Abstract
Free full text

Angiotensin II-mediated microvascular thrombosis
Associated Data
Abstract
Hypertension is associated with an increased risk of thrombosis that appears to involve an interaction between the renin-angiotensin system and hemostasis. In this study we determined whether angiotensin II-mediatedthrombosis occurs in arterioles and/or venules, and assessed the involvement of type-1 (AT1), type-2 (AT2) and type 4 (AT4) angiotensin II receptors, as well as receptors for endothelin-1 (ET-1) and bradykinin (BK-1, BK-2) in angiotensin II-enhanced microvascular thrombosis. Thrombus development in mouse cremaster microvessels was quantified after light/dye injury using the time of onset of the thrombus and time to blood flow cessation. Wild type and AT1-receptor deficient mice were implanted with an angiotensin II-loaded Alzet pump for 2 wks. Angiotensin II administration in both wild type and AT1-receptor deficient mice significantly accelerated thrombosis in arterioles. Genetic deficiency and pharmacological antagonism of AT1-receptors did not alter the thrombosis response to angiotensin II. Isolated murine platelets aggregated in response to low (pM), but not high (nM), concentrations of angiotensin II. The platelet aggregation response to angiotensin II was dependent on AT1-receptors. Antagonism of AT2-receptors in vivo significantly prolonged the onset of angiotensin II enhanced thrombosis, while an AT4-receptor antagonist prolonged the time to flow cessation. Selective antagonism of either ET-1 or BK-1 receptors largely prevented both the onset and flow cessation responses to chronic angiotensin II infusion. Our findings indicate that angiotensin II-induced hypertension is accompanied by enhanced thrombosis in arterioles and this response is mediated by a mechanism that involves AT2, AT4, BK-1 and ET-1 receptor-mediated signaling.
Introduction
The risk factors for cardiovascular disease (CVD), including hypertension, are often accompanied by alterations in platelet function and coagulation, which translate clinically as an increased risk for thrombosis1. While the higher incidence of thromboembolic events associated with CVD risk factors are more often manifested in large arteries, large vein involvement is also supported by epidemiological evidence2. Animal studies have also revealed that hypercholesterolemia enhances thrombus formation in arterioles but not in venules3. Enhanced thrombus formation in the microvasculature may contribute to the small vessel disease that can accompany chronic hypertension and other CVD risk factors, potentially leading to microinfarctions in brain and heart. However, it remains unclear whether other CV risk factors, such as hypertension, also promote thrombosis in the microvasculature.
Abnormalities in platelet activation/reactivity, coagulation, and fibrinolysis are commonly associated with hypertension, which could explain the increased risk for thrombus formation in this patient population1. An activated RAS and its key mediator AngII have been implicated in the prothrombotic state associated with hypertension1,4. This contention is supported by clinical evidence that hypertensive patients treated with either an angiotensin converting enzyme inhibitor or an Ang II receptor blocker exhibit a lower incidence of prothrombotic events5,6. Furthermore, Ang II administration in experimental animals recapitulates many of the abnormalities in platelet function and the enhanced thrombosis in large arteries described in patients with hypertension7,8. Whether AngII and its receptors also promote thrombus formation in the microvasculature remains unclear.
The physiological and pathophysiological responses to AngII involve the participation of a variety of mediators, cell surface receptors and intracellular signaling pathways. AngII can directly activate cells by engaging with AT1-, AT2-, and AT4-receptors9 or do so indirectly via the generation of endothelin-1 and/or bradykinin10,11, which then interact with their own receptor to mediate cell activation. A role for bradykinin in arterial thrombogenesis is supported by reports describing delayed thrombosis in mice that are genetically deficient in kininogen (a precursor to bradykinin in the kallikrein-kinin system)12, and by the ability of bradykinin to induce tissue factor expression and enhance the procoagulant properties of endothelial cells13. Endothelin-1, which is linked to Ang II via the AT1 receptor14, can also activate the coagulation cascade and induce microvascular thrombosis15,16.
The overall objectives of the present study were to: 1) determine whether AngII-hypertension alters thrombus formation in the microvasculature and 2) assess the involvement of receptors for AngII (ATr1, AT2r, AT4r), bradykinin (BK-1r & BK-2r) and endothelin-1 (ET-1r) in AngII-mediated microvascular thrombogenesis. Our findings indicate that AngII enhances thrombus formation in the microvasculature. While AT1r on platelets was shown to mediate AngII-induced platelet aggregation in vitro, this receptor population does not appear to contribute to microvascular thrombus formation. AT2r receptor activation appears to mediate the initiation (onset) of AngII-enhanced microvascular thrombosis, while AT4r activation contributes to the stabilization phase (blood flow cessation) of the thrombosis response. Antagonism of either the bradykinin-1 or endothelin-1 receptor largely prevented the accelerated onset and blood flow cessation responses elicited in arterioles by AngII.
Methods
Mice
Male C57BL/6 (WT) mice and AngII receptor type 1a knockout (B6.129P2-Agtr1atm1Unc/J) mice (AT1r−/−) were obtained from Jackson Laboratories (Bar Harbor, ME) or bred in the LSU Health Sciences Center animal facility. The mice were housed under specific pathogen-free (SPF) conditions and fed standard laboratory chow and water until the desired age (6–8 week). All experimental procedures involving the use of animals were reviewed and approved by the Institutional Animal Care and Use Committee of LSU Health Sciences Center and performed according to the criteria outlined by the National Institutes of Health Guide for the Care and Use of Laboratory Animals.
Angiotensin II infusion
Saline or AngII (1 μg/kg/min) was infused over 14 days using micro-osmotic pumps (Alzet, Cupertino, CA) which were implanted subcutaneously in the intrascapular region of isofluorane anesthetized mice. A topical antibiotic (Neosporin) was applied to prevent post-operative infection at the site of implantation. Blood pressure was monitored with a computerized tail cuff system (Hatteras Inst. Inc.).
Induction of microvascular thrombosis in cremaster muscle
On the 15th day following micro-osmotic pump implantation, the cremaster muscle prepared for intravital microscopic observation and microvascular thrombosis was induced by the light/dye method, as previously described.17 Thrombus formation was quantified by determining: 1) the time of onset of platelet deposition/aggregation within the microvessel (onset time), and 2) the time required for complete flow cessation for ≥30 sec (cessation time) after light/dye injury. For a more detailed method, see the online Data Supplement.
In vitro platelet aggregation
Platelet rich plasma (PRP) collected from mice was used to quantify AngII- and thrombin-induced platelet aggregation using a low-angle light scattering technique, as previously described.17 For a more detailed description of these procedures, see the online Data Supplement.
Murine plasminogen activator-1 (PAI-1) assay
Plasma PAI-1 was measured in control mice and in mice with an AngII pump using a murine PAI-1 total antigen ELISA kit (Innovative Research ™, Michigan, USA).
Experimental design
Thrombus formation in cremaster microvessels after light/dye exposure was determined in the following experimental groups: 1) WT mice implanted with a saline- or AngII loaded pump (WT/AngII), 2) WT/AngII mice treated with either the AT1r antagonist losartan (25 mg/kg per day, Merck&Co, Inc) in drinking water for 7 days prior to the experiment18, the AT2 antagonist PD123319 (30mg/kg, i.v., Sigma Chemicals)19 30 min prior to the experiment, or the AT4r antagonist Divalinal (from Dr. Harding’s laboratory) 8 mg/kg i.v. 45 min prior to the experiment20, 3) AT1r−/− mice with or without an AngII-loaded pump, 4) AT1r−/− mice with an AngII-loaded pump and treated with AT2r antagonist PD12319, 5) WT/AngII mice treated with either the BK-1 receptor antagonist des-Arg10 -HOE140 or BK-2r antagonist (HOE-140) 30 min prior to the experiment (50μg/kg i.v., Sigma), and 6) WT/AngII mice treated with the endothlin-1A receptor antagonist ABT-627 (Atrasentan) 5 mg/kg/day10 in drinking water for 7 days prior to the experiment.
Statistical analyses
All data are presented as means ± SEM, with ≥ 5 mice per group. For comparisons between groups, a one-way analysis of variance was used followed by either a Newman-Keuls or Students t-test.
Results
AngII pump implantation yielded a significantly elevated blood pressure in WT mice (138.4 ± 3.0 mmHg) compared to WT mice with a saline-loaded pump (104.1±4.6 mmHg) or WT mice not subjected to pump implantation (103±2.3 mmHg). The values for shear rate in microvessels and arterial blood pressure in anesthetized mice at 10 min after FITC-dextran administration are summarized in Table S1 (see online Data Supplement at http://hyper.ahajournals.org). Shear rates did not differ significantly between the experimental groups for both arterioles and venules. Selective antagonism of AT1r or AT2r significantly blunted the AngII-induced increase in blood pressure in WT mice. The AngII-induced hypertension was not observed in AT1r−/− mice.
Figure 1S (see online Data Supplement) illustrates the changes in the time of onset and time to flow cessation in arterioles (A&C) and venules (B&D) of normal, sham-operated WT mice (A&B), WT mice with a saline-loaded pump (A&B), and WT mice with an AngII-loaded pump (C&D). Since WT mice without pump implantation and WT mice with saline pumps did not differ relative to the time required to elicit both the onset of thrombosis and complete flow cessation, the two groups were pooled and used as controls (C&D). As previously reported, both onset and flow cessation times in control mice were markedly longer in arterioles than in the paired venules21. In arterioles, AngII infusion significantly accelerated both the onset (initiation) and cessation phases of light/dye-induced thrombus formation (C). While a similar tendency was noted in venules, these changes did not reach statistical significance. Since thrombus formation in arterioles is far more responsive to AngII infusion than in venules, all subsequent figures focus on the arteriolar responses.
Figure 1A illustrates the effects of pharmacological antagonism (losartan) or genetic deletion of AT1r on thrombus formation in arterioles of mice with an AngII pump. The data show that the time for initiation (onset) of thrombus formation in losartan-treated WT/AngII mice is significantly reduced (accelerated initiation of thrombus formation) compared to untreated WT/AngII mice, while the response to AngII infusion was not altered in AT1r−/− mice. The time to flow cessation in mice with an AngII pump was not affected in either AT1r−/− mice or in WT mice treated with losartan. A comparison of the thrombosis responses between arterioles of WT and AT1r−/− without pumps revealed an accelerated initiation (onset) of thrombosis with no difference in the time to flow cessation (Figure 1B). A similar enhancement of thrombosis (p<0.05) was noted in venules of AT1r−/− (onset =0.43±0.084 min, cessation =4.6±0.3 min, n=3) compared to WT (onset = 1.84±0.18 min, cessation =9.36±1.24 min, n=4) mice.
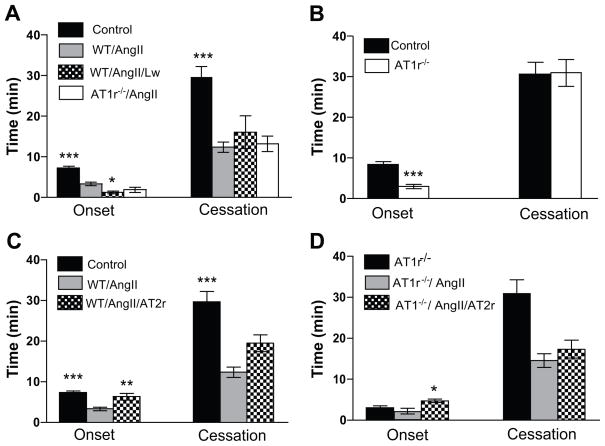
Roles of AT1- and AT2-receptors in AngII-enhanced, light/dye-induced thrombus formation in cremaster muscle arterioles. Control=Wild Type mice and Saline, WT=Wild Type mice, AT1r−/− =AT1 receptor deficient mice, Lw=losartan, AT2r=the AT2r antagonist PD123319. * indicates p<0.05 compared to WT/AngII (A), ** p<0.01 vs WT/AngII (C), *** p<0.001 vs WT/AngII (A&C) and vs control (B), * p<0.05 vs ATr1−/−/Ang (D).
Figure 1C illustrates the effects of PD123319 on the AngII-enhanced thrombosis response of arterioles to light/dye injury. AT2r blockade resulted in a significant recovery in the time to onset of thrombosis, but did not alter the time to flow cessation. Figure 1D illustrates how treatment of AT1r−/− mice with PD123319 affected the AngII-enhanced thrombosis responses. The combined effect of AT1r deficiency and AT2r blockade was similar to that noted with PD123319 treatment alone, i.e., a significant prolongation of thrombus initiation without a change in the cessation phase.
Figure 2 shows that treatment of WT mice with an AT4r antagonist (Divalinal) has no effect on AngII-enhanced initiation of thrombosis (onset), but it significantly prolongs the time required for complete flow cessation.

Role of AT4 receptors in AngII-enhanced, light/dye-induced thrombus formation in cremaster arterioles of control and AngII pump-implanted mice. Control=WT mice and saline, WT/AngII/AT4=Divalinal treated mice.*** p<0.01 and ** p<0.01 vs WT/AngII.
Figure 3 summarizes the actions of bradykinin 1&2-receptors antagonists (A) and an endothelin-1 (B) receptor antagonist on AngII-enhanced arteriolar thrombosis. These experiments reveal that blocking either the BK-1 or ET-1A receptor completely prevents both the accelerated onset and cessation of thrombosis normally associated with AngII infusion.
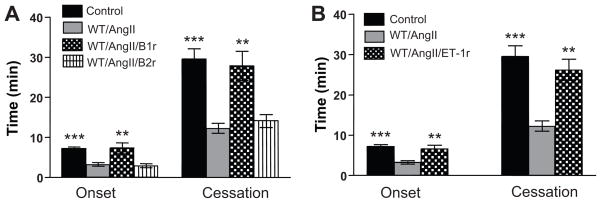
Role of bradykinin-1&2 receptors (A) and the endothelin-1A receptor (B) in AngII-enhanced, light/dye-induced thrombus formation in cremaster muscle arterioles. Control=WT mice and saline, B1r antagonist=des-Arg10-HOE140 (B1 receptor antagonist), B2r antagonist=HOE-140 (bradykinin-2 receptor antagonist), ET1r antagonist=ABT-627 (endothelin-1A receptor antagonist). **p<0.01 vs WT/AngII, *** p<0.001 vs WT/AngII.
The response of plasma PAI-1 to AngII infusion is shown in Figure 2S (see online Data Supplement). The data show that PAI-1 is significantly elevated in AngII pump mice compared to control mice (1.84±0.2 vs 1.0±0.21 ng/ml).
In vitro studies were undertaken to determine whether exposure of murine platelets to AngII elicits cell aggregation (Figure 4). These experiments revealed that, while micromolar and nanomolar concentrations of AngII do not induce platelet aggregation (panel C), picomolar levels elicit a brisk aggregation response. While platelets derived from WT mice exhibit a brisk aggregation response to 10 pmol/l AngII, platelets from AT1r−/− mice were unresponsive to the same AngII concentration (panel C). The half-maximum aggregation response to AngII (EC50) was found to be 10 pmol/l (panel A), which yielded a normalized mean aggregation velocity of 21.3±3.1 rel. units. As shown in Figure 3S, panel A (see online Data Supplement), thrombin elicits a dose-dependent aggregation response in murine platelets in the nanomolar range (or from 0.25 U/ml to 4.16 U/ml). The EC50 for thrombin in WT platelets was 0.63U/ml (6.77 nM). Exposure of platelets from AT1r−/− mice to the thrombin EC50 concentration produced an aggregation response that was enhanced (p<0.05) by approximately 35% (from 132±13.4 to 177.8±3.4 relative units).
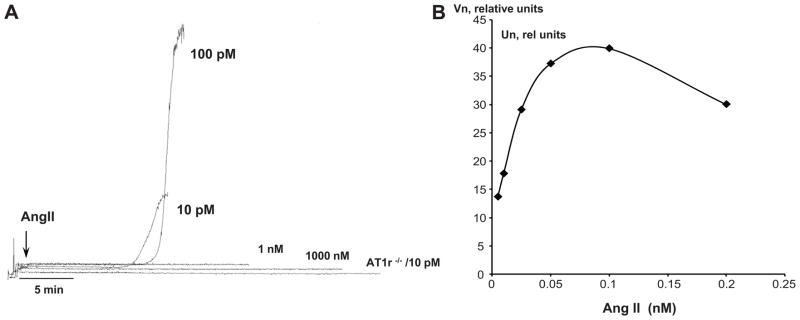
Aggregation velocity of murine platelets exposed to AngII. Panel A shows demonstrates the brisk aggregation induced by 10, 100 pmol/l AngII with WT platelets, the absence of an aggregation response in WT platelets exposed 1 nmol/l or 1 micromol/l AngII, and the lack of aggregation by AT1r-deficient (AT1r−/−) platelets in response to 10 pmol/l AngII. Panel B shows the normalized velocity of platelet aggregation (Vn) as a function of AngII concentration, from which an EC50 value of 10 pmol/l was derived.
In a separate group of platelet preparations (panel B of Figure 3S), we determined whether platelet preparations that were not responsive to 1 nM AngII were also non-responsive to thrombin. These experiments revealed that prior exposure to 1 nM AngII enhances, rather than blunts, the platelet aggregation response to thrombin. 10 pM AngII exposure did not exert this alter the aggregation response to thrombin.
Discussion
Hypertensive patients exhibit an increased risk for thrombosis, which links this CVD risk factor to diseases such as stroke and myocardial infarction. While abnormalities in platelet function, coagulation/fibrinolysis, and vessel wall activation have been implicated in the prothrombotic phenotype associated with hypertension4, 22, the mechanisms underlying this complication of hypertension remain poorly understood. The RAS has received considerable attention in this regard since drugs that target the RAS significantly reduce the incidence of thrombosis in hypertensive patients, animal studies have revealed a similar role for the RAS in the accelerated carotid artery thrombosis associated with renovascular hypertension1, 23, and a functional link between RAS activation and impaired fibrinolysis via PAI-1 has been demonstrated 24, 25. The present study provides evidence that the prothrombogenic effects of AngII II are not limited to large arteries and extend to the microvasculature. The accelerated microvascular thrombosis associated with chronic AngII infusion is most striking in arterioles, with a less reproducible response noted in venules. AngII infusion was also associated with significantly elevated plasma PAI-1 levels. While murine platelets were shown to respond to low picomolar levels of AngII in an AT1r-dependent manner, the AngII-enhanced arteriolar thrombosis appears to occur independently of AT1r activation. Instead, our findings implicate AT2r in the initiation (onset) and AT4r in the flow cessation phases of AngII-mediated thrombosis. Bradykinin-1 and endothelin-1A receptors (unlike AT2r and AT4r) appear to contribute to both the initiation and flow cessation phases of AngII-enhanced thrombus formation.
Patients with hypertension exhibit evidence for platelet activation and increased aggregation5, responses that can be reproduced by infusion of AngII in animals7. These observations are consistent with evidence that human platelets express a low density of high affinity AT1 receptors that bind to AngII26,27. Since the AngII-mediated enhancement of light/dye-induced thrombosis may reflect a direct action of the peptide on platelets, we evaluated whether AngII promotes the aggregation of murine platelets. We found that picomolar levels of AngII elicit a brisk aggregation response of WT platelets, while nanomolar and higher concentrations have no effect. The observed insensitivity to higher concentrations of AngII was not observed with thrombin and may reflect rapid desensitization of AT1 receptors27, which could account for the previously reported absence of aggregation of rat platelets following exposure to 10−7M AngII7. A role for receptor desensitization is supported by a report that employed AT1a receptor transfected CHO-K1 cells to demonstrate AT1r within 50 seconds following exposure to 10−9 M AngII.27 Despite desensitization of AT1r following AngII exposure, the platelets may remain responsive to other agonists like thrombin. We show in this study that prior exposure of murine platelets to 10−9 M AngII does not diminish the platelet aggregation response to thrombin, instead it is enhanced, suggesting that the signaling mechanisms within platelets are not compromised by the high levels of AngII. The enhanced sensitivity of AngII exposed platelets to thrombin is consistent with a previous report that describes an increased thrombin receptor expression in smooth muscle cells exposed to 10−9 M AngII for 2 hrs.28 In our model, the AngII-mediated platelet aggregation appears to be dependent on AT1r inasmuch as platelets derived from AT1r−/− mice did not respond to picomolar levels of AngII. A role for AT2r and AT4r in AngII mediated aggregation appears unlikely based on the complete absence of an aggregation response by AT1r deficient platelets and based on studies of human platelets26. These findings, when considered with our in vivo data, suggest that AngII-enhanced thrombus formation likely reflects an action of AngII on the vessel wall, rather than on platelets.
The absence of a role for AT1r in the enhanced arteriolar thrombus formation associated with chronic AngII infusion contrasts with previous reports describing the effectiveness of AT1r antagonists in blunting the platelet-endothelial cell adhesion induced in venules by acute AngII exposure29 or by hypercholesterolemia18. Since bone marrow chimeras produced from AT1r−/− mice have revealed that the enhanced platelet adhesion response to hypercholesterolemia is mediated by vascular wall (not platelet) AT1r, it is possible that the discrepancy between the roles of AT1r in platelet adhesion vs thrombosis may relate to inherent differences between arterioles and venules30. While the present study fails to implicate AT1r in the arteriolar thrombosis associated with chronically elevated AngII, we do provide some evidence for a protective role of AT1r in the initiation of thrombosis in both arterioles and venules in the absence of exogenous AngII. This observation is consistent with our in vitro aggregation results that show an exaggerated platelet aggregation response of AT1r−/− platelets to thrombin.
Previous studies by others have demonstrated a link between AngII and the kallikrein-kinin system in the modulation of coagulation, thrombus formation31, and fibrinolysis (impaired due to increased PAI-1 expression)32. BK-1 receptors are induced following tissue injury, inflammation, and AngII-infusion11,33. A prothrombotic role for bradykinin is supported by the prolonged time for carotid artery occlusion following thrombus induction in mice genetically deficient in kininogen, the substrate for kallikrein-mediated generation of bradykinin12. The BK-1r induction may occur as a result of sustained BK-2r activation and consequent desensitization33. The comparable effects of the AT2r and bradykinin BK-1r antagonists in prolonging the initiation (onset) of thrombosis during chronic AngII infusion are also consistent with an interaction between angiotensin and bradykinin receptors. Our observation that the BK-1r antagonist also prolonged the time for complete flow cessation after light/dye injury indicates that BK-1r dependent signaling plays a crucial role in the stabilization phase of thrombus formation.
An interesting and potentially important observation in this study is that an endothelin-1A receptor antagonist was as effective as the BK-1r antagonist in preventing the AngII-mediated acceleration of flow cessation during thrombus formation. The similarity of the protective responses afforded by antagonists to BK-1r and ET-1Ar suggests that the two receptors act through a common mechanism. AngII and its metabolites are known to stimulate the release of PAI-134,35, which would impair fibrinolysis and enhance thrombus development34–36. In this regard, it is noteworthy that both bradykinin and endothelin can mediate an increased expression of PAI-132,37. Heterodimerization of AngII and bradykinin receptors represents another potential mechanism however such interactions have been demonstrated for AT1r and BK2r38, neither of which appear to play a role in our in vivo model. While it is well established that AngII can elicit endothelin release from endothelial cells by engaging AT1r39, it remains unclear if/how endothelin-1 production and release by endothelial cells are linked to AT2 and/or BK-1 receptors. Additional support for a role for endothelin-1 in thrombus formation is provided by reports describing the ability of endothelin-1 to impair fibrinolysis via increased PAI-expression37, elicit a dose-dependent increase in plasma thrombin-antithrombin levels, increase the expression of von Willibrand factor on endothelial cells, and elicit thrombus formation in the rat mesenteric microvasculature15,16.
There is strong evidence linking the AT4 receptor to fibrinolysis and thrombosis. AT4r activation appears to result in increased active PAI-1 levels in murine plasma and an impairment of fibrinolysis25. This contention is supported by experiments demonstrating that AT4r-deficient (IRAP−/−) mice exhibit a prolongation of arterial thrombus formation25, while PAI-1 antagonism prevents thrombosis40. AT4r-mediated impairment of fibrinolysis is consistent with our observation that Divalinal prolonged the time to flow cessation, but not the initiation phase of thrombus development in AngII infused mice. The possibility that impaired fibrinolysis accounts for the shared actions of AT4r, ET-1Ar, and BK-1r on the flow cessation response in mice receiving AngII is supported by reports linking both endothelin and bradykinin to impaired fibrinolysis via PAI-1 induction32,37 as well as evidence that links AT4r signaling to endogenous endothelin41. Furthermore, we demonstrate in this study that chronic AngII infusion results in a significantly elevated PAI-1 level in mouse plasma. While connections between the different receptors evaluated in this study are suggested by circumstantial evidence in the literature, it is clear that additional work is needed to more clearly define how these receptor populations interact to mediate AngII induced microvascular thrombosis.
Perspectives
While clinical and experimental evidence clearly implicates AngII in the genesis of atherothrombosis, our findings indicate that the prothrombotic effects of the peptide also extend to the microcirculation. However, unlike AngII-mediated large vessel thrombosis, which involves activation of AT1r, the response elicited in arterioles does not appear to involve classical AT1r stimulation. Instead, AngII-mediated microvascular thrombosis involves the activation of AT2r and AT4r, with the former mediating thrombus initiation and the latter thrombus stabilization. AT1r and AT4r appear to interact with BK-1 and ET-1A receptors to mediate microvascular thrombus development. These findings suggest several novel therapeutic avenues for the prevention of microvascular thrombosis and small vessel disease that may result from chronic hypertension and other CVD risk factors.
Acknowledgments
Sources of Fundings
Present study was supported by a grant from the National Heart Lung and Blood Institute (R01 HL26441-29) & the Malcolm Feist Endowment for Cardiovascular Research at LSU Health Science Center – Shreveport.
Footnotes
Conflict of Interest/Disclosures: None
References
Full text links
Read article at publisher's site: https://doi.org/10.1161/hypertensionaha.110.158220
Read article for free, from open access legal sources, via Unpaywall:
https://www.ahajournals.org/doi/pdf/10.1161/HYPERTENSIONAHA.110.158220
Free after 12 months at intl-hyper.ahajournals.org
http://intl-hyper.ahajournals.org/cgi/reprint/56/6/1089.pdf
Free after 12 months at intl-hyper.ahajournals.org
http://intl-hyper.ahajournals.org/cgi/content/full/56/6/1089
Free to read at intl-hyper.ahajournals.org
http://intl-hyper.ahajournals.org/cgi/content/abstract/56/6/1089
Citations & impact
Impact metrics
Citations of article over time
Alternative metrics
Article citations
Increased Prolylcarboxypeptidase Expression Can Serve as a Biomarker of Senescence in Culture.
Molecules, 29(10):2219, 09 May 2024
Cited by: 0 articles | PMID: 38792081 | PMCID: PMC11123917
Role of the RAAS in mediating the pathophysiology of COVID-19.
Pharmacol Rep, 76(3):475-486, 23 Apr 2024
Cited by: 0 articles | PMID: 38652364 | PMCID: PMC11126499
Review Free full text in Europe PMC
Trauma diagnostic-related target proteins and their detection techniques.
Expert Rev Mol Med, 26:e7, 11 Apr 2024
Cited by: 1 article | PMID: 38602081 | PMCID: PMC11062145
Review Free full text in Europe PMC
Angiotensin ii therapy in refractory septic shock: which patient can benefit most? A narrative review.
J Anesth Analg Crit Care, 4(1):13, 21 Feb 2024
Cited by: 3 articles | PMID: 38383521 | PMCID: PMC10882873
Review Free full text in Europe PMC
The Renin Angiotensin System as a Therapeutic Target in Traumatic Brain Injury.
Neurotherapeutics, 20(6):1565-1591, 27 Sep 2023
Cited by: 4 articles | PMID: 37759139 | PMCID: PMC10684482
Review Free full text in Europe PMC
Go to all (63) article citations
Data
Data behind the article
This data has been text mined from the article, or deposited into data resources.
BioStudies: supplemental material and supporting data
Similar Articles
To arrive at the top five similar articles we use a word-weighted algorithm to compare words from the Title and Abstract of each citation.
Role of T lymphocytes in angiotensin II-mediated microvascular thrombosis.
Hypertension, 58(5):959-965, 12 Sep 2011
Cited by: 30 articles | PMID: 21911709 | PMCID: PMC3199307
Role of endothelin receptors in the hypertensive state of kinin B(2) knockout mice subjected to a high-salt diet.
Clin Sci (Lond), 103 Suppl 48:380S-384S, 01 Aug 2002
Cited by: 11 articles | PMID: 12193127
Mechanisms underlying the cerebral microvascular responses to angiotensin II-induced hypertension.
Microcirculation, 17(8):641-649, 01 Nov 2010
Cited by: 46 articles | PMID: 21044218 | PMCID: PMC3058857
Prejunctional effects of angiotensin II and bradykinin in the heart and blood vessels.
J Auton Pharmacol, 19(6):321-325, 01 Dec 1999
Cited by: 7 articles | PMID: 10961737
Review
Funding
Funders who supported this work.
NHLBI NIH HHS (3)
Grant ID: R01 HL26441-29
Grant ID: R01 HL026441
Grant ID: R01 HL026441-29