Abstract
Free full text

Preparing the “Soil”: The Premetastatic Niche
Abstract
Current focus on cancer metastasis has centered on the intrinsic factors regulating the cell autonomous homing of the tumor cells to the metastatic site. Specific up-regulation of fibronectin and clustering of bone marrow–derived cellular infiltrates coexpressing matrix metalloproteinases in distant tissue sites before tumor cell arrival are proving to be indispensable for the initial stages of metastasis. These bone marrow–derived hematopoietic progenitors that express vascular endothelial growth factor receptor 1 mobilize in response to the unique array of growth factors produced by the primary tumor. Their arrival in distant sites represents early changes in the local microenvironment, termed the “premetastatic niche,” which dictate the pattern of metastatic spread. Focus on the early cellular and molecular events in cancer dissemination and selectivity will likely lead to new approaches to detect and prevent metastasis at its earliest inception.
Introduction
Despite its clinical relevance, metastasis remains the most poorly understood aspect of carcinogenesis. Elucidating the complex chain of molecular and cellular events, from onset to establishment, remains paramount to understanding tumor spread. To date, most attention has centered on the changing adhesive and migratory capabilities of tumor cells, but the journey of metastatic tumor cells, from extravasation into the circulation to eventual implantation and survival at distant metastatic sites, is an arduous one. This process requires certain regulatory genes and invasive factors necessary for the egress of primary tumor cells into the circulation and for eventual lodging at distant metastatic sites (1, 2). A growing body of knowledge recognizes the multiple signal transduction pathways, the details of the epithelial-to-mesenchymal transition, and the contribution of cell-to-cell and cell-to-matrix interactions as essential elements of the complex multistep process of metastasis (3). One component in this evolving process that remains particularly perplexing is the tissue-specific pattern of metastatic progression in cancer. Unraveling an explanation for preferential metastasis to specific organs has been ongoing and challenging ever since Paget first introduced the “seed and soil hypothesis” over a century ago (4).
Genetic and Environmental Mediators of Organ-Specific Metastasis
Emerging evidence suggests that genetic regulation and activation of specific chemokines/cytokines and proteases may direct metastasis to a designated organ. In examining subsets of breast tumor cells, Kang et al. (5) and Minn et al. (6) have identified a distinct set of genes that, in combination, can predict metastasis either to lung or to bone compared with the parental cell line, which has a more diverse gene expression profile. For lung metastasis, these specific genes, including inhibitor of differentiation 1 (Id1), VCAM1, matrix metalloproteinase 1 (MMP-1), and CXCL1 represent markers and mediators of tumor cell survival and growth (6). Several studies have also shown that loss of specific genes among cancer cells lends to a greater propensity for metastasis (7). KiSS1, a gene found deleted or rearranged in both melanoma and breast cancer, has its metastatic suppressive effect by reducing MMP-9 expression (8). Several other metastasis-suppressor genes found deleted in tumors allow for acquisition of unique and specific properties that connect tumor cells with the metastatic microenvironment (9). G-coupled proteins linked to their respective ligands provide a mechanism for tumor and tissue site pairing, as has been shown for CXCR4 and its only known ligand CXCL12 [stromal-derived factor 1 (SDF-1)]. CXCR4-expressing breast cancer cells can respond to the high CXCL12 activity in lung tissue, helping to explain the lung specificity seen in breast cancer metastasis (10, 11). Similarly, skin tissue expresses high levels of CCL27, a soluble ligand for the CCR10 chemokine receptor that is highly expressed in melanoma (11). Although the genetic and phenotypic makeup of a tumor contribute to tissue-specific metastasis, it is equally necessary to recognize the involvement of the tumor microenvironment in tumor dissemination. Early changes observed in tissue, before evidence of carcinogenesis—such as early and persistent inflammatory responses, matrix remodeling, increases in reactive oxygen species, and other bioactive oncogenic molecules—suggest that local tissue environmental changes can be a critical component of tissue-specific metastasis. For instance, Hiratsuka et al. (12) have shown alterations in MMPs with the up-regulation of MMP-9 preceding tumor metastasis to the lung. The priming and receptiveness of specific tissues are necessary for the development of a permissive microenvironment for incoming tumor cells, which is essential for metastasis to distant organs.
Bone Marrow Progenitors to the Tumor Microenvironment
Cancer cells and their associated stromal cells secrete a multitude of chemokines that direct the migration, proliferation, and differentiation of the vascular cell network to support the tumor and metastatic microenvironment. For example, vascular endothelial growth factor A (VEGF-A) activates mature, locally embedded endothelial cells as well as bone marrow–derived hematopoietic progenitor cells (HPCs) and endothelial progenitor cells (EPCs; refs. 13–17). In the early developing primary tumor, the recruitment and incorporation of bone marrow–derived VEGF receptor 2+ (VEGFR2+) EPCs into vessels supported by perivascular VEGFR1+ HPCs are required for neovascularization (18). These nascent blood vessels are not dependent on tumor size but rather are induced by circulating tumor-specific chemokines that up-regulate key factors such as Id genes, p27/p130, MMPs, and soluble kit ligand (19–21). These signals promote progenitor cell proliferation and trafficking between niche-dependent compartments in the bone marrow that enable mobilization to the circulation (22, 23). The differential transcriptional regulation of the two VEGF receptors may explain their distinct roles in tumor vessel formation. Factors such as hypoxia, inflammation, ischemia, and accompanying epigenetic and genetic changes in tumors promote the mobilization of bone marrow–derived EPCs and HPCs (13–15). The VEGFR1 promoter, unlike that of VEGFR2, contains a hypoxia-inducible factor-1 consensus binding site acting as an enhancer, which results in up-regulation of VEGFR1 expression (24). VEGFR1-expressing cells have been shown to be essential to extravasation and retention of VEGFR2+ EPCs necessary for neovascularization (25). Previous work has established that bone marrow–derived cells and tumor-associated stromal cells enhance primary tumor neoangiogenesis and growth; however, their contribution to the premetastatic microenvironment and metastatic specificity has not been previously explored.
VEGFR1+ HPCs Define the Premetastatic Niche
By examining the earliest steps before influx of metastatic tumor cells in distant organs, we have defined an essential cellular event leading to the formation of the “premetastatic niche” (26). By flow cytometry and immunofluorescence, these bone marrow–derived cells, labeled with green fluorescent protein (GFP), arrive and form clusters of cells in the tissue parenchyma at common sites of metastasis before evidence of fluorescently labeled red tumor cells. At these sites, bone marrow–derived cells express VEGFR1 and coexpress several other hematopoietic markers, including CD34, CD11b, c-kit, and Sca-1, maintaining their progenitor cell status within the tissue parenchyma in the premetastatic niche. As a result of multiple ongoing events in response to primary tumor chemokines, the VEGFR1+ HPCs proliferate and circulate in the bloodstream but also preferentially localize to areas of increased fibronectin, newly synthesized by resident fibroblasts and fibroblast-like cells, which often reside at the subcapsular region of an organ. The VEGFR1+ HPCs express the integrin VLA-4 (α4β1), allowing them to adhere specifically to the newly synthesized fibronectin for the initiation of cellular cluster formation (Fig. 1, top left; Fig. 1, bottom). The MMP-9 produced by HPCs may degrade the basement membrane, accelerating the extravasation of more VEGFR1+ cells into the niche. This stromal invasion of HPCs into the premetastatic microenvironment possibly represents a pathologic state unto itself with the disruption of the normal physiologic processes in the lung and other tissues. Moreover, Id3 is coexpressed within the premetastatic niche and may be involved with the proliferation and mobilization of HPCs from the bone marrow to distinct sites, maintaining an activated progenitor state within these cellular clusters. The VEGFR1+ HPCs, along with fibronectin and associated stromal cells, alter the local microenvironment, which leads to the activation of other integrins and chemokines such as SDF-1 that promote attachment, survival, and growth of tumor cells. At these established GFP-labeled premetastatic sites, tumor cells tagged with DsRed fluorescence bind and permit progression to micrometastatic lesions (Fig. 1, top middle; Fig. 1, bottom). Soon after the implantation of tumor cells, VEGFR2+ endothelial progenitors are then recruited to promote vasculogenesis, furthering maturation to a fully developed metastatic lesion at the site of the premetastatic niche (Fig. 1, top right). The dependence of the evolving metastatic process on these changes is further illustrated when monoclonal antibodies are used. Inhibiting VEGFR1+ HPCs eliminates the premetastatic niche, and targeting VEGFR2+ EPCs results in the formation of small micrometastasis without vascularization, preventing the formation of full metastatic lesions (26). Similar to carcinoma-associated fibroblasts and tumor-associated macrophages, which promote tumor progression via the creation of a supportive microenvironment, the VEGFR1+ bone marrow–derived cells could foster inflammation and sustain tumor cell growth at distant organ sites (27–29). The bone marrow–derived HPCs forming the premetastatic niche were found in both implanted tumor and spontaneous transgenic tumor models.
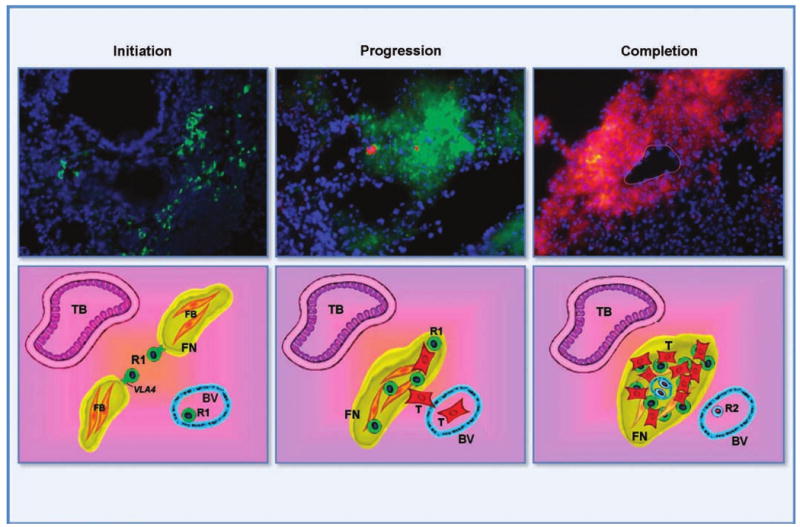
Top row, HPCs from bone marrow niche to premetastatic niche. Initiation, HPCs form cellular clusters in the lung before tumor cell arrival. Progression, migrating tumor cells adhere and grow specifically at these sites. Completion, angiogenic elements, such as VEGFR2+ EPCs, are required for completion of the vascularized metastatic lesion. Green, VEGFR1+ HPCs; red, tumor cells; blue, 4′,6-diamidino-2-phenylindole nuclear staining; yellow, overlap of green VEGFR1 + GFP HPCs and red tumor cells. Circular outline, blood vessel within the metastatic tumor. Bottom row, a schematic of the metastatic process. There is up-regulation of fibronectin by activated fibroblasts followed by attachment of VLA4+ VEGFR1+ HPCs. These cells form clusters that initiate microenvironmental changes necessary for future metastasis. Adhesion and proliferation of tumor cells occur at sites of VEGFR1+ cellular clusters. Influx of endothelial progenitor cells form vasculature to make a mature metastatic lesion. R1, VEGFR1+ HPCs (green); R2, VEGFR2+ CEPs (blue); T, tumor (red); yellow, fibronectin (FN); orange, fibroblasts (FB); TB, terminal bronchiole; BV, bronchiole vein.
Tumor Chemokines May Determine the Tumor Metastatic Profile
Importantly, it is the primary tumor cell with its production of a unique array of tumor chemokines that orchestrates formation of the premetastatic niche and specifically dictates the pattern of tumor spread. Nearly all tumors produce high levels of VEGF, with fewer cancers synthesizing both VEGF and placental growth factor (PlGF, a ligand that binds VEGFR1 exclusively; refs. 30, 31). PlGF/VEGF-derived tumors tend to mobilize and recruit more VEGFR1-expressing cells with resultant-enhanced HPC clustering and metastasis at multiple sites (32, 33). Moreover, PlGF promotes the proliferation of fibroblast-like cells that produce subcapsular fibronectin, the natural ligand for VLA-4+VEGFR1+ HPCs in many organ sites, whereas VEGF promotes newly synthesized fibronectin predominately at the peribronchial and distal capsular regions of the lung. Consequently, a tumor-specific chemokine profile may dictate the patterns of cancer spread. Experiments with the administration of media conditioned by tumor cells show that tumor-secreted factors are sufficient to induce bone marrow–derived cell mobilization and cluster formation. For example, media conditioned by melanoma, high in both PlGF and VEGF-A, which up-regulated newly synthesized fibronectin in many organs, is able to reprogram the metastatic profile of another tumor type such as a lung cancer, high in VEGF-A but low in PlGF (26). With the addition of melanoma-conditioned medium, lung carcinoma was redirected to multiple organs such as spleen and intestine, typical sites of melanoma metastasis. The pattern of cluster formation mimics the pattern of spread specific to that tumor type. Communications between tumor cells and distant tissue sites via circulating factors can prime and direct location of future metastatic tumor growth.
VEGFR1 Activation Promotes Adhesion and Homing of Tumor and Endothelial Cells
Although not well described, VEGFR1 may provide essential directional cues to initiate homing of the circulating endothelial precursors and tumor cells. In embryogenesis, VEGFR1-deficient mice develop excessive endothelial proliferation and overgrowth of immature vessels, resulting in vascular malformations and lethality at E8.5, and suggesting that VEGFR1 plays an essential role in vascular remodeling (34, 35). In adult endothelial cells, VEGFR1 preferentially modulates the reorganization of actin by p38 mitogen-activated protein kinase, eliciting endothelial cell motility (36). Moreover, activated VEGFR1+ HPCs have been shown to release angiogenic factors such as VEGF, angiopoietins, platelet-derived growth factor, and brain-derived neurotrophic factor, which further enhance vessel formation and stability (37, 38). Inhibition of VEGFR1 in primary tumors prevents endothelial cell migration by interfering with the chemotactic response and diminishing perivascular investment (18). Thus, incorporation of bone marrow–derived VEGFR2+ EPCs into rapidly expanding tumors requires corecruitment of VEGFR1+ HPCs to confer stability to the neovessels. In addition, VEGFR1, but not VEGFR2, is responsible for VEGF-A–stimulated migration of monocytes or macrophages that further support the tumor stromal microenvironment (31). For metastasis, VEGFR1 may similarly play a role in migration and proliferation of both tumor and endothelial cells (39). Activation of Src family kinase by VEGFR1-mediated signaling may explain enhanced mobilization of tumor cells (39). This function seems to be critical for the conditioning of the future metastatic site for implantation and growth of tumor cells. Blocking VEGFR1+ HPCs either early on in primary tumor development or following the formation of the premetastatic niche leads to the prevention of the metastatic process (26, 40). In situations where the tumor cells also express VEGFR1, inhibiting this receptor may prove to be a double-edged sword in targeting migrating tumor cells as well as the premetastatic microenvironment (39, 41–43).
Removal of VEGFR1 Microenvironment May Predispose to Tumor Dormancy
VLA4, the essential integrin expressed with VEGFR1, is critical for the assembly of the premetastatic niche. Anti-VLA4 antibodies impair circulating VLA4+VEGFR1+ HPC binding to fibronectin, reducing cluster formation and inhibiting metastatic spread (26). Moreover, we show that in vitro preconditioning with either anti-VLA4 or anti-VEGFR1 antibodies inhibits the proliferation and binding affinity of tumor cells with VEGFR1+ HPCs, illustrating their direct role in adhesion and growth of tumor cells. In addition to these properties, VEGFR1+ cells may release local and systemic chemokines and growth factors that serve as chemoattractants for tumor cells and endothelial cells. In liver sinusoidal endothelial cells, the activation of VEGFR1 results in the paracrine release of hepatocyte growth factor, interleukin-6, and other hepatotrophic molecules that stimulate the proliferation of hepatocytes (44). In the premetastatic niche, SDF-1/CXCR4 chemokine axis participates in the homing and retention of HPCs as well as tumor cells (26). VEGFR1+ HPCs provide the foundation to influence migration, proliferation, and growth of the metastatic tumor. Even late in metastasis, VEGFR1+ cells are present especially at the periphery of the metastatic lesion likely maintaining tumor survival and growth. It is conceivable that without this contribution of VEGFR1+ cells and its supportive microenvironment, there is abatement of tumor growth resulting in possible dormancy until reintroduction of VEGFR1+ HPCs (45).
The Premetastatic Niche: A Novel Predictor of Metastasis
The organ-specific pattern of metastatic spread has been known for centuries. Recent insights into the contribution of the primary tumor microenvironment in tumor progression complement our findings in the premetastatic site. The viable rim of a primary tumor, or “the invasive front,” consists of a unique subset of tumor cells interfacing with bone marrow–derived and organ-specific supportive cells, namely activated fibroblasts and mononuclear cells. This represents a similar paradigm to the premetastatic sites where tumor cells are entering a new microenvironment. Newly introduced tumor cells relying on their unique genetic profile, combined with the genetic and cellular changes in these premetastatic tissue sites, can be powerful in driving metastasis. It is possible that VEGFR1 activation, which leads to increased activity of epithelial-to-mesenchymal transition-associated transcription factors Snail, Twist, and Slug in the primary tumors, may also regulate VEGFR1+ HPCs in the premetastatic niche (46). More effort is necessary to investigate the earliest changes in tumorigenesis and metastasis and will help to increase our understanding of these processes and therefore direct therapies to the most crucial initiating events. Evidence for alterations at the premetastatic niche, such as newly synthesized fibronectin, VEGFR1+ cellular infiltration, and metalloprotease production suggests that research focusing exclusively on the intrinsic properties of tumor cells is insufficient. These findings both compliment and help to explain a new approach to understanding the metastatic process. Further studies will lead to greater knowledge of the genetic underpinnings, which are involved in the establishment of the premetastatic niche. Confirmation of this process in human cancers illustrates the potential use of the premetastatic niche as a surrogate marker for impending metastatic disease and may provide novel targets for therapy, such as neutralizing antibodies against VEGFR1, VLA-4, as well as small-molecule inhibitors for expression of Id genes. Further definition of the molecular and cellular pathways that constitute the premetastatic niche is ongoing and will likely lead to new and improved therapeutic strategies. Novel approaches to detect and prevent metastasis at an earlier stage, before the arrival of tumor cells, may prove most effective in cancer therapeutics, in particular in an adjuvant setting.
Acknowledgments
Grant support: American Society of Clinical Oncology Young Investigator Award, Hope Street Kids fellowship, Association for Research of Childhood Cancer grant, The Doris Duke Charitable Foundation Clinical Scientist Development Award, and the American Hellenic Educational Progressive Association foundation grant (R. Kaplan); and National Cancer Institute grant RO1CA098234, Emerald Foundation, Theodore A. Rapp Foundation, Malcolm Hewitt Wiener Foundation, and Children's Cancer and Blood Foundation (D. Lyden).
References
Full text links
Read article at publisher's site: https://doi.org/10.1158/0008-5472.can-06-2407
Read article for free, from open access legal sources, via Unpaywall:
https://aacrjournals.org/cancerres/article-pdf/66/23/11089/2556793/11089.pdf
Citations & impact
Impact metrics
Article citations
Pre-metastatic niche: formation, characteristics and therapeutic implication.
Signal Transduct Target Ther, 9(1):236, 25 Sep 2024
Cited by: 1 article | PMID: 39317708 | PMCID: PMC11422510
Review Free full text in Europe PMC
Vascular heterogeneity of tight junction Claudins guides organotropic metastasis.
Nat Cancer, 5(9):1371-1389, 17 Sep 2024
Cited by: 0 articles | PMID: 39289595
Cargo-eliminated osteosarcoma-derived small extracellular vesicles mediating competitive cellular uptake for inhibiting pulmonary metastasis of osteosarcoma.
J Nanobiotechnology, 22(1):360, 22 Jun 2024
Cited by: 0 articles | PMID: 38907233 | PMCID: PMC11193292
Mechanism and clinical progression of solid tumors bone marrow metastasis.
Front Pharmacol, 15:1390361, 06 May 2024
Cited by: 0 articles | PMID: 38770000 | PMCID: PMC11102981
Review Free full text in Europe PMC
Role of Hypoxia and Rac1 Inhibition in the Metastatic Cascade.
Cancers (Basel), 16(10):1872, 14 May 2024
Cited by: 2 articles | PMID: 38791951 | PMCID: PMC11120288
Review Free full text in Europe PMC
Go to all (369) article citations
Similar Articles
To arrive at the top five similar articles we use a word-weighted algorithm to compare words from the Title and Abstract of each citation.
VEGFR1-positive haematopoietic bone marrow progenitors initiate the pre-metastatic niche.
Nature, 438(7069):820-827, 01 Dec 2005
Cited by: 1902 articles | PMID: 16341007 | PMCID: PMC2945882
[Bone marrow-derived cells contribute to niche formation in cancer progression].
Clin Calcium, 18(4):480-487, 01 Apr 2008
Cited by: 1 article | PMID: 18379030
Review
Cellular basis of cancer metastasis: A review of fundamentals and new advances.
Acta Histochem, 108(5):327-334, 26 May 2006
Cited by: 43 articles | PMID: 16730054
Review
Cancer biology: emissaries set up new sites.
Nature, 438(7069):750-751, 01 Dec 2005
Cited by: 19 articles | PMID: 16341000
Funding
Funders who supported this work.
Howard Hughes Medical Institute
NCI NIH HHS (2)
Grant ID: R01CA098234
Grant ID: R01 CA098234
NHLBI NIH HHS (14)
Grant ID: R01 HL058707-03
Grant ID: P01 HL067839
Grant ID: P01 HL067839-030004
Grant ID: R01 HL061849-02
Grant ID: R01 HL061849-04
Grant ID: R01 HL061849-05
Grant ID: P01 HL067839-020004
Grant ID: R01 HL058707-04
Grant ID: R01 HL061849
Grant ID: R01 HL061849-03S1
Grant ID: P01 HL067839-010004
Grant ID: P01 HL067839-040004
Grant ID: P01 HL067839-050004
Grant ID: R01 HL061849-03