Abstract
Free full text

Initiation context modulates autoregulation of eukaryotic translation initiation factor 1 (eIF1)
Abstract
The central feature of standard eukaryotic translation initiation is small ribosome subunit loading at the 5′ cap followed by its 5′ to 3′ scanning for a start codon. The preferred start is an AUG codon in an optimal context. Elaborate cellular machinery exists to ensure the fidelity of start codon selection. Eukaryotic initiation factor 1 (eIF1) plays a central role in this process. Here we show that the translation of eIF1 homologs in eukaryotes from diverse taxa involves initiation from an AUG codon in a poor context. Using human eIF1 as a model, we show that this poor context is necessary for an autoregulatory negative feedback loop in which a high level of eIF1 inhibits its own translation, establishing that variability in the stringency of start codon selection is used for gene regulation in eukaryotes. We show that the stringency of start codon selection (preferential utilization of optimal start sites) is increased to a surprising degree by overexpressing eIF1. The capacity for the cellular level of eIF1 to impact initiation through the variable stringency of initiation codon selection likely has significant consequences for the proteome in eukaryotes.
Translation initiation in eukaryotes is substantially different from that in bacteria. The standard mode involves scanning of a complex involving the small ribosomal subunit from the 5′ cap to the initiation codon. There is limited reinitiation following translation of a coding sequence (1, 2). Genetic and biochemical studies have revealed several eukaryotic factors involved in selecting the correct initiation codon (3–6). Further analyses pointed toward eukaryotic initiation factor 1 (eIF1) as the key mediator of this process (7–10). eIF1 binds near the P-site of the small ribosomal subunit (11); this binding is thought to lead to an open conformation of the preinitiation complex favoring scanning (12, 13). Release of eIF1 leads to formation of a closed conformation favoring initiation; dissociation of eIF1 from the preinitiation complex is the commitment step in start-codon selection (4). Both in vitro and in vivo experiments have shown that eIF1 is crucial for discrimination between initiation at AUG or near-cognate non-AUG codons (4, 6–8).
Work accomplished nearly 30 y ago showed that, in mammals, strong bias exists for the six nucleotides preceding the preferred initiation AUG and the nucleotide following it (14). Subsequently, it was demonstrated that sequences in this region determine the efficiency of initiation at an AUG (15). The sequence GCC(A/G)CCAUGG, referred to as the Kozak consensus, is optimal for initiation; the underlined nucleotides at positions −3 and +4 (relative to the +1 A of AUG, shown in italics) are most important for efficient initiation (15).
In vitro, eIF1 is crucial for discriminating between AUG codons in poor and good initiation contexts and also for discriminating against AUGs that are too close to the mRNA 5′-end (7). Bacterial initiation factor 3 (IF3) is a functional analog of eIF1 in that, like eIF1, it has the ability to discriminate between initiation at AUG and near-cognate non-AUG codons. Even though they are not homologs, eIF1 and IF3 can functionally substitute each others’ ability to dissociate aberrant initiation complexes in the heterologous system (16).
Autoregulation of the synthesis of translation factors is often observed (17). Initiation of IF3 translation begins with an AUU codon that is an important component of a negative feedback regulatory loop. High levels of IF3 protein increase discrimination against initiation at its AUU start codon, which leads to reduced synthesis of the protein (18, 19). The present work addresses whether a counterpart autoregulatory mechanism controls initiation at the eIF1 start codon.
Results
The Initiation Codon of eIF1 in Diverse Eukaryotes Has Poor Initiation Context.
To investigate whether eIF1 might be subject to autoregulation similar to that of IF3 in bacteria, the initiation site of eIF1 was analyzed. Sequences from more than 500 eIF1 homologs were assembled and compared. eIF1 ORFs are relatively short (110–120 codons). The majority have a single AUG initiation codon and no other downstream in-frame AUG. A minority have one or more additional in-frame AUG codons present in the second half of the ORF that are unlikely to initiate translation of a functional protein. Examination of the eIF1 sequences precluded the possibility of non-AUG initiation for eIF1 that would be analogous to the AUU initiation for bacterial IF3. The data indicate that the first in-frame AUG is the sole initiation codon available for translation of the eIF1-coding region.
The context of the eIF1 AUG initiator revealed a startling conserved pattern that deviates dramatically from the optimal consensus. In almost every case, the initiation context appears to be very poor. Of 233 metazoan eIF1 sequences examined, 217 (93%) lack both a purine at −3 and a G at +4 (Fig. 1A and Fig. S1A); this is also the case for 88 of 91 (97%) fungal sequences examined (Fig. 1B and Fig. S1B) and for 220 eIF1 sequences (Fig. 1C and Fig. S1C) from the plant kingdom examined. The disparity between eIF1 context and preferred context is also observed when the specific contexts for each individual species are compared (Fig. S2). We found that some flowering plants have an additional eIF1 paralog, which is apparently under relaxed selective pressure and likely has a nonredundant function. Its sequence has a conserved +4 G (Fig. S3). During the preparation of this manuscript, another group reported similar conservation of poor initiation context on the basis of an analysis of seven eIF1 eukaryotic homologs (20).
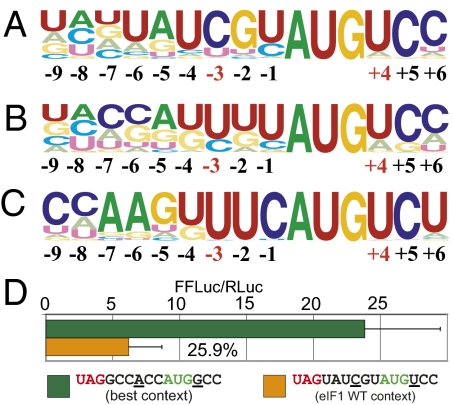
Starting codon context of eIF1 genes is poor, which leads to inefficient initiation. (A) Logo representation of the initiation context of 233 metazoan eIF1 homologs. (B) Representation of the initiation context of 91 fungal eIF1 homologs. (C) Representation of the initiation context of 220 plant eIF1 homologs (for discussion of excluded plant paralogs, see legend for Fig. S3). Letter heights are proportional to the frequency of conservation. The consensus conservation is shown at the top of each logo; the position relative to the A of the AUG start codon is indicated below (crucial positions −3 and +4 are in red). Alignments of the sequences used to generate the logogram are shown in Fig. S1. (D) Relative initiation efficiency of consensus mammalian initiation context and human EIF1 initiation context (arbitrary units). The results are derived from dual luciferase transfection experiments as described in Materials and Methods.
Humans have two paralogs of eIF1 (EIF1 and EIF1B); both have poor initiation contexts. We used human EIF1 as a representative example to study the potential translational regulation of eIF1. The human EIF1 initiation site is UAUCGUAUGUCC, which is identical to the metazoan eIF1 consensus (Fig. 1A). To investigate the translation initiation potential of different codons in different sequence contexts, sequences were positioned to enable the possibility of initiating translation of a firefly luciferase reporter in a vector that was transfected into HEK-293T cells. As expected, the wild-type native initiation context of EIF1 initiation appeared inefficient because a reporter initiating with AUG in this context was expressed fourfold less well than a reporter initiating with AUG in the consensus Kozak context (Fig. 1D).
Overexpression of eIF1 Protein Leads to Autoregulatory Repression.
If the native EIF1 initiation site were a sensor for negative feedback regulation, then initiation from this site should become increasingly inefficient as the intracellular concentration of eIF1 increases. This prediction was tested by cotransfecting a plasmid expressing exogenous human EIF1 initiated by AUG in different contexts and a plasmid expressing a firefly reporter that has an initiation context identical to that of native human EIF1 (see Fig. 2A for diagram). The initiation codon of the exogenous EIF1 was placed in either of three contexts: (i) native context (eIF1 WT: Fig. 2B, lanes 1 and 2), (ii) optimal consensus context (eIF1 good: Fig. 2B, lanes 3 and 4), and (iii) a near-consensus context that enabled preservation of the identity of the second incorporated amino acid (eIF1 good*: Fig. 2B, lanes 5 and 6). Western blot analysis indicated that exogenous EIF1 expressed in its native initiation context led to a minimal visible increase of eIF1 protein levels compared with cells containing only endogenous eIF1 (compare lane 1 and 2 to lane 7 in Fig. 2B), but nonetheless the difference in eIF1 levels reproducibly affected the translation of luciferase reporters initiated from poor contexts (see below). These levels did not increase when the amount of transfecting DNA was increased 10-fold (compare lane 1 to lane 2 in Fig. 2B). In contrast, exogenous EIF1 expressed with the AUG in consensus context (eIF1 good) or in near-consensus context (eIF1 good*) led to overexpression of the eIF1 protein, and this overexpression increased further when the amount of transfecting DNA was increased (lanes 3–6 in Fig. 2B). These results are consistent with eIF1 overexpression leading to reduced initiation from the native-context EIF1 AUG. The levels of eIF1 protein initiated by an AUG in consensus or near-consensus contexts were not correspondingly repressed when the level of transfected DNA increased, indicating that the negative feedback loop was not active when the initiation context was improved.
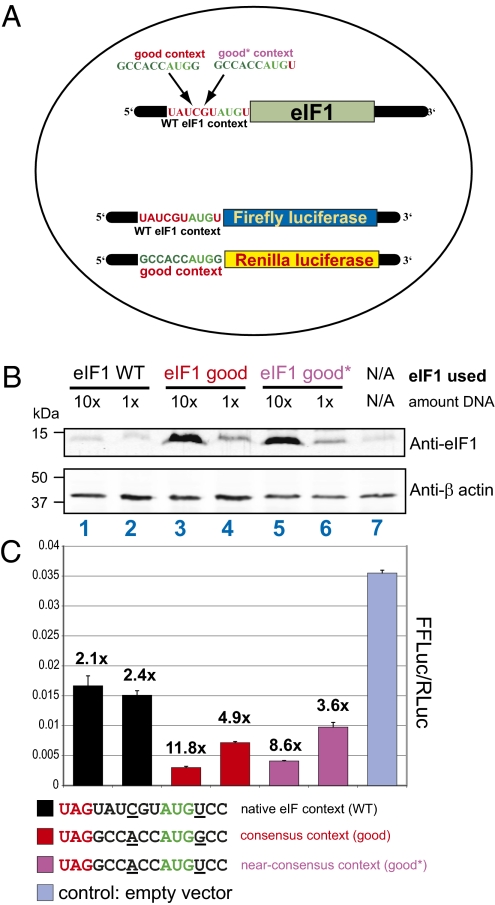
Overexpression of eIF1 is autoregulatory. (A) Schematic of the triple transfections used in this experiment. The initiation context for each expressed exogenous mRNA is indicated. (B) Western blot of protein lysates from cells transfected with vectors expressing human eIF1. The type of initiation context of each eIF1 construct is indicated above the actual sequence. Black type: EIF1 initiated by the native initiation context; red type: EIF1 initiated by perfect Kozak consensus context; maroon type: EIF1 initiated by near-consensus context that preserves the wild-type EIF1 coding sequence. In each case, the context is bracketed upstream by an in-frame stop codon blocking potential translation initiation further 5′. In lanes marked “10×”, 10-fold more vector with insert was transfected compared with lanes marked “1×,” where the difference is made up with inert vector. Anti-β-actin antibody is used to control for loading differences. The control cells are transfected with the same amount of inert vector. (C) Ratio from dual luciferase measurements from the same cells for which Western analysis is shown in B. The firefly reporter in all cotransfections is initiated with an AUG in the native (wild-type) human EIF1 context. Renilla luciferase is initiated by an AUG in the consensus (good) context. The fold reduction of firefly reporter activity (normalized to Renilla reporter activity) relative to firefly reporter expression in the empty vector control cells is shown above each column.
These findings and conclusions were corroborated in a quantitative manner by measuring relative firefly luciferase activity from the cotransfected firefly luciferase reporter whose initiator AUG was in the native EIF1 context (Fig. 2C). The results clearly showed that increased levels of eIF1 protein decreased initiation of the firefly luciferase reporter. In cells cotransfected with the highest amount of vector that expresses eIF1, initiated from AUG in a consensus context (eIF1good), synthesis of the reporter was reduced nearly 12-fold (Fig. 2C).
Overexpression of eIF1 Protein Leads to Reduced Initiation from AUG Codons in Poor Contexts and from Noncanonical Initiation Codons.
The effect of eIF1 levels on the initiation at AUG codons in different contexts was next investigated systematically. The AUG codon for firefly luciferase was placed in the context GCCNCCAUGN where the two key nucleotides involved in context specificity (positions −3 and +4) were varied to generate all 16 possible combinations. The firefly reporter containing the wild-type eIF1 initiator context, which has additional deviations from the consensus besides −3/+4 changes, was examined in parallel. Vectors expressing the reporter with these different initiation contexts were separately cotransfected with either wild-type EIF1 (“eIF1 wild type”) or EIF1 initiated by AUG in the near-consensus context (“eIF1 good*”). Although both EIF1-coding regions specified identical residues, the eIF1 good* context enabled deregulated overexpression of eIF1 (Fig. 2). Luciferase reporter activity was used as a measure of the relative efficiencies of translation initiation in these 17 different contexts (Fig. 3A).
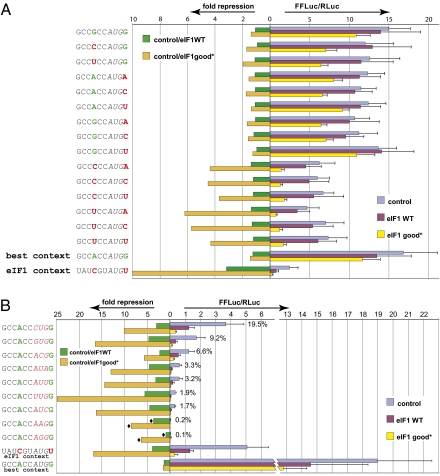
eIF1 overexpression inhibits initiation on poorly recognized codons. The results are from dual luciferase measurements. Renilla luciferase is initiated by an AUG in a consensus (good) context and is used to normalize firefly luciferase values. The firefly reporter is initiated by the codon, and the context, indicated on the left. (A and B) Firefly luciferase measurements from cells cotransfected with (i) empty vector (control)—gray-blue bars; (ii) “10×” vector expressing eIF1 in its native initiation context—maroon bars; or (iii) “10×” vector expressing eIF1 in the near consensus (good*) context—yellow bars. Right-facing bars show relative expression of firefly and Renilla luciferase. Left-facing bars show the ratio of normalized firefly luciferase expression (fold repression) calculated by comparing the values of (i) cells transfected with empty vector (control) and cells transfected with vector containing eIF1 initiated with the wild-type context (green bars); or (ii) cells transfected with empty vector and cells transfected with vector containing eIF1 initiated with the good* context (orange bars). (A) Results from experiments varying the context at positions −3 and +4. Varied nucleotides are shown in green or red, depending on whether they are predicted to provide a good or a poor context for initiation, respectively. (B) Results from experiments changing the start codon to one of the nine possible near cognate non-AUG codons. The fold repression marked by “” is probably an underestimate because the firefly activity is close to background level even under control conditions. The results in A and B represent independent experiments accounting for the slight difference in the values from firefly luciferases starting with “best” and “eIF1” contexts.
The results obtained from cells containing normal levels of eIF1 (gray-blue bars, Fig. 3A) are consistent with published bioinformatic and experimental results. The combination of A/G at −3/+4 positions was the most efficient for initiation, and all six combinations lacking both a −3 purine and a +4 G were at least twofold less efficient for initiation (Fig. 3A). Not unexpectedly, the reporter initiated from the native eIF1 context was expressed less efficiently than all of the other reporters, which is consistent with the results shown in Fig. 1D.
Expression of repressible (auto-regulatable) eIF1 (eIF1 WT: maroon bars in Fig. 3A) had little effect on the expression of firefly reporters in any context other than the reporter initiating with AUG in the eIF1 native context, which is in agreement with the results shown in Fig. 2A. Expression of derepressed eIF1 (eIF1 good*: yellow bars in Fig. 3A) resulted in reduced expression of all firefly reporters but had only minor effects on firefly reporters whose AUG initiators were flanked by a −3 purine and/or a +4 G (i.e., good contexts): the reduction never exceeded twofold of control cells (orange bars in Fig. 3A). In contrast, expression of all firefly reporters whose AUG codons were in poor contexts was reduced 3.5- to 6-fold (orange bars in Fig. 3A). The largest reduction (10-fold) in expression, and thus initiation, was seen for the reporter containing an AUG in the native EIF1 context. Under conditions of deregulated eIF1 expression, initiation at AUG in the “best” consensus context was 48-fold higher than initiation at AUG in the native EIF1 context. These results show that AUGs in poor initiation contexts were the most sensitive to increased levels of eIF1.
In additional experiments, the effect of eIF1 levels on initiation of all nine codons differing from AUG by a single nucleotide, but placed in the consensus initiation context, was investigated. Some of these codons have previously been shown to support initiation in mammals (21, 22). In the presence of endogenous levels of cellular eIF1 (control: gray-blue bars in Fig. 3B), initiation at these noncanonical start codons varied (relative to a “best context” AUG control) from 19.5% for CUG to 0.1% for AGG (Fig. 3B). Comparable data exist for Saccharomyces cerevisiae (23–25) and plants (26). Interestingly, comparing the plant studies with the results presented here indicates that both the order in which these codons support initiation, and the relative degree to which they do so, were similar. A recent S. cerevisiae study reported initiation rates at non-AUG codons as high as 7% of that at AUG in the same context (25). The relative efficiency of initiation for these non-AUG codons in yeast in that study resembled what we observed for mammalian cells but differed from earlier studies in yeast that indicated inefficient initiation with non-AUG codons with the most efficient non-AUG codon being AUA (23, 24).
Expression of derepressed EIF1 (eIF1 good*: yellow bars Fig. 3B) led to inhibition of initiation at all non-AUG codons (Fig. 3B). In general, the less efficient a non-AUG codon was for initiation, the greater the inhibition following expression of derepressed EIF1, with the highest level of inhibition, nearly 25-fold, observed for initiation at UUG cotransfected with a construct expressing EIF1 starting with AUG in the good* context (orange bars in Fig. 3B). The magnitude of inhibition was difficult to measure for the least-efficient codons AAG and AGG because of the near background levels of reporter expression even with endogenous levels of eIF1.
When reporters starting with AUG in the various GCCNCCAUGN contexts (as shown in Fig. 3A) were cotransfected with plasmids expressing EIF1 in its native context (eIF1 WT), there was at most a modest effect on expression (green bars in Fig. 3A). In contrast, when the reporter was initiated by any non-AUG codon and EIF1 WT was cotransfected, there was a marked reduction of reporter expression (green bars in Fig. 3B). For example, when UUG was used as the firefly luciferase reporter initiation codon, expression was reduced fivefold when EIF1 in its native context was cotransfected. Thus, despite the fact that overexpressing EIF1 starting with its native context leads to only a modest increase of intracellular levels of eIF1 (as seen in Fig. 2B, lane 1 compared with lane 7), initiation at these poor initiation sites is very sensitive to increased eIF1 intracellular concentrations.
Discussion
Our results suggest that the abundance of eIF1 impacts the choice of initiation site under physiological conditions in vivo. The up to 25-fold reduction at poor initiation sites when eIF1 is overexpressed suggests that there is selective pressure for normal initiation to be quite relaxed—presumably to allow limited translation of ORFs originating from poor initiation sites. Our results are consistent with previous in vitro experiments showing that added eIF1 can dissociate initiation complexes preassembled in its absence on AUG codons in poor context (7). They are also consistent with in vivo studies in yeast showing that eIF1 overexpression can suppress Sui− (suppression of initiation codon mutation) phenotypes resulting from certain mutant alleles of eIF5 and NIP1/eIF3c (6).
Evidence suggests that the concentration of certain molecules and ions can alter the fidelity of initiation on poorly recognized translation initiation codons and that this might be used for gene regulation. In vitro experiments demonstrate that increasing concentrations of Mg2+ improve initiation on non-AUG codons and also AUG codons in poor context (21). Highly conserved negative feedback regulation was found for genes in the polyamine pathway in which initiation at an AUU codon induced by increasing concentrations of polyamines seems to play a crucial role (27). Most recently, amino acid starvation was shown to increase global initiation at non-AUG codons in yeast (28).
Our data show that human eIF1 is subject to a negative autoregulatory feedback loop that affects translation initiation. On the basis of the sequence conservation of the eIF1 initiation context, this may be a universal mechanism operating in eukaryotes. This autoregulatory mechanism underscores the importance of the control of translation initiation as a genetic regulatory mechanism. Although eukaryotic and bacterial translational mechanisms are different, these domains of life use conceptually similar negative autoregulatory loops to control the synthesis of the functionally similar initiation factors, eIF1 and IF3, that are crucial for selecting translational start sites. Thus our findings provide a bridge connecting eukaryotic and prokaryotic gene regulation. The demonstration of the differential utilization of initiator sites in suboptimal contexts in response to changes in the cellular levels of eIF1 opens the possibility that an additional genome-wide control mechanism exists.
Materials and Methods
Plasmids.
Sense and antisense oligonucleotide pairs 1–28 (Table S1) were annealed and ligated either into PstI/BamHI-digested (oligonucleotide pairs 1–27) dual luciferase vector p2-Luc (29) to make pSV40-firefly constructs or into BamHI/XbaI digested (oligonucleotide pair 28) p2-Luc to make pSV40-Renilla. eIF1-WT, eIF1-good, and eIF1-good* were amplified by RT-PCR on RNA isolated from HEK-293T cells using sense primers 29 (WT), 30 (good), and 31 (good*) separately with antisense primer 32. Amplicons were cloned into NheI/XbaI-digested phRL-CMV (Promega). All constructs were verified by sequencing. Plasmid used for control transfections was pcDNA3 (Invitrogen).
Cell Culture and Transfections.
HEK-293T cells were obtained from the ATCC and maintained in DMEM supplemented with 10% FBS, 1 mM l-glutamine, and antibiotics. HEK-293T cells were transfected in quadruplicate with Lipofectamine 2000 reagent (Invitrogen), using the 1-d protocol in which suspended cells are added directly to the DNA complexes in half-area 96-well plates. For each transfection, the following were added to each well: 25 ng phRL-CMV eIF1 vector (-WT, -good, or -good* as indicated; not added for tranfections shown in Fig. 1D), 2.5 ng pSV40-firefly vector (with initiation codon and/or context as indicated in Figs. 1D, ,2A,2A, and 3 A and B), 0.1 ng pSV40-Renilla vector, and 0.2 μL Lipofectamine 2000 in 25 μL Opti-Mem (Gibco). The transfecting DNA complexes in each well were incubated with 4 × 104 cells suspended in 50 μL DMEM + 10% FBS. Transfected cells were incubated overnight at 37 °C in 5% CO2 for 19 h.
Dual Luciferase Assay.
Luciferase activities were determined using the Dual Luciferase Stop & Glo Reporter Assay System (Promega). Relative light units were measured on a Veritas Microplate Luminometer with two injectors (Turner Biosystems). Transfected cells were washed once with 1× PBS and then lysed in 12.6 μL of 1× passive lysis buffer (PLB), and light emission was measured following injection of 25 μL of either Renilla or firefly luciferase substrate. Firefly luciferase activity was calculated relative to the activity of a cotransfected control plasmid expressing Renilla luciferase (pSV40-Renilla). All data points were averaged and the SD was calculated. In Fig. 2C, data points and the corresponding SDs represent results from a single experiment done in triplicate; however, the results are representative of four additional independent experiments. In Fig. 3, data shown represent the mean and SD from three independent experiments, each done in quadruplicate (12 independent data points).
Western Analysis.
Cells were transfected in six-well plates with Lipofectamine 2000 reagent using the 1-d protocol described above, with either 3 μg (10×) or 0.3 μg (1×) phRL-CMV eIF1 vector (WT, good, or good*) as indicated. To maintain identical levels of transfecting DNA, those transfections with 0.3 μg phRL-CMV eIF1 vector were mixed with 2.7 μg pcDNA3 carrier DNA (control cells were transfected with 3 μg pcDNA3). In addition, 0.3 μg pSV40-firefly-eIF1 vector, 12 ng pSV40-Renilla vector, and 12 μL Lipofectamine 2000 were added to each well in 1,500 μL Opti-Mem. The transfecting DNA complexes in each well were incubated with 2.4 × 106 HEK-293T cells suspended in 3,000 μL DMEM + 10% FBS and incubated at 37 °C in 5% CO2 for 19 h. Transfected cells were lysed in 100 μL radioimmunoprecipitation assay (RIPA) buffer on ice for 20 min. Cell debris was removed from cell lysates by centrifugation at 15,000 × g at 4 °C for 15 min. Then 10-μL aliquots of the cleared lysates were diluted to 50 μL in 1× PLB; 12.5-μL aliquots of these were assayed as described above using the dual luciferase assay.
Proteins were resolved by 15% Bis/Tris·PAGE (MES buffer) and transferred to nitrocellulose membranes (Protran), which were incubated at 4 °C overnight in 0.25% gelatin with a 1:50 dilution of goat anti-eIF1 (Santa Cruz D-15) and a 1:10,000 dilution of mouse anti-β-actin (Sigma). Immunoreactive bands were detected on membranes after incubation with appropriate fluorescently labeled secondary antibodies using a LI-COR Odyssey Infrared Imaging Scanner (LI-COR Biosciences).
Acknowledgments
We thank Pavel V. Baranov and the members of the Atkins and Sachs laboratories for discussions. This work was supported by Science Foundation Ireland Grant 08/IN.1/B1889 (to J.F.A.) and by National Institutes of Health Grant R01 GM079523 (to J.F.A.) and Grant R01 GM47498 (to M.S.S.).
Footnotes
The authors declare no conflict of interest.
This article is a PNAS Direct Submission.
This article contains supporting information online at www.pnas.org/lookup/suppl/10.1073/pnas.1009269107/-/DCSupplemental.
References
Articles from Proceedings of the National Academy of Sciences of the United States of America are provided here courtesy of National Academy of Sciences
Full text links
Read article at publisher's site: https://doi.org/10.1073/pnas.1009269107
Read article for free, from open access legal sources, via Unpaywall:
https://europepmc.org/articles/pmc2964218?pdf=render
Free after 6 months at www.pnas.org
http://www.pnas.org/cgi/content/abstract/107/42/18056
Free after 6 months at www.pnas.org
http://www.pnas.org/cgi/content/full/107/42/18056
Free after 6 months at www.pnas.org
http://www.pnas.org/cgi/reprint/107/42/18056.pdf
Citations & impact
Impact metrics
Citations of article over time
Alternative metrics
Article citations
Nuclear release of eIF1 restricts start-codon selection during mitosis.
Nature, 635(8038):490-498, 23 Oct 2024
Cited by: 1 article | PMID: 39443796
Exploiting Translation Machinery for Cancer Therapy: Translation Factors as Promising Targets.
Int J Mol Sci, 25(19):10835, 09 Oct 2024
Cited by: 0 articles | PMID: 39409166 | PMCID: PMC11477148
Review Free full text in Europe PMC
Structural basis of AUC codon discrimination during translation initiation in yeast.
Nucleic Acids Res, 52(18):11317-11335, 01 Oct 2024
Cited by: 0 articles | PMID: 39193907 | PMCID: PMC11472065
Critical cis-parameters influence STructure assisted RNA translation (START) initiation on non-AUG codons in eukaryotes.
NAR Genom Bioinform, 6(2):lqae065, 11 Jun 2024
Cited by: 0 articles | PMID: 38863530
Translational regulation enhances distinction of cell types in the nervous system.
Elife, 12:RP90713, 16 Jul 2024
Cited by: 1 article | PMID: 39010741 | PMCID: PMC11251722
Go to all (104) article citations
Data
Data behind the article
This data has been text mined from the article, or deposited into data resources.
BioStudies: supplemental material and supporting data
Similar Articles
To arrive at the top five similar articles we use a word-weighted algorithm to compare words from the Title and Abstract of each citation.
Stringency of start codon selection modulates autoregulation of translation initiation factor eIF5.
Nucleic Acids Res, 40(7):2898-2906, 07 Dec 2011
Cited by: 85 articles | PMID: 22156057 | PMCID: PMC3326321
eIF1 discriminates against suboptimal initiation sites to prevent excessive uORF translation genome-wide.
RNA, 26(4):419-438, 08 Jan 2020
Cited by: 24 articles | PMID: 31915290 | PMCID: PMC7075259
eIF1 Loop 2 interactions with Met-tRNAi control the accuracy of start codon selection by the scanning preinitiation complex.
Proc Natl Acad Sci U S A, 115(18):E4159-E4168, 16 Apr 2018
Cited by: 20 articles | PMID: 29666249 | PMCID: PMC5939108
Should I stay or should I go? Eukaryotic translation initiation factors 1 and 1A control start codon recognition.
J Biol Chem, 283(41):27345-27349, 30 Jun 2008
Cited by: 34 articles | PMID: 18593708 | PMCID: PMC2562056
Review Free full text in Europe PMC
Funding
Funders who supported this work.
NIGMS NIH HHS (3)
Grant ID: R01 GM047498
Grant ID: R01 GM079523
Grant ID: R01 GM47498