibroblasts and myofibroblast precursors should become clearer. There is already significant evidence suggesting that hepatic stellate cells, functioning as support cells in the niche, play an important role in liver progenitor cell expansion and differentiation. Numerous studies have demonstrated a close physical relationship between stellate cells, the extracellular matrix, and oval cells, and have suggested that the two cell types proliferate in tandem (Roskams, 2008, Zhang et al., 2009, Braun et al., 2003, Paku et al., 2001). Co-culture of stellate cells and progenitor cells (either resident liver progenitor cells or circulating mesenchymal stem cells) results in increased proliferation and hepatocytic differentiation of progenitor cells (Nagai et al., 2002, Lin et al., 2008, Deng et al., 2008). Whether this requires cell-cell contact is not clear; nor is it known what the soluble mediators are, although some studies suggest that hepatocyte growth factor and sonic hedgehog play a role (Nagai et al., 2002, Lin et al., 2008, Paku et al., 2001). Progenitor cell expansion is affected by multiple soluble factors, many of which are produced by stellate cells ((Santoni-Rugiu et al., 2005) and see section 2). Notably, in all studies, the hepatic stellate cells used for co-culture experiments have been activated (myofibroblastic); in the one study that addressed this question specifically, activated (rather than quiescent) stellate cells were required for the observed effects on progenitor cells (Deng et al., 2008). The relationship between stellate cells and progenitor cells may be bidirectional: one group found that co-culture with mesenchymal stem cells resulted in stellate cell activation (Parekkadan et al., 2007).
Several studies using the choline-deficient, ethionine-supplemented diet have shown that there is a close physical association between progenitor cells and myofibroblasts (which may or may not be derived from stellate cells) and that the extent of fibrosis correlates with the degree of progenitor cell expansion (Knight et al., 2007b, Knight et al., 2007a). Ruddell and colleagues found that lymphotoxin-β (LTβ)-expressing progenitor cells were adjacent to LTβ receptor expressing myofibroblasts, and have suggested that there is a paracrine loop operational between the two cell types, mediated by LTβ (Ruddell et al., 2009).
The controversial topic of Thy-1 positive cells may provide further insight into the role of mesenchymal cells in the progenitor cell niche. The hematopoietic cell marker Thy-1 was originally identified as a marker of oval cells (Petersen et al., 1998). More recently, several groups have shown that Thy-1 positive cells are actually mesenchymal cells and are an essential component of the progenitor cell niche, required for oval cell differentiation (Hoppo et al., 2004, Koenig et al., 2006, Yovchev et al., 2008, Dudas et al., 2007, Dezso et al., 2007). Although the identity of Thy-1 positive cells remains unresolved, the literature on this topic has highlighted several interesting points: 1) that oval cells, whether or not Thy-1 positive, are in close physical contact with myofibroblasts and other mesenchymal cells in models of oval cell proliferation; 2) that oval cells themselves may express mesenchymal markers, including desmin and type I collagen; and 3) that Thy-1 positive cells are heterogeneous, some although not all appearing myofibroblastic (expressing α-SMA). Unpublished work for our laboratory suggests that the Thy-1 positive cells are negative for elastin (a marker of portal fibroblasts and myofibroblasts), suggesting that they are not portal fibroblasts, while others have shown that they are not hepatic stellate cells (Yovchev et al., 2009). This raises the possibility that there is an important and as yet undefined mesenchymal cell population contributing to the oval cell niche. In our studies of Foxl1 and bipotential progenitor cells in the liver, however, we observed that progenitor cells were encircled by α-SMA negative cells expressing elastin, apparently portal fibroblasts (Fig. 1) (Sackett et al., 2009). The relationship between Foxl1-expressing progenitors and oval cells remains unclear, as does the relationship between the elastin positive cells surrounding Foxl1 progenitors and the possible Thy-1 positive mesenchymal cells surrounding oval cells.
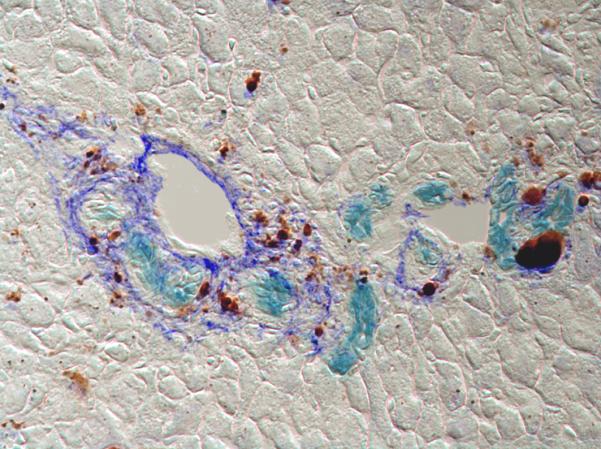
Foxl1-Cre positive progenitor cells (aqua blue) are encircled by portal fibroblasts (royal blue) in the portal areas of DDC injured liver, suggestive of a progenitor cell niche. Brown pigment represents heme breakdown product of DDC.
These studies in aggregate raise interesting questions about cross-talk between hepatic stellate cells, myofibroblasts, other mesenchymal cells, and progenitor cells in wound healing: if myofibroblasts mediate progenitor cell proliferation and differentiation, do progenitor cells in turn mediate stellate cell activation and the differentiation of other myofibroblast populations? Is it an essential feature of wound healing that scar formation and parenchymal reconstitution go hand in hand? Even more interesting is the question of whether activated stellate cells and other myofibroblasts are part of the progenitor cell niche or rather the means whereby progenitor cells exit the niche, and whether quiescent stellate cells may serve the opposite function of retaining progenitor cells in the niche. Future research will undoubtedly be aimed at understanding the heterogeneity of both progenitor cells and the supporting cells of their niches.
4.2 The role of matrix in the progenitor cell niche
Van Hul et al. recently generated liver injury via a choline-deficient, ethionine-supplemented diet to demonstrate in mice that myofibroblast activation and increased ECM deposition temporally preceded progenitor cell expansion, and that all progenitor cells, regardless of whether they were present as isolated cells or in clusters, were embedded in ECM and surrounded by myofibroblasts that were presumably the source of the new matrix (Van Hul et al., 2009). The investigators reported that α-SMA-positive myofibroblasts and matrix seemed to form a “leading edge” ahead of the expanding progenitor cells. Interestingly, in this study progenitor cells that appeared partially differentiated were beyond the limit of newly deposited matrix, raising the possibility that they exited the neomatrix zone upon differentiation. The Van Hul study is one of several reporting changes in local matrix deposition associated with progenitor cell activation (Roskams, 2008, Zhang et al., 2009, Braun et al., 2003, Paku et al., 2001, Van Hul et al., 2009). The function of this matrix in progenitor cell function is not fully understood - that a specialized matrix is required for the expansion but not differentiation of progenitor cells is one possibility. Certainly the importance of matrix to progenitor cell function implies a mechanistic link between regeneration and fibrosis.
5. Conclusions
One of the most interesting questions raised by the recent literature concerns the relationship between myofibroblasts and progenitor cells. Determining whether one is the source of the other, and how they contribute soluble factors and matrix to the niche in quiescent and activated states will be important to understanding the physiology of repair. Hepatic stellate cells in particular appear to play a special role in progenitor cell biology and appear to have previously unappreciated functions in fibrosis. The new view of stellate cells, the most studied cells in fibrosis, underscores the impact research in stem cell biology has had on models of fibrosis.
Defining the different stem cell niches - at the level of both progenitor cells and supporting cells - should be a top priority for future research. This is essential for determining whether different etiologies of injury in humans lead to different mechanisms of fibrosis and regeneration, and for designing disease-specific therapies. Additionally, the identification of markers specific to different cell populations in different niches will make it possible to perform lineage-tracing studies that provide compelling proof of the existence of progenitor cell plasticity. The identification of specific markers would also facilitate the development of genetic models to modulate the expression of individual signaling molecules in different progenitor cell populations in vivo, and ultimately to selectively enhance proliferation and differentiation while at the same time minimizing scar formation.
While there has been tremendous progress in our understanding of many aspects of progenitor cell biology in recent years, knowledge of the role of progenitor cells in fibrosis and injury repair is clearly still incomplete. Many challenges and questions will need to be addressed before we can harness the reparative potential of these cells in patients with acute and chronic liver diseases.
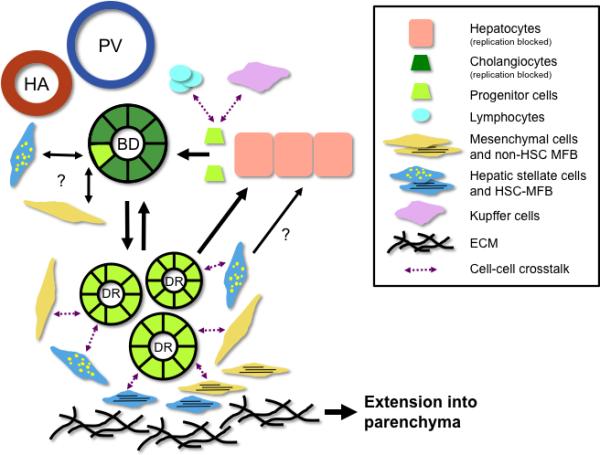
Model highlighting key aspects of proposed contributions of hepatic progenitors during fibrosis. There are multiple potential progenitor cell niches, including the Canal of Hering and bile ductules. In response to liver injury, progenitor cells proliferate to expand the ductular reaction (DR). Bidirectional signaling pathways (purple dashed arrows) between progenitor cells, portal mesenchymal cells (including portal fibroblasts and myofibroblasts), hepatic stellate cells (HSC) and HSC myofibroblasts, hepatocytes, lymphocytes, and Kupffer cells contribute to ductular expansion by stimulating progenitor cell proliferation, bidirectional cellular transdifferentiation (black solid arrows) and remodeling/deposition of extracellular matrix (ECM). The net result is thought to be extension of fibrosis deposition and cell regeneration into the parenchyma (thick black arrow). HA, hepatic artery; PV, portal vein; BD, bile ductule.
Acknowledgements
R.G.W. is supported by NIH R01-DK058123 and by a grant from the Fred and Suzanne Biesecker Pediatric Liver Center. L.E.G. is supported by the Penn Institute for Regenerative Medicine and NIH Center Grant P-30-DK050306.
Abbreviations
DDC | 3,5-diethoxycarbonyl-1,4-dihydrocollidine |
ECM | extracellular matrix |
GFAP | glial fibrillary acidic protein |
α-SMA | α-smooth muscle actin |
Footnotes
Publisher's Disclaimer: This is a PDF file of an unedited manuscript that has been accepted for publication. As a service to our customers we are providing this early version of the manuscript. The manuscript will undergo copyediting, typesetting, and review of the resulting proof before it is published in its final citable form. Please note that during the production process errors may be discovered which could affect the content, and all legal disclaimers that apply to the journal pertain.
References
- Alison M, Golding M, Lalani el N, Sarraf C. Wound healing in the liver with particular reference to stem cells. Philos Trans R Soc Lond B Biol Sci. 1998;353:877–894. [Europe PMC free article] [Abstract] [Google Scholar]
- Apte U, Thompson MD, Cui S, Liu B, Cieply B, Monga SP. Wnt/beta-catenin signaling mediates oval cell response in rodents. Hepatology. 2008;47:288–295. [Abstract] [Google Scholar]
- Asahina K, Tsai SY, Li P, Ishii M, Maxson RE, Jr., Sucov HM, Tsukamoto H. Mesenchymal origin of hepatic stellate cells, submesothelial cells, and perivascular mesenchymal cells during mouse liver development. Hepatology. 2009;49:998–1011. [Europe PMC free article] [Abstract] [Google Scholar]
- Baba S, Fujii H, Hirose T, Yasuchika K, Azuma H, Hoppo T, Naito M, Machimoto T, Ikai I. Commitment of bone marrow cells to hepatic stellate cells in mouse. J Hepatol. 2004;40:255–260. [Abstract] [Google Scholar]
- Beaussier M, Wendum D, Schiffer E, Dumont S, Rey C, Lienhart A, Housset C. Prominent contribution of portal mesenchymal cells to liver fibrosis in ischemic and obstructive cholestatic injuries. Lab Invest. 2007;87:292–303. [Abstract] [Google Scholar]
- Bird TG, Lorenzini S, Forbes SJ. Activation of stem cells in hepatic diseases. Cell Tissue Res. 2008;331:283–300. [Europe PMC free article] [Abstract] [Google Scholar]
- Bisgaard HC, Holmskov U, Santoni-Rugiu E, Nagy P, Nielsen O, Ott P, Hage E, Dalhoff K, Rasmussen LJ, Tygstrup N. Heterogeneity of ductular reactions in adult rat and human liver revealed by novel expression of deleted in malignant brain tumor 1. Am J Pathol. 2002;161:1187–1198. [Europe PMC free article] [Abstract] [Google Scholar]
- Braun KM, Thompson AW, Sandgren EP. Hepatic microenvironment affects oval cell localization in albumin-urokinase-type plasminogen activator transgenic mice. Am J Pathol. 2003;162:195–202. [Europe PMC free article] [Abstract] [Google Scholar]
- Cassiman D, Roskams T. Beauty is in the eye of the beholder: emerging concepts and pitfalls in hepatic stellate cell research. J Hepatol. 2002;37:527–535. [Abstract] [Google Scholar]
- Cheng JH, She H, Han YP, Wang J, Xiong S, Asahina K, Tsukamoto H. Wnt antagonism inhibits hepatic stellate cell activation and liver fibrosis. Am J Physiol Gastrointest Liver Physiol. 2008;294:G39–49. [Abstract] [Google Scholar]
- Chiba T, Zheng YW, Kita K, Yokosuka O, Saisho H, Onodera M, Miyoshi H, Nakano M, Zen Y, Nakanuma Y, Nakauchi H, Iwama A, Taniguchi H. Enhanced self-renewal capability in hepatic stem/progenitor cells drives cancer initiation. Gastroenterology. 2007;133:937–950. [Abstract] [Google Scholar]
- Clouston AD, Powell EE, Walsh MJ, Richardson MM, Demetris AJ, Jonsson JR. Fibrosis correlates with a ductular reaction in hepatitis C: roles of impaired replication, progenitor cells and steatosis. Hepatology. 2005;41:809–818. [Abstract] [Google Scholar]
- Dalgetty DM, Medine CN, Iredale JP, Hay DC. Progress and future challenges in stem cell-derived liver technologies. Am J Physiol Gastrointest Liver Physiol. 2009;297:G241–248. [Abstract] [Google Scholar]
- Deng X, Chen YX, Zhang X, Zhang JP, Yin C, Yue HY, Lin Y, Han ZG, Xie WF. Hepatic stellate cells modulate the differentiation of bone marrow mesenchymal stem cells into hepatocyte-like cells. J Cell Physiol. 2008;217:138–144. [Abstract] [Google Scholar]
- Dezso K, Jelnes P, Laszlo V, Baghy K, Bodor C, Paku S, Tygstrup N, Bisgaard HC, Nagy P. Thy-1 is expressed in hepatic myofibroblasts and not oval cells in stem cell-mediated liver regeneration. Am J Pathol. 2007;171:1529–1537. [Europe PMC free article] [Abstract] [Google Scholar]
- Dudas J, Mansuroglu T, Batusic D, Saile B, Ramadori G. Thy-1 is an in vivo and in vitro marker of liver myofibroblasts. Cell Tissue Res. 2007;329:503–514. [Abstract] [Google Scholar]
- Duncan AW, Dorrell C, Grompe M. Stem cells and liver regeneration. Gastroenterology. 2009;137:466–481. [Europe PMC free article] [Abstract] [Google Scholar]
- Fabris L, Cadamuro M, Guido M, Spirli C, Fiorotto R, Colledan M, Torre G, Alberti D, Sonzogni A, Okolicsanyi L, Strazzabosco M. Analysis of liver repair mechanisms in Alagille syndrome and biliary atresia reveals a role for notch signaling. Am J Pathol. 2007;171:641–653. [Europe PMC free article] [Abstract] [Google Scholar]
- Fausto N. Liver regeneration and repair: hepatocytes, progenitor cells, and stem cells. Hepatology. 2004;39:1477–1487. [Abstract] [Google Scholar]
- Friedman SL. Hepatic stellate cells: protean, multifunctional, and enigmatic cells of the liver. Physiol Rev. 2008;88:125–172. [Europe PMC free article] [Abstract] [Google Scholar]
- Fuchs E, Tumbar T, Guasch G. Socializing with the neighbors: stem cells and their niche. Cell. 2004;116:769–778. [Abstract] [Google Scholar]
- Geisler F, Nagl F, Mazur PK, Lee M, Zimber-Strobl U, Strobl LJ, Radtke F, Schmid RM, Siveke JT. Liver-specific inactivation of Notch2, but not Notch1, compromises intrahepatic bile duct development in mice. Hepatology. 2008;48:607–616. [Abstract] [Google Scholar]
- Guyot C, Lepreux S, Combe C, Doudnikoff E, Bioulac-Sage P, Balabaud C, Desmouliere A. Hepatic fibrosis and cirrhosis: the (myo)fibroblastic cell subpopulations involved. Int J Biochem Cell Biol. 2006;38:135–151. [Abstract] [Google Scholar]
- Higashiyama R, Moro T, Nakao S, Mikami K, Fukumitsu H, Ueda Y, Ikeda K, Adachi E, Bou-Gharios G, Okazaki I, Inagaki Y. Negligible Contribution of Bone Marrow-Derived Cells to Collagen Production During Hepatic Fibrogenesis in Mice. Gastroenterology. 2009 [Abstract] [Google Scholar]
- Hinz B, Phan SH, Thannickal VJ, Galli A, Bochaton-Piallat ML, Gabbiani G. The myofibroblast: one function, multiple origins. Am J Pathol. 2007;170:1807–1816. [Europe PMC free article] [Abstract] [Google Scholar]
- Holt AP, Salmon M, Buckley CD, Adams DH. Immune interactions in hepatic fibrosis. Clin Liver Dis. 2008;12:861–882. x. [Europe PMC free article] [Abstract] [Google Scholar]
- Hoppo T, Fujii H, Hirose T, Yasuchika K, Azuma H, Baba S, Naito M, Machimoto T, Ikai I. Thy1-positive mesenchymal cells promote the maturation of CD49f-positive hepatic progenitor cells in the mouse fetal liver. Hepatology. 2004;39:1362–1370. [Abstract] [Google Scholar]
- Houlihan DD, Newsome PN. Critical review of clinical trials of bone marrow stem cells in liver disease. Gastroenterology. 2008;135:438–450. [Abstract] [Google Scholar]
- Kalinichenko VV, Bhattacharyya D, Zhou Y, Gusarova GA, Kim W, Shin B, Costa RH. Foxf1 +/− mice exhibit defective stellate cell activation and abnormal liver regeneration following CCl4 injury. Hepatology. 2003;37:107–117. [Abstract] [Google Scholar]
- Kallis YN, Forbes SJ. The bone marrow and liver fibrosis: friend or foe? Gastroenterology. 2009 in press. [Abstract] [Google Scholar]
- Kamiya A, Kakinuma S, Yamazaki Y, Nakauchi H. Enrichment and Clonal Culture of Progenitor Cells During Mouse Postnatal Liver Development. Gastroenterology. 2009a [Abstract] [Google Scholar]
- Kamiya A, Kakinuma S, Yamazaki Y, Nakauchi H. Enrichment and clonal culture of progenitor cells during mouse postnatal liver development in mice. Gastroenterology. 2009b;137:1114–1126. e1111–1114. 1126. [Abstract] [Google Scholar]
- Katoonizadeh A, Nevens F, Verslype C, Pirenne J, Roskams T. Liver regeneration in acute severe liver impairment: a clinicopathological correlation study. Liver Int. 2006;26:1225–1233. [Abstract] [Google Scholar]
- Kisseleva T, Uchinami H, Feirt N, Quintana-Bustamante O, Segovia JC, Schwabe RF, Brenner DA. Bone marrow-derived fibrocytes participate in pathogenesis of liver fibrosis. J Hepatol. 2006;45:429–438. [Abstract] [Google Scholar]
- Knight B, Akhurst B, Matthews VB, Ruddell RG, Ramm GA, Abraham LJ, Olynyk JK, Yeoh GC. Attenuated liver progenitor (oval) cell and fibrogenic responses to the choline deficient, ethionine supplemented diet in the BALB/c inbred strain of mice. J Hepatol. 2007a;46:134–141. [Abstract] [Google Scholar]
- Knight B, Lim R, Yeoh GC, Olynyk JK. Interferon-gamma exacerbates liver damage, the hepatic progenitor cell response and fibrosis in a mouse model of chronic liver injury. J Hepatol. 2007b;47:826–833. [Abstract] [Google Scholar]
- Koenig S, Probst I, Becker H, Krause P. Zonal hierarchy of differentiation markers and nestin expression during oval cell mediated rat liver regeneration. Histochem Cell Biol. 2006;126:723–734. [Abstract] [Google Scholar]
- Kordes C, Sawitza I, Haussinger D. Hepatic and pancreatic stellate cells in focus. Biol Chem. 2009 [Abstract] [Google Scholar]
- Kordes C, Sawitza I, Muller-Marbach A, Ale-Agha N, Keitel V, Klonowski-Stumpe H, Haussinger D. CD133+ hepatic stellate cells are progenitor cells. Biochem Biophys Res Commun. 2007;352:410–417. [Abstract] [Google Scholar]
- Kuwahara R, Kofman AV, Landis CS, Swenson ES, Barendswaard E, Theise ND. The hepatic stem cell niche: identification by label-retaining cell assay. Hepatology. 2008;47:1994–2002. [Europe PMC free article] [Abstract] [Google Scholar]
- Li Z, Dranoff JA, Chan EP, Uemura M, Sevigny J, Wells RG. Transforming growth factor-beta and substrate stiffness regulate portal fibroblast activation in culture. Hepatology. 2007;46:1246–1256. [Abstract] [Google Scholar]
- Libbrecht L, Desmet V, Van Damme B, Roskams T. Deep intralobular extension of human hepatic ‘progenitor cells’ correlates with parenchymal inflammation in chronic viral hepatitis: can ‘progenitor cells’ migrate? J Pathol. 2000;192:373–378. [Abstract] [Google Scholar]
- Lim YS, Lee HC, Lee HS. Switch of cadherin expression from E- to N-type during the activation of rat hepatic stellate cells. Histochem Cell Biol. 2007;127:149–160. [Abstract] [Google Scholar]
- Lin N, Tang Z, Deng M, Zhong Y, Lin J, Yang X, Xiang P, Xu R. Hedgehog-mediated paracrine interaction between hepatic stellate cells and marrow-derived mesenchymal stem cells. Biochem Biophys Res Commun. 2008;372:260–265. [Abstract] [Google Scholar]
- Loo CK, Wu XJ. Origin of stellate cells from submesothelial cells in a developing human liver. Liver Int. 2008;28:1437–1445. [Abstract] [Google Scholar]
- Loomes KM, Russo P, Ryan M, Nelson A, Underkoffler L, Glover C, Fu H, Gridley T, Kaestner KH, Oakey RJ. Bile duct proliferation in liver-specific Jag1 conditional knockout mice: effects of gene dosage. Hepatology. 2007;45:323–330. [Abstract] [Google Scholar]
- Lowes KN, Brennan BA, Yeoh GC, Olynyk JK. Oval cell numbers in human chronic liver diseases are directly related to disease severity. Am J Pathol. 1999;154:537–541. [Europe PMC free article] [Abstract] [Google Scholar]
- Lozier J, McCright B, Gridley T. Notch signaling regulates bile duct morphogenesis in mice. PLoS One. 2008;3:e1851. [Europe PMC free article] [Abstract] [Google Scholar]
- Michalopoulos GK. Liver regeneration: Alternative epithelial pathways. Int J Biochem Cell Biol. 2009 [Europe PMC free article] [Abstract] [Google Scholar]
- Mishra L, Banker T, Murray J, Byers S, Thenappan A, He AR, Shetty K, Johnson L, Reddy EP. Liver stem cells and hepatocellular carcinoma. Hepatology. 2009;49:318–329. [Europe PMC free article] [Abstract] [Google Scholar]
- Miyata E, Masuya M, Yoshida S, Nakamura S, Kato K, Sugimoto Y, Shibasaki T, Yamamura K, Ohishi K, Nishii K, Ishikawa F, Shiku H, Katayama N. Hematopoietic origin of hepatic stellate cells in the adult liver. Blood. 2008;111:2427–2435. [Abstract] [Google Scholar]
- Morrison SJ, Spradling AC. Stem cells and niches: mechanisms that promote stem cell maintenance throughout life. Cell. 2008;132:598–611. [Europe PMC free article] [Abstract] [Google Scholar]
- Myung SJ, Yoon JH, Gwak GY, Kim W, Lee JH, Kim KM, Shin CS, Jang JJ, Lee SH, Lee SM, Lee HS. Wnt signaling enhances the activation and survival of human hepatic stellate cells. FEBS Lett. 2007;581:2954–2958. [Abstract] [Google Scholar]
- Nagai H, Terada K, Watanabe G, Ueno Y, Aiba N, Shibuya T, Kawagoe M, Kameda T, Sato M, Senoo H, Sugiyama T. Differentiation of liver epithelial (stem-like) cells into hepatocytes induced by coculture with hepatic stellate cells. Biochem Biophys Res Commun. 2002;293:1420–1425. [Abstract] [Google Scholar]
- Nguyen LN, Furuya MH, Wolfraim LA, Nguyen AP, Holdren MS, Campbell JS, Knight B, Yeoh GC, Fausto N, Parks WT. Transforming growth factor-beta differentially regulates oval cell and hepatocyte proliferation. Hepatology. 2007;45:31–41. [Abstract] [Google Scholar]
- Nusse R. Wnt signaling and stem cell control. Cell Res. 2008;18:523–527. [Abstract] [Google Scholar]
- Oertel M, Shafritz DA. Stem cells, cell transplantation and liver repopulation. Biochim Biophys Acta. 2008;1782:61–74. [Europe PMC free article] [Abstract] [Google Scholar]
- Omenetti A, Diehl AM. The adventures of sonic hedgehog in development and repair. II. Sonic hedgehog and liver development, inflammation, and cancer. Am J Physiol Gastrointest Liver Physiol. 2008;294:G595–598. [Abstract] [Google Scholar]
- Omenetti A, Porrello A, Jung Y, Yang L, Popov Y, Choi SS, Witek RP, Alpini G, Venter J, Vandongen HM, Syn WK, Baroni GS, Benedetti A, Schuppan D, Diehl AM. Hedgehog signaling regulates epithelial-mesenchymal transition during biliary fibrosis in rodents and humans. J Clin Invest. 2008;118:3331–3342. [Europe PMC free article] [Abstract] [Google Scholar]
- Omenetti A, Yang L, Li YX, McCall SJ, Jung Y, Sicklick JK, Huang J, Choi S, Suzuki A, Diehl AM. Hedgehog-mediated mesenchymal-epithelial interactions modulate hepatic response to bile duct ligation. Lab Invest. 2007;87:499–514. [Abstract] [Google Scholar]
- Overturf K, Al-Dhalimy M, Finegold M, Grompe M. The repopulation potential of hepatocyte populations differing in size and prior mitotic expansion. Am J Pathol. 1999;155:2135–2143. [Europe PMC free article] [Abstract] [Google Scholar]
- Paku S, Schnur J, Nagy P, Thorgeirsson SS. Origin and structural evolution of the early proliferating oval cells in rat liver. Am J Pathol. 2001;158:1313–1323. [Europe PMC free article] [Abstract] [Google Scholar]
- Parekkadan B, van Poll D, Megeed Z, Kobayashi N, Tilles AW, Berthiaume F, Yarmush ML. Immunomodulation of activated hepatic stellate cells by mesenchymal stem cells. Biochem Biophys Res Commun. 2007;363:247–252. [Europe PMC free article] [Abstract] [Google Scholar]
- Petersen B, Shupe T. Location is everything: the liver stem cell niche. Hepatology. 2008;47:1810–1812. [Abstract] [Google Scholar]
- Petersen BE, Goff JP, Greenberger JS, Michalopoulos GK. Hepatic oval cells express the hematopoietic stem cell marker Thy-1 in the rat. Hepatology. 1998;27:433–445. [Abstract] [Google Scholar]
- Richardson MM, Jonsson JR, Powell EE, Brunt EM, Neuschwander-Tetri BA, Bhathal PS, Dixon JB, Weltman MD, Tilg H, Moschen AR, Purdie DM, Demetris AJ, Clouston AD. Progressive fibrosis in nonalcoholic steatohepatitis: association with altered regeneration and a ductular reaction. Gastroenterology. 2007;133:80–90. [Abstract] [Google Scholar]
- Roskams T. Relationships among stellate cell activation, progenitor cells, and hepatic regeneration. Clin Liver Dis. 2008;12:853–860. ix. [Abstract] [Google Scholar]
- Roskams T, Yang SQ, Koteish A, Durnez A, DeVos R, Huang X, Achten R, Verslype C, Diehl AM. Oxidative stress and oval cell accumulation in mice and humans with alcoholic and nonalcoholic fatty liver disease. Am J Pathol. 2003;163:1301–1311. [Europe PMC free article] [Abstract] [Google Scholar]
- Ruddell RG, Knight B, Tirnitz-Parker JE, Akhurst B, Summerville L, Subramaniam VN, Olynyk JK, Ramm GA. Lymphotoxin-beta receptor signaling regulates hepatic stellate cell function and wound healing in a murine model of chronic liver injury. Hepatology. 2009;49:227–239. [Abstract] [Google Scholar]
- Russo FP, Alison MR, Bigger BW, Amofah E, Florou A, Amin F, Bou-Gharios G, Jeffery R, Iredale JP, Forbes SJ. The bone marrow functionally contributes to liver fibrosis. Gastroenterology. 2006;130:1807–1821. [Abstract] [Google Scholar]
- Ryan MJ, Bales C, Nelson A, Gonzalez DM, Underkoffler L, Segalov M, Wilson-Rawls J, Cole SE, Moran JL, Russo P, Spinner NB, Kusumi K, Loomes KM. Bile duct proliferation in Jag1/fringe heterozygous mice identifies candidate modifiers of the Alagille syndrome hepatic phenotype. Hepatology. 2008;48:1989–1997. [Abstract] [Google Scholar]
- Sackett SD, Li Z, Hurtt R, Gao Y, Wells RG, Brondell K, Kaestner KH, Greenbaum LE. Foxl1 is a marker of bipotential hepatic progenitor cells in mice. Hepatology. 2009;49:920–929. [Europe PMC free article] [Abstract] [Google Scholar]
- Santoni-Rugiu E, Jelnes P, Thorgeirsson SS, Bisgaard HC. Progenitor cells in liver regeneration: molecular responses controlling their activation and expansion. APMIS. 2005;113:876–902. [Abstract] [Google Scholar]
- Sawitza I, Kordes C, Reister S, Haussinger D. The niche of stellate cells within rat liver. Hepatology. 2009 in press. [Abstract] [Google Scholar]
- Sell S, Leffert HL. Liver cancer stem cells. J Clin Oncol. 2008;26:2800–2805. [Europe PMC free article] [Abstract] [Google Scholar]
- Sicklick JK, Choi SS, Bustamante M, McCall SJ, Perez EH, Huang J, Li YX, Rojkind M, Diehl AM. Evidence for epithelial-mesenchymal transitions in adult liver cells. Am J Physiol Gastrointest Liver Physiol. 2006;291:G575–583. [Abstract] [Google Scholar]
- Strick-Marchand H, Masse GX, Weiss MC, Di Santo JP. Lymphocytes support oval cell-dependent liver regeneration. J Immunol. 2008;181:2764–2771. [Abstract] [Google Scholar]
- Suskind DL, Muench MO. Searching for common stem cells of the hepatic and hematopoietic systems in the human fetal liver: CD34+ cytokeratin 7/8+ cells express markers for stellate cells. J Hepatol. 2004;40:261–268. [Abstract] [Google Scholar]
- Suzuki K, Tanaka M, Watanabe N, Saito S, Nonaka H, Miyajima A. p75 Neurotrophin receptor is a marker for precursors of stellate cells and portal fibroblasts in mouse fetal liver. Gastroenterology. 2008;135:270–281. e273. [Abstract] [Google Scholar]
- Tan J, Hytiroglou P, Wieczorek R, Park YN, Thung SN, Arias B, Theise ND. Immunohistochemical evidence for hepatic progenitor cells in liver diseases. Liver. 2002;22:365–373. [Abstract] [Google Scholar]
- Thorgeirsson SS. Hepatic stem cells in liver regeneration. FASEB J. 1996;10:1249–1256. [Abstract] [Google Scholar]
- Tuchweber B, Desmouliere A, Bochaton-Piallat ML, Rubbia-Brandt L, Gabbiani G. Proliferation and phenotypic modulation of portal fibroblasts in the early stages of cholestatic fibrosis in the rat. Lab Invest. 1996;74:265–278. [Abstract] [Google Scholar]
- Van Hul NK, Abarca-Quinones J, Sempoux C, Horsmans Y, Leclercq IA. Relation between liver progenitor cell expansion and extracellular matrix deposition in a CDE-induced murine model of chronic liver injury. Hepatology. 2009;49:1625–1635. [Abstract] [Google Scholar]
- Villeneuve J, Pelluard-Nehme F, Combe C, Carles D, Chaponnier C, Ripoche J, Balabaud C, Bioulac-Sage P, Lepreux S. Immunohistochemical study of the phenotypic change of the mesenchymal cells during portal tract maturation in normal and fibrous (ductal plate malformation) fetal liver. Comp Hepatol. 2009;8:5. [Europe PMC free article] [Abstract] [Google Scholar]
- Yang L, Jung Y, Omenetti A, Witek RP, Choi S, Vandongen HM, Huang J, Alpini GD, Diehl AM. Fate-mapping evidence that hepatic stellate cells are epithelial progenitors in adult mouse livers. Stem Cells. 2008;26:2104–2113. [Europe PMC free article] [Abstract] [Google Scholar]
- Yovchev MI, Grozdanov PN, Zhou H, Racherla H, Guha C, Dabeva MD. Identification of adult hepatic progenitor cells capable of repopulating injured rat liver. Hepatology. 2008;47:636–647. [Abstract] [Google Scholar]
- Yovchev MI, Zhang J, Neufeld DS, Grozdanov PN, Dabeva MD. Thymus cell antigen-1-expressing cells in the oval cell compartment. Hepatology. 2009;50:601–611. [Abstract] [Google Scholar]
- Zeng G, Awan F, Otruba W, Muller P, Apte U, Tan X, Gandhi C, Demetris AJ, Monga SP. Wnt’er in liver: expression of Wnt and frizzled genes in mouse. Hepatology. 2007;45:195–204. [Abstract] [Google Scholar]
- Zhang L, Theise N, Chua M, Reid LM. The stem cell niche of human livers: symmetry between development and regeneration. Hepatology. 2008;48:1598–1607. [Abstract] [Google Scholar]
- Zhang W, Chen XP, Zhang WG, Zhang F, Xiang S, Dong HH, Zhang L. Hepatic non-parenchymal cells and extracellular matrix participate in oval cell-mediated liver regeneration. World J Gastroenterol. 2009;15:552–560. [Europe PMC free article] [Abstract] [Google Scholar]
- Zhou H, Rogler LE, Teperman L, Morgan G, Rogler CE. Identification of hepatocytic and bile ductular cell lineages and candidate stem cells in bipolar ductular reactions in cirrhotic human liver. Hepatology. 2007;45:716–724. [Abstract] [Google Scholar]
- Zong Y, Panikkar A, Xu J, Antoniou A, Raynaud P, Lemaigre F, Stanger BZ. Notch signaling controls liver development by regulating biliary differentiation. Development. 2009;136:1727–1739. [Europe PMC free article] [Abstract] [Google Scholar]
Full text links
Read article at publisher's site: https://doi.org/10.1016/j.biocel.2009.11.006
Read article for free, from open access legal sources, via Unpaywall:
https://europepmc.org/articles/pmc2888761?pdf=render
Citations & impact
Impact metrics
Citations of article over time
Article citations
Spatial molecular and cellular determinants of STAT3 activation in liver fibrosis progression in non-alcoholic fatty liver disease.
JHEP Rep, 5(2):100628, 22 Nov 2022
Cited by: 12 articles | PMID: 36687470 | PMCID: PMC9850198
Chondroitin Sulfate Protects the Liver in an Experimental Model of Extra-Hepatic Cholestasis Induced by Common Bile Duct Ligation.
Molecules, 27(3):654, 20 Jan 2022
Cited by: 2 articles | PMID: 35163920 | PMCID: PMC8839946
Indole-3-Carboxaldehyde Restores Gut Mucosal Integrity and Protects from Liver Fibrosis in Murine Sclerosing Cholangitis.
Cells, 10(7):1622, 29 Jun 2021
Cited by: 17 articles | PMID: 34209524 | PMCID: PMC8305598
Age-Related Features of the Response of the Liver and Stem Cells during Modeling of Liver Cirrhosis.
Bull Exp Biol Med, 171(1):127-133, 28 May 2021
Cited by: 1 article | PMID: 34046793
Dual Pharmacological Targeting of HDACs and PDE5 Inhibits Liver Disease Progression in a Mouse Model of Biliary Inflammation and Fibrosis.
Cancers (Basel), 12(12):E3748, 13 Dec 2020
Cited by: 6 articles | PMID: 33322158 | PMCID: PMC7763137
Go to all (41) article citations
Similar Articles
To arrive at the top five similar articles we use a word-weighted algorithm to compare words from the Title and Abstract of each citation.
Beyond fibrosis: stellate cells as liver stem cells.
Z Gastroenterol, 53(12):1425-1431, 14 Dec 2015
Cited by: 5 articles | PMID: 26666280
Review
The characteristics of activated portal fibroblasts/myofibroblasts in liver fibrosis.
Differentiation, 92(3):84-92, 31 Aug 2016
Cited by: 68 articles | PMID: 27591095 | PMCID: PMC5079826
Review Free full text in Europe PMC
Characterisation of a stereotypical cellular and extracellular adult liver progenitor cell niche in rodents and diseased human liver.
Gut, 59(5):645-654, 01 May 2010
Cited by: 114 articles | PMID: 20427399 | PMCID: PMC3034133
The diversity and plasticity of adult hepatic progenitor cells and their niche.
Liver Int, 37(9):1260-1271, 23 Feb 2017
Cited by: 26 articles | PMID: 28135758 | PMCID: PMC5534384
Review Free full text in Europe PMC
Funding
Funders who supported this work.
NIDDK NIH HHS (5)
Grant ID: R01 DK058123-09
Grant ID: R01 DK058123
Grant ID: P30 DK050306
Grant ID: R01-DK058123
Grant ID: P-30-DK050306