Abstract
Free full text

Therapeutic potential of adult bone marrow-derived mesenchymal stem cells in diseases of the skeleton
Abstract
Mesenchymal stem cells (MSCs) are the most popular among the adult stem cells in tissue engineering and regenerative medicine. Since their discovery and functional characterization in the late sixties and early seventies, MSCs or MSC-like cells have been obtained from various mesodermal and non-mesodermal tissues, although majority of the therapeutic applications involved bone marrow derived MSCs. Based on its mesenchymal origin, it was predicted earlier that MSCs only can differentiate into mesengenic lineages like bone, cartilage, fat or muscle. However, varied isolation and cell culturing methods identified subsets of MSCs in the bone marrow which not only differentiated into mesenchymal lineages, but also into ectodermal and endodermal derivatives. Although, true pluripotent status is yet to be established, MSCs have been successfully used in bone and cartilage regeneration in osteoporotic fracture and arthritis respectively and in the repair of cardiac tissue following myocardial infarction. Immunosuppressive properties of MSCs extend utility of MSCs to reduce complications of graft versus host disease and rheumatoid arthritis. Homing of MSCs to sites of tissue injury, including tumor, is well established. In addition to their ability in tissue regeneration, MSCs can be genetically engineered ex vivo for delivery of therapeutic molecule(s) to the sites of injury or tumorigenesis as cell therapy vehicles. MSCs tend to lose surface receptors for trafficking and have been reported to develop sarcoma in long-term culture. In this article, we reviewed the current status of MSCs with special emphasis to therapeutic application in bone-related diseases.
History and therapeutic relevance of mesenchymal stem cells
According to National Institute of Health resources for stem cell research, an adult stem cell is defined as “an undifferentiated cell, found among differentiated cells in a tissue or organ that can renew itself and can differentiate to yield some or all of the major specialized cell types of the tissue or organ.” Adult stem cells help to maintain and repair tissues of residence, and inherited or acquired genetic defect in them may lead to tissue malfunction and deformity. Pluripotent status of the adult stem cells is often questioned as they have not been successfully tested to produce a viable offspring when injected into a tetraploid murine blastocyst (Eggan & Jaenisch, 2003). Hence, adult stem cells are rather termed as multipotent based on their property to differentiate into selective adult tissue lineages of similar and dissimilar embryonic origin (Jiang et al, 2002). Therapeutic application of postnatal adult stem cells gained attention as it potentially bypasses the ethical debate regarding use of fertilized embryos to generate stem cells. Moreover, despite successful multi-lineage differentiation into functional tissues serious safety concerns have been raised due to frequent incidence of teratoma formation from embryonic stem cells isolated from both mouse and human blastocysts (Knoepfler PS, 2009). In contrast, use of hematopoietic stem cells (HSCs) is as old as bone marrow transplantation in patients with blood diseases ranging from sickle cell anemia to multiple myeloma and leukemia (Hsieh et al, 2009; Brown et al, 2009; Duarte et al, 2010). The non-hematopoietic component of the bone marrow comprises of bone marrow stromal cells among which the MSCs are most extensively used in tissue regeneration. MSCs were first identified by AJ Friedenstein (1970) as mononuclear and plastic adherent in a monolayer culture of guinea pig bone marrow. The cells formed fibroblast-like colonies, that Friedenstein termed colony forming units-fibroblast (CFU-F) which also showed mesengenic potential towards osteogenesis, chondrogenesis and adipogenesis. Since then cells with MSC like characteristics have been identified not only in mesodermal tissues (bone marrow, trabecular bone, synovium, cartilage, fat, muscle and tonsil) but also in endoderm (thymus, liver) and ectoderm (skin, hair follicle, dura mater and dental pulp) derived tissues (Phinney & Prockop, 2007) although majority of the therapeutic studies involved bone marrow derived MSCs. MSC-like cells were also obtained from the peripheral blood but they lack stro1 and CD105 on their surface (Kuznetsov et al, 2001). Moreover, subpopulation of MSCs were obtained from the bone marrow cultures in fibronectin coated culture dish under low oxygen tension, which could differentiate into neuron and pancreartic islet cells beside standard osteoblast, chondrocyte and adipocyte differentiation. These cells were called marrow isolated adult multipotent inducible (MIAMI) cells (D'Ippolito et al, 2006). However, it is very difficult to predict if bone marrow stromal cells harbor differentially-committed MSC progenitors or their fate is determined by diverse isolation and culture conditions. Since its inception MSCs have become an important tool in tissue engineering and regenerative medicine ranging from generation of cardiomyocytes after a myocardial infarction to restore skeletal damage pertaining to osteoporosis, fracture and osteolytic bone metastases (Kumar et al, 2004; Rabin et al, 2007; Fritz et al, 2008;Chanda et al, 2009). Besides, HSC and endothelial progenitor cells (EPC) enhance MSC function by promoting osteoblastic differentiation and neovascularization respectively at the injury site(s). Therefore, the involvement of HSC and EPC in bone diseases will also be discussed.
Developmental origin of mesenchymal stem cells
According to the International Society of Cellular Therapy (ISCT), human MSC must be positive for CD105, CD73, CD90 and negative for CD45, CD34, CD14 or CD11b, CD79a or CD 19 and HLA-DR and must differentiate into osteoblast, chondrocyte and adipocytes under standard differentiation conditions (Dominici et al, 2006). Based on detailed anatomical mapping, cells with the features and qualities of MSC were detected as early as fetal development and persisted all through postnatal life. Although the exact origin of MSC can be debated, evidence indicates that MSC originates alongwith hematopoietic stem cells and endothelial cells in the aorta gonad mesonephros (AGM) of the developing embryo from the mesothelial cells. MSC obtained from the AGM region showed similar tri-lineage differentiation potential, expressed pluripotential markers like stage-specific embryonic antigen (SSEA), oct-4 and nanog, and share identical immunologic properties besides supporting hematopoiesis similar to MSCs obtained from the adult bone marrow (De-Miguel et al, 2009). Mendes et al, 2005, reported the presence MSCs also in the embryonic circulation. It can be speculated that MSCs might originate during the early embryonic development and migrate to various tissues including bone marrow and reside therein throughout adult life, participating in tissue regeneration, repair and hematopoiesis. Primordial germ cells (PGCs) are derived from the epiblast stem cells and their immigration into the AGM region coincides with the origin of MSC, HSC and endothelial cells. Recent identification of PGC-like cells in the bone marrow suggests an alternative hypothesis that PGC are deposited in the developing organs during embryogenesis which may be the sources of various adult stem cells (De-Miguel et al, 2009). Recently, Crisan and colleagues (2008) proposed that MSCs might also originate from the pericytes.
Immune modulation by the MSC
Immunosuppressive and anti-inflammatory functions have been demonstrated by MSCs in vitro and in vivo. MSC is a rare cell in the bone marrow (1 in 108 cells) and the number and quality of MSCs steadily decline with age (Caplan AI, 2009). MSCs derived from the patient or syngeneic individual (identical siblings) may have the same genetic defect. Further, MSCs isolated from an older individual undergo more adipocytic differentiation and may not be suitable for autologous transplantations. In this situation, use of allogeneic MSC is proposed as a suitable alternative and immunosuppressive and anti-inflammatory functions of MSCs, help them evade elimination by the host immune system. MSC minimally express MHC-I but do not express MHC-II or costimulatory molecules (CD80, CD86, CD40) on their cell surface. MHC-I expression is maintained in the MSC even after differentiation into osteoblast, chondrocyte and adipocytes. IFN-γ can stimulate MHC-II expression in the MSC, but ineffective in stimulating MHC-II expression after tri-lineage differentiation (Chen & Tuan, 2008).
MSCs were shown to inhibit T cell proliferation and activation in response to mitogenic or antigenic stimulation in vitro. MSCs also suppressed maturation and function of both naïve and memory T cells as well as CD4+ and CD8+ T cells in a mixed lymphocyte cultures. MSCs induce T cell anergy by arresting them at the G0-G1 phase of cell cycle and also prevent CD8 T cell mediated lysis (Tse et al, 2003). Several studies have shown that MSCs can suppress the functions of B cells, natural killer cells, dendritic cells and inhibit expression of co-stimulatory ligands on the surface of the antigen presenting cells (Chen & Tuan, 2008).
Factors regulating the homing of MSC to the damaged or tumor tissues
Therapeutic efficacy of MSC depends on efficient mobilization from their bone marrow niches and trafficking through the circulation to the injured or stressed tissue. Cells at the injury site are known to produce various cytokines, chemokines and growth factors which attract the MSCs upon interaction with respective receptors present on their cell surfaces. SDF1-α and its chemotactic receptor, CXCR4 are known to play a very critical role in the trafficking of the hematopoietic stem cells, endothelial progenitor cells and in prostate and breast cancer metastasis to bone and lung (Karp et al, 2009). MSCs express CXCR4 on their surface which allows them to relocate to the damaged tissues following a SDF1-α gradient. AMD-3000 (also known as mozobil, plerixafor) blocks binding of SDF-1α to CXCR4 and has been demonstrated to inhibit recruitment of MSCs to the ischemic heart (Abbott et al, 2004). Apoptotic and necrotic neural and cardiac tissues release hepatocyte growth factor (HGF) which can recruit MSCs in a dose dependent manner through interaction with c-Met, a receptor for HGF. Compromising HGF bioactivity significantly reduced MSC migration (Vogel et al, 2010). The chemokine CCL2 (MCP-1) is also produced at the site of inflammation which recruits MSC expressing the receptor CCR2 (Dwyer et al, 2007). FROUNT is a regulator of CCR2 and facilitates MSC chemotaxis towards the MCP-1 source. Replacement of FROUNT by a truncated version D-FROUNT prevented MSC migration (Belema-Bedada et al, 2008). Another chemokine signaling pathway reported to have significant role in MSC migration and recruitment is CCL25 (TECK) binding CCR9 (Stich et al, 2008).
Vasculogenesis is an important aspect of tissue repair (Kumar et al, 2010). VEGF stimulates MSC mobilization and recruitment to ischemic or damaged tissues although MSCs do not express receptors for VEGF. Ball et al, 2007 reported VEGF-A acts through platelet derived growth factor (PDGF) receptors, which determines the vascular cell fate of MSC. Although chemokine signaling initiates homing of MSCs, it still requires multiple steps for their passage through the blood vessel and extravasate at the site of injury or inflammation. Evidence suggests that the MSCs follow somewhat similar mechanisms utilized by the immune cells to migrate to inflammatory sites. Endothelial transmigration is mediated by active interactions between the molecules (glycoprotein or glycolipids, PSGL-1, L-selectin, integrins, GPCR, LFA-1, Mac-1) expressed on leukocyte surface and their receptors (E-selectin, P-selectin, PNAd, MAdCAM, VCAM-1, ICAMs) on the endothelium (Luster et al, 2005). Once at the site of tissue damage MSCs have to extravasate (diapedesis), which involves transient disassembly of the endothelial junctions and penetration through the underlying basement membrane. MMP2, secreted by the MSCs has been shown to mediate this process. Blocking MMP-2 activity using an antibody or siRNA against MMP-2 or TIMP-3 prevented transendothelial migration of MSCs (De Becker et al, 2007).
Therapeutic application of MSC mostly engage ex vivo expanded cells which show reduced migration and homing potential in vivo. Ex vivo expanded MSC do not express CXCR4 which may compromise their transit to the injury site, although report exists that treatment of these MSC with a cytokine cocktail could restore CXCR4 expression (Shi et al, 2007). Among the family of integrins, α4β1 integrin is a cell surface heterodimer, which mediates cell-cell and cell-extracellular matrix interactions through adhesion to the vascular cell adhesion molecule (VCAM)-1 and to the IIICS region of fibronectin. Evidence for the requirement of α4 integrin for bone marrow engraftment of stem cells has been substantiated in studies. The α4 integrin has been shown to affect homing of not only the hematopoietic stem cells but also of metastatic tumor cells to bone. In our laboratory, cultured MSCs were genetically engineered to overexpress α4 integrin which dimerized with β1 integrin, already in abundance in the MSCs thus forming the biologically active integrin. When these MSCs were administered in a murine model via tail vein resulted in efficient migration into bone (Kumar & Ponnazhagan, 2007, Figure 1). Chemical coupling of sialyl Lewis X moiety on the MSC surface via streptavidinbiotin conjugation were shown in vitro to induce robust MSC rolling response on P-selectin coated substrates (Sarkar et al, 2008). Route of MSC infusion is also a determining factor in efficient homing of MSCs to the region of interest. Infusion of excess MSCs through intravenous (i.v.) route often leads to trapping of cells in the lung, liver and spleen. Intra arterial or intra-cardiac delivery is a better option compared to i.v. infusion as it bypasses the filtering organs (Schrepfer et al, 2007).
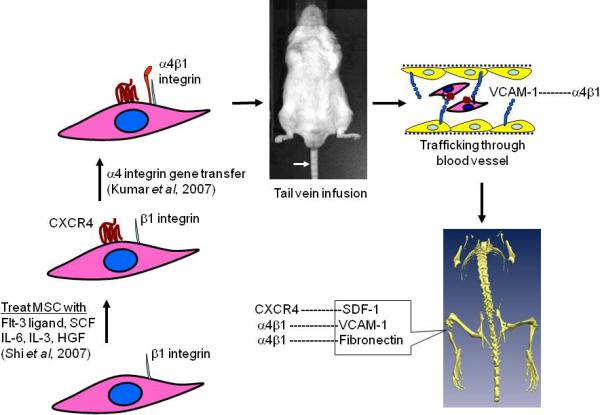
Enhancing bone homing potential of culture expanded MSC: MSC loses CXCR4 receptors ex vivo, thus fail to respond in vivo to a SDF-1α gradient released by the bone marrow cells at the fractured site (s). Pre-treatment of MSC with a cytokine cocktail restores CXCR4 surface expression by the MSC and efficiently migrate to bone. Bone homing capacity of the MSC can be further improved via transduction of α4 integrin (absent in MSC), which dimerizes with β1 integrin (abundant in MSC). α4β1 integrin is expressed on the surface of the MSC, which resulted in efficient transendothelial migration and bone homing via interaction with its ligands, VCAM-1(CD-106) and fibronectin.
Therapeutic application of MSC in bone diseases
Osteogenesis Imperfecta
Osteogenesis imperfecta (OI) is deblitating genetic disorder of MSC characterized by production of defective type 1 collagen, generalized osteopenia which leads to bone deformities, brittle bones with susceptibility to fracture and short stature. Mutation in COL1A1 and COL1A2 collagen genes perturb normal collagen fibril assembly (Pelagiadis et al, 2008). Fetal liver derived, allogeneic and HLA mismatched MSC were injected into the fetus with OI at 32 week through the umbillical vein. The study showed allogeneic MSC of fetal liver origin after transplantation in utero underwent site specific differentiation to bone and persisted for an extended time (Le Blanc et al, 2005). In utero, fetal MSC transplantation into OI mice significantly reduced fracture rates and skeletal abnormalities. MSC delivered systemically to 2 day-old, developing OI mouse engrafted in bones, localized to areas of active bone formation and differentiated into osteoblasts and contributed to bone formation in vivo (Guillot et al, 2008). Children with severe deforming OI were intravenously infused with unmanipulated bone marrow. Representative specimens of trabecular bone showed histological changes indicative of new bone formation. MSC infusion following bone marrow transplantation seems to have enhanced therapeutic effect (Horwitz et al, 2002).
Infantile hypophosphatasia
Infantile hypophosphatasia (IH) is an electrolyte disturbance in which there is an abnormally low level of phosphate in the blood. Biochemically the disease is characterized by deficiency in the tissue-nonspecific isoenzyme alkaline phosphatase (ALP) activity in the serum. ALP deficiency in the osteoblasts impairs skeletal mineralization causing rickets and osteomalacia. An eight month old girl, diagnosed with IH received T cell depleted haplo-identical bone marrow transplantation from her sibling with some clinical improvement. When the disease recurred 6 months after the transplantation, at age 21 months she received a second transplantation with ex vivo expanded bone marrow stromal cells which led to significant and prolonged clinical and radiographic improvement. Sufficient number of donor MSCs were able to engraft which formed functional osteoblasts and chondrocytes with marked improvement of her skeletal disease (Whyte et al, 2003).
Osteoporosis
Osteoporosis affects both sexes with a women to men ratio of 1.6 at some point in their lives and marked by progressive bone loss resulting in osteopenia and high risk of fractures. Decreased bone formation and increased bone resorption are thought to be responsible for the complications of osteoporosis. Adult MSC obtained from osteoporotic mice showed apoptosis in culture (Turgeman et al, 2002). Osteoporosis is more common in postmenopausal women having estrogen (E2) deficiency. E2 replacement therapy restores bone via upregulation of osteogenic genes like alkaline phosphatase, collagen 1, transforming growth factor-β, bone morphogenetic protein-2 and Cbfa-1/Runx-2 in the MSC (Zhou et al, 2001). Administration of BMP-2 was able to increase MSC number, osteogenic activity and decline in rate of osteoblast apoptosis in a number of studies. Kumar et al, 2004 engineered murine MSC to over-express BMP-2 and such modified MSCs showed significant osteoblastic differentiation compared to naïve MSCs in culture. MSCs, overexpressing BMP-2 has been shown to restore bone defects in aged rats (Tsuda et al, 2005).
Angiogenesis is critical to tissue regeneration at the fracture site (Duvall et al, 2007). Delivery of angiogenic factors (VEGF, PDGF and basic FGF) or transplantation of proangiogenic cells (EPC) in the scaffolding materials was helpful but not sufficient. Recently a great deal of interest has been focused on co-localization of the MSC and HSC niches within the bone marrow and their functional interdependence on each other. While HSC promotes osteogenic differentiation of MSC, MSC facilitates the mobilization of HSC (Jung et al, 2008). When HSC and MSC were transplanted together in micropores of 3D calcium phosphate scaffolds, HSC-MSC co-seeded graft yielded marked vascular number and diameter 4 weeks after ectopic implantation in immunodeficient mice. Significant increase in human osteocalcin expression was also observed in the MSCs in comparison to MSC seeded without HSC. VEGF stimulates HSC transformation into endothelial like cells, which formed intercellular tubular structures in vitro (Moioli et al, 2008). MSC concurrently over-expressing BMP-2 and VEGF resulted in enhanced bone regeneration in a murine model of segmental bone defect (Kumar et al, 2010). Osteopontin (OPN) is an ECM protein and regulates angiogenesis, callus formation and mechanical strength in early and late stages of bone remodeling and ECM organization. Studying bone repair in wild type and OPN (-/-) mice using a femoral structure model, Duvall et al, 2007 concluded that OPN deficiency delayed early vascularization, altered matrix organization and late remodeling and reduced biomechanical properties.
Arthritis
Osteoarthritis (OA)
Also known as degenerative arthritis, is a group of diseases and mechanical abnormalities involving degradation of joints, including articular cartilage and subchondral bone next to it. Clinical signs of OA include joint pain, tenderness, stiffness, cracking and locking of joints and local inflammation (Lawrence et al, 2008). Chondrogenic potential of bone marrow derived MSC have been tested in animal models (Grigolo et al, 2009) and humans (Centeno et al, 2008). When MSCs seeded in biodegradable scaffolds were implanted in the knee joints resulted in significant tissue regeneration. The presence of MSCs in the synovial membrane was recently reported. Studies also indicated the MSCs from synovial membranes have greater capacity for chondrogenic differentiation compared to bone marrow MSC (Fan et al, 2009).
Rheumatoid arthritis
TNF-α and IL-6 are pro-inflammatory cytokines and primarily responsible for the complications of rheumatoid arthritis (RA) (Taylor & Feldman, 2009; Nishimoto N, 2010). IL-10 is produced by the T-helper type 2 cells, activated monocytes/macrophages and certain T-regulatory cells (Choi et al, 2008). Systemic injection of MSC, engineered to express IL-10 significantly reduced rheumatoid arthritis symptoms compared to naive MSC (Choi et al, 2008). RA is also accompanied by joint destruction. Several therapeutic approaches also involved autologous chondrocyte implantation or in situ mobilization and recruitment of bone marrow MSCs. But severe inflammatory condition in the joints frequently led to impaired cartilage formation or degeneration of the neocartilage. Allogeneic MSC transplantation significantly reduced the inflammatory state in the joints beside cartilage formation (Ringe & Sittinger, 2009).
Bone metastasis
The ability of MSC to home to sites of tissue injury is well known and the microenvironment of a solid tumor is similar to the milieu of injured or stressed tissue (Dvorak HF, 1986). Most evidence in the recent literature suggested migration of MSCs along with other bone marrow derived cells to the sites of primary tumor and pre-metastatic niche, eventually forming a vital component of tumor stroma (Hall B, 2007). MSCs infused through mouse tail vein were detected exclusively in the A375SM melanomas growing in the lungs (Studeny et al, 2004). The knowledge prompted researchers to test the usefulness of MSCs in targeting and simultaneously delivering anti-tumor agents to the sites of tumorigenesis.
Carcinoma of the prostate, breast, thyroid, lung and kidney are the most common malignancies in adults that preferentially metastasize to bone. Advanced stage breast and prostate cancers relocates in bone in more than 70% of the cases (Jemal A et al, 2009). Majority of the bone metastatic tumors produce osteolytic lesions. The only exception is prostate cancer which results in osteoblastic lesions although there is osteolytic component involved in it for the initial establishment in the bone (Guise et al, 2003). Osteolysis is a major cause of morbidity and mortality in patients with advanced disease and prevention of bone loss has been considered as an effective therapeutic strategy. Primary mechanism for bone destruction in bone metastasis is due to osteoclastic bone resorption. Tumor cells in the bone augment the production of RANKL by osteoblasts and stromal cells, which upon binding to RANK on the surface of preosteoclasts leads to osteoclast activation. Osteoprotegerin (OPG) is a decoy receptor for RANKL and prevents RANK-RANKL interaction (Blair et al, 2006). When MSCs, transduced with a lentivirus encoding human OPG, were delivered systemically were able to inhibit osteoclast activation and prevent trabecular bone loss in a xenogeneic model of KMS-12-BM myeloma bone disease (Rabin et al, 2007). From our own laboratory, we have shown that MSCs, constitutively expressing OPG, was sufficient to prevent osteoclastogenesis and restore trabecular and cortical structure in a SCID mouse model of osteolytic bone metastasis (Chanda et al, 2009, Figure 2 A,B&C). MSCs, genetically engineered to overexpress OPG inspite of having significant therapeutic effect lead to an osteopetrotic condition, which also reduced the marrow space. When similar number of MSCs (5×105) was tested against an established prostate tumor in the bone, therapeutic benefits were apparent but not significant, which suggests the requirement of optimal number of MSCs. Our study also indicated that therapeutic effect by the donor MSCs is imparted by forming woven bone around the growing tumor in the bone (Figure 2D). The formation of woven bone was not due to osteogenic differentiation of MSC by the prostate tumor cells, rather initiated by osteoclastic bone resorption required by the cancer cells to grow in the bone. The protease Urokinase-type plasminogen activator (uPA) aids in the progression of prostate cancer via extracellular matrix degradation, cell motility and angiogenesis. uPA binds to its receptor uPAR by its non-catalytic amino terminal fragment (ATF). ATF by itself does not have any protease activity and may be antagonistic to uPA action. Genetically engineered MSCs expressing ATF when delivered in a mouse model of prostate cancer bone metastasis was able to inhibit tumor growth by inhibiting angiogenesis besides also showing osteogenic potential (Fritz et al, 2008).
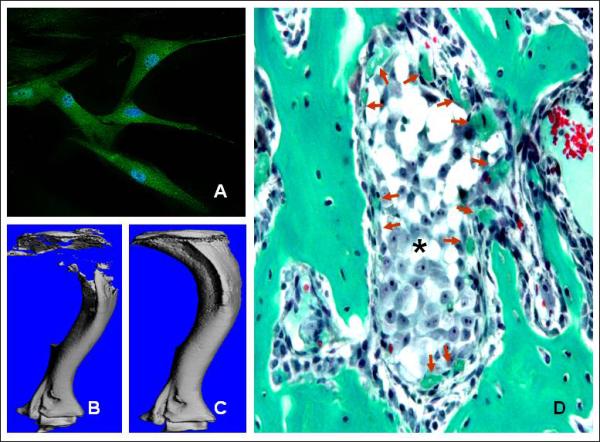
Therapeutic potential of naïve MSC in osteolytic bone metastasis. (A) Immunostaining indicated murine and human MSC constitutively express osteoprotegerin in culture (green fluorescence). (B) Growth of bone metastatic prostate cancer cells (PC3) in the mouse tibia generates severe osteolytic lesions. (C) MSC expressing OPG prevents bone loss via inhibition of osteoclastogenesis which is required by the tumor cells for growth in bone. (D) Besides, MSCs formed woven bone around the tumor nests (*) which further reduced tumor progression. Bone sections were stained with Goldner's trichrome stain and collagen is stained green.
Factors affecting osteogenic differentiation of MSC
Elevated glucose levels or diabetes negatively affect differentiation of bone marrow MSC into osteoblasts which may lead to osteopenia. Insulin can reverse the inhibition of osteogenic differentiation by effectively metabolizing glucose (Gopalkrishnan et al 2006). Rosiglitazone enhances peripheral insulin sensitivity in subjects with type II diabetes and insulin resistance by activating the nuclear receptor PPAR-γ (Gruntmanis et al, 2010). PPAR-γ is a key transcription factor for adipogenic differentiation while inhibits osteoblastogenesis (Soroceanu et al, 2004). Estrogen (E2) deficiency in post menopausal women is also a major contributor of osteoporotic bone loss and E2 replacement therapy prevents osteolysis by upregulating the mRNA expression of osteogenic genes for alkaline phosphatase, collagen, transforming growth facor-β, BMP-2 and Runx-2 (cbfa-1) in the MSCs (Zhou et al, 2001). Insulin growth factor-1 also has been shown to induce bone formation in the aged mouse model of distraction osteogenesis (Fowlkes et al, 2006). Disuse causes rapid loss of skeletal mass and strength of load-bearing bones in young as well as adult animals and humans (Perrien et al, 2007). Communication between MSC and the endothelial cells within the bone marrow is evident. When VEGF stimulated endothelial cells were co-cultured with bone marrow derived MSCs, osteogenic transformation of MSC was inhibited due to inhibition of expression of key transcription factor, osterix (Meury et al, 2006). Parathyroid hormone (PTH) is produced by the parathyroid gland and maintains the levels of calcium in the blood by releasing calcium from bones. While continuous PTH infusion increases bone resorption, intermittent PTH treatment stimulates bone formation by reactivation of quiescent bone surfaces and reducing osteoblast apoptosis (Rickard et al, 2006). Collagen types I, III, and V, syndecan-1, perlecan, fibronectin, laminin, biglycan, and decorin are major components of the extracellular matrix (ECM) produced by the bone marrow cells and play an important role in maintaining MSC stemness (Chen et al, 2007). When MSC were cultured ex vivo on synthetic ECM were able to generate five times more bone in vivo compared to MSC grown without any ECM. Several other factors are known to modulate osteogenic differentiation of MSC. Alcohol has negative impact on bone formation by promoting adipogenesis (Gong & Wezeman, 2004). While demethylating agent azacytidine, rapamycin, histone deacetylase inhibitors decrease proliferation and multiple proliferation potential of MSC (Isomoto et al, 2007; Martin-Rendon et al, 2008; Lee et al, 2009), bisphosphonates (zoledronic acid) and naringin, (a component of the Chinese medicinal plant Rhizoma drynariae) have been shown to promote osteoblastogenesis (Ebert R, 2009; Zhang P, 2009).
Future directions
Increased numbers of MSC have been obtained from peripheral blood of injured mice and their potential to differentiate into osteoblast, chondrocyte and adipocyte is superior to MSC isolated from uninjured mice (Wang et al, 2008). This information was very useful in identifying factors involved in systemic influx of MSC. Pretreatment of mice with VEGF followed by administration of a CXCR4 inhibitor compound AMD 3000 significantly enhanced the bone marrow MSC pool in the peripheral blood making it easier to isolate from a donor individual compared to bone marrow aspirations (Pitchford et al, 2009). Multipotent MSCs are also mobilized into the peripheral blood in laboratory rats exposed to chronic hypoxia (Rochefort, 2006). Wu et al, 2008 reported in a brain injury model, 1×106 cells were sufficient to produce a therapeutic response whereas infusion of 3×106 cells did not enhance any therapeutic efficacy which also breaks the myth that a lot of MSCs are required for tissue repair.
According to the United States National Institute of Health information currently there are only 107 active MSC-based clinical trials and 10% of those trials represent bone related diseases. This is due to several safety concerns that have been raised regarding therapeutic use of MSC in human degenerative diseases especially due to sarcoma transformation (Helman & Meltzer, 2003). Sarcoma is very common in MSC cultured ex vivo for several passages and administration of murine MSC into nude mice resulted in osteosarcoma formation in more than one study (Tolar et al, 2007). Suva et al (2009) isolated a CD133+ sub-population of tumor cells from Ewing's sarcoma which displayed capacity to initiate and sustain tumor growth besides differentiating into mesenchymal lineages in vitro suggesting the possibility of MSCs’ role as cancer stem cell. On a positive note, MSCs reported to generate sarcoma in culture or in vivo were of murine origin. Although human bone marrow derived MSC are reported to attain senescence by 25 passages in culture (Bernardo et al, 2007), extensive analysis should be performed to screen donor MSC for genetic defects before transplantation in patients.
MSCs have been shown to promote tumor growth and metastasis in various studies (Karnoub et al, 2007). Number of MSCs tends to decline with age and tumor cells were found to proliferate more in age old mice than young mice suggesting there is something else besides laying the blame on the MSC. Evidence suggest that healthy tumor microenvironments suppress tumor growth, and it is only after acquiring tumor-like genetic lesions the fibroblasts appear to promote tumor progression (Hall et al, 2007). In our study MSC did not promote or negatively effect proliferation of bone metastatic prostate cancer cells grown in 3-dimension in vitro, rather inhibited tumor growth in vivo by formation of woven bone around the tumor within the tibiae (Chanda et al, 2009). Therefore, taking advantage of tumor homing properties of the MSCs and simultaneously arming them with tumoricidal mechanisms, it will be possible to selectively target solid tumors.
Acknowledgements
Financial support from the National Institutes of Health grants, R01AR050251, R01CA133737, and the U.S. Army Department of Defense grants BC044440 and PC050949 is gratefully acknowledged.
References
- Abbott JD, Huang Y, Liu D, Hickey R, Krause DS, Giordano FJ. Stromal cell-derived factor-1alpha plays a critical role in stem cell recruitment to the heart after myocardial infarction but is not sufficient to induce homing in the absence of injury. Circulation. 2004;110:3300–5. [Abstract] [Google Scholar]
- Ball SG, Shuttleworth CA, Kielty CM. Vascular endothelial growth factor can signal through platelet-derived growth factor receptors. J Cell Biol. 2007;177:489–500. [Europe PMC free article] [Abstract] [Google Scholar]
- Belema-Bedada F, Uchida S, Martire A, Kostin S, Braun T. Efficient homing of multipotent adult mesenchymal stem cells depends on FROUNT-mediated clustering of CCR2. Cell Stem Cell. 2008;2:566–75. [Abstract] [Google Scholar]
- Bernardo ME, Zaffaroni N, Novara F, Cometa AM, Avanzini MA, Moretta A, et al. Human bone marrow derived mesenchymal stem cells do not undergo transformation after long-term in vitro culture and do not exhibit telomere maintenance mechanisms. Cancer Res. 2007;67:9142–9. [Abstract] [Google Scholar]
- Blair JM, Zhou H, Seibel MJ, Dunstan CR. Mechanisms of disease: roles of OPG, RANKL and RANK in the pathophysiology of skeletal metastasis. Nat Clin Pract Oncol. 2006;3:41–9. [Abstract] [Google Scholar]
- Brown P, Hunger SP, Smith FO, Carroll WL, Reaman GH. Novel targeted drug therapies for the treatment of childhood acute leukemia. Expert Rev Hematol. 2009;2:145–158. [Europe PMC free article] [Abstract] [Google Scholar]
- Caplan AI. Why are MSCs therapeutic? New data: new insight. J Pathol. 2009;217:318–24. [Abstract] [Google Scholar]
- Centeno CJ, Busse D, Kisiday J, Keohan C, Freeman M, Karli D. Regeneration of meniscus cartilage in a knee treated with percutaneously implanted autologous mesenchymal stem cells. Med Hypotheses. 2008;71:900–8. [Abstract] [Google Scholar]
- Chanda D, Isayeva T, Kumar S, Hensel JA, Sawant A, Ramaswamy G, et al. Therapeutic potential of adult bone marrow-derived mesenchymal stem cells in prostate cancer bone metastasis. Clin Cancer Res. 2009;15:7175–85. [Europe PMC free article] [Abstract] [Google Scholar]
- Chen FH, Tuan RS. Mesenchymal stem cells in arthritic diseases. Arthritis Res Ther. 2008;10:223. [Europe PMC free article] [Abstract] [Google Scholar]
- Chen XD, Dusevich V, Feng JQ, Manolagas SC, Jilka RL. Extracellular matrix made by bone marrow cells facilitates expansion of marrow-derived mesenchymal progenitor cells and prevents their differentiation into osteoblasts. J Bone Miner Res. 2007;22:1943–56. [Abstract] [Google Scholar]
- Choi JJ, Yoo SA, Park SJ, Kang YJ, Kim WU, Oh IH, et al. Mesenchymal stem cells overexpressing interleukin-10 attenuate collagen-induced arthritis in mice. Clin Exp Immunol. 2008;153:269–76. [Abstract] [Google Scholar]
- Crisan M, Yap S, Casteilla L, Chen CW, Corselli M, Park TS, et al. A perivascular origin for mesenchymal stem cells in multiple human organs. Cell Stem Cell. 2008;3:301–13. [Abstract] [Google Scholar]
- De Becker A, Van Hummelen P, Bakkus M, Vande Broek I, De Wever J, De Waele M, et al. Migration of culture-expanded human mesenchymal stem cells through bone marrow endothelium is regulated by matrix metalloproteinase-2 and tissue inhibitor of metalloproteinase-3. Haematologica. 2007;92:440–9. [Abstract] [Google Scholar]
- De Miguel MP, Arnalich Montiel F, Lopez Iglesias P, Blazquez Martinez A, Nistal M. Epiblast-derived stem cells in embryonic and adult tissues. Int J Dev Biol. 2009;53:1529–40. [Abstract] [Google Scholar]
- D'Ippolito G, Howard GA, Roos BA, Schiller PC. Isolation and characterization of marrow-isolated adult multilineage inducible (MIAMI) cells. Exp Hematol. 2006;34:1608–10. [Abstract] [Google Scholar]
- Dominici M, Le Blanc K, Mueller I, Slaper-Cortenbach I, Marini F, Krause D, et al. Minimal criteria for defining multipotent mesenchymal stromal cells. The International Society for Cellular Therapy position statement. Cytotherapy. 2006;8:315–7. [Abstract] [Google Scholar]
- Duarte RF, Shaw BE, Marín P, Kottaridis P, Ortiz M, Morante C, et al. Plerixafor plus granulocyte CSF can mobilize hematopoietic stem cells from multiple myeloma and lymphoma patients failing previous mobilization attempts: EU compassionate use data. Bone Marrow Transplant. 2010 Mar 22; [Abstract] [Google Scholar]
- Duvall CL, Taylor WR, Weiss D, Wojtowicz AM, Guldberg RE. Impaired angiogenesis, early callus formation, and late stage remodeling in fracture healing of osteopontin-deficient mice. J Bone Miner Res. 2007;22:286–97. [Abstract] [Google Scholar]
- Dvorak HF. Tumors: wounds that do not heal. Similarities between tumor stroma generation and wound healing. N Engl J Med. 1986;315:1650–9. [Abstract] [Google Scholar]
- Dwyer RM, Potter-Beirne SM, Harrington KA, Lowery AJ, Hennessy E, Murphy JM, et al. Monocyte chemotactic protein-1 secreted. by primary breast tumors stimulates migration of mesenchymal stem cells. Clin Cancer Res. 2007;13:5020–7. [Abstract] [Google Scholar]
- Ebert R, Zeck S, Krug R, Meissner-Weigl J, Schneider D, Seefried L, et al. Pulse treatment with zoledronic acid causes sustained commitment of bone marrow derived mesenchymal stem cells for osteogenic differentiation. Bone. 2009;44:858–64. [Abstract] [Google Scholar]
- Eggan K, Jaenisch R. Differentiation of F1 embryonic stem cells into viable male and female mice by tetraploid embryo complementation. Methods Enzymol. 2003;365:25–39. [Abstract] [Google Scholar]
- Fan J, Varshney RR, Ren L, Cai D, Wang DA. Synovium-derived mesenchymal stem cells: a new cell source for musculoskeletal regeneration. Tissue Eng Part B Rev. 2009;15:75–86. [Abstract] [Google Scholar]
- Fowlkes JL, Thrailkill KM, Liu L, Wahl EC, Bunn RC, Cockrell GE, et al. Effects of systemic and local administration of recombinant human IGF-I (rhIGF-I) on de novo bone formation in an aged mouse model. J Bone Miner Res. 2006;21:1359–66. [Europe PMC free article] [Abstract] [Google Scholar]
- Friedenstein AJ, Chailakhjan RK, Lalykina KS. The development of fibroblast colonies in monolayer cultures of guinea-pig bone marrow and spleen cells. Cell Tissue Kinet. 1970;3:393–403. [Abstract] [Google Scholar]
- Fritz V, Noël D, Bouquet C, Opolon P, Voide R, Apparailly F, et al. Antitumoral activity and osteogenic potential of mesenchymal stem cells expressing the urokinase-type plasminogen antagonist amino-terminal fragment in a murine model of osteolytic tumor. Stem Cells. 2008;26:2981–90. [Abstract] [Google Scholar]
- Gong Z, Wezeman FH. Inhibitory effect of alcohol on osteogenic differentiation in human bone marrow-derived mesenchymal stem cells. Alcohol Clin Exp Res. 2004;28:468–79. [Abstract] [Google Scholar]
- Gopalakrishnan V, Vignesh RC, Arunakaran J, Aruldhas MM, Srinivasan N. Effects of glucose and its modulation by insulin and estradiol on BMSC differentiation into osteoblastic lineages. Biochem Cell Biol. 2006;84:93–101. [Abstract] [Google Scholar]
- Grigolo B, Lisignoli G, Desando G, Cavallo C, Marconi E, Tschon M, et al. Osteoarthritis treated with mesenchymal stem cells on hyaluronan-based scaffold in rabbit. Tissue Eng Part C Methods. 2009;15:647–58. [Abstract] [Google Scholar]
- Gruntmanis U, Fordan S, Ghayee HK, Abdullah SM, See R, Ayers CR, et al. The Peroxisome Proliferator-Activated Receptor-gamma Agonist Rosiglitazone Increases Bone Resorption in Women with Type 2 Diabetes: A Randomized, Controlled Trial. Calcif Tissue Int. 2010 Mar 31; [Abstract] [Google Scholar]
- Guillot PV, Abass O, Bassett JH, Shefelbine SJ, Bou-Gharios G, Chan J, et al. Intrauterine transplantation of human fetal mesenchymal stem cells from first-trimester blood repairs bone and reduces fractures in osteogenesis imperfecta mice. Blood. 2008;111:1717–25. [Abstract] [Google Scholar]
- Guise TA, Yin JJ, Mohammad KS. Role of endothelin-1 in osteoblastic bone metastases. Cancer. 2003;97:779–84. [Abstract] [Google Scholar]
- Hall B, Andreeff M, Marini F. The participation of mesenchymal stem cells in tumor stroma formation and their application as targeted gene delivery vehicles. Handb. Exp. Pharmacol. 2007;180:263–283. [Abstract] [Google Scholar]
- Helman LJ, Meltzer P. Mechanisms of sarcoma development. Nat Rev Cancer. 2003;3:685–94. [Abstract] [Google Scholar]
- Horwitz EM, Gordon PL, Koo WK, Marx JC, Neel MD, McNall RY, et al. Isolated allogeneic bone marrow-derived mesenchymal cells engraft and stimulate growth in children with osteogenesis imperfecta: Implications for cell therapy of bone. Proc Natl Acad Sci U S A. 2002;99:8932–7. [Europe PMC free article] [Abstract] [Google Scholar]
- Hsieh MM, Kang EM, Fitzhugh CD, Link MB, Bolan CD, Kurlander R, et al. Allogeneic hematopoietic stem-cell transplantation for sickle cell disease. N Engl J Med. 2009;361:2309–17. [Europe PMC free article] [Abstract] [Google Scholar]
- Isomoto S, Hattori K, Ohgushi H, Nakajima H, Tanaka Y, Takakura Y. Rapamycin as an inhibitor of osteogenic differentiation in bone marrow-derived mesenchymal stem cells. Orthop Sci. 2007;12:83–8. [Abstract] [Google Scholar]
- Jemal A, Siegel R, Ward E, Hao Y, Xu J, Thun MJ. Cancer statistics, 2009. CA Cancer J Clin. 2009;59:225–49. [Abstract] [Google Scholar]
- Jiang Y, Vaessen B, Lenvik T, Blackstad M, Reyes M, Verfaillie CM. Multipotent progenitor cells can be isolated from postnatal murine bone marrow, muscle, and brain. Exp Hematol. 2002;30:896–904. [Abstract] [Google Scholar]
- Jung Y, Song J, Shiozawa Y, Wang J, Wang Z, Williams B, et al. Hematopoietic stem cells regulate mesenchymal stromal cell induction into osteoblasts thereby participating in the formation of the stem cell niche. Stem Cells. 2008;26:2042–51. [Europe PMC free article] [Abstract] [Google Scholar]
- Karnoub AE, Dash AB, Vo AP, Sullivan A, Brooks MW, Bell GW, et al. Mesenchymal stem cells within tumor stroma promote breast cancer metastasis. Nature. 2007;449:557–563. [Abstract] [Google Scholar]
- Karp JM, Leng Teo GS. Mesenchymal stem cell homing: the devil is in the details. Cell Stem Cell. 2009;4:206–16. [Abstract] [Google Scholar]
- Knoepfler PS. Deconstructing stem cell tumorigenicity: a roadmap to safe regenerative medicine. Stem Cells. 2009;27:1050–6. [Europe PMC free article] [Abstract] [Google Scholar]
- Kumar S, Mahendra G, Nagy TR, Ponnazhagan S. Osteogenic differentiation of recombinant adeno-associated virus 2-transduced murine mesenchymal stem cells and development of an immunocompetent mouse model for ex vivo osteoporosis gene therapy. Hum Gene Ther. 2004;15:1197–206. [Abstract] [Google Scholar]
- Kumar S, Ponnazhagan S. Bone homing of mesenchymal stem cells by ectopic alpha 4 integrin expression. FASEB J. 2007;21:3917–27. [Abstract] [Google Scholar]
- Kumar S, Wan C, Ramaswamy G, Clemens TL, Ponnazhagan S. Mesenchymal Stem Cells Expressing Osteogenic and Angiogenic Factors Synergistically Enhance Bone Formation in a Mouse Model of Segmental Bone Defect. Mol Ther. 2010 Jan 12; [Europe PMC free article] [Abstract] [Google Scholar]
- Kuznetsov SA, Mankani MH, Gronthos S, Satomura K, Bianco P, Robey PG. Circulating skeletal stem cells. J Cell Biol. 2001;153:1133–40. [Europe PMC free article] [Abstract] [Google Scholar]
- Lawrence RC, Felson DT, Helmick CG, Arnold LM, Choi H, Deyo RA, et al. Estimates of the prevalence of arthritis and other rheumatic conditions in the United States. Part II. Arthritis Rheum. 2008;58:26–35. [Europe PMC free article] [Abstract] [Google Scholar]
- Le Blanc K, Götherström C, Ringdén O, Hassan M, McMahon R, Horwitz E, et al. Fetal mesenchymal stem-cell engraftment in bone after in utero transplantation in a patient with severe osteogenesis imperfecta. Transplantation. 2005;79:1607–14. [Abstract] [Google Scholar]
- Lee S, Park JR, Seo MS, Roh KH, Park SB, Hwang JW, et al. Histone deacetylase inhibitors decrease proliferation potential and multilineage differentiation capability of human mesenchymal stem cells. Cell Prolif. 2009;42:711–20. [Abstract] [Google Scholar]
- Luster AD, Alon R, von Andrian UH. Immune cell migration in inflammation: present and future therapeutic targets. Nat Immunol. 2005;6:1182–90. [Abstract] [Google Scholar]
- Martin-Rendon E, Sweeney D, Lu F, Girdlestone J, Navarrete C, Watt SM. 5-Azacytidine-treated human mesenchymal stem/progenitor cells derived from umbilical cord, cord blood and bone marrow do not generate cardiomyocytes in vitro at high frequencies. Vox Sang. 2008;95:137–48. [Abstract] [Google Scholar]
- Mendes SC, Robin C, Dzierzak E. Mesenchymal progenitor cells localize within hematopoietic sites throughout ontogeny. Development. 2005;132:1127–36. [Abstract] [Google Scholar]
- Meury T, Verrier S, Alini M. Human endothelial cells inhibit BMSC differentiation into mature osteoblasts in vitro by interfering with osterix expression. J Cell Biochem. 2006;98:992–1006. [Abstract] [Google Scholar]
- Moioli EK, Clark PA, Chen M, Dennis JE, Erickson HP, Gerson SL, et al. Synergistic actions of hematopoietic and mesenchymal stem/progenitor cells in vascularizing bioengineered tissues. PLoS One. 2008;3:e3922. [Europe PMC free article] [Abstract] [Google Scholar]
- Nishimoto N. Interleukin-6 as a therapeutic target in candidate inflammatory diseases. Clin Pharmacol Ther. 2010;87:483–7. [Abstract] [Google Scholar]
- Pelagiadis I, Dimitriou H, Kalmanti M. Biologic characteristics of mesenchymal stromal cells and their clinical applications in pediatric patients. J Pediatr Hematol Oncol. 2008;30:301–9. [Abstract] [Google Scholar]
- Perrien DS, Akel NS, Dupont-Versteegden EE, Skinner RA, Siegel ER, Suva LJ, et al. Aging alters the skeletal response to disuse in the rat. Am J Physiol Regul Integr Comp Physiol. 2007;292:988–96. [Abstract] [Google Scholar]
- Phinney DG, Prockop DJ. Concise review: mesenchymal stem/multipotent stromal cells: the state of transdifferentiation and modes of tissue repair--current views. Stem Cells. 2007;25:2896–902. [Abstract] [Google Scholar]
- Pitchford SC, Furze RC, Jones CP, Wengner AM, Rankin SM. Differential mobilization of subsets of progenitor cells from the bone marrow. Cell Stem Cell. 2009;4:62–72. [Abstract] [Google Scholar]
- Rabin N, Kyriakou C, Coulton L, Gallagher OM, Buckle C, et al. A new xenograft model of myeloma bone disease demonstrating the efficacy of human mesenchymal stem cells expressing osteoprotegerin by lentiviral gene transfer. Leukemia. 2007;21:2181–91. [Abstract] [Google Scholar]
- Rickard DJ, Wang FL, Rodriguez-Rojas AM, Wu Z, Trice WJ, Hoffman SJ, et al. Intermittent treatment with parathyroid hormone (PTH) as well as a non-peptide small molecule agonist of the PTH1 receptor inhibits adipocyte differentiation in human bone marrow stromal cells. Bone. 2006;39:1361–72. [Abstract] [Google Scholar]
- Ringe J, Sittinger M. Tissue engineering in the rheumatic diseases. Arthritis Res Ther. 2009;11:211. [Europe PMC free article] [Abstract] [Google Scholar]
- Rochefort GY, Delorme B, Lopez A, Hérault O, Bonnet P, Charbord P, et al. Multipotential mesenchymal stem cells are mobilized into peripheral blood by hypoxia. Stem Cells. 2006;24:2202–8. [Abstract] [Google Scholar]
- Sarkar D, Vemula PK, Teo GS, Spelke D, Karnik R, Wee le Y, et al. Chemical engineering of mesenchymal stem cells to induce a cell rolling response. Bioconjug Chem. 2008;19:2105–9. [Abstract] [Google Scholar]
- Schrepfer S, Deuse T, Reichenspurner H, Fischbein MP, Robbins RC, Pelletier MP. Stem cell transplantation: the lung barrier. Transplant Proc. 2007;39:573–6. [Abstract] [Google Scholar]
- Shi M, Li J, Liao L, Chen B, Li B, Chen L, et al. Regulation of CXCR4 expression in human mesenchymal stem cells by cytokine treatment: role in homing efficiency in NOD/SCID mice. Haematologica. 2007;92:897–904. [Abstract] [Google Scholar]
- Sorocéanu MA, Miao D, Bai XY, Su H, Goltzman D, Karaplis AC. Rosiglitazone impacts negatively on bone by promoting osteoblast/osteocyte apoptosis. J Endocrinol. 2004;183:203–16. [Abstract] [Google Scholar]
- Stich S, Loch A, Leinhase I, Neumann K, Kaps C, Sittinger M, et al. Human periosteum-derived progenitor cells express distinct chemokine receptors and migrate upon stimulation with CCL2, CCL25, CXCL8, CXCL12, and CXCL13. Eur J Cell Biol. 2008;87:365–76. [Abstract] [Google Scholar]
- Studeny M, Marini FC, Dembinski JL, Zompetta C, Cabreira-Hansen M, Bekele BN, et al. Mesenchymal stem cells: Potential precursors for tumor stroma and targeted delivery vehicles for anticancer agents. J. Natl. Can. Inst. 2004;96:1593–1603. [Abstract] [Google Scholar]
- Suvà ML, Riggi N, Stehle JC, Baumer K, Tercier S, Joseph JM, et al. Identification of cancer stem cells in Ewing's sarcoma. Cancer Res. 2009;69:1776–81. [Abstract] [Google Scholar]
- Taylor PC, Feldmann M. Anti-TNF biologic agents: still the therapy of choice for rheumatoid arthritis. Nat Rev Rheumatol. 2009;5:578–82. [Abstract] [Google Scholar]
- Tolar J, Nauta AJ, Osborn MJ, Panoskaltsis Mortari A, McElmurry RT, et al. Sarcoma derived from cultured mesenchymal stem cells. Stem Cells. 2007;25:371–9. [Abstract] [Google Scholar]
- Tse WT, Pendleton JD, Beyer WM, Egalka MC, Guinan EC. Suppression of allogeneic T-cell proliferation by human marrow stromal cells: implications in transplantation. Transplantation. 2003;75:389–97. [Abstract] [Google Scholar]
- Tsuda H, Wada T, Yamashita T, Hamada H. Enhanced osteoinduction by mesenchymal stem cells transfected with a fiber-mutant adenoviral BMP2 gene. J Gene Med. 2005;7:1322–34. [Abstract] [Google Scholar]
- Turgeman G, Aslan H, Gazit Z, Gazit D. Cell-mediated gene therapy for bone formation and regeneration. Curr Opin Mol Ther. 2002;4:390–4. [Abstract] [Google Scholar]
- Vogel S, Trapp T, Börger V, Peters C, Lakbir D, Dilloo D, et al. Hepatocyte growth factor-mediated attraction of mesenchymal stem cells for apoptotic neuronal and cardiomyocytic cells. Cell Mol Life Sci. 2010;67:295–303. [Abstract] [Google Scholar]
- Wang JA, He A, Hu X, Jiang Y, Sun Y, Jiang J, et al. Anoxic preconditioning: a way to enhance the cardioprotection of mesenchymal stem cells. Int J Cardiol. 2009;133:410–2. [Abstract] [Google Scholar]
- Whyte MP, Kurtzberg J, McAlister WH, Mumm S, Podgornik MN, Coburn SP, et al. Marrow cell transplantation for infantile hypophosphatasia. J Bone Miner Res. 2003;18:624–36. [Abstract] [Google Scholar]
- Wu J, Sun Z, Sun HS, Wu J, Weisel RD, Keating A, et al. Intravenously administered bone marrow cells migrate to damaged brain tissue and improve neural function in ischemic rats. Cell Transplant. 2008;16:993–1005. [Abstract] [Google Scholar]
- Zhang P, Dai KR, Yan SG, Yan WQ, Zhang C, Chen DQ, et al. Effects of naringin on the proliferation and osteogenic differentiation of human bone mesenchymal stem cell. Eur J Pharmacol. 2009;607:1–5. [Abstract] [Google Scholar]
- Zhou S, Zilberman Y, Wassermann K, Bain SD, Sadovsky Y, Gazit D. Estrogen modulates estrogen receptor alpha and beta expression, osteogenic activity, and apoptosis in mesenchymal stem cells (MSCs) of osteoporotic mice. J Cell Biochem Suppl. 2001;36:144–55. [Abstract] [Google Scholar]
Full text links
Read article at publisher's site: https://doi.org/10.1002/jcb.22701
Read article for free, from open access legal sources, via Unpaywall:
https://europepmc.org/articles/pmc2946500?pdf=render
Citations & impact
Impact metrics
Article citations
Mesenchymal stem cells: An efficient cell therapy for tendon repair (Review).
Int J Mol Med, 52(2):70, 30 Jun 2023
Cited by: 6 articles | PMID: 37387410 | PMCID: PMC10373123
Review Free full text in Europe PMC
Cellular Interaction of Bone Marrow Mesenchymal Stem Cells with Polymer and Hydrogel 3D Microscaffold Templates.
ACS Appl Mater Interfaces, 14(11):13013-13024, 13 Mar 2022
Cited by: 12 articles | PMID: 35282678 | PMCID: PMC8949723
Combined Mesenchymal Stem Cells and Cartilage Acellular Matrix Injection Therapy for Osteoarthritis in Goats.
Tissue Eng Regen Med, 19(1):177-187, 13 Jan 2022
Cited by: 3 articles | PMID: 35023025 | PMCID: PMC8782990
The therapeutic potential of mesenchymal stem cells in treating osteoporosis.
Biol Res, 54(1):42, 20 Dec 2021
Cited by: 10 articles | PMID: 34930472 | PMCID: PMC8686520
Review Free full text in Europe PMC
The Combined Use of Platelet-Rich Plasma and Adipose-Derived Mesenchymal Stem Cells Promotes Healing. A Review of Experimental Models and Future Perspectives.
Biomolecules, 11(10):1403, 24 Sep 2021
Cited by: 9 articles | PMID: 34680036 | PMCID: PMC8533225
Review Free full text in Europe PMC
Go to all (71) article citations
Similar Articles
To arrive at the top five similar articles we use a word-weighted algorithm to compare words from the Title and Abstract of each citation.
Bone marrow mesenchymal cells: how do they contribute to tissue repair and are they really stem cells?
Arch Immunol Ther Exp (Warsz), 59(5):369-378, 26 Jul 2011
Cited by: 58 articles | PMID: 21789625
Review
The angiogenic properties of mesenchymal stem/stromal cells and their therapeutic potential.
Br Med Bull, 108:25-53, 23 Oct 2013
Cited by: 146 articles | PMID: 24152971 | PMCID: PMC3842875
Review Free full text in Europe PMC
Adult mesenchymal stem cells for tissue engineering versus regenerative medicine.
J Cell Physiol, 213(2):341-347, 01 Nov 2007
Cited by: 1024 articles | PMID: 17620285
Review
An update on human periapical cyst-mesenchymal stem cells and their potential applications in regenerative medicine.
Mol Biol Rep, 47(3):2381-2389, 06 Feb 2020
Cited by: 7 articles | PMID: 32026284
Review
Funding
Funders who supported this work.
NCI NIH HHS (2)
Grant ID: R01 CA133737
Grant ID: R01CA133737
NIAMS NIH HHS (2)
Grant ID: R01 AR050251
Grant ID: R01AR050251