Abstract
Free full text

Over-expression of ST3Gal-I promotes mammary tumorigenesis
Abstract
Changes in glycosylation are common in malignancy, and as almost all surface proteins are glycosylated, this can dramatically affect the behavior of tumor cells. In breast carcinomas, the O-linked glycans are frequently truncated, often as a result of premature sialylation. The sialyltransferase ST3Gal-I adds sialic acid to the galactose residue of core 1 (Galβ1,3GalNAc) O-glycans and this enzyme is over-expressed in breast cancer resulting in the expression of sialylated core 1 glycans. In order to study the role of ST3Gal-I in mammary tumor development, we developed transgenic mice that over-express the sialyltransferase under the control of the human membrane-bound mucin 1 promoter. These mice were then crossed with PyMT mice that spontaneously develop mammary tumors. As expected, ST3Gal-I transgenic mice showed increased activity and expression of the enzyme in the pregnant and lactating mammary glands, the stomach, lungs and intestine. Although no obvious defects were observed in the fully developed mammary gland, when these mice were crossed with PyMT mice, a highly significant decrease in tumor latency was observed compared to the PyMT mice on an identical background. These results indicate that ST3Gal-I is acting as a tumor promoter in this model of breast cancer. This, we believe, is the first demonstration that over-expression of a glycosyltransferase involved in mucin-type O-linked glycosylation can promote tumorigenesis.
Introduction
Glycosylation is one of the most common forms of posttranslational modifications. Glycans covalently attached to proteins function in the correct folding of the protein, to acquire resistance to proteases, to allow the protein to interact with its ligands, to direct its location and to allow the correct recycling (Hakomori 2002). Thus changes in glycosylation can dramatically affect the function of glycoproteins and, as almost all surface proteins are glycosylated, alter the behavior of cells. Malignant transformation is accompanied by changes in both N- and O-glycosylation of proteins. For example, β1,6GlcNAc branching of N-glycans attached to Asn-X-Ser/Thr is often increased (Guo et al. 2008) whereas mucin-type O-linked glycans attached to Ser or Thr are generally reduced in length (Burchell et al. 2001).
Glycosylation is determined by the expression and relative activities of glycosyltransferases in particular tissues (Brockhausen 2006; Paulson et al. 2006; Brockhausen 2009). In the mammary gland, mucin-type O-glycans added to proteins are branched core 2-based structures (see Figure 1). However, in the change to malignancy, simple unbranched glycans such as Tn (GalNAc), STn (NeuAcα2,6GalNAc) and T (Galβ1,3GalNAc) antigens are found attached to O-glycans, with the core 2 structures being absent or reduced (see Figure 1). A number of mechanisms have been shown to be responsible for aberrant mucin-type glycosylation. In human cervical cancer, expression of Tn and STn has been shown to result from mutations in Cosmc that encodes for a molecular chaperone required for the active core 1 β3-Gal-transferase or T-synthase (Ju et al. 2008). However, in breast carcinomas, changes in the expression of glycosyltransferases appear to be the dominant mechanism. Thus expression of STn has been shown to be due to the activation of transcription of ST6GalNAc-I, which has the ability to successfully compete with other glycosyltransferases that act on the same substrates (Julien et al. 2006; Sewell et al. 2006). Moreover, in breast carcinomas, increased expression of ST3Gal-I is observed and this is correlated with increased tumor grade (Burchell et al. 1999). ST3Gal-I competes for the core 2-initiating enzyme for their common core 1 substrate (see Figure 1), therefore increased expression of ST3Gal-I results in the expression of sialylated core 1 (NeuAcα2,3Galβ1,3GalNAc, also known as sialylated T) rather than the normal branched core 2 glycans (Dalziel et al. 2001).
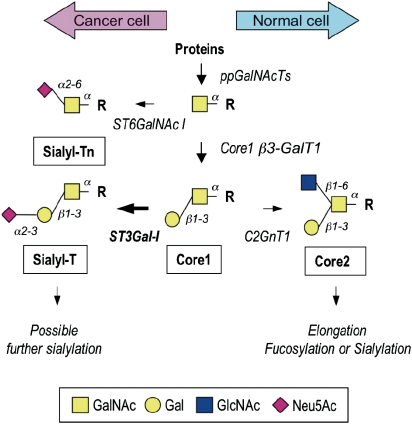
Initial steps of the biosynthesis of O-glycans in the mammary gland. Enzymes responsible for the biosynthesis of various O-glycan structures are indicated next to the arrows. Polypeptide GalNAc-transferase (ppGalNAcTs) initiates O-glycosylation by transferring GalNAc to serine or threonine residues (R). α6-Sialyltransferase (ST6GalNAc I) synthesizes the sialyl-Tn antigen by adding a sialylα6 residue to GalNAc. Alternatively, core 1 β3-Gal-transferase (C1GalT) synthesizes core 1, the T antigen. The sialyl-T structure is synthesized by α3-sialyltransferase ST3Gal-I. Further sialylation of sialyl-T can include α6-sialylation of GalNAc to form the desialylated T antigen and/or α2-8 sialylation of the α3-linked sialic acid residue. In the normal mammary gland, core 2 β6-GlcNAc-transferase (C2GnT) can introduce a branch, both arms of which can be further elongated and sialylated or fucosylated
The human membrane-bound mucin known as MUC1, which is expressed by luminal mammary epithelial cells, is highly upregulated in breast carcinomas and has often been used as a model to study the changes in O-linked glycosylation that occurs in breast cancer. Sialylated core 1 is a common O-glycan, often found attached to glycoproteins carrying O-linked glycans, for examples see Thomas and Winzler (1969) and Moody et al. (2003), but it is not normally found on epithelial mucins such as MUC1 (Hanisch et al. 1989). Moreover, at least 50% of the molecular weight of mucins is made up of glycans and there are hundreds of sites for O-linked glycosylation (Hollingsworth and Swanson 2004). Thus the repetitive nature of sialylated core 1 on mucins such as MUC1 in breast carcinomas may function quite differently to the more sparsely spaced sialylated core 1 glycans normally found on nonmucinous glycoproteins.
We have previously shown that murine mammary tumors expressing MUC1 carrying sialylated core 1 glycans grow significantly faster in MUC1 transgenic mice than the same tumors expressing MUC1 carrying the core 2 glycans (Mungul et al. 2004). In the present study, we have developed transgenic mice expressing ST3Gal-I running from the MUC1 promoter that allows upregulation of the sialyltransferase at pregnancy and lactation, and in mammary tumors (Graham et al. 2001). Although increased ST3Gal-I activity was detected in the mammary gland, the ST3Gal-I transgenic mice showed no obvious defects in the pregnant mammary gland and were capable of feeding their pups. However, when these mice were crossed with mice transgenic for the polyomavirus middle T antigen (PyMT) driven by the mouse mammary tumor virus (MMTV) promoter, over-expression of ST3Gal-I resulted in a significant decrease in tumor latency indicating that ST3Gal-I is acting as a tumor promoter in this model of breast cancer. This, we believe, is the first demonstration that over-expression of a glycosyltransferase involved in mucin-type O-linked glycosylation can promote tumorigenesis.
Results
Generation of the human ST3Gal-I transgenic mouse
Human ST3Gal-I cDNA (889-1952, GenBank L29555) was cloned into the pNASSβ vector downstream of the MUC1 promoter region (−1401 to +//0) (see Figure 2A). This region of the MUC1 promoter has been shown to be sufficient to direct tissue-specific expression in vivo and to allow upregulation during pregnancy and lactation, and in PyMT inducible mammary tumors (Graham et al. 2001). Two mice were shown to transmit the inserted gene and express hST3Gal-I in the expected tissues (Graham et al. 2001), and mice homozygous for hST3Gal-I were generated by littermate mating.
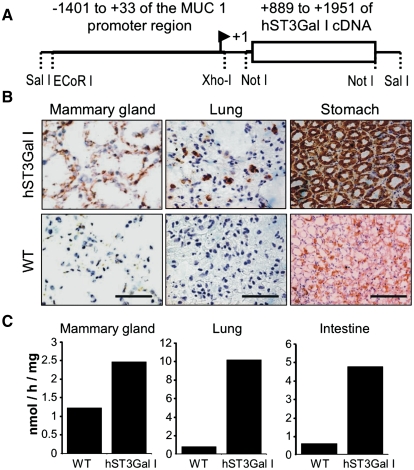
hST3Gal-I transgenic mice express the sialyltransferase in the expected tissues. (A) Construct used to inject fertilized eggs to develop the hST3Gal-I transgenic mice. (B) Frozen sections of tissue from hST3Gal-I transgenic mice and wild-type controls stained with the 4B10 antibody which specifically recognizes human ST3Gal-I. Scale bar represent 100 μm. (C) Enzymatic activity of ST3Gal in the indicated tissues from hST3Gal-I transgenic mice and wild-type control. Galβ1-3GalNAcα-pnp was used as the acceptor substrate (see Materials and methods). Assays were carried out in duplicate, which showed a variation of less than 10%
As expected from the activity of the MUC1 promoter, immunohistochemistry showed that hST3Gal-I was expressed in the pancreas, salivary glands (data not shown), lung, stomach, and pregnant and lactating mammary gland (see Figure 2B). This corresponded to an increase in sialyltransferase activity in the tissues measured using Galβ1-3GalNAcα-pnp as the acceptor substrate (see Figure 2C). Although hST3Gal-I expression and sialyltransferase activity were shown to be elevated in the mammary gland, the gland appeared normal at pregnancy and mice homozygote for hST3Gal-I were able to feed their pups which gained weight in a manner comparable to wild-type C57/Bl6 mice (data not shown).
Development of spontaneous mammary tumors over-expressing ST3Gal-I
To investigate the effect of over-expressing ST3Gal-I on spontaneous mammary tumor development, female hST3Gal-I+-/+- mice were crossed with male MMTV-PyMT mice, these mice were designated ST3Gal-I/PyMT. Female MMTV-PyMT mice develop multifocal mammary tumors (Guy et al. 1992) and have been used as a murine model resembling human breast cancer (Gendler and Mukherjee 2001; Maglione et al. 2001). As the hST3Gal-I transgenic mice were developed on a pure C57/Bl6 background (see Materials and methods), female offspring from male MMTV-PyMT crossed with female C57/Bl6 mice were used as controls (control/PyMT). Females from the ST3Gal-I/PyMT and the control/PyMT all developed mammary tumors with a 100% penetrance.
Expression of human ST3Gal-I was observed in tumors taken from the ST3Gal-I/PyMT but not in tumors taken from the control/PyMT (Figure 3A). This resulted in increased ST3Gal activity in tumors from the ST3Gal-1/PyMT mice compared to the control (Figure 3B). In contrast, the activity of polypeptide GalNAc transferases and C2Gn transferases was the same in the two groups of mice. The glycans expressed by the tumors were then analyzed by mass spectrometry to investigate if an increase in sialyltransferase activity resulted in an overall change in the total O-linked glycans linked to glycoproteins. Sialylated core 1 glycans with the sialic acid 2,3 linked to Gal were found in the ST3Gal-1/PyMT; however, these glycans were also observed in the control/PyMT tumors. Tumors from both types of mice also showed extended core 1 and core 2 structures partially decorated with sialic acid and fucose residues, and looking at this gross level, no consistent changes in the profile of O-glycans could be seen between tumors originating from the STGal-I/PyMT mice or the control/PyMT mice (see Supplementary data).
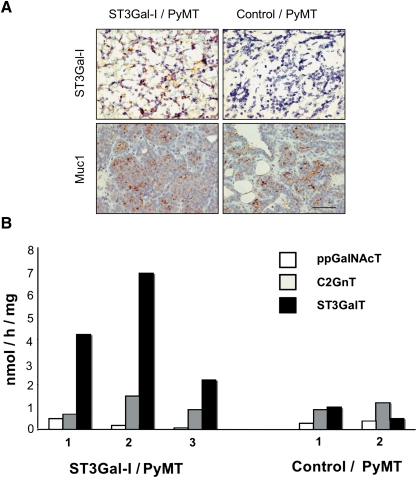
Mammary tumors arising in the ST3Gal-I/PyMT show expression of hST3Gal-I and increased ST3Gal enzyme activity. (A) Top panel, frozen sections of mammary tumors arising in the ST3Gal-I/PyMT mice and in Control/PyMT were stained with the 4B10 monoclonal antibody, which specifically recognizes human ST3Gal-I; bottom panel, formalin-fixed, paraffin-embedded sections of tumors stained with the polyclonal antibody, CT1, which recognizes the cytoplasmic tail of Muc1. (B) Snap-frozen tumors from ST3Gal-I/PyMT and Control/PyMT mice were assayed for polypeptide GalNAc-transferase (ppGalNAcT), core 2 β6-GlcNAc-transferase (C2GnT) and α3-sialyltransferase (ST3GalT) activity as described in Materials and methods. Figures on the X-axis refer to individual mice within each group. Assays were carried out in duplicate, which showed a variation of less than 10%
Over-expression of ST3Gal-I in MMTV-PyMT mice results in the earlier appearance of tumors
In the control/PyMT mice (n = 23), mammary tumors were first detected at 60 days. When the mice were 99 days old, 100% of the animals had at least one palpable tumor. In contrast, in the ST3Gal-I/PyMT mice (n = 33), the first mouse developed a tumor at 38 days old, and by 77 days all the mice had developed palpable mammary tumors. Analysis of the tumor-free survival of the mice (Figure 4A) showed that the ST3Gal-I/PyMT mice developed tumors significantly earlier than the control/PyMT animals (P < 0.001).
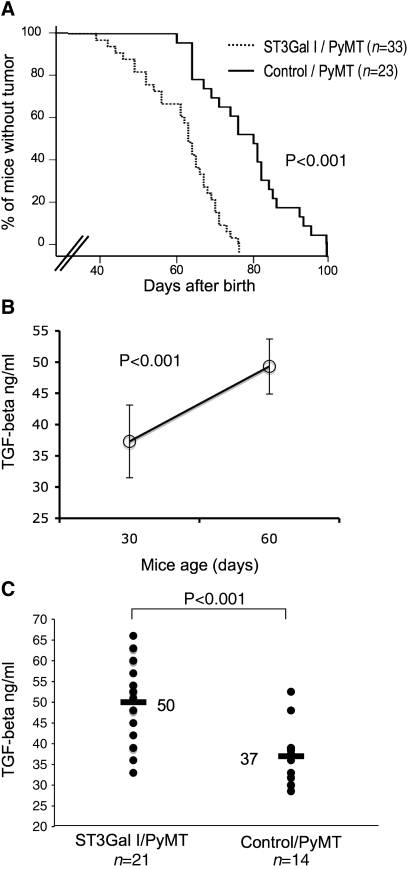
Mammary tumors develop faster in the ST3Gal-I/PyMT mice compared to the controls. (A) Kinetics of tumor formation in ST3Gal-I/PyMT and Control/PyMT mice. The difference between ST3Gal-I/PyMT and control mice was highly significant (P < 0.001), evaluated by the Breslow test. (B) Sera from control/PyMT mice (n = 6) were taken from the same mice at days 30 and 60 after birth and assayed for TGFβ. TGFβ levels increased significantly over time and were associated with the appearance and the number of mammary tumors. (C) Sera taken from ST3Gal-I/PyMT (n = 21) and Control/PyMT (n = 14) mice at a mean time of 38 and 42 days from birth, respectively, were assayed for TGFβ using a commercial ELISA kit. The difference between groups was significant (P < 0.001) evaluated by two-tailed T test
Tumors arising in PyMT mice can secrete cytokines, such as TGFβ, that can be measured in the blood (Mukherjee et al. 2003). Figure 4B shows that the amount of TGFβ in the serum significantly increases with time, indicating that it is reflecting the growth of the tumor. Determination of the level of TGFβ in the serum of the mice can therefore give an indication of tumor size and this can be analyzed before the appearance of palpable tumors. The concentration of TGFβ was determined in the serum taken before tumors could be detected by palpation, the mean time being 38 days from birth for the ST3Gal-1/PyMT mice and 42 days for the control/PyMT mice. Figure 4C shows significantly higher levels of TFGβ in the sera from ST3Gal-I/PyMT mice compared to that of control/PyMT mice (P < 0.001). This further demonstrates that in the ST3Gal-I/PyMT, mice development of tumors is initiated significantly earlier than mice on the same background but without the ST3Gal-I transgene.
Increased ST3Gal-I activity does not alter the interaction of Muc1 with c-Src
The ability of the PyMT to give rise to tumors is due to the interaction of PyMT with the Src family of kinases. Activation of c-Src is essential for the induction of mammary tumors in the MMTV-PyMT transgenic mice (Guy et al. 1994). Muc1, the murine ortholog of human MUC1 mucin that is upregulated in carcinomas, is expressed by PyMT-induced mammary tumors and can interact with c-Src and affect downstream Src signaling (Al Masri and Gendler 2005). The development of PyMT-induced mammary tumors in Muc1 null mice is significantly delayed indicating a role of Muc1 in tumor development (Spicer et al. 1995). As this mucin carries a large amount of O-linked sugars and its glycosylation is affected by the expression and activity of ST3Gal-I (Dalziel et al. 2001), we investigated whether increased expression of ST3Gal-I can alter the ability of Muc1 to interact with c-src and downstream effectors.
Using a polyclonal antibody to the cytoplasmic tail of Muc1, which is independent of the glycosylation, we initially looked at the expression of Muc1 in the PyMT-induced mammary tumors. Tumors from the ST3Gal-I/PyMT mice and control/PyMT mice expressed Muc1, and by immunohistochemistry, there was no difference in the level of expression (see Figure 3A). Mammary tumor lysates were immunoprecipitated with a monoclonal antibody to the cytoplasmic tail of Muc1 (CT2) or with anti-c-Src antibody and, after running on sodium dodecyl sulfate–polyacrylamide gel electrophoresis (SDS–PAGE), were immunoblotted with anti-c-Src or anti-Muc1 (CT1). On SDS–PAGE, the cytoplasmic tail of Muc1 is separated from the rest of the molecule and runs as band of around 25 kDa (Schroeder et al. 2001). As can be seen in Figure 5A, the interaction between c-Src and Muc1 is confirmed by the coimmunoprecipitations. However, although there is heterogeneity in the degree of interaction among the individual mice, there is no consistent difference between the groups.
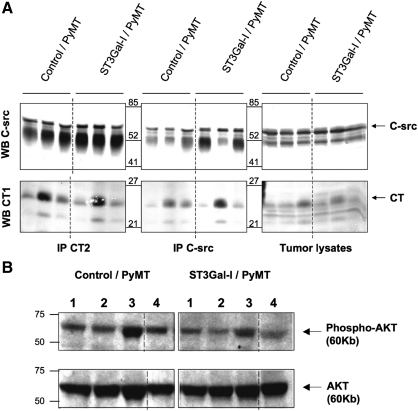
Increased ST3Gal activity does not affect the interaction of Muc1 with c-Src or downstream signaling. (A) Tumor lysates (1 mg) from Control/PyMT (n = 3) and ST3Gal-I/PyMT (n = 3) mice were immunoprecipitated with anti-c-Src or anti Muc1 (CT2) and immunoblotted for c-Src (upper panels) or Muc1 (CT1) (lower panels). (B) Tumor lysates (30 μg) from Control/PyMT mice (n = 4) and ST3Gal-I/PyMT mice (n = 4) were immunoblotted for phosphorylated AKT (upper panel) or total AKT (lower panel)
PI3kinase is also a direct target of PyMT oncogenesis and phosphorylates AKT. The interaction between the p83 subunit of PI3kinase and AKT is facilitated by the presence of Muc1 (Al Masri and Gendler 2005). We therefore investigated the phosphorylation of AKT in tumors from the two groups of mice. Although the level of phosphorylation differed in tumors from different mice, again there was no consistent difference between the two groups (see Figure 5B).
Morphology of the mammary tumors arising in the ST3Gal-I transgenic mice
Paraffin-embedded sections of mammary tumors taken from the ST3Gal-I/PyMT (n = 42 tumors) and control/PyMT (n = 33 tumors) were analyzed blind by a breast pathologist (SP) for differences in morphology and mitosis. Although tumors from both sets of mice showed areas of sheets of cells and areas of differentiation (Figure 6A) and necrosis, there was a trend for the tumors from the STGal-I/PyMT mice to be more differentiated (Figure 6B) although this did not reach significance, P = 0.086. The mitotic index was assessed by counting the number of mitosis observed in 10 high-power fields of each tumor. Although tumors from both groups of mice were highly mitotic with 37 mitoses on average per 10 high-power fields for control/PyMT and 42 for ST3Gal-I/PyMT mice, we did not observe a significant difference between the groups (Figure 6B).
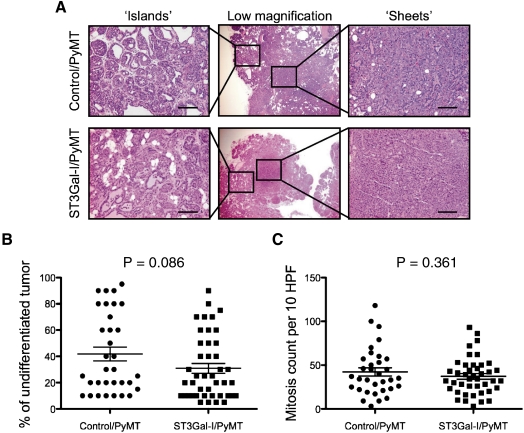
Morphology of tumors from ST3Gal-I/PyMT and Control/PyMT. (A) H&E staining of tumors from ST3Gal-I/PyMT mice and Control/PyMT showing areas of differentiation “islands of cell” and undifferentiation “sheets” of cells. Bars: 25 μm. (B) The percentage of undifferentiation in the sections of the tumors analyzed was estimated, each dot represents an individual tumor, ST3Gal-I/PyMT n = 42, Control/PyMT n = 33. (C) Mitotic count per 10 high-power fields (HPF), ST3Gal-I/PyMT n = 42, Control/PyMT n = 33
Discussion
Changes in glycosylation are observed in nearly all malignancies, including breast cancers. While normal mammary epithelial cells exclusively express core 2-based O-glycans carrying mucin-type O-linked glycosylation, cancer cells can express shorter truncated O-glycans (Burchell et al. 2001). The expression of these truncated glycans results in new epitopes being expressed on breast cancer cells. During the last two decades, the use of monoclonal antibodies (mAbs) has helped to demonstrate that expression of some truncated glycans, such as STn, is associated with poor prognosis in breast cancer. This suggests that these carbohydrates may have some function in cancer development (Miles et al. 1994; Julien et al. 2006).
The core 1 structure (Galβ1-3GalNAcα-Ser/Thr), otherwise known as T antigen, can be sialylated on α2-3 position of the Gal residue by the sialyltransferase ST3Gal-I, resulting in the Neu5Acα2-3Galβ1-3GalNAcα-Ser/Thr sometimes referred as sialyl-T (ST) structure. This is a common glycan expressed by breast cancers and one of the dominant glycoforms of the MUC1 mucin found in the serum of breast cancer patients (Storr et al. 2008). Moreover, MUC1 expressing ST (as recognized by the monoclonal antibody MY.1E12) is also associated with the progression of prostrate (Arai et al. 2005) and colon cancer (Suzuki et al. 2004).
The expression of ST by breast cancers is due to the over-expression of ST3Gal-I, the level of expression of this enzyme being related to tumor grade (Burchell et al. 1999). In vitro studies have shown that over-expression of ST3Gal-I is sufficient to cause a change from core 2-based glycans to ST being carried by mucins (Dalziel et al. 2001; Brockhausen 2006). Although ST is a common O-linked glycan, often expressed on glycoproteins found in blood (Thomas and Winzler 1969; Moody et al. 2003), its abundant expression on epithelial mucins is characteristic of cancer. As the interactions between glycans and their binding proteins (e.g. lectins) occur with a relatively low affinity compared to protein–protein interactions, the expression of multiple novel glycans on mucins can increase the avidity of binding and could therefore create new interactions. However, it is not clear if the expression of the ST glycan by epithelial cancers has any direct role in driving tumorigenesis. To address this question, we developed a transgenic mouse that expresses hST3Gal-I from the MUC1 promoter to induce sialyltransferase expression in epithelial tissues including the mammary gland (Graham et al. 2001). This hST3Gal-I transgenic mouse has then been crossed with the MMTV-PyMT mouse that develops spontaneous mammary tumors (Guy et al. 1992). In this in vivo context, which has been designed to be as closed as possible to natural cancer development, we have been able to assess the effect of the over-expression of ST3Gal-I on tumor development. The data presented here clearly show that ST3Gal-I promotes mammary gland carcinogenesis, as tumors arose significantly earlier in hST3Gal-I/PyMT mice than in control mice.
Increased sialyltransferase activity was detected in the ST3Gal-I/PyMT tumors. However, despite this, we could not detect any difference in glycosylation when tumors from these mice were compared to control/PyMT tumors using mass spectrometry to establish the comprehensive pattern of O-glycans (see Supplementary data). It could be that changes of glycosylation induced by increased ST3Gal-I only affect a specific and restricted portion of the tumor glycoproteins, which would not be detected using this gross analysis. However, it has been shown that spontaneous murine mammary tumors, like human breast cancer, exhibit changes in O-linked glycosylation (Graham et al. 1996) and indeed sialylated core 1 glycans were observed in the control/PyMT mice (see Supplementary data). Thus, it appears that when the tumors have developed and are larger enough to necessitate culling of the mice, the effect of expression of the ST3Gal-I transgene may not be able to compound the effect of endogenous aberrant glycosylation.
Glycosylation of extracellular domains can affect signaling and interactions of intracellular domains of a protein (Guo et al. 2007). We therefore looked at the interactions of Muc1, which carries many O-glycans and has been implicated in the tumorigenesis of PyMT-induced tumors. Although the interaction with c-Src reported by others (Al Masri and Gendler 2005) was confirmed, no differences could be observed in tumors from the two sets of animals and no differences were observed in downstream signaling. Moreover, when analyzed at the endpoint of the experiment, the tumors derived from the hST3Gal-I/PyMT mice or control mice did not show significant differences in terms of morphology or mitotic index, suggesting that ST3Gal-I expression did not affect the histopathological type of the tumor.
In a transplantable tumor model, we demonstrated that transplantable murine mammary tumors expressing MUC1 carrying ST developed significantly faster than tumors expressing MUC1 carrying core 2-based glycans. However, this response was not seen in immunosuppressed mice, suggesting an immunological mechanism (Mungul et al. 2004). We therefore analyzed the PyMT spontaneous tumors for evidence of a change in immune response when ST3Gal-I is over-expressed. We found no difference in the number of infiltrating lymphocytes (data not shown) or in the level of expression of cytokines (IFNγ, TNFα, TGFβ, IL2 and IL10) detected in the tumors by quantitative reverse transcription–polymerase chain reaction (qRT–PCR) (data not shown) when comparing tumors from ST3Gal-I/PyMT to tumors from control/PyMT mice.
Comparison of the levels of circulating TGFβ suggests that the difference in the development of the tumor already occurred in the mice prior to the detection of palpable tumor masses. Taken together, our results indicate that ST3Gal-I exerts its influence early in tumor development. It is now therefore important to analyze any differences at this early stage. Several mechanisms could be responsible for promoting tumorigenesis, one of them being the ability of the tumor to escape the immune surveillance early in tumor development, as suggested by the results from the transplantable tumor model (Mungul et al. 2004). Certainly changes in mucin-type glycosylation can affect how MUC1 interacts with the immune system, and while the expression of some glycans may be active in stimulating an immune response (Napoletano et al. 2007), other glycans such as ST, may inhibit a response (Mungul et al. 2004), suggesting that the type of O-glycans expressed by cancer cells may be able to modulate the immune response to the tumor.
In conclusion, we have demonstrated for the first time that over-expression of ST3Gal-I is functionally involved in oncogenesis. This suggests that ST3Gal-I over-expression in breast cancer is not just a collateral effect of carcinogenesis but may provide some advantages to tumor development. Our data also suggest that ST3Gal-I exerts its effect early in tumor development.
Materials and methods
Development of hST3Gal-I transgenic mice
Human ST3Gal-I (+88///Q to +197//Q) (accession number: L29555, EC:2.4.99.4) was amplified by PCR from a plasmid containing hST3Gal-I full cDNA with the following primers designed to insert restriction sites for Not 1 at both 5′ and 3′ ends of the amplicon:
Forward primer: 5′-ATCAGCTAGCGCGGCCGCTTCCTGCTACCCATCGT-3′
Reverse primer: 5′-TAGAAGTTCCCCTCTACTCCTAGGCGC-3′.
Using these Not I sites, the resulting cDNA was inserted into the pNASSβ plasmid (Clontech Laboratories Inc, Mountain View, California), downstream of the promoter region of human MUC1 (from −1401 to +//0) previously cloned in this plasmid (Graham et al. 2001). To confirm expression, the resulting construct was transfected into Cos7 cells, and the transfected cells were stained with a monoclonal antibody to hST3Gal-I. Transfected cells showed positive staining in the Golgi as expected (data not shown).
A 2.5-kb Sal I-Sca I fragment containing the MUC1 promoter fused to ST3Gal-I was purified (Qiagen kit) and dissolved in injection buffer (10 mM Tris, 0.1 mM ethylenediaminetetraacetic acid pH 7.4 prepared in ultrapure water). DNA (5 ng/L) was injected into the pronucleus of pure C57/Bl6 mouse embryos that were subsequently transferred into day 1-plugged pseudopregnant C57/Bl6 female mice.
Transgenic mice were identified by PCR on DNA isolated from tail snips, amplifying a 550-bp segment of DNA using oligonucleotides primers within the 3′ end of the MUC1 promoter and within the 5′ end of the ST3Gal-I. Two founder mice were identified that expressed hST3Gal-I in the expected tissues and from one founder, mice homozygous for hST3Gal-I were developed by littermate mating. Homozygosity was confirmed by backcrossing mice into WT C57Bl/6, which resulted in 100% of the offspring carrying hST3Gal-I.
Development of spontaneous tumors in hST3Gal-I transgenic mice
Homozygous hST3Gal-I female mice on a pure C57Bl/6 background were mated with FVB male mice heterozygous for the polyomavirus middle T antigen driven by the mouse mammary tumor virus (MMTV-PyMT mice (Guy et al. 1992)) promoter to obtain the ST3Gal-I/PyMT mice. Control mice (Control/PyMT mice) were derived by crossing female C57Bl/6 mice with MMTV-PyMT male mice. The offspring were screened for the PyMT by PCR of DNA prepared from tail snips using the following primers:
Forward primer: 5′-CCAGAACTCCTGTATCCAGAAGCG-3′
Reverse primer 5′-GGATGAGCTGGGGTACTTGTTCCCC-3′.
Female mice carrying the PyMT were examined three times per week for the development of tumors. All animal work was under project license number PPL 70/6847, strictly adhering to Home Office guidelines.
Immunohistochemistry
Frozen sections of mouse tissue and tumors were fixed in ice-cold acetone for 10 min. For staining for mouse Muc1, endogenous peroxidase activity was blocked in 1/100 H2O2/methanol for 10 min. Sections were blocked with 50% fetal calf serum and then incubated with a monoclonal antibody 4B10 to human ST3Gal-I (Vallejo-Ruiz et al. 2001) or a polyclonal antibody to the human MUC1 cytoplasmic tail that also reacts with mouse Muc1 (Pemberton et al. 1992). After extensive washing, the slides were incubated with biotinylated rabbit antimouse Ig or biotinylated goat antirabbit Ig (DAKO Denmark A/S, Glostrup, Denmark), respectively, for 30 min, washed and incubated with streptavidin biotin complexes linked to horse radish peroxidase (DAKO). Binding was visualized with diaminobenzidine (DAKO), and the sections were counterstained with hematoxylin. Hematoxylin and eosin (H&E) staining on formalin-fixed, paraffin-embedded tissue was used for additional histological analysis.
Glycosyltransferase assays
Homogenates of mouse tissues were prepared by mincing tissues and hand homogenizing in five times volume of 0.25 M sucrose. Protein contents were determined by the Bio-Rad Laboratories Inc, Berkeley, California protein assay (Bradford) using bovine serum albumin (BSA) as the standard. All enzymes were assayed in at least duplicate determinations, and results varied by <10% between duplicates. Assays lacking exogenously added acceptor substrate provided the background radioactivity.
Sialyltransferase
ST3Gal transferase assay mixtures contained in a total volume of 40 μL: 2 mM Galβ1-3GalNAcα-p-nitrophenyl (Galβ1-3GalNAcα-pnp) acceptor substrate, 0.1 M Tris–HCl, pH 7, 0.125% Triton X-100, 5 mM MnCl2, 0.4 mM CMP-[3H]sialic acid (2420 cpm/nmol) and 10 μL homogenate (0.08–0.15 mg protein). The mixtures were incubated for 1 h at 37°C. Reactions were stopped with 0.2 mL water and freezing, mixtures filtered through 0.1 mL of Bio-Gel P2 and washed three times with 0.4 mL water. After lyophilizing combined eluates, 120 μL of water was added and 80 μL injected into high-performance liquid chromatography (HPLC), using an analytical amine column and acetonitrile/15 mM K-phosphate, pH 5.2 = 75/25 as the mobile phase. The absorbance at 195 nm and radioactivity of 2 min fractions were monitored. Sialic acid eluted at about 25–30 min and enzyme product at 14–18 min. Radioactivity of fractions was determined by scintillation counting. Radioactive CMP-sialic acid did not elute under these conditions but can be eluted with acetonitrile/15 mM K-phosphate, pH 5.2 = 50/50.
Polypeptide GalNAc-transferase
Polypeptide GalNAc-transferase activity was assayed in mixtures containing in a total volume of 40 μL: 0.5 mM acceptor substrate AQPTPPP, 0.125 M MES buffer, pH 7, 0.125% Triton X-100, 10 mM AMP, 10 mM MnCl2, 0.9 mM UDP-[3H]GalNAc (3795 cpm/nmol) and 10 μL homogenate (0.08–0.15 mg protein). The mixtures were incubated for 1 h at 37°C. Reactions were stopped with 0.6 mL water and freezing. Mixtures were applied to columns of 0.4 mL AG1x8, which were washed two times with 0.6 mL water. Eluates were lyophilized, and enzyme product was separated by HPLC using a C18 column and acetonitrile/water = 6/94 as the mobile phase. The absorbance at 195 nm and radioactivity of 2-min fractions were monitored. Radioactivity of fractions was determined by scintillation counting.
Core 2 β6GlcNAc-transferase
Core 2 β6GlcNAc-transferase assay mixtures contained in a total volume of 40 μL: 2 mM Galβ1-3GalNAcα-Bn acceptor substrate, 0.125 M GlcNAc, 0.125 M MES buffer, pH 7, 0.125% Triton X-100, 10 mM AMP, 0.5 mM UDP-[3H]GlcNAc (5230 cpm/nmol) and 10 μL homogenate (0.06–0.24 mg protein). The mixtures were incubated, in duplicates, for 1 h at 37°C. Product was isolated as described for polypeptide GalNAc-transferase by AG1x8 and HPLC using a C18 column and acetonitrile/water = 12/88 as the mobile phase.
TGFβ quantification
The concentration of TGFβ in mouse serum was measured by a commercially available two-site sandwich enzyme-linked immunosorbent assay (ELISA) from R&D Systems Europe Ltd, Abingdon, UK according to manufacturer’s instructions. Assays were performed in duplicate and experiments repeated twice.
Immunoprecipitation
Snap-frozen tumors were crushed using a Mikro-dismembrator II (B Braun Biotech, Melsungen, Germany). Tumor powders were dissolved in lysis buffer (Tris/HCl 50 mM, NaCl 150 mM, Triton X-100 0.1%) containing the complete mini antiprotease cocktail (Roche, Lewes, UK) and incubated for 6 h. Lysates were homogenized with an Ultra-Turrax T8 (Ika, Fisher Scientific, Loughborough, UK) and further incubated for 2 h. Total tumor lysates (2 mg of proteins) were precleared using 50 μL of A/G proteins agarose beads (Roche) for 2 h. The lysates were then incubated with 5 μg of anti-c-Src antibody (Santa Cruz Biotechnology Inc, Santa Cruz, California) or 100 μL of anti-CT supernatant (CT2 antibody), overnight at 4°C.
A/G protein beads (100 μL) were subsequently added and incubated for 4 h. Immunoprecipitated proteins were washed using phosphate-buffered saline, then eluted from the bead using elution buffer (0.1 M glycine at pH 2.5) for two incubations of 15 min each. All procedures were carried out at 4°C using ice-cold reagents.
Electrophoresis and western blotting
The immunoprecipitates were loaded onto 4–12% gradient acrylamide gels, submitted to SDS–PAGE electrophoresis under reducing conditions and electro-transferred onto nitrocellulose membranes (Biotrace NT; Gelman Sciences Inc, Ann Arbor, Michigan) in accordance with standard procedures. Membranes were blocked in 1% BSA in Tris-buffered saline (TBS) and incubated with primary antibodies, anti-c-Src or a polyclonal antibody (CT1) to the cytoplasmic tail of Muc1, in TBS 0.05% Tween-20, for 1 h. After washing, labeled proteins were revealed using appropriate secondary antibodies conjugated to alkaline-phosphatase and NBT/X-phosphate revelation reagent (Roche).
Western blots for AKT and Phospho-AKT were performed using antibodies from Cell Signalling Technology Ltd, Herts, UK using a phospho-Ser473 specific antibody and visualized using ECL system (GE Healthcare Life Sciences, Little Chalfont, UK).
Supplementary data
Supplementary data for this article is available online at http://glycob.oxfordjournals.org/.
Funding
This work was supported by grants from the Cancer Research UK and the Canadian Cystic Fibrosis Foundation. The authors also acknowledge financial support from the Department of Health via the National Institute for Health Research (NIHR) comprehensive Biomedical Research Centre award to Guy’s & St Thomas’ NHS Foundation Trust in partnership with King’s College London and King’s College Hospital NHS Foundation Trust.
Acknowledgments
We would like to thank Janet MacDonald, Gary Martin and Del Watling for their excellent help with the animal experiments. We would also like to thank Dr. Sandra Gendler of the Mayo Clinic College of Medicine, Scottsdale, for her generous gift of the CT2 antibody and Dr. Joseph Lau, Roswell Park Cancer Institute, Buffalo, for his generous gift of the ST3Gal-I cDNA.
Glossary
Abbreviations
BSA | bovine serum albumin |
ELISA | enzyme-linked immunosorbent assay |
HPLC | high-performance liquid chromatography |
MMTV | mouse mammary tumor virus |
MUC1 | human membrane-bound mucin 1 |
Muc1 | murine membrane-bound mucin 1 |
PyMT | polyomavirus middle T antigen |
qRT–PCR | quantitative reverse transcription–polymerase chain reaction |
SDS–PAGE | sodium dodecyl sulfate–polyacrylamide gel electrophoresis |
ST | sialyl-T |
STn | sialylated N-acetylgalactosamine-Ser/Thr (NeuAcα2,6GalNAc) |
T | disaccharide D-Gal-beta 1,3-GalNAc-Ser/Thr (Galβ1,3GalNAc-Ser/Thr) |
TBS | Tris-buffered saline |
TGFβ | transforming growth factor beta |
Tn | N-acetylgalactosamine-Ser/Thr (GalNAc-Ser/Thr) |
References
- Al Masri A, Gendler SJ. Muc1 affects c-Src signaling in PyV MT-induced mammary tumorigenesis. Oncogene. 2005;24:5799–5808. [Abstract] [Google Scholar]
- Arai T, Fujita K, Fujime M, Irimura T. Expression of sialylated MUC1 in prostate cancer: Relationship to clinical stage and prognosis. Int J Urol. 2005;12:654–661. [Abstract] [Google Scholar]
- Brockhausen I. Mucin-type O-glycans in human colon and breast cancer: Glycodynamics and functions. EMBO Rep. 2006;7:599–604. [Europe PMC free article] [Abstract] [Google Scholar]
- Brockhausen I. Biosynthesis of complex mucin-type O-glycans. In: Wang G, editor. Comprehensive Natural Products Chemistry II. London, UK: Elsevier Limited; 2009. [Google Scholar]
- Burchell J, Poulsom R, Hanby A, Whitehouse C, Cooper L, Clausen H, Miles D, Taylor-Papadimitriou J. An alpha2,3 sialyltransferase (ST3Gal I) is elevated in primary breast carcinomas. Glycobiology. 1999;9:1307–1311. [Abstract] [Google Scholar]
- Burchell JM, Mungul A, Taylor-Papadimitriou J. O-linked glycosylation in the mammary gland: Changes that occur during malignancy. J Mammary Gland Biol Neoplasia. 2001;6:355–364. [Abstract] [Google Scholar]
- Dalziel M, Whitehouse C, McFarlane I, Brockhausen I, Gschmeissner S, Schwientek T, Clausen H, Burchell JM, Taylor-Papadimitriou J. The relative activities of the C2GnT1 and ST3Gal-I glycosyltransferases determine O-glycan structure and expression of a tumor-associated epitope on MUC1. J Biol Chem. 2001;276:11007–11015. [Abstract] [Google Scholar]
- Gendler SJ, Mukherjee P. Spontaneous adenocarcinoma mouse models for immunotherapy. Trends Mol Med. 2001;7:471–475. [Abstract] [Google Scholar]
- Graham RA, Burchell JM, Beverley P, Taylor-Papadimitriou J. Intramuscular immunisation with MUC1 cDNA can protect C57 mice challenged with MUC1-expressing syngeneic mouse tumour cells. Int J Cancer. 1996;65:664–670. [Abstract] [Google Scholar]
- Graham RA, Morris JR, Cohen EP, Taylor-Papadimitriou J. Up-regulation of MUC1 in mammary tumors generated in a double-transgenic mouse expressing human MUC1 cDNA, under the control of 1.4-kb 5′ MUC1 promoter sequence and the middle T oncogene, expressed from the MMTV promoter. Int J Cancer. 2001;92:382–387. [Abstract] [Google Scholar]
- Guo HB, Nairn A, Harris K, Randolph M, Alvarez-Manilla G, Moremen K, Pierce M. Loss of expression of N-acetylglucosaminyltransferase Va results in altered gene expression of glycosyltransferases and galectins. FEBS Lett. 2008;582:527–535. [Europe PMC free article] [Abstract] [Google Scholar]
- Guo HB, Randolph M, Pierce M. Inhibition of a specific N-glycosylation activity results in attenuation of breast carcinoma cell invasiveness-related phenotypes: Inhibition of epidermal growth factor-induced dephosphorylation of focal adhesion kinase. J Biol Chem. 2007;282:22150–22162. [Abstract] [Google Scholar]
- Guy CT, Cardiff RD, Muller WJ. Induction of mammary tumors by expression of polyomavirus middle T oncogene: A transgenic mouse model for metastatic disease. Mol Cell Biol. 1992;12:954–961. [Europe PMC free article] [Abstract] [Google Scholar]
- Guy CT, Muthuswamy SK, Cardiff RD, Soriano P, Muller WJ. Activation of the c-Src tyrosine kinase is required for the induction of mammary tumors in transgenic mice. Genes Dev. 1994;8:23–32. [Abstract] [Google Scholar]
- Hakomori S. Glycosylation defining cancer malignancy: New wine in an old bottle. Proc Natl Acad Sci USA. 2002;99:10231–10233. [Europe PMC free article] [Abstract] [Google Scholar]
- Hanisch FG, Uhlenbruck G, Peter-Katalinic J, Egge H, Dabrowski J, Dabrowski U. Structures of neutral O-linked polylactosaminoglycans on human skim milk mucins. A novel type of linearly extended poly-N-acetyllactosamine backbones with Gal beta(1-4)GlcNAc beta(1-6) repeating units. J Biol Chem. 1989;264:872–883. [Abstract] [Google Scholar]
- Hollingsworth MA, Swanson BJ. Mucins in cancer: Protection and control of the cell surface. Nat Rev Cancer. 2004;4:45–60. [Abstract] [Google Scholar]
- Ju T, Lanneau GS, Gautam T, Wang Y, Xia B, Stowell SR, Willard MT, Wang W, Xia JY, Zuna RE, et al. Human tumor antigens Tn and sialyl Tn arise from mutations in Cosmc. Cancer Res. 2008;68:1636–1646. [Abstract] [Google Scholar]
- Julien S, Adriaenssens E, Ottenberg K, Furlan A, Courtand G, Vercoutter-Edouart AS, Hanisch FG, Delannoy P, Le Bourhis X. ST6GalNAc I expression in MDA-MB-231 breast cancer cells greatly modifies their O-glycosylation pattern and enhances their tumourigenicity. Glycobiology. 2006;16:54–64. [Abstract] [Google Scholar]
- Maglione JE, Moghanaki D, Young LJ, Manner CK, Ellies LG, Joseph SO, Nicholson B, Cardiff RD, MacLeod CL. Transgenic Polyoma middle-T mice model premalignant mammary disease. Cancer Res. 2001;61:8298–8305. [Abstract] [Google Scholar]
- Miles DW, Happerfield LC, Smith P, Gillibrand R, Bobrow LG, Gregory WM, Rubens RD. Expression of sialyl-Tn predicts the effect of adjuvant chemotherapy in node-positive breast cancer. Br J Cancer. 1994;70:1272–1275. [Europe PMC free article] [Abstract] [Google Scholar]
- Moody AM, North SJ, Reinhold B, Van Dyken SJ, Rogers ME, Panico M, Dell A, Morris HR, Marth JD, Reinherz EL. Sialic acid capping of CD8beta core 1-O-glycans controls thymocyte-major histocompatibility complex class I interaction. J Biol Chem. 2003;278:7240–7246. [Abstract] [Google Scholar]
- Mukherjee P, Madsen CS, Ginardi AR, Tinder TL, Jacobs F, Parker J, Agrawal B, Longenecker BM, Gendler SJ. Mucin 1-specific immunotherapy in a mouse model of spontaneous breast cancer. J Immunother. 2003;26:47–62. [Abstract] [Google Scholar]
- Mungul A, Cooper L, Brockhausen I, Ryder K, Mandel U, Clausen H, Rughetti A, Miles DW, Taylor-Papadimitriou J, Burchell JM. Sialylated core 1 based O-linked glycans enhance the growth rate of mammary carcinoma cells in MUC1 transgenic mice. Int J Oncol. 2004;25:937–943. [Abstract] [Google Scholar]
- Napoletano C, Rughetti A, Agervig Tarp MP, Coleman J, Bennett EP, Picco G, Sale P, Denda-Nagai K, Irimura T, Mandel U, et al. Tumor-associated Tn-MUC1 glycoform is internalized through the macrophage galactose-type C-type lectin and delivered to the HLA class I and II compartments in dendritic cells. Cancer Res. 2007;67:8358–8367. [Abstract] [Google Scholar]
- Paulson JC, Blixt O, Collins BE. Sweet spots in functional glycomics. Nat Chem Biol. 2006;2:238–248. [Abstract] [Google Scholar]
- Pemberton L, Taylor-Papadimitriou J, Gendler SJ. Antibodies to the cytoplasmic domain of the MUC1 mucin show conservation throughout mammals. Biochem Biophys Res Commun. 1992;185:167–175. [Abstract] [Google Scholar]
- Schroeder JA, Thompson MC, Gardner MM, Gendler SJ. Transgenic MUC1 interacts with epidermal growth factor receptor and correlates with mitogen-activated protein kinase activation in the mouse mammary gland. J Biol Chem. 2001;276:13057–13064. [Abstract] [Google Scholar]
- Sewell R, Backstrom M, Dalziel M, Gschmeissner S, Karlsson H, Noll T, Gatgens J, Clausen H, Hansson GC, Burchell J, et al. The ST6GalNAc-I sialyltransferase localizes throughout the Golgi and is responsible for the synthesis of the tumor-associated sialyl-Tn O-glycan in human breast cancer. J Biol Chem. 2006;281:3586–3594. [Abstract] [Google Scholar]
- Spicer AP, Rowse GJ, Lidner TK, Gendler SJ. Delayed mammary tumor progression in Muc-1 null mice. J Biol Chem. 1995;270:30093–30101. [Abstract] [Google Scholar]
- Storr SJ, Royle L, Chapman CJ, Hamid UM, Robertson JF, Murray A, Dwek RA, Rudd PM. The O-linked glycosylation of secretory/shed MUC1 from an advanced breast cancer patient’s serum. Glycobiology. 2008;18:456–462. [Abstract] [Google Scholar]
- Suzuki H, Shoda J, Kawamoto T, Shinozaki E, Miyahara N, Hotta S, Iizuka Y, Nakahara A, Tanaka N, Yanaka A, et al. Expression of MUC1 recognized by monoclonal antibody MY.1E12 is a useful biomarker for tumor aggressiveness of advanced colon carcinoma. Clin Exp Metastasis. 2004;21:321–329. [Abstract] [Google Scholar]
- Thomas DB, Winzler RJ. Structural studies on human erythrocyte glycoproteins. Alkali-labile oligosaccharides. J Biol Chem. 1969;244:5943–5946. [Abstract] [Google Scholar]
- Vallejo-Ruiz V, Haque R, Mir AM, Schwientek T, Mandel U, Cacan R, Delannoy P, Harduin-Lepers A. Delineation of the minimal catalytic domain of human Galbeta1-3GalNAc alpha2, 3-sialyltransferase (hST3Gal I) Biochim Biophys Acta. 2001;1549:161–173. [Abstract] [Google Scholar]
Articles from Glycobiology are provided here courtesy of Oxford University Press
Full text links
Read article at publisher's site: https://doi.org/10.1093/glycob/cwq085
Read article for free, from open access legal sources, via Unpaywall:
https://academic.oup.com/glycob/article-pdf/20/10/1241/16653325/cwq085.pdf
Free after 12 months at glycob.oxfordjournals.org
http://glycob.oxfordjournals.org/cgi/reprint/20/10/1241.pdf
Free to read at glycob.oxfordjournals.org
http://glycob.oxfordjournals.org/cgi/content/abstract/20/10/1241
Free after 12 months at glycob.oxfordjournals.org
http://glycob.oxfordjournals.org/cgi/content/full/20/10/1241
Citations & impact
Impact metrics
Citations of article over time
Article citations
Predictors of Remission or Combined Remission and Low Disease Activity in Rheumatoid Arthritis Patients in Taiwan: A Prospective Cohort Study.
J Clin Med, 13(9):2521, 25 Apr 2024
Cited by: 1 article | PMID: 38731049 | PMCID: PMC11084563
Unraveling the impact of sialic acids on the immune landscape and immunotherapy efficacy in pancreatic cancer.
J Immunother Cancer, 11(11):e007805, 01 Nov 2023
Cited by: 5 articles | PMID: 37940346 | PMCID: PMC10632901
Mucin1 as a potential molecule for cancer immunotherapy and targeted therapy.
J Cancer, 15(1):54-67, 01 Jan 2024
Cited by: 4 articles | PMID: 38164273 | PMCID: PMC10751670
Review Free full text in Europe PMC
SIGLEC-5/14 Inhibits CD11b/CD18 Integrin Activation and Neutrophil-Mediated Tumor Cell Cytotoxicity.
Int J Mol Sci, 24(24):17141, 05 Dec 2023
Cited by: 0 articles | PMID: 38138970 | PMCID: PMC10742634
Sialic-Acid-Related Enzymes of B Cells and Monocytes as Novel Markers to Discriminate Improvement Categories and to Fulfill Two Remission Definitions in Rheumatoid Arthritis.
Int J Mol Sci, 24(16):12998, 20 Aug 2023
Cited by: 1 article | PMID: 37629178 | PMCID: PMC10455111
Go to all (72) article citations
Data
Data behind the article
This data has been text mined from the article, or deposited into data resources.
BioStudies: supplemental material and supporting data
Nucleotide Sequences
- (2 citations) ENA - L29555
Protein structures in PDBe
-
(1 citation)
PDBe - 3GalView structure
Similar Articles
To arrive at the top five similar articles we use a word-weighted algorithm to compare words from the Title and Abstract of each citation.
An alpha2,3 sialyltransferase (ST3Gal I) is elevated in primary breast carcinomas.
Glycobiology, 9(12):1307-1311, 01 Dec 1999
Cited by: 100 articles | PMID: 10561455
MUC1 in human and murine mammary carcinoma cells decreases the expression of core 2 β1,6-N-acetylglucosaminyltransferase and β-galactoside α2,3-sialyltransferase.
Glycobiology, 22(8):1042-1054, 25 Apr 2012
Cited by: 20 articles | PMID: 22534569
Cyclooxygenase-2 enzyme induces the expression of the α-2,3-sialyltransferase-3 (ST3Gal-I) in breast cancer.
J Biol Chem, 287(53):44490-44497, 01 Dec 2012
Cited by: 15 articles | PMID: 23275522 | PMCID: PMC3531762
O-linked glycosylation in the mammary gland: changes that occur during malignancy.
J Mammary Gland Biol Neoplasia, 6(3):355-364, 01 Jul 2001
Cited by: 123 articles | PMID: 11547903
Review
Funding
Funders who supported this work.