Abstract
Free full text

Redirection of Silencing Targets by Adenosine-to-Inosine Editing of miRNAs
Abstract
Primary transcripts of certain microRNA (miRNA) genes are subject to RNA editing that converts adenosine to inosine. However, the importance of miRNA editing remains largely undetermined. Here we report that tissue-specific adenosine-to-inosine editing of miR-376 cluster transcripts leads to predominant expression of edited miR-376 isoform RNAs. One highly edited site is positioned in the middle of the 5′-proximal half “seed” region critical for the hybridization of miRNAs to targets. We provide evidence that the edited miR-376 RNA silences specifically a different set of genes. Repression of phosphoribosyl pyrophosphate synthetase 1, a target of the edited miR-376 RNA and an enzyme involved in the uric-acid synthesis pathway, contributes to tight and tissue-specific regulation of uric-acid levels, revealing a previously unknown role for RNA editing in miRNA-mediated gene silencing.
Many developmental and cellular processes are regulated by microRNA (miRNA)–mediated RNA interference (RNAi) (1–4). After incorporation into the RNA-induced silencing complex, miRNAs guide the RNAi machinery to their target genes by forming RNA duplexes, resulting in sequence-specific mRNA degradation or translational repression (1, 2, 4). The generation of mature miRNAs requires the processing of primary transcripts (pri-miRNAs) (5), and A → I RNA editing occurs to certain pri-miRNAs (6–8).
Human chromosome 14 and syntenic regions of the distal end of mouse chromosome 12 harbor the miR-376 cluster of miRNA genes (9). The six human miR-376 RNAs (miR-376a2, -376b, -368, -B1, and -B2) (Fig. 1A) and three mouse miR-376a-c RNAs (fig. S1A) have highly similar sequences (fig. S2). Expression of miR-376 RNAs is detected in the placenta, developing embryos, and adult tissues (9, 10).
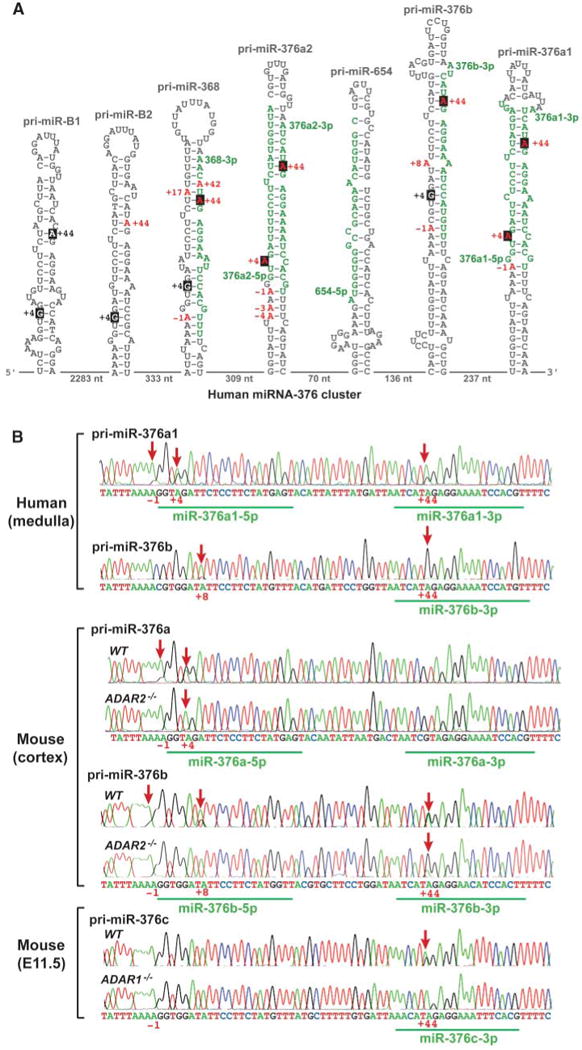
A → I RNA editing of pri–miR-376 RNAs. (A) Hairpin structures of six human pri–miR-376 RNAs are shown. Editing sites (red A's) are numbered with the 5′ end of the human miR-376a1-5p sequence counted as +1. Regions processed into mature miRNAs are highlighted in green. The two most highly edited A's (+4 and +44 sites, red As) are highlighted in black. The genomically encoded G at +4 is also highlighted in black. The genomic distance between miRNA genes is indicated by the numbers at the bottom. (B) Analysis of pri–miR-376a1 and pri–miR-376b RNAs in human and mouse brains (wild-type and ADAR2−/−) and pri–miR-376c RNAs in mouse E11.5 embryos (wild-type and ADAR1−/−) by sequencing of reverse transcription polymerase chain reaction (RT-PCR) products. Thus, an A → I RNA editing site is detected as an A → G change in the cDNA sequencing chromatogram. Editing of human pri–miR-376b at the +44 site is almost 100%, as seen by the presence of a sole G peak without an A peak. Editing sites are indicated by red arrows.
All of the miR-376 RNA cluster members are transcribed into a long primary transcript encompassing the entire region and (except human miR-B1) undergo extensive and simultaneous A → I editing at one or both of two specific sites (+4 and +44) in select human and mouse tissues and specific subregions of the brain (Fig. 2 and table S1) (11). The +4 site of some pri–miR-376 cluster genes (e.g., human -376b and -368) is genomically encoded as G and thus not subject to A → I editing (Fig. 1A). Certain miR-376 members, such as pri–miR-376a2, -376b, and -368, are nearly 100% edited at the +44 site in the human cortex and medulla (Figs. 1B and and22 and table S1), whereas no editing was detected in other tissues (e.g., the +4 site of human pri–miR-376a1 in liver and the +44 site of mouse pri–miR-376a in all tissues). In select members of the cluster, substantial editing (~20 to 55%) occurs at the −1 site, and infrequent editing occurs at several additional sites (table S1). In contrast, no editing was detected in human pri–miR-654 and mouse pri–miR-300. Although these two pri–miRNAs are located within the miR-376 cluster, their sequences are very different from those of miR-376 family members (fig. S2), indicating the strict selectivity of the editing machinery for pri–miR-376 family members and for specific A residues (+4 or +44 sites) within their foldback hairpin structures.
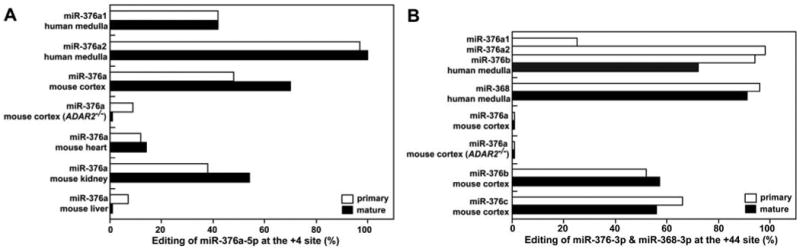
Characterization of edited pri– and mature miR-376 RNAs in various tissues. (A) Editing frequency of pri– and mature miR-376a RNAs (5p strand) at the +4 site from various human and mouse tissues. (B) Editing frequency of pri– and mature miR-376a-c and miR-368 RNAs (3p strand) at the +44 site from subregions of human and mouse brains. The human mature miR-376a1-3p, miR-376a2-3p, and miR-376b-3p are not distinguishable in our assay, so the observed editing frequency represents the average of these three isoforms. [(A) and (B)] The editing frequency of pri-miRNAs is the ratio of the G peak over the sum of the G and A peaks of the sequencing chromatogram of RT-PCR products. Two separate measurements resulted in identical values. The editing frequency of mature miRNAs is a ratio of the cDNA clones containing the A → G change over the total cDNA clones examined (>50 isolates).
Two adenosine deaminases acting on RNA (ADARs), ADAR1 and ADAR2, are known to be involved in A → I editing (12–15). ADAR2−/− mice are viable (16), whereas ADAR1−/− mouse embryos die at embryonic day 12.0 (E12.0) (17, 18). Analysis of RNA extracted from the brain cortices of ADAR2−/− mice and ADAR1−/− mouse E11.5 embryos revealed differences in the pri–miR-376 sites edited by ADAR1 and ADAR2. Editing of the −1 site of pri–miR-376a, pri–miR-376b, and pri–miR-376c, as well as the +4 site of pri–miR-376a, is almost eliminated in the cortex of ADAR2−/− mice. In contrast, the frequency of editing at the +44 site of pri–miR-376b and -376c is higher in ADAR2−/− mice, whereas editing of the +44 site was eliminated in ADAR1−/− embryos (Fig. 1B and table S1). The results indicate that the −1 and +4 sites are mainly edited by ADAR2. The +44 site is selectively edited by ADAR1. ADAR2, if coexpressed with ADAR1, appears to suppress ADAR1 activity.
Editing of pri-miRNAs could be biologically important by virtue of an effect on either the level of expression or on the function of miRNAs (6–8, 13). Characterization of complementary DNA (cDNA) sequences corresponding to miR-376 cluster members revealed that the edited forms of mature miR-376 RNAs are highly expressed in certain tissues (Fig. 2). For instance, 41% of the miR-376a1-5p and 92% of the miR-368-3p molecules were edited at the +4 site and at the +44 site, respectively, in human medulla oblongata. In wild-type mouse cortex, 56% of miR-376c-3p molecules were edited at the +44 site, whereas 54% of the miR-376a-5p molecules were edited at the +4 site in wild-type mouse kidney. As expected from the editing frequency of pri–miR-376a2 RNA (98%), only the edited version of mature miR-376a2-5p was detected in the human medulla. Thus, editing does not affect the processing steps required for expression of mature miR-376 RNAs (Fig. 2).
Both of the major editing sites in pri–miR-376 RNAs (+4 and +44) are located within the functionally critical 5′-proximal “seed” sequences of miR-376-5p and -3p, suggesting that edited mature miR-376 RNAs may target genes different from those targeted by the unedited miR-376 RNAs. To investigate whether a single A → I base change at the +4 site of miR-376a-5p (which has identical human and mouse forms) would affect the selection of its target genes, we used an in-house computational algorithm, Diana-MicroT2, that predicts miRNA-to-target interactions, followed by the application of a species-conservation filter. We further filtered the predictions to retain only genes with multiple 3′ untranslated-region (UTR) target sites, with at least one of the sites being conserved. This filter yielded 78 target genes for unedited miR-376a-5p and 82 target genes for edited miR-376a-5p (conserved between human and mouse), with only two in common (fig. S3A and table S2). We randomly selected three unedited-version targets [SFRS11 (arginine/serine-rich splicing factor 11), SLC16A1 (solute carrier family 16-A1), and TTK (threonine and tyrosine kinase)] and three targets of the edited version [PRPS1 (phosphoribosyl pyrophosphate synthetase 1), ZNF513 (zinc finger protein 513), and SNX19 (sorting nexin 19)] for experimental verification (fig. S3B).
Luciferase expression was examined in HeLa cells cotransfected with reporter constructs containing the target sites for unedited or edited miR-376a-5p in their 3′UTRs (fig. S3C), together with unedited or edited miR-376a-5p RNAs. First, we used the edited miR-376a-5p RNA that had a G residue substituted for A at the +4 site. Specific repression of the edited-version and unedited-version target genes by edited and unedited miR-376a-5p RNAs, respectively, was observed (Fig. 3A). This selective silencing must be due to binding of edited (or unedited) miR-376a-5p RNAs specifically to their predicted target sites, because reciprocal cotransfection experiments resulted in no significant silencing (Fig. 3A). Experiments with the edited miR-376a-5p RNA that had an I residue at the +4 site again resulted in specific repression of the edited-version targets (Fig. 3B), revealing an equivalent contribution of I:C and G:C base pairs for hybridization of miRNAs to their targets. The A residue at the +4 site of unedited miR-376a-5p pairs with U residues of the unedited-version targets, whereas the I residue of the edited isoform pairs with C residues of the edited-version targets (fig. S3B). Our results suggest that a single A → I base change is sufficient to redirect silencing miRNAs to a new set of targets.
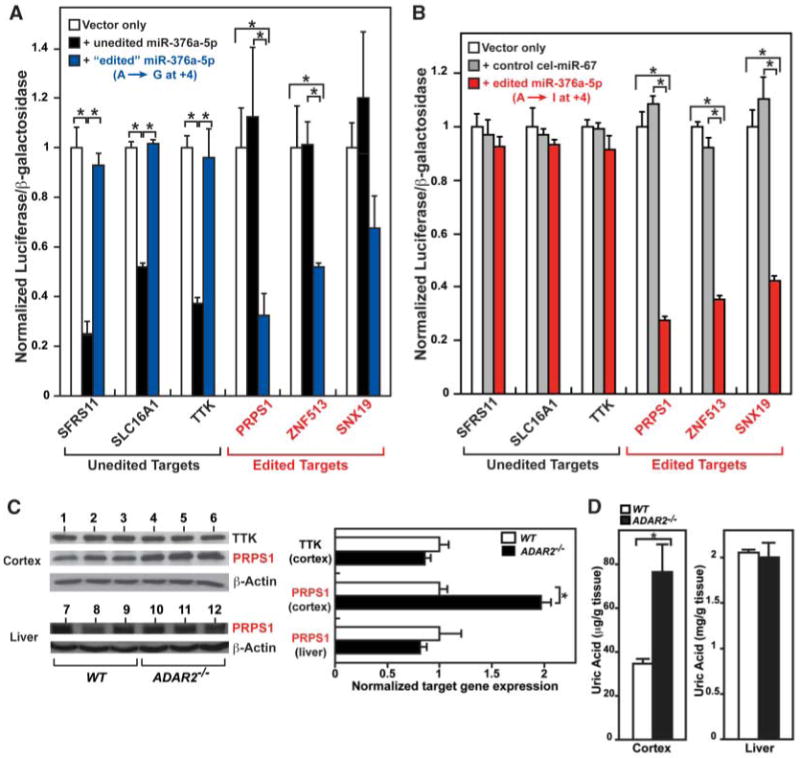
Physiological effects of miR-376a RNA editing. (A) Confirmation of the edited miR-376a targets. Relative luciferase activities in HeLa cells cotransfected with unedited miR-376a-5p (black bars) and edited miR-376a-5p (A → G at the +4 site) (blue bars), respectively. Reciprocal experiments were also conducted; for example, miR-376a-5p edited-version target sites challenged by unedited miR-376a and vice versa. (B) Luciferase activities were examined in HeLa cells cotransfected with a negative control cel-miR-67 (gray bars) and edited miR-376a-5p (A → I at the +4 site) (red bars), respectively. [(A) and (B)] The luciferase activities were compared statistically by Mann-Whitney U tests. Significant differences are indicated by asterisks, P < 0.05. Error bars, SEM (n = 3, where n is the number of independent measurements taken). (C) Western blot analysis of TTK and PRPS1 expression levels in the wild-type and ADAR2−/− mouse cortex and liver. Significant differences are indicated by asterisks, P < 0.05. Error bars, SEM (n = 3). (D) Uric-acid levels in the wild-type and ADAR2−/− mouse cortex and liver. Significant difference is indicated by an asterisk, P < 0.01. Error bars, SEM (n = 6).
To confirm the in vivo effects of miR-376 RNA editing, we measured endogenous expression levels of unedited-version (TTK) and edited-version (PRPS1) miR-376a-5p target genes in wild-type and ADAR2−/− mouse cortices. The +4 site of miR-376a-5p is edited by ADAR2 almost exclusively (Fig. 1B). No edited mature miR-376a-5p is expressed in ADAR2−/− mice, whereas both unedited and edited miR-376a-5p is expressed in the brain cortex, heart, and kidney of wild-type mice (Fig. 2A). Only unedited mature miR-376a-5p RNAs were detected in the liver of wild-type mice because of an almost total lack of pri-miR376a RNA editing in this tissue (Fig. 2A and table S1). PRPS1, which contains multiple target sites for the edited version of miR-376a-5p within its 3′UTR, had levels that were almost two times lower in the wild-type mouse cortex than in the ADAR2−/− mouse cortex. In contrast, no difference in PRPS1 expression was detected between the wild-type and ADAR2−/− liver (Fig. 3C). We also confirmed that the expression level of total miR-376a-5p is not significantly different between wild-type and ADAR2−/− tissues by primer-extension analysis. Thus, the edited miR-376a-5p, expressed only in select tissues of wild-type mice, does indeed repress this target gene in a tissue-specific manner (Fig. 3C). No significant difference in TTK expression was detected between wild-type and ADAR2−/− cortices. The results indicate that unedited miR-376a-5p RNAs are sufficient to regulate this particular target gene, despite a threefold difference in expression between wild-type and ADAR2−/− mice (Fig. 2A).
PRPS1 is an essential housekeeping enzyme involved in purine metabolism and the uric-acid synthesis pathway. An X-chromosome–linked human disorder characterized by gout and neurodevelopmental impairment with hyperuricemia is caused by a two- to fourfold increase of PRPS1 levels, indicating the requirement for tight control of PRPS1 levels and activities (19). In order to confirm the biological importance of miR-376 editing, we examined uric-acid levels and found that tissue-specific repression of PRPS1 levels was indeed reflected in a twofold increase in uric-acid levels in ADAR2-null cortex (Fig. 3D). Thus, editing of miR-376a appears to be one of the mechanisms that ensure tight regulation of uric-acid levels in select tissues such as the brain cortex.
A systematic survey of human pri-miRNA sequences identified A → I editing sites in ~6% of all pri-miRNAs examined (6). However, this estimate may be too low (6): In vitro editing studies of randomly selected pri-miRNAs predict that as much as 50% of all pri-miRNAs may have specific A → I editing sites (8). Although expression of only one edited viral miRNA [miR-K12-10b (Kaposi sarcoma-associated virus miRNA)] has been reported previously (20), we predict that many additional edited isoforms of cellular miRNAs are likely to be identified in the future.
Acknowledgments
This work was supported in part by grants from NIH, the Juvenile Diabetes Research Foundation, the Commonwealth Universal Research Enhancement Program, and the Pennsylvania Department of Health (K.N.); and by a grant from NSF (AG.H.). P.S. is also supported by a predoctoral NIH training grant. We thank M. Higuchi and P. H. Seeburg for ADAR2−/− mice, Q. Wang for mouse embryo RNAs, Z. Mourelatos and J. M. Murray for reading and comments, and S. Lui and U. Samala for technical assistance.
Footnotes
Supporting Online Material: www.sciencemag/org/cgi/content/full/315/5815/1137/DC1
Materials and Methods
Fig. S1 to S3
Tables S1 to S3
References
References and Notes
Full text links
Read article at publisher's site: https://doi.org/10.1126/science.1138050
Read article for free, from open access legal sources, via Unpaywall:
https://europepmc.org/articles/pmc2953418?pdf=render
Citations & impact
Impact metrics
Citations of article over time
Alternative metrics
Article citations
Global A-to-I RNA editing during myogenic differentiation of goat MuSCs.
Front Vet Sci, 11:1439029, 09 Oct 2024
Cited by: 0 articles | PMID: 39444736 | PMCID: PMC11496035
Programmable editing of primary MicroRNA switches stem cell differentiation and improves tissue regeneration.
Nat Commun, 15(1):8358, 27 Sep 2024
Cited by: 0 articles | PMID: 39333549 | PMCID: PMC11436717
Exosomal miRNAs and isomiRs: potential biomarkers for type 2 diabetes mellitus.
Precis Clin Med, 7(3):pbae021, 20 Sep 2024
Cited by: 0 articles | PMID: 39347441 | PMCID: PMC11438237
Review Free full text in Europe PMC
Loss of ADAR1 protein induces changes in small RNA landscape in hepatocytes.
RNA, 30(9):1164-1183, 16 Aug 2024
Cited by: 0 articles | PMID: 38844344 | PMCID: PMC11331409
Spatial Visualization of A-to-I Editing in Cells Using Endonuclease V Immunostaining Assay (EndoVIA).
ACS Cent Sci, 10(7):1396-1405, 08 Jul 2024
Cited by: 0 articles | PMID: 39071059 | PMCID: PMC11273454
Go to all (519) article citations
Data
Data behind the article
This data has been text mined from the article, or deposited into data resources.
BioStudies: supplemental material and supporting data
Similar Articles
To arrive at the top five similar articles we use a word-weighted algorithm to compare words from the Title and Abstract of each citation.
Adenosine-to-Inosine Editing of MicroRNA-487b Alters Target Gene Selection After Ischemia and Promotes Neovascularization.
Circ Res, 122(3):444-456, 28 Dec 2017
Cited by: 42 articles | PMID: 29284691
Frequency and fate of microRNA editing in human brain.
Nucleic Acids Res, 36(16):5270-5280, 06 Aug 2008
Cited by: 223 articles | PMID: 18684997 | PMCID: PMC2532740
RNA editing of the microRNA-151 precursor blocks cleavage by the Dicer-TRBP complex.
EMBO Rep, 8(8):763-769, 22 Jun 2007
Cited by: 249 articles | PMID: 17599088 | PMCID: PMC1978079
A-to-I editing of coding and non-coding RNAs by ADARs.
Nat Rev Mol Cell Biol, 17(2):83-96, 09 Dec 2015
Cited by: 543 articles | PMID: 26648264 | PMCID: PMC4824625
Review Free full text in Europe PMC
Funding
Funders who supported this work.
NCI NIH HHS (2)
Grant ID: P01 CA072765-050002
Grant ID: P01 CA072765
NHLBI NIH HHS (2)
Grant ID: R01 HL070045
Grant ID: R01 HL070045-04
NIGMS NIH HHS (2)
Grant ID: R01 GM040536-16
Grant ID: R01 GM040536