Abstract
Free full text

THE CELL CYCLE AND MYC INTERSECT WITH MECHANISMS FOR PLURIPOTENCY AND REPROGRAMMING
SUMMARY
Pluripotent stem cells have long-term proliferative capacity, an unusual mode of cell cycle regulation and can divide independently of extrinsic mitogenic signals. The last few years has seen evidence emerge that links cell cycle regulation to the maintenance and establishment of pluripotency. Myc transcription factors appear to be central to this regulation. This review addresses these links and discusses how cell cycle controls and Myc impact on the maintenance and establishment of pluripotent cells.
Pluripotent cells in early embryos: cell cycle control and rapid cell division
Uncommitted, pluripotent cells in vertebrate pre-gastrula embryos undergo extraordinarily rapid rounds of cell division. This is associated with cell cycles consisting of alternating rounds of DNA replication (S-phase) and chromosome segregation-cell separation (M-phase) without intervening gap phases, such as in cleavage-stage Xenopus embryos (Murray and Kirschner, 1989). Since all the maternal determinants for development are deposited in the egg prior to fertilization, zygotic transcription is not required until the mid-blastula transition when uncommitted cells become specified. The nature of this cell cycle structure thus promotes the rapid amplification in cell number necessary for early embryonic development. Variations of this general theme also apply during early Drosophila and zebrafish development to enable rapid expansion in cell number just prior to gastrulation (Edgar and Lehner, 1996; Yarden and Geiger, 1996).
In rodents, peri-implantation development is dependent on zygotic programs and so cell cycle progression is driven by de novo RNA and protein synthesis. Expansion of the rodent embryo immediately prior to gastrulation is dependent on a rapid burst of pluripotent cell proliferation, with average generation times estimated to be ~4.5–8.0 hours (Snow, 1977; Lawson et al., 1991). These cells exhibit an unusual cell cycle structure, characterized by a short G1 gap phase and a high proportion of S-phase cells (~65%). Since G1 is the period of the cell cycle when cell growth primarily occurs, this has often been used to rationalize why pluripotent cells are small in size. As pluripotent cells become specified into one of the three germ layers, the cell cycle typically restructures so that the length of G1 is extended, leading to increased division times and a general increase in cell volume (Lawson et al., 1991). These observations raise the question as to whether there is some mechanistic relationship between pluripotency, cell cycle structure and cell size control.
A cell cycle structure synonymous with pluripotency
Cell cycle regulation of pluripotent cells has been extensively characterized in embryonic stem cells (ESCs), first with mouse (see White and Dalton, 2005) and more recently with rhesus monkey (Fluckiger et al., 2006) and human (Neganova et al., 2009; Zhang et al., 2009) lines. In general, pluripotent ESCs retain the cell cycle dynamics of their counterparts in the peri-implantation stage embryo (Snow, 1977; Lawson et al., 1991; Stead et al., 2002). Murine ESCs divide rapidly with generation times of ~8–10 hours and spend ~65% of their time in S-phase and ~15% of their time in G1. Upon differentiation, the cell cycle is remodeled such that the percentage of cells in G1 increases to over 40% and generation times increase to >16 hours (Stead et al., 2002; White et al., 2005). These features of cell cycle control appear to be conserved in rhesus monkey ESCs (Fluckiger et al., 2006), murine epiblast stem cells (EpiSCs; Tesar et al. 2007; Brons et al., 2007), human and murine induced pluripotent stem cells (iPSCs), and hESCs (Figure 1; Ohtsuka and Dalton, 2008; Dalton, 2009). Although hESCs and human iPSCs exhibit a cell cycle structure similar to the other pluripotent cell types discussed so far, their cell division cycle times are significantly slower (~32–38 hours; Ohtsuka and Dalton, 2008; Dalton, 2009) indicating that rapid division is not a necessary property of pluripotent cells. This is supported by other observations showing that slowing the mESC cell cycle with chemical inhibitors has no measurable impact on self-renewal or differentiation potential (Stead et al., 2002). We are unaware of any accurate estimates of generation times for human pluripotent cells in early development and so it is difficult to evaluate if these differences reflect species-species variations or, are due to technical issues associated with culture conditions. It is generally thought, based on crude population doubling measurments, that hESC proliferation accelerates when cells become aneuploid, presumably due to the acquisition of selective growth advantages (Brimble et al., 2004; Draper et al., 2004; Mitalipova et al., 2005). There are caveats to this however, since differences in apoptotic rates under different conditions can also impact on population expansion. Nevertheless, care must be taken when addressing this and other aspects of pluripotent cell biology. Although iPSCs are considered to be fully reprogrammed, partially reprogrammed cells (PRCs) can also be obtained using established methods for generating iPSCs (Mikkelsen et al., 2008; Silva et al., 2008; Sridharan et al., 2009). These cells self-renew but do not express markers characteristic of pluripotent cells and exhibit a cell cycle profile intermediate between pluripotent cells and adult fibroblasts (Figure 1).
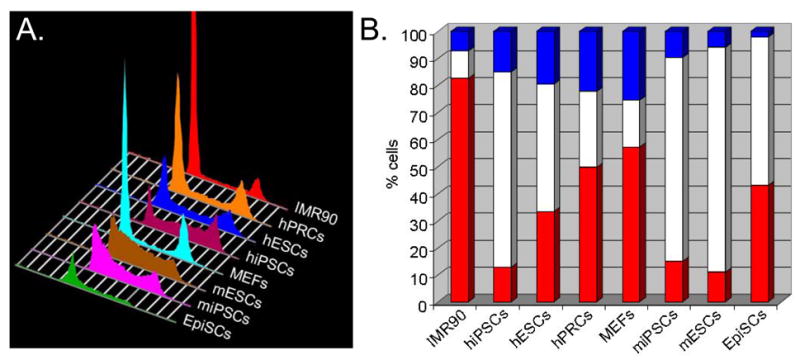
This figure represents typical cell cycle profiles from a range of pluripotent cell types (mESC, hESC, miPSC, hiPSC and mEpiSC) and primary fibroblasts. Pluripotent cells spend a high proportion of time in S-phase cells and a low proportion of time in G1 (Savatier et al., 1996; Stead et al., 2002; Fluckiger et al., 2006; Ohtsuka and Dalton, 2008; Dalton, 2009; Neganova et al., 2009; Zhang et al., 2009). MEFs and human IMR90 fibroblasts show a lower % of cells in S-phase and an increased proportion in G1, relative to pluripotent cells. hPRCs display a cell cycle structure intermediate between differentiated fibroblasts and pluripotent cells. The positions of G1 cells (2n DNA content), S-phase cells and G2/M cells (4n DNA content) are indicated. The Y-axis represents the relative number of cells and the X-axis represents the DNA content of cells, a read-out for cell cycle position.
In total, rapid cell division is not a pre-requisite for the self-renewing, pluripotent state. Instead, we suggest that mechanisms underpinning cell cycle structure are more central to the mechanisms governing the stem cell state. The following discussion will tackle what the relationship between cell cycle, self-renewal and pluripotency may be.
The significance of a cell cycle lacking full gap phases
Pluripotent cells of the epiblast, embryonal carcinoma cells (ECCs), embryonic germ cells (EGCs), ESCs, EpiSCs, early primitive ectoderm-like cells (EPL cells) and iPSCs all exhibit cell cycles consisting of a truncated G1 phase and a high proportion of cells in S-phase (see Figure 1; Savatier et al., 1996; Stead et al., 2002; White et al., 2005). The possible relationship between cell cycle structure, self-renewal and differentiation was first addressed over 20 years ago by Christine Mummery in ECCs. These early studies in ECCs indicate that cells preferentially initiate differentiation from the G1 phase (Mummery et al., 1987; Jonk et al., 1992). The underlying principle behind these studies was that a short G1 limits the ‘window of opportunity’ for which a cell can be responsive to differentiation cues. For example, if differentiation is dependent on the threshold of an induction signal or, if there is a cumulative effect over time, it makes sense that shortening G1 would serve to limit the potential for differentiation. This model predicts that an extended G1 phase would make cells more responsive to differentiations signals and that cell cycle remodeling would be a pre-requisite for efficient differentiation. An extended period of time in S-phase may further shield cells from extrinsic differentiation cues, by reducing the proportion of time in G1, and may also be advantageous to maintain an ‘open’ euchromatic chromatin structure that is characteristic of pluripotent cells (Herrera et al., 1996). This general idea is supported by studies in hESCs demonstrating that inhibition of G1 progression compromises pluripotency (Filipczyk et al., 2007).
Devoting a high proportion of time to S-phase could also facilitate the maintenance of chromatin in a euchromatic state. The highly euchromatic genome of pluripotent cells is likely to facilitate rapid activation (or repression) of genes in response to positional specification cues. This can be thought of as ‘differentiation competency’ and, as argued above, could be regulated by cell cycle control mechanisms. On a similar note, it has been proposed that as transcription factors are displaced from condensing chromatin during mitosis, this could represent an opportunity to reset developmentally important transcriptional programs during the following G1 phase (Egli et al., 2008). The length of G1 would therefore serve as a potential window in which this could occur. If chromatin in pluripotent cells exists in an open configuration, repressing and activating blocks of genes could require a longer remodeling period than available during the short G1 phase of pluripotent cells. This and other scenarios presented by us require that the length of G1 increases coincidentally with differentiation. Technically, this is a very difficult issue to resolve and as of now, has not been addressed in cells outside of ECCs.
Rodent and primate pluripotent cells are generally thought to undergo symmetric divisions, where two self-renewing daughter cells are generated following each mitosis (Zwaka et al., 2005). It is unclear if iPSCs divide by an asymmetric or symmetric mode but, for the purposes of this discussion, we assume they generate two identical daughter cells at mitosis. One issue that receives little attention when pluripotent cells are discussed is their relatively small size (Takahashi and Yamanaka, 2006; Tesar et al., 2007) and how cell volume generally increases during differentiation. Based on other studies, where the length of G1 has been shown to be a major period of cell growth (Saucedo and Edgar, 2002; Jorgensen and Tyers, 2004; Baserga, 2007), it is likely that increased cell size is associated with cell cycle restructuring. More broadly this issue raises questions about how cells reset their size as they transition between different states. These questions underscore the need to characterize in greater detail how cell cycle and cell volume controls are coordinated in pluripotent cells, not just from a stem cell perspective but also from a developmental perspective where size and cell cycle controls are incredibly dynamic at around the time of implantation (Snow, 1977; Lawson et al., 1991).
Regulation of proliferation in self-renewing, pluripotent cells
To appreciate the remarkable features of pluripotent cell cycle control, it is useful to be reminded of the established principles underpinning growth control in mammalian cells of somatic origin. The established view involves the strict requirement for mitogenic signaling in order for a cell to proceed through G1 and into S-phase. In the absence of active mitogenic signaling, cells exit the cycle at some point in G1 and enter a quiescent phase (Go) but, retain the capacity to re-enter the cell cycle upon further mitogenic stimulation. This proliferative ‘gateway’, known as the restriction (R-) point, must be passed through in every cell cycle and mechanistically constitutes a nexus between mitogenic signaling pathways and the cell cycle machinery (Pardee, 1974). Once past this control point, cells become committed to completing the cell cycle and require no further mitogenic signaling. Well-known exceptions to this principle are tumor cells which acquire mutations in one or more of the regulatory genes required for R-point control. Consequently, cells become mitogen independent and cycle in the absence of growth factors (Sherr, 1996).
As most is known about mESCs, we will begin by considering them first. In contrast to a typical somatic cell, signaling through the mitogenic Ras/Mek/Erk pathway does not enhance cell cycle progression of mESCs, instead promoting their differentiation (Burdon et al., 1999), which coincides with a deceleration in progression through the cell cycle (White et al., 2005). Consistent with this, growth factor withdrawal from mESC cultures has no impact on proliferation rates but, eventually contributes to apoptosis (Schratt et al., 2001; E. Stead and S. Dalton, unpublished). Mitogen-independent cell cycle control in mESCs is therefore reminiscent of tumor cells and reiterates the well established connection between immortalization and factor-independent growth control (see Ohtsuka and Dalton, 2008). A caveat to this discussion is that autocrine factors produced by pluripotent cells may be required for their proliferation.
At an early stage during differentiation, mESCs become dependent on extrinsic factors and lose their tumorigenic capacity (Chambers and Smith, 2004; Blum and Benvinisty, 2008), implying some link between tumorigenicity and mitogen-independent proliferation in pluripotent cells (see Ying et al., 2008). The tumorigenic properties of pluripotent cells can be rationalized by the activity of several genes, which in other contexts are involved in tumor progression. The proto-oncogene c-myc for example, plays key roles in pluripotent cell establishment and maintenance (Cartwright et al., 2005). Moreover, an ESC-specific form of Ras (ERas) that drives PI3K is required for robust teratoma formation in transplantation assays and contributes to the rapid cell division of mESCs (Takahashi et al., 2003). A role for PI3K signaling in rapid mESC cell division was implied initially from experiments where inactivation of PTEN accelerated progression through G1 (Sun et al., 1999). Although PI3K is firmly established as a major regulator of ESC self-renewal (see Paling et al., 2004; Takahashi et al. 2005; Watanabe et al., 2006; McLean et al., 2007), how it accelerates G1 progression in pluripotent cells has not been defined. Clues to answer this question come from studies showing that PI3K/mTOR co-ordinates cell cycle progression by coupling it to cell growth control (Alvarez et al., 2003; Fingar et al., 2004; Figure 2). In contrast to pluripotent cells of rodent origin, hESCs lack a functional ERas gene (Kameda and Thomson, 2005), perhaps explaining differences in proliferation rates between rodents and human cell lines. The mitogenic requirements for hESCs are now only starting to be understood and it is unclear if they are totally independent of extrinsic factors. One potential factor that could impact on hESC proliferation, Fgf2, exhibits no mitogenic activity but rather, serves to sustain pluripotency (Filipczyk et al., 2007). Other extrinsic factors that drive PI3K activity, such as Igf and insulin, could perform pro-mitogenic functions, however.
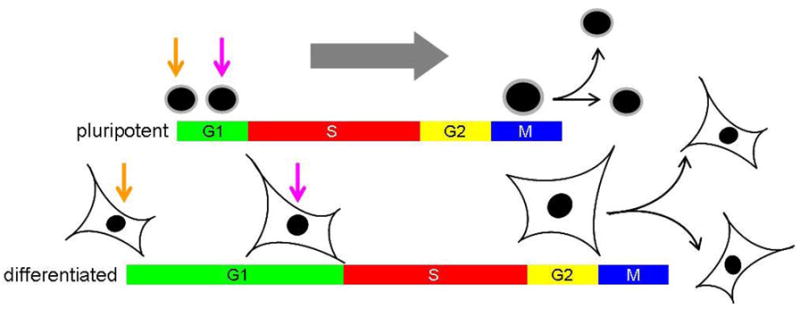
The figure represents a summary of the pathways discussed throughout this review, now thought to be important for cell cycle and cell size control in pluripotent cells. The core transcriptional network inherent to pluripotent stem cells impacts on this network at several points. Evidence links Myc to all major points in the regulatory network. For example, Myc regulates the cell cycle directly through regulation of Cdk activity and indirectly through miRNAs. We speculate that accelerated progress through G1 can account for the small size of pluripoent cells. Interestingly, cells with inactive Rb-family members progress through G1 rapidly and have reduced cell volume.
When the cell cycle of rodent pluripotent cells is examined, several additional regulatory themes appear to be shared with tumor cells. Such analysis indicates that mESCs lack an intact R-point because the retinoblastoma protein (pRb) is held in a constitutively hyper-phosphorylated state (Savatier et al., 1994), E2F transcription factors are free of pRb family members and E2F-dependent transcription displays no periodicity throughout the cell cycle (Stead et al., 2002). These observations can be accounted for by unusually high levels of cyclin dependent kinase 2 (Cdk2)-cyclin E/A, Cdk6-cyclin D3 activity and by the absence of Cdk inhibitory molecules such as p21Cip1, p27Kip1 and INK family members such as p16Ink4a (Stead et al., 2002; Faast et al., 2004). With the exception of Cdk1-cyclin B, all other Cdk activities are constitutively active throughout the cell cycle. This explains why tumor suppressor proteins such as pRb are held in an inactive state and how pluripotent cells have only a brief G1 phase. Many features of mESC cell cycle control and cell growth can in fact be recapitulated in mouse embryo fibroblasts (MEFs) following genetic inactivation of the three pRb family members (pRb, p107, p130). For example, Rb-triple knock-out (TKO) MEFs progress through G1 at an accelerated rate and exhibit a cell cycle profile similar to that of mESCs (Sage et al., 2000). Up to 65% of Rb-TKO MEFS are in S-phase compared to 40% in wild-type cells (Dannenberg et al., 2000). Moreover, Rb-TKO cells are resistant to contact inhibition, they proliferate independently of growth factors, fail to undergo replicative senescence and show a 40–50% decrease in cell volume (Sage et al., 2000; Dannenberg et al., 2000). The decrease in cell size is likely to reflect accelerated progression through G1. All of these characteristics are hallmarks of ESCs and were discussed earlier in this review. Perhaps most intriguing however, Rb-TKO cells show a reduced efficiency for differentiation into multiple lineages (Dannenberg et al., 2000). This suggests that active pRb family members are required for differentiation, perhaps by controlling chromatin remodeling (Macaluso et al., 2006) or by helping to establish a new mode of G1 cell cycle control. The establishment of cell cycle regulated Cdk activity during the early steps of differentiation could provide a mechanism to explain how pRb family members become activated, thereby enabling cells to differentiate efficiently. Inactivation of the Rb family by precocious Cdk activity (Stead et al., 2002; Faast et al., 2004) could therefore be a focal point for regulation of the cell cycle and self-renewal, just as it is in transformed cells.
Rhesus monkey ESCs are similar to mESCs as they also display constitutive cyclin-Cdk activities, proliferate by a mitogen-independent mechanism and cycle rapidly with a short G1 phase (Fluckiger et al., 2006). In contrast, hESCs cycle slowly (~32–38 hours) but retain a cell cycle with a high proportion of S-phase and low percentage of cells in G1 (Neganova et al., 2009). There is also some evidence indicating that Cdk activities display modest periodicity (2–3 fold fluctuations) during the cell cycle in hESCs- a departure from the absence of periodicity in murine and rhesus ESCs (Neganova et al., 2009). This suggests that cyclical Cdk activity may be associated with slower rounds of division and that constitutive activity is related to more rapid division. Following this theme, the cell cycle structure characteristic of pluripotent cells therefore seems to be independent of the pattern of Cdk activity. Instead, rates of division seem to correlate more with its constitutive or cyclic pattern of regulation in the cell cycle. Differences in the magnitude of overall Cdk activities could also be a contributing factor since murine pluripotent cells divide extremely rapidly and have unprecedentedly high global Cdk activity (Stead et al., 2002; Faast et al., 2004).
If Cdk regulation in human pluripotent cells is more reminiscent of that in untransformed somatic cells, it is unlikely that constitutive Cdk activity per se is a requirement for self-renewal and pluripotency. Instead, we are left to contemplate other factors that may underpin self-renewal and pluripotency such as cell cycle structure.
A direct relationship between core regulators of the pluripotent state and the cell cycle has recently been described (see Figure 2). First, involving Oct4 and Sox2 which regulate miR-302, a regulator of cyclin D1, Rb, E2F1 and p130 (Card et al., 2008). A second report, by Zhang and co-workers (2009), implicates Nanog in the regulation of CDK6 and CDC25A, key components of the G1 cell cycle machinery. The over-arching implication of these studies is that an intimate relationship exists between mechanisms of pluripotency and cell cycle regulation. The role of cell cycle regulation in the establishment of pluripotency will be discussed later in the context of reprogramming and iPSC derivation (see below).
Cell cycle remodeling and differentiation: is periodicity important?
As pluripotency is lost during differentiation, cells undergo a wide-range of biological changes. These include, remodeling of the cell cycle, adjustment of cell size controls, loss of tumorigenic capacity, acquisition of contact-inhibited growth and mitogen-dependence. Growth factor dependence has not been evaluated in human pluripotent cells but, murine ESCs transition from being factor-independent to factor-dependent as they differentiate (Schratt et al., 2001; Ying et al., 2008). This is consistent with the imposition of R-point control in G1 during early cell fate commitment (Stead et al., 2002; Faast et al., 2004; White et al., 2005).
Since we know most about mESCs it is easiest to first comment on the above-mentioned aspects of differentiation in the context of rodent cells. One thing that immediately stands out when G1 controls are evaluated is the lack of any apparent growth restraints on the cell cycle machinery. When mESCs differentiate, Cdk activities become cell cycle regulated and decrease in magnitude (White et al., 2005). How cells transition from one mode of Cdk regulation to the other is unclear but, this decline in Cdk activity can be accounted for by the up-regulation of Cdk inhibitory molecules such as p21Cip1, p27Kip1 and members of the INK family. The INK family show specific patterns of up-regulation depending on which lineage is specified (Zindy et al., 1997; Faast et al., 2004), indicating that lineage specific mechanisms of cell cycle regulation operate during the early stages of cell fate commitment. Up-regulation of inhibitory proteins is accompanied by a general decline in cyclin levels, which themselves become cell cycle dependent due to changes in their regulation at the transcriptional and post-transcriptional levels. All of these observations are likely to be interconnected but it is unclear what triggers this series of events. The key regulatory event that transitions cells from being tumor-like to a cell with intact growth controls and cell cycle regulated activities is still not understood.
Perhaps the most relevant points of regulation that can account for dramatic growth control changes, are those associated with establishment of the R-point machinery. This includes the introduction of cell cycle regulated pRb phosphorylation and E2F-dependent transcription. Imposition of G1 growth controls seem to explain the switch from factor-independency to factor-dependency, establishment of contact inhibition and even changes in cell size controls. It is tempting then, to suggest that these changes also underpin mechanistic aspects of cell fate commitment but, it is unclear if these events are related to ‘cause or effect’. We anticipate that rhesus ESCs will undergo similar changes in cell cycle regulation in differentiation to that described for murine cells.
Human pluripotent cells represent a different scenario as at least some G1 controls appear to be operative (Becker et al., 2007; Ghule et al., 2007; Neganova et al., 2009). Nevertheless, hESCs must remodel their cell cycle as they differentiate, so as to increase the proportion of time in G1 and to allow for changes in size control. How this occurs is unclear but, according to earlier work, is likely to be underpinned by changes in Cdk2 activity (Stead et al., 2002). Neganova et al. (2009) recently tested this idea in hESCs and provide evidence which indicates that elevated Cdk2 activity in G1 is required for maintenance of the pluripotent state. These findings are consistent with the earlier report of Filipczyk et al. (2007) showing that normal progression through G1 is required to maintain pluripotency. These are perhaps the most compelling arguments made yet to invoke a role for the cell cycle machinery in maintenance of pluripotency.
Cell cycle control, immortalization and size control: Do all roads lead to Myc?
When thinking about potential regulators of stem cell self-renewal and pluripotent cell cycle control, one possibility immediately comes to mind- the Myc transcription factor family (c-, N- and L-myc). The Myc family of basic helix-loop-helix transcription factors, in particular c- and N-Myc, have well established roles in control of cell cycle progression, cell immortalization and tumor progression (Meyer and Penn, 2008). Moreover, Myc has established roles in blocking cell differentiation and in maintaining progenitor populations in vivo (Knoepfler et al., 2002; Kenney et al., 2004; Satoh et al., 2004; Okubo et al., 2005). Myc has many roles in normal proliferative control and cell fate determination but when its activity is de-regulated, it becomes oncogenic. It should be noted that in some contexts, Myc can promote progenitor differentiation, rather maintain them (Wilson et al., 2004; Watt et al., 2008), and so the Myc family appears to have context-dependent effects on cell fate. The exact function of Myc transcription factors have been difficult to decipher, probably because of their context-dependent functions and because they regulate hundreds, or possibly thousands of genes in vivo (Fernandez et al., 2003). It has been speculated that in contrast to a typical transcription factor, which regulates a limited number of genes through cis-acting regulatory elements, Myc functions as an epigenetic ‘licensing’ factor. In this model, Myc binds to regulatory regions of many genes which together are part of a transcriptional program required for cell fate determination and identity (see Guccione et al., 2006). The complexity of Myc-dependent gene regulation is made greater by the fact that Myc activates and repress target genes through E-box dependent and independent mechanisms (Ryan and Birnie, 1996). We will now review the evidence establishing Myc as being critical for maintenance and establishment of the pluripotent state and as in doing so, emphasizing its potential roles in regulating cell cycle control and self-renewal.
Unlike other transcription factors, such as Oct4, Nanog and Sox2, which have key roles in maintaining the self-renewing pluripotent state, there is no direct evidence linking Myc to the development of pluripotent cells in the peri-implantation stage embryo. The absence of an early developmental phenotype in c-/N-myc knockout mice can be explained by the overlapping expression of these functionally redundant family members (Malynn et al., 2000). Simultaneous inactivation of multiple Myc family members has so far not been reported in early development and will be required before this lingering question can be formally answered. There are precedents however, where c- and N-myc perform redundant roles in stem cell proliferation and survival- a recently described example being in hematopoietic stem cells (Laurenti et al., 2008).
Since a self-renewing stem cell must divide indefinitely and avoid replicative senescence it is not surprising that factors shown to be important for cell transformation, such as Myc, are also involved in self-renewal. The first evidence for this came from Cartwright et al. (2005) where it was shown that ectopic Myc expression could relieve the dependency of mESCs for LIF. More recently, ectopic Myc expression has been shown to promote a metastable pluripotent state (Hanna et al., 2009). A role for Myc in mESC self-renewal is consistent with its dramatic down-regulation during the early stages of differentiation (Cartwright et al., 2005).
There are several lines of evidence to support the idea that elevated Myc activity accounts for many of the cell cycle properties of pluripotent cells. First, elevated c-myc accelerates progression through G1 by positively regulating cyclin-Cdk activity (Amati et al., 1998). This regulation occurs at multiple levels, including transcriptional and post-transcriptional control involving the up-regulation of cyclins and suppression of Cdk inhibitors such as p21Cip1 and p27Kip1. Second, cell cycle changes that occur during the early stages of pluripotent cell differentiation, coincide with the collapse of Myc levels (Cartwright et al., 2005; White et al., 2005). In addition to previous reports in cancer cell models, the regulatory subunit of telomerase (TERT) has been confirmed as an in vivo target of Myc in mESCs (Kim et al., 2008). These observations begin to place Myc at the center of a regulatory network that links self-renewal mechanisms to the cell cycle machinery in pluripotent cells (see Figure 2).
How Myc contributes to the establishment and maintenance of pluripotent cells has been the subject of several recent reports focusing on the identification of in vivo target genes (Kim et al., 2008; Kidder et al., 2008). Cell cycle genes clearly emerge as being regulatory targets, consistent with Myc being the centerpiece for cell cycle regulation in pluripotent cells. These studies still leave many questions unanswered but the key to this may lie with the role of ESC-specific microRNAs (miRNAs) which have recently been shown to co-ordinate the expression of cell cycle control molecules such as p21Cip1 and cyclin D2 (Wang et al., 2008; Lee et al., 2008). These reports establish that when miRNA function is lost, p21 and cyclin D2 become elevated, resulting in loss of pluripotentcy. Judson and co-workers have recently suggested that maintaining the expression of ESC-specific miRNAs may in fact be regulated directly by Myc (Judson et al., 2009; discussed below). Maintaining the balance of cell cycle regulatory molecules therefore appears to be critical for the pluripotent state. Myc appears to be central to this tightly inter-connected mechanism.
The cell cycle and Myc: part of the reprogramming mechanism required for iPSC formation?
As discussed previously, pluripotent cells have a signature cell cycle structure, where cells spend a proportionally short period of time in G1 and a proportionally long period of time in S-phase (see Figure 1). Cell types generally used to generate iPSCs have quite a different cell cycle structure and as reprogramming occurs, a pluripotent cell cycle structure and mechanism of control is established. This cell cycle structure does not appear to be imposed however, in partially reprogrammed cells (PRCs) and so the characteristic cell cycle structure is established during the later stages of reprogramming (Figure 1). It is unknown if re-establishment of pluripotent cell cycle control mechanisms is ‘cause or effect’ with regards to reprogramming but we would argue, based on the evidence to be presented, that it is integrally involved. We will now discuss our current understanding of these issues in an attempt to define the role of cell cycle control mechanisms and Myc in cell reprogramming.
The arguments used previously to invoke a role for cell cycle control in maintenance of the pluripotent state also apply to the maintenance of iPSCs and so this topic will not be covered again. Instead, we will discuss potential mechanisms where the cell cycle and its control by Myc could impact on the establishment of the pluripotent state, such as in iPSC generation (see Knoepfler 2008). The most obvious role for Myc in the context of cell cycle control could be a requirement for the originating cells to be cycling when exposed to reprogramming factors. This scenario has been posed by Maherali et al. (2008) to explain why some cell types reprogram more efficiently and rapidly than others. Keratinocytes for example, reprogram more efficiently than fibroblasts and express higher levels of Myc. Differences in Myc expression levels in different cell types could also explain why exogenous Myc is dispensible for iPSC formation in some cell types. In this scenario, endogenous Myc activity could be sufficient to work in conjunction with other exogenous factors. Neural progenitors for example, do not require exogenous Myc for reprogramming because they express high endogenous levels (Han et al., 2008). The question remaining then is- when and why is Myc required for reprogramming? One argument is that Myc simply maintains cells in a proliferative state in which they respond better to reprogramming factors. In this scenario, Myc and the cell cycle machinery would also halt the onset of replicative senescence. Zhao and Daley (2008) have pointed out that a pre-condition for reprogramming may be the inactivation of senescence factors such as pRb and p53-perhaps by Myc-dependent regulation of Cdk. It has not escaped our attention that this amounts to the establishment of a transformed-like state where tumor suppressors are inactivated and oncogenes activated to generate a quasi-immortal, self-renewing cell. A mechanistic survey of four reprogramming factors (c-myc, Klf4, Oct4 and Sox2) found that c-myc is critical for the early stages of reprogramming (Sridharan et al., 2009). This study suggests that in addition to maintaining cells in a proliferative state, Myc performs a role in silencing genes associated with differentiation and in broadly modifying the epigenetic signature of a cell by enhancing global histone acetylation (see Knoepfler et al., 2006). This effect can be thought of as a ‘priming’ function which enables cells to become receptive to other factors required for the latter stages of reprogramming. This scenario is consistent with a model where Myc controls large blocks of genes involved in cell fate determination. Besides the initial stages of reprogramming, Myc is likely to perform roles at multiple stages of iPSC formation (Figure 3) since it is likely that cells in the process of reprogramming need to be in a proliferative state. This is also suggested from the early work of Cartwright et al. (2005) who initially demonstrated roles for c-myc in pluripotentcy maintenance and also more recently from Hanna and co-workers (2009) who showed that c-myc promotes a metastable pluripotent state. Finally, c-myc controls the expression of ESC-specific miRNAs that can be used as a substitute for Myc in reprogramming of fibroblasts, in conjunction with Oct4, Sox2 and Klf4 (Judson et al., 2009). In total, these data strongly argue that Myc is a critical reprogramming factor that serves multiple functions at different stages of iPSC formation/maintenance. These are likely to involve cell cycle-related and cell cycle-independent functions (Figure 3).
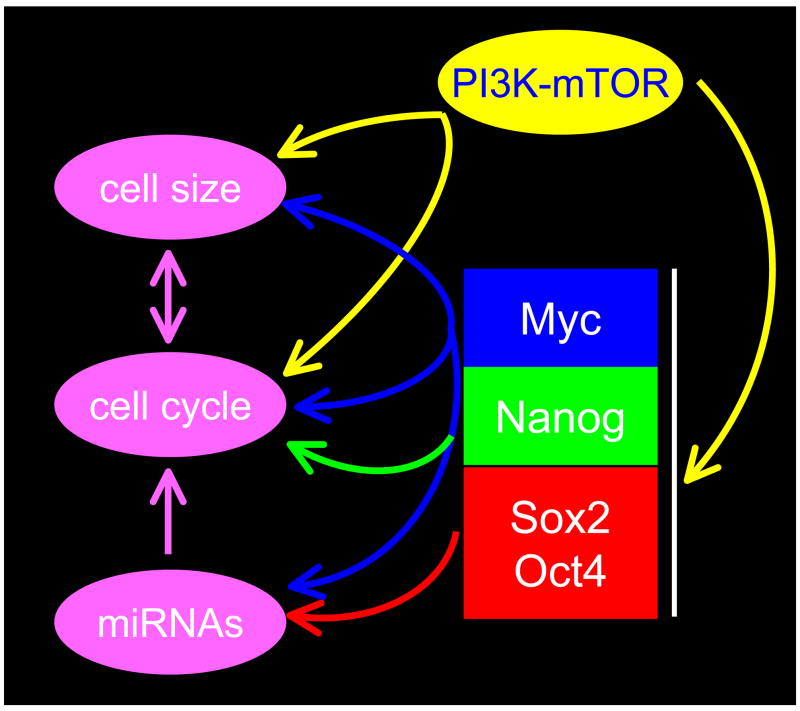
Cells subject to reprogramming cues undergo a three step process as they transition towards iPSCs. The initial step involves Myc which causes global changes in genes expression, including repression of differentiation genes, without induction of pluripotency genes (see Sridharan et al., 2009; Zhao and Daley et al., 2008; Mahereli et al., 2008; Judson et al., 2009; Silva et al., 2008; Mikkelesen et al., 2008). Other reprogramming factors, possibly in combination with Myc (Huangfu et al., 2008), then drive the formation of partially reprogrammed cells (PRCs) and then fully reprogrammed iPSCs. Roles for Myc have also been defined in the maintenance of iPSCs and other pluripotent cell types, some of which are directly related to cell cycle control (Cartwright et al., 2005; Wang et al., 2008; Judson et al., 2009; Hanna et al., 2009). Possible points at which Myc could be involved, but for which there are no solid data, are indicated by broken arrows. For example, it is likely that for reprogramming cells need to be constantly kept cycling by Myc.
Closing comments
This review has discussed the broad relationship between mechanisms of pluripotency, cell cycle control and reprogramming. Figures 2 and and33 shows a summary of the inter-relationships between these pathways and how Myc transcription factors may participate in these processes. The Myc family are well established regulators of G1 progression and can impact on cell size by driving cells into S-phase by mechanisms impacting on Cdk activity. Myc is not the only component of the transcription factor network that plays a role in cell cycle control of pluripotent cells. Sox2 and Oct4 control the cell cycle through miRNAs and Nanog regulates G1 progression at the transcriptional level. Together, these observations provide clear relationships between the fundamentals of pluripotency and the cell division cycle. While the model portrayed here highlights the key components of cell cycle and size control in pluripotent cells, the details of how everything fits together needs to be clarified in greater detail. We are left with many unanswered questions but recent progress, over the last 3 years in particular, bodes well for a more complete understanding in the near future. In total, the connections between cell cycle, pluripotency and reprogramming are somewhat unexpected findings that solidify a unique mode of cell cycle regulation as an integral component of the pluripotent state.
Acknowledgments
This work was supported by grants to SD from the National Institute of Child Health and Human Development (HD049647), the National Institute for General Medical Sciences (GM75334) and the Juvenile Diabetes Research Foundation (JDRF). We thank members of the Dalton laboratory, past and present, for many insightful discussions over the years.
References
- Alvarez B, Garrido E, Garcia-Sanz JA, Carrera AC. Phosphoinositide 3-kinase activation regulates cell division time by coordinated control of cell mass and cell cycle progression rate. J Biol Chem. 2003;278:26466–26473. [Abstract] [Google Scholar]
- Amati B, Alevizopoulos K, Vlach J. Myc and the cell cycle. Front Biosci. 1998;3:d250–268. [Abstract] [Google Scholar]
- Baserga R. Is cell size important? Cell Cycle. 2007;6:814–816. [Abstract] [Google Scholar]
- Becker KA, Ghule PN, Therrien JA, Lian JB, Stein JL, van Wijnen AJ, Stein GS. Self-renewal of human embryonic stem cells is supported by a shortened G1 cell cycle phase. J Cell Physiol. 2006;209:883–893. [Abstract] [Google Scholar]
- Blum B, Benvenisty N. The tumorigenicity of human embryonic stem cells. Adv Cancer Res. 2008;100:133–158. [Abstract] [Google Scholar]
- Brimble SN, Zeng X, Weiler DA, Luo Y, Liu Y, Lyons IG, Freed WJ, Robins AJ, Rao MS, Schulz TC. Karyotypic stability, genotyping, differentiation, feeder-free maintenance, and gene expression sampling in three human embryonic stem cell lines derived prior to August 9, 2001. Stem Cells and Dev. 2004;13:585–596. [Abstract] [Google Scholar]
- Brons IG, Smithers LE, Trotter MW, Rugg-Gunn P, Sun B, Chuva de Sousa Lopes SM, Howlett SK, Clarkson A, Ahrlund-Richter L, Pedersen RA, Vallier L. Derivation of pluripotent epiblast stem cells from mammalian embryos. Nature. 1997;448:191–195. [Abstract] [Google Scholar]
- Burdon T, Stracey C, Chambers I, Nichols J, Smith A. Suppression of SHP-2 and ERK signaling promotes self-renewal of mouse embryonic stem cells. Dev Biol. 1999;210:30–43. [Abstract] [Google Scholar]
- Card DA, Hebbar PB, Li L, Trotter KW, Komatsu Y, Mishina Y, Archer TK. Oct4/Sox2-regulated miR-302 targets cyclin D1 in human embryonic stem cells. Mol Cell Biol. 2008;28:6426–6438. [Europe PMC free article] [Abstract] [Google Scholar]
- Cartwright P, McLean C, Sheppard A, Rivett D, Jones K, Dalton S. LIF/STAT3 controls ES cell self-renewal and pluripotency by a Myc-dependent mechanism. Development. 2005;132:885–896. [Abstract] [Google Scholar]
- Chambers I, Smith A. Self-renewal of teratocarcinoma and embryonic stem cells. Oncogene. 2004;23:7150–7160. [Abstract] [Google Scholar]
- Dalton S. In: Regulatory networks in stem cells. Rajasekhar VK, Vemuri MC, editors. Humana Press; 2009. pp. 3–12. [Google Scholar]
- Dannenberg JH, van Rossum A, Schuijff L, te Riele H. Ablation of the retinoblastoma gene family deregulates G(1) control causing immortalization and increased cell turnover under growth-restricting conditions. Genes Dev. 2000;14:3051–3064. [Europe PMC free article] [Abstract] [Google Scholar]
- Draper JS, Smith K, Gokhale P, Moore HD, Maltby E, Johnson J, Meisner L, Zwaka TP, Thomson JA, Andrews PW. Recurrent gain of chromosomes 17q and 12 in cultured human embryonic stem cells. Nat Biotechnol. 2004;22:53–54. [Abstract] [Google Scholar]
- Edgar BA, Lehner CF. Developmental control of cell cycle regulators: a fly’s perspective. Science. 1996;274:1646–1652. [Abstract] [Google Scholar]
- Egli D, Birkhoff G, Eggan K. Mediators of reprogramming: transcription factors and transitions through mitosis. Nat Rev Mol Cell Biol. 2008;9:505–516. [Abstract] [Google Scholar]
- Faast R, White J, Cartwright P, Crocker L, Sarcevic B, Dalton S. Cdk6-cyclin D3 activity in murine ES cells is resistant to inhibition by p16(INK4a) Oncogene. 2004;23:491–502. [Abstract] [Google Scholar]
- Fernandez PC, Frank SR, Wang L, Schroeder M, Liu S, Greene J, Cocito A, Amati B. Genomic targets of the human c-Myc protein. Genes Dev. 2003;17:1115–1129. [Europe PMC free article] [Abstract] [Google Scholar]
- Filipczyk AA, Laslett AL, Mummery C, Pera MF. Differentiation is coupled to changes in the cell cycle regulatory apparatus of human embryonic stem cells. Stem Cell Res. 2007;1:45–60. [Abstract] [Google Scholar]
- Fingar DC, Richardson CJ, Tee AR, Cheatham L, Tsou C, Blenis J. mTOR controls cell cycle progression through its cell growth effectors S6K1 and 4E-BP1/eukaryotic translation initiation factor 4E. Mol Cell Biol. 2004;24:200–216. [Europe PMC free article] [Abstract] [Google Scholar]
- Fluckiger AC, Marcy G, Marchand M, Négre D, Cosset FL, Mitalipov S, Wolf D, Savatier P, Dehay C. Cell Cycle features of primate embryonic stem cells. Stem Cells. 2006;24:547–556. [Europe PMC free article] [Abstract] [Google Scholar]
- Ghule PN, Becker KA, Harper JW, Lian JB, Stein JL, van Wijnen AJ, Stein GS. Cell cycle dependent phosphorylation and subnuclear organization of the histone gene regulator p220(NPAT) in human embryonic stem cells. J Cell Physiol. 2007;213:9–17. [Abstract] [Google Scholar]
- Guccione E, Martinato F, Finocchiaro G, Luzi L, Tizzoni L, Dall’ Olio V, Zardo G, Nervi C, Bernard L, Amati B. Myc-binding-site recognition in the human genome is determined by chromatin context. Nat Cell Biol. 2006;8:764–70. [Abstract] [Google Scholar]
- Han DW, Do JT, Gentile L, Stehling M, Lee HT, Schöler HR. Pluripotential reprogramming of the somatic genome in hybrid cells occurs with the first cell cycle. Stem Cells. 2008;26:445–454. [Abstract] [Google Scholar]
- Hanna J, Markoulaki S, Mitalipova M, Cheng AW, Cassady JP, Staerk J, Carey BW, Lengner CJ, Foreman R, Love J, Gao Q, Kim J, Jaenisch R. Metastable pluripotent states in NOD-mouse-derived ESCs. Cell Stem Cell. 2009;4:1–12. [Europe PMC free article] [Abstract] [Google Scholar]
- Herrera RE, Chen F, Weinberg RA. Increased histone H1 phosphorylation and relaxed chromatin structure in Rb-deficient fibroblasts. Proc Natl Acad Sci U S A. 1996;93:11510–11515. [Europe PMC free article] [Abstract] [Google Scholar]
- Huangfu D, Osafune K, Maehr R, Guo W, Eijkelenboom A, Chen S, Muhlestein W, Melton DA. Induction of pluripotent stem cells from primary human fibroblasts with only Oct4 and Sox2. Nat Biotechnol. 2008;26:1269–1275. [Abstract] [Google Scholar]
- Jonk LJ, de Jonge ME, Kruyt FA, Mummery CL, van der Saag PT, Kruijer W. Aggregation and cell cycle dependent retinoic acid receptor mRNA expression in P19 embryonal carcinoma cells. Mech Dev. 1992;36:165–172. [Abstract] [Google Scholar]
- Jorgensen P, Tyers M. How cells coordinate growth and division. Current Biology. 2004;14:R1014–1027. [Abstract] [Google Scholar]
- Judson RL, Babiarz JE, Venere M, Blelloch R. Embryonic stem cell-specific miRNAs promote induced pluripotency. Nat Biotechnol. 2009;27:459–461. [Europe PMC free article] [Abstract] [Google Scholar]
- Kameda T, Thomson JA. Human ERas gene has an upstream premature polyadenylation signal that results in a truncated, noncoding transcript. Stem Cells. 2005;23:1535–1540. [Abstract] [Google Scholar]
- Kenney AM, Widlund HR, Rowitch DH. Hedgehog and PI-3 kinase signaling converge on Nmyc1 to promote cell cycle progression in cerebellar neuronal precursors. Development. 2004;131:217–228. [Abstract] [Google Scholar]
- Kidder BL, Yang J, Palmer S. Stat3 and c-Myc genome-wide promoter occupancy in embryonic stem cells. PLoS One Epub. 2008;3:e3932. [Europe PMC free article] [Abstract] [Google Scholar]
- Kim J, Chu J, Shen X, Wang J, Orkin SH. An extended transcriptional network for pluripotency of embryonic stem cells. Cell. 2008;132:1049–1061. [Europe PMC free article] [Abstract] [Google Scholar]
- Knoepfler PS. Why myc? An unexpected ingredient in the stem cell cocktail. Cell Stem Cell. 2008;2:18–21. [Abstract] [Google Scholar]
- Knoepfler PS, Cheng PF, Eisenman RN. N-myc is essential during neurogenesis for the rapid expansion of progenitor cell populations and the inhibition of neuronal differentiation. Genes Dev. 2002;16:2699–712. [Europe PMC free article] [Abstract] [Google Scholar]
- Knoepfler PS, Zhang XY, Cheng PF, Gafken PR, McMahon SB, Eisenman RN. Myc influences global chromatin structure. EMBO J. 2006;25:2723–2734. [Europe PMC free article] [Abstract] [Google Scholar]
- Laurenti E, Varnum-Finney B, Wilson A, Ferrero I, Blanco-Bose WE, Ehninger A, Knoepfler PS, Cheng PF, MacDonald HR, Eisenman RN, Bernstein ID, Trumpp A. Hematopoietic stem cell function and survival depend on c-Myc and N-Myc activity. Cell Stem Cell. 2008;3:611–624. [Europe PMC free article] [Abstract] [Google Scholar]
- Lawson KA, Meneses JJ, Pedersen RA. Clonal analysis of epiblast fate during germ layer formation in the mouse embryo. Development. 1991;113:891–911. [Abstract] [Google Scholar]
- Lee NS, Kim JS, Cho WJ, Lee MR, Steiner R, Gompers A, Ling D, Zhang J, Strom P, Behlke M, Moon SH, Salvaterra PM, Jove R, Kim KS. miR-302b maintains “stemness” of human embryonal carcinoma cells by post-transcriptional regulation of Cyclin D2 expression. Biochem Biophys Res Commun. 2008;377:434–440. [Abstract] [Google Scholar]
- Macaluso M, Montanari M, Giordano A. Rb family proteins as modulators of gene expression and new aspects regarding the interaction with chromatin remodeling enzymes. Oncogene. 2006;25:5263–5267. [Abstract] [Google Scholar]
- Maherali N, Ahfeldt T, Rigamonti A, Utikal J, Cowan C, Hochedlinger K. A high-efficiency system for the generation and study of human induced pluripotent stem cells. Cell Stem Cell. 2008;3:340–345. [Europe PMC free article] [Abstract] [Google Scholar]
- Malynn BA, de Alboran IM, O’Hagan RC, Bronson R, Davidson L, DePinho RA, Alt FW. N-myc can functionally replace c-myc in murine development, cellular growth, and differentiation. Genes Dev. 2000;14:1390–1399. [Europe PMC free article] [Abstract] [Google Scholar]
- McLean AB, D’Amour KA, Jones KL, Krishnamoorhthy M, Kulik MJ, Reynolds DM, Sheppard AM, Liu H, Xu Y, Baetge EE, Dalton S. Activin A efficiently specifies definitive endoderm from human embryonic stem cells only when phosphatidyl 3-kinase signaling is suppressed. Stem Cells. 2007;25:29–38. [Abstract] [Google Scholar]
- Meyer N, Penn LZ. Reflecting on 25 years with MYC. Nat Rev Cancer. 2008;8:976–990. [Abstract] [Google Scholar]
- Mikkelsen TS, Hanna J, Zhang X, Ku M, Wernig M, Schorderet P, Bernstein BE, Jaenisch R, Lander ES, Meissner A. Dissecting direct reprogramming through integrative genomic analysis. Nature. 2008;454:49–55. [Europe PMC free article] [Abstract] [Google Scholar]
- Mitalipova MM, Rao RR, Hoyer DM, Johnson JA, Meisner LF, Jones KL, Dalton S, Stice SL. Preserving the genetic integrity of human embryonic stem cells. Nat Biotechnol. 2005;23:19–20. [Abstract] [Google Scholar]
- Mummery CL, van Rooijen MA, van den Brink SE, de Laat SW. Cell cycle analysis during retinoic acid induced differentiation of a human embryonal carcinoma-derived cell line. Cell Differ. 1987;20:153–160. [Abstract] [Google Scholar]
- Murray AW, Kirschner MW. Cyclin synthesis drives the early embryonic cell cycle. Nature. 1989;339:275–280. [Abstract] [Google Scholar]
- Neganova I, Zhang X, Atkinson S, Lako M. Expression and functional analysis of G1 to S regulatory components reveals an important role for CDK2 in cell cycle regulation in human embryonic stem cells. Oncogene. 2009;28:20–30. [Abstract] [Google Scholar]
- Ohtsuka S, Dalton S. The biology of embryonic pluripotency. Gene Therapy. 2008;15:74–81. [Abstract] [Google Scholar]
- Okubo T, Knoepfler PS, Eisenman RN, Hogan BL. Nmyc plays an essential role during lung development as a dosage-sensitive regulator of progenitor cell proliferation and differentiation. Development. 2005;132:1363–1374. [Abstract] [Google Scholar]
- Paling NR, Wheadon H, Bone HK, Welham MJ. Regulation of embryonic stem cell self-renewal by phosphoinositide 3-kinase-dependent signaling. J Biol Chem. 2004;279:48063–48070. [Abstract] [Google Scholar]
- Pardee AB. A restriction point for control of normal animal cell proliferation. Proc Natl Acad Sci U S A. 1974;71:1286–1290. [Europe PMC free article] [Abstract] [Google Scholar]
- Ryan KM, Birnie GD. Myc oncogene: the enigmatic family. Biochem J. 1996;314:713–721. [Europe PMC free article] [Abstract] [Google Scholar]
- Sage J, Mulligan GJ, Attardi LD, Miller A, Chen S, Williams B, Theodorou E, Jacks T. Targeted disruption of the three Rb-related genes leads to loss of G(1) control and immortalization. Genes Dev. 2000;14:3037–3050. [Europe PMC free article] [Abstract] [Google Scholar]
- Satoh Y, Matsumura I, Tanaka H, Ezoe S, Sugahara H, Mizuki M, Shibayama H, Ishiko J, Nakajima K, Kanakura Y. Roles for c-Myc in self-renewal of hematopoietic stem cells. J Biol Chem. 2004;279:24986–24993. [Abstract] [Google Scholar]
- Saucedo LJ, Edgar BA. Why size matters: altering cell size. Curr Opin Gen Dev. 2002;12:565–571. [Abstract] [Google Scholar]
- Savatier P, Huang S, Szekely L, Wiman KG, Samarut J. Contrasting patterns of retinoblastoma protein expression in mouse embryonic stem cells and embryonic fibroblasts. Oncogene. 1994;9:809–818. [Abstract] [Google Scholar]
- Savatier P, Lapillonne H, van Grunsven LA, Rudkin BB, Samarut J. Withdrawal of differentiation inhibitory activity/leukemia inhibitory factor up-regulates D-type cyclins and cyclin-dependent kinase inhibitors in mouse embryonic stem cells. Oncogene. 1996;12:309–322. [Abstract] [Google Scholar]
- Schratt G, Weinhold B, Lundberg AS, Schuck S, Berger J, Schwarz H, Weinberg RA, Rüther U, Nordheim A. Serum response factor is required for immediate-early gene activation yet is dispensable for proliferation of embryonic stem cells. Mol Cell Biol. 2001;21:2933–2943. [Europe PMC free article] [Abstract] [Google Scholar]
- Sherr CJ. Cancer cell cycles. Science. 1996;274:1672–1677. [Abstract] [Google Scholar]
- Silva J, Barrandon O, Nichols J, Kawaguchi J, Theunissen TW, Smith A. Promotion of reprogramming to ground state pluripotency by signal inhibition. PLoS Biol. 2008;6:e253. [Abstract] [Google Scholar]
- Snow MHL. Gastrulation in the mouse: growth and regionalization of the epiblast. J Embryol Exp Morph. 1977;42:293–303. [Google Scholar]
- Sridharan R, Tchieu J, Mason MJ, Yachechko R, Kuoy E, Horvath S, Zhou Q, Plath K. Role of the murine reprogramming factors in the induction of pluripotency. Cell. 2009;136:364–377. [Europe PMC free article] [Abstract] [Google Scholar]
- Stead E, White J, Faast R, Conn S, Goldstone S, Rathjen J, Dhingra U, Rathjen P, Walker D, Dalton S. Pluripotent cell division cycles are driven by ectopic Cdk2, cyclin A/E and E2F activities. Oncogene. 2002;21:8320–8333. [Abstract] [Google Scholar]
- Sun H, Lesche R, Liliental DM, Zhang H, Gao J, Gavrilova N, Mueller B, Liu X, Wu H. PTEN modulates cell cycle progression and cell survival by regulating phosphatidylinositol 3,4,5-triphosphate and AKT/protein kinase B signaling pathway. Proc Natl Acad Sci USA. 1999;96:6199–6204. [Europe PMC free article] [Abstract] [Google Scholar]
- Takahashi K, Mitsui K, Yamanaka S. Role of ERas in promoting tumour-like properties in mouse embryonic stem cells. Nature. 2003;423:541–545. [Abstract] [Google Scholar]
- Takahashi K, Murakami M, Yamanaka S. Role of the phosphoinositide 3-kinase pathway in mouse embryonic stem (ES) cells. Biochem Soc Trans. 2005;33:1522–1525. [Abstract] [Google Scholar]
- Takahashi K, Yamanaka S. Induction of pluripotent stem cells from mouse embryonic and adult fibroblast cultures by defined factors. Cell. 2006;126:663–676. [Abstract] [Google Scholar]
- Tesar PJ, Chenoweth JG, Brook FA, Davies TJ, Evans EP, Mack DL, Gardner RL, McKay RD. New cell lines from mouse epiblast share defining features with human embryonic stem cells. Nature. 2007;448:196–199. [Abstract] [Google Scholar]
- Wang Y, Baskerville S, Shenoy A, Babiarz JE, Baehner L, Blelloch R. Embryonic stem cell-specific microRNAs regulate the G1-S transition and promote rapid proliferation. Nat Genet. 2008;40:1478–1483. [Europe PMC free article] [Abstract] [Google Scholar]
- Watanabe S, Umehara H, Murayama K, Okabe M, Kimura T, Nakano T. Activation of Akt signaling is sufficient to maintain pluripotency in mouse and primate embryonic stem cells. Oncogene. 2006;25:2697–2707. [Abstract] [Google Scholar]
- Watt FM, Frye M, Benitah SM. MYC in mammalian epidermis: how can an oncogene stimulate differentiation? Nat Rev Cancer. 2008;8:234–242. [Europe PMC free article] [Abstract] [Google Scholar]
- White J, Dalton S. Cell cycle control of embryonic stem cells. Stem Cell Rev. 2005;1:131–138. [Abstract] [Google Scholar]
- White J, Stead E, Faast R, Conn S, Cartwright P, Dalton S. Developmental activation of the Rb-E2F pathway and establishment of cell cycle-regulated cyclin-dependent kinase activity during embryonic stem cell differentiation. Mol Biol Cell. 2005;16:2018–2027. [Europe PMC free article] [Abstract] [Google Scholar]
- Wilson A, Murphy MJ, Oskarsson T, Kaloulis K, Bettess MD, Oser GM, Pasche AC, Knabenhans C, Macdonald HR, Trumpp A. c-Myc controls the balance between hematopoietic stem cell self-renewal and differentiation. Genes Dev. 2004;18:2747–2763. [Europe PMC free article] [Abstract] [Google Scholar]
- Yarden A, Geiger B. Zebrafish cyclin E regulation during early embryogenesis. Dev Dyn. 1996;206:1–11. [Abstract] [Google Scholar]
- Ying QL, Wray J, Nichols J, Batlle-Morera L, Doble B, Woodgett J, Cohen P, Smith A. The ground state of embryonic stem cell self-renewal. Nature. 2008;453:519–523. [Abstract] [Google Scholar]
- Zhang X, Neganova I, Przyborski S, Yang C, Cooke M, Atkinson SP, Anyfantis G, Fenyk S, Keith WN, Hoare SF, Hughes O, Strachan T, Stojkovic M, Hinds PW, Armstrong L, Lako M. A role for NANOG in G1 to S transition in human embryonic stem cells through direct binding of CDK6 and CDC25A. J Cell Biol. 2009;184:67–82. [Europe PMC free article] [Abstract] [Google Scholar]
- Zhao R, Daley GQ. From fibroblasts to iPS cells: induced pluripotency by defined factors. J Cell Biochem. 2008;105:949–955. [Abstract] [Google Scholar]
- Zindy F, Quelle DE, Roussel MF, Sherr CJ. Expression of p16INK4a tumor suppressor versus other INK4 family members during mouse development and aging. Oncogene. 1997;15:203–211. [Abstract] [Google Scholar]
- Zwaka TP, Thomson JA. Differentiation of human embryonic stem cells occurs through symmetric cell division. Stem Cells. 2005;23:146–149. [Abstract] [Google Scholar]
Full text links
Read article at publisher's site: https://doi.org/10.1016/j.stem.2009.07.003
Read article for free, from open access legal sources, via Unpaywall:
http://www.cell.com/article/S1934590909003373/pdf
Citations & impact
Impact metrics
Article citations
p21 Regulates Wnt-Notch balance via DREAM/MMB/Rb-E2F1 and maintains intestinal stem cell homeostasis.
Cell Death Discov, 10(1):413, 28 Sep 2024
Cited by: 0 articles | PMID: 39341834 | PMCID: PMC11438959
RNF122 promotes glioblastoma growth via the JAK2/STAT3/c-Myc signaling Axis.
CNS Neurosci Ther, 30(9):e70017, 01 Sep 2024
Cited by: 1 article | PMID: 39218810 | PMCID: PMC11366496
Titanium dioxide nanostructure-loaded Adriamycin surmounts resistance in breast cancer therapy: ABCA/P53/C-myc crosstalk.
Future Sci OA, 10(1):FSO979, 15 May 2024
Cited by: 1 article | PMID: 38827789 | PMCID: PMC11140649
Overexpression of Alpha-1 Antitrypsin Increases the Proliferation of Mesenchymal Stem Cells by Upregulation of Cyclin D1.
Int J Mol Sci, 25(4):2015, 07 Feb 2024
Cited by: 0 articles | PMID: 38396691 | PMCID: PMC10889413
<i>miR-1204</i> Positioning in 8q24.21 Involved in the Tumorigenesis of Colorectal Cancer by Targeting <i>MASPIN</i>.
Protein Pept Lett, 31(7):544-558, 01 Jan 2024
Cited by: 0 articles | PMID: 39082173
Go to all (187) article citations
Other citations
Similar Articles
To arrive at the top five similar articles we use a word-weighted algorithm to compare words from the Title and Abstract of each citation.
Myc represses primitive endoderm differentiation in pluripotent stem cells.
Cell Stem Cell, 7(3):343-354, 01 Sep 2010
Cited by: 132 articles | PMID: 20804970 | PMCID: PMC2954754
Roles for MYC in the establishment and maintenance of pluripotency.
Cold Spring Harb Perspect Med, 3(12):a014381, 01 Dec 2013
Cited by: 72 articles | PMID: 24296349 | PMCID: PMC3839598
Review Free full text in Europe PMC
Regeneration and reprogramming compared.
BMC Biol, 8:5, 20 Jan 2010
Cited by: 66 articles | PMID: 20089153 | PMCID: PMC2826312
Quick, Coordinated and Authentic Reprogramming of Ribosome Biogenesis during iPSC Reprogramming.
Cells, 9(11):E2484, 15 Nov 2020
Cited by: 4 articles | PMID: 33203179 | PMCID: PMC7697288
Funding
Funders who supported this work.
NICHD NIH HHS (2)
Grant ID: HD049647
Grant ID: R01 HD049647
NIGMS NIH HHS (8)
Grant ID: P01 GM085354-03
Grant ID: P01 GM085354-02S10001
Grant ID: P01 GM085354-02S1
Grant ID: P01 GM085354
Grant ID: P01 GM085354-010001
Grant ID: P01 GM085354-020001
Grant ID: P01 GM085354-01
Grant ID: GM75334