Abstract
Free full text

Genotyping mdx, mdx3cv, and mdx4cv mice by primer competition PCR
Abstract
Mdx, mdx3cv and mdx4cv mice are among the most commonly used models for Duchenne muscular dystrophy. Their disease is caused by point mutations in the dystrophin gene. Despite wide use of these models, genotyping has not always been straightforward. Current methods require multiple PCRs, post-PCR manipulations and/or special equipment/reagents. Here we report a simple, robust PCR genotyping method based on primer competition. This approach could also be applied in genotyping other point mutation models.
Introduction
Duchenne muscular dystrophy (DMD) is the most common lethal childhood muscle disease. It is caused by dystrophin deficiency. Dystrophin-null mice have been widely used to study the molecular pathogenesis of DMD and to develop novel therapies. Mdx, mdx3cv and mdx4cv mice are among the most commonly used DMD mouse models. Mdx mice carry a spontaneous nonsense mutation in exon 23 of the dystrophin gene.1,2 Mdx3cv and mdx4cv mice are generated by N-ethylnitrosourea mutagenesis.3 In mdx3cv mice, a point mutation in intron 65 results in aberrant splicing and disrupted dystrophin expression.4 In mdx4cv mice, a nonsense point mutation in exon 53 leads to premature translation termination.5
In contrast to the severe clinical manifestations seen in human patients, dystrophin-deficient mice display a very mild phenotype. This makes clinical diagnosis extremely challenging if not impossible. A simple, reliable genotyping method would substantially facilitate the use of these models in preclinical studies.
Many methods have been developed for genotyping mdx mice (reviewed in Trebbin et al.6). Traditional approaches include allele-specific oligonucleotide hybridization, restriction enzyme digestion of the PCR products, snap-back single-strand conformation polymorphism, and the amplification-resistant mutation system (ARMS).7–10 The ARMS assay has also been adapted for mdx3cv and mdx4cv genotyping.11 While quite useful, these methods often involve labor-intensive procedures and require delicate custom refinement. The SNaPshot and high-resolution melt (HRM) assays are new techniques developed in the last couple of years to improve mdx genotyping.6,12 These methods increase the specificity and sensitivity and allow detection of heterozygous samples. However, they require unconventional instruments such as the HRM PCR machine/software or a capillary electrophoresis system. The SNaPshot method also requires additional post-PCR manipulations. Most recently, a direct sequencing protocol has been developed to allow for an accurate diagnosis.13
Here we describe a simple and highly reproducible PCR protocol for mdx, mdx3cv and mdx4cv genotyping. It can be easily implemented in a routine laboratory setting. The method is based on the competition between the wild type allele-specific and mutant allele-specific primers in a single PCR.14 The specificity is further enhanced by a two-stage selective amplification protocol.
Materials and Methods
Animals
All animal experiments were approved by the Animal Care and Use Committee of the University of Missouri and were in accordance with NIH guidelines. C57Bl/6J (wild type BL6), C57Bl/10Snj (wild type BL10), C57BL/10ScSn-Dmdmdx/J (mdx), B6Ros.Cg-Dmdmdx-3Cv/J (mdx3cv) and B6Ros.Cg-Dmdmdx-4Cv/J (mdx4cv) mice were purchased from The Jackson Laboratory (Bar Harbor, ME). Heterozygous mice were generated in house by crossing BL10 and mdx, or by crossing BL6 with mdx3cv or mdx4cv.
DNA extraction and PCR conditions
Tail DNA was extracted using either a high salt precipitation or a brief alkaline lysis protocol.15,16 The PCR reaction was carried out in a 20 μL volume containing 4 μL of 5 × GoTaq Flexi buffer (Promega, Fitchburg, WI), 0.2 mM dNTPs, 2 mM MgCl2, 1 unit GoTaq DNA polymerase (Promega), ~150 ng tail DNA, and three primers including a common primer (0.66 μM), a wild type allele-specific primer (0.66 μM) and a mutant allele-specific primer (0.66 μM). The PCR condition was set as (1) an initial denaturation at 95°C for 2 min; (2) five cycles of the first stage amplification including 95°C denaturation for 20 sec, 60°C (for mdx and mdx3cv) or 62°C (for mdx4cv) annealing for 20 sec, and 72°C extension for 20 sec; (3) 23 cycles of the second stage amplification. This is essentially the same as the first stage amplification, except the annealing temperature is raised to 64°C (for mdx and mdx3cv) or 66°C (for mdx4cv); and (4) a final extension at 72°C for 1 min. The PCR was performed in an Eppendorf master cycler personal (Hamburg, Germany). The PCR products were resolved in a 3% agarose gel.
The primers and the size of the expected PCR products
Three primers were used in mdx genotyping including a common forward primer (DL1577), a wild type allele-specific reverse primer (DL1509) and a mutant allele-specific reverse primer (DL1573). Three primers were used in mdx3cv genotyping including a common forward primer (DL1578), a wild type allele-specific reverse primer (DL1524) and a mutant allele-specific reverse primer (DL1574). Three primers were used in mdx4cv genotyping including a common reverse primer (DL1579), a wild type allele-specific forward primer (DL1532) and a mutant allele-specific forward primer (DL1575). The primer sequences and the sizes of the expected PCR products are listed in Figure 1.
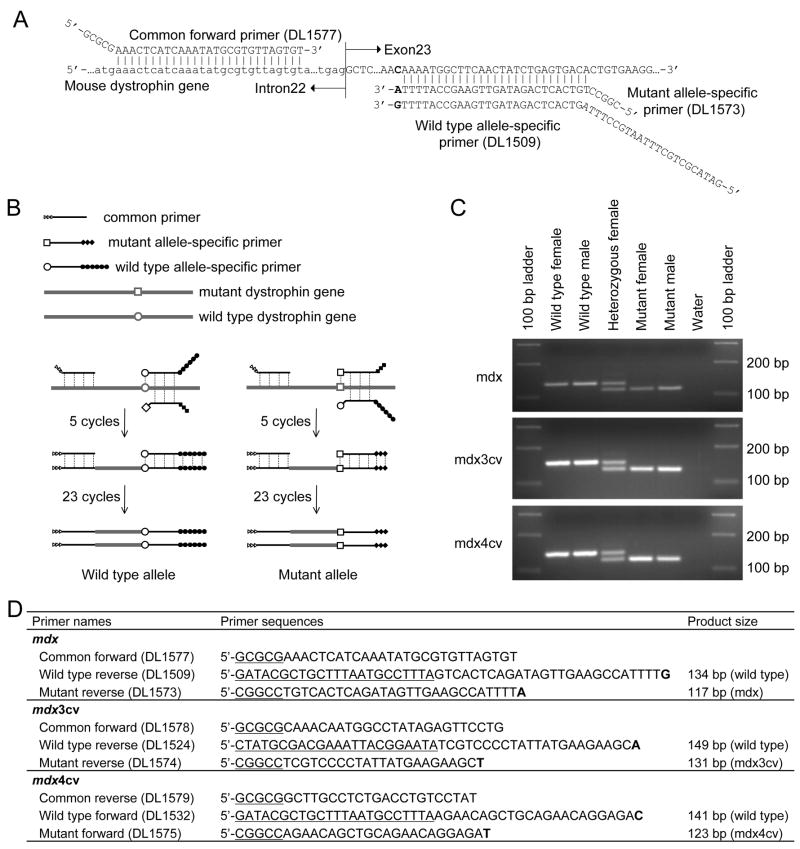
(A) Schematic outline of the primers used for mdx genotyping. The common forward primer is located at intron 22. The allele-specific reverse primers are located at exon 23. Small letter, intron sequence; bolded letter, nucleotide at the position of mutation. In the wild type mouse dystrophin gene, it is “C”, while it should be “T” in the mutated gene of mdx mice (not shown). Synthetic tails of the primers do not match with the dystrophin gene sequence. (B) Schematic outline of the primer competition PCR method. The first five cycles are performed at a relatively low annealing temperature aiming to amplify the mouse genomic DNA. The allele-specific primers compete with each other for the binding and extension on the genomic DNA templates. The following 23 cycles are carried out at an annealing temperature 4°C higher. This allows preferential amplification of the products from the first stage PCR. Open circle (○), the wild type nucleotide at the position of mutation; open box (□), the mutated nucleotide at the position of mutation; open triangle (), the synthetic tail added to the common forward primer; filled circle (●), the synthetic tail added to the wild type allele-specific primer; filled diamond (
), the synthetic tail added to the mutant allele-specific primer. (C) Representative genotyping results from each indicated strain. Water, negative control without the tail DNA template. (D) PCR primers and the expected PCR band sizes for mdx, mdx3cv and mdx4cv genotyping. The bolded letters represent allele-specific nucleotides at the position of the mutation. The underlined nucleotides are not present in the dystrophin gene. In the case of common and mutant allele-specific primers, they are GC-rich nucleotides. They increase the melting temperature to meet the need of the second stage PCR. In the case of wild type allele-specific primers, these nucleotides are chosen arbitrarily. They allow the wild type allele to yield a larger PCR product.
Results
The challenge in genotyping a point mutation lies in the difficulties of discriminating the wild type and mutant alleles. In the ARMS method, two separate PCRs are carried out using allele-specific primers that are different only at the last nucleotide. This often leads to non-specific amplification of the wrong allele, especially when the reaction condition is suboptimal.6,7,11,12 Occasionally, a unique restriction enzyme site may exist in either the wild type or mutant allele PCR products. This enables the diagnosis by the digestion pattern.10,17
To distinguish the wild type and mutant alleles in a single PCR reaction, we introduced several modifications (Fig. 1B). First, we added a long non-specific tail to the wild type allele-specific primer. This resulted in a larger band from the wild type allele (Fig. 1). Second, we mixed the wild type and mutant allele-specific primers together in the reaction. Competition between these two primers reduces erroneous binding and extension.14 Third, we designed a two-stage PCR reaction. The first stage included five cycles at a relatively low annealing temperature. During this stage, the genomic DNA was used as the template. Because of the competition between the wild type and mutant specific primers, the PCR products are enriched with the correct amplicons. The bulk of the PCR reaction (23 cycles) was performed at a more stringent condition at an annealing temperature 4°C higher. Since the amplicons of the first stage PCR carried DNA sequences perfectly matched with the primers, they were preferentially amplified. Untoward annealing (to the genomic DNA template) and amplification (of the genomic DNA) from the mismatched primer were minimized. To facilitate the second stage PCR, we have included a short GC-rich tail to the common and the mutant allele-specific primers.
We applied this strategy to mdx, mdx3cv and mdx4cv genotyping (Fig. 1C). Consistent results were obtained for homozygous (female), heterozygous (female) and hemizygous (male) mice irrespective of the strain and age (Fig. 1C). Of 288 genotypings performed for mdx mice, a correct diagnosis was reached on 96.4% occasions. The success rate for mdx3cv (77 reactions) and mdx4cv (99 reactions) reached 94.8% and 99%, respectively. The occasional failures were all correctly diagnosed after re-extracting the genomic DNA and redoing PCR.
Discussion
Point mutation dystrophin-null mice are powerful tools in DMD research. They have been intensively used to study dystrophin domain function, identify genetic modifier and test new therapy. The genotyping protocol described here will be very useful, especially when complicated crossings are involved. This strategy may also help to genotype point mutations in other disease models.
Acknowledgments
Grant support: This work was supported by grants from the National Institutes of Health (AR-49419, DD) and the Muscular Dystrophy Association (DD).
Abbreviations
- ARMS
- amplification-resistant mutation system
- DMD
- Duchenne muscular dystrophy
- HMR
- high-resolution melt
- PCR
- polymerase chain reaction
References
Full text links
Read article at publisher's site: https://doi.org/10.1002/mus.21873
Read article for free, from open access legal sources, via Unpaywall:
https://europepmc.org/articles/pmc3051167?pdf=render
Citations & impact
Impact metrics
Citations of article over time
Smart citations by scite.ai
Explore citation contexts and check if this article has been
supported or disputed.
https://scite.ai/reports/10.1002/mus.21873
Article citations
Leucyl-tRNA Synthetase Contributes to Muscle Weakness through Mammalian Target of Rapamycin Complex 1 Activation and Autophagy Suppression in a Mouse Model of Duchenne Muscular Dystrophy.
Am J Pathol, 194(8):1571-1580, 16 May 2024
Cited by: 0 articles | PMID: 38762116
Pannexin 1 dysregulation in Duchenne muscular dystrophy and its exacerbation of dystrophic features in mdx mice.
Skelet Muscle, 14(1):8, 26 Apr 2024
Cited by: 0 articles | PMID: 38671506 | PMCID: PMC11046831
Why is early-onset atrial fibrillation uncommon in patients with Duchenne muscular dystrophy? Insights from the mdx mouse.
Cardiovasc Res, 120(5):519-530, 01 Apr 2024
Cited by: 1 article | PMID: 38270932 | PMCID: PMC11060487
Dmd mdx mice have defective oligodendrogenesis, delayed myelin compaction and persistent hypomyelination.
Dis Model Mech, 17(4):dmm050115, 01 Apr 2024
Cited by: 0 articles | PMID: 38721692 | PMCID: PMC11095635
RhoA/ROCK signalling activated by ARHGEF3 promotes muscle weakness via autophagy in dystrophic mdx mice.
J Cachexia Sarcopenia Muscle, 14(4):1880-1893, 13 Jun 2023
Cited by: 3 articles | PMID: 37311604 | PMCID: PMC10401546
Go to all (47) article citations
Data
Similar Articles
To arrive at the top five similar articles we use a word-weighted algorithm to compare words from the Title and Abstract of each citation.
DMD(mdx3Cv) and DMD(mdx4Cv) dystrophin mutations in mice: rapid polymerase chain reaction genotyping.
Neuromuscul Disord, 12(4):366-370, 01 May 2002
Cited by: 5 articles | PMID: 12062254
A novel and simple method for genotyping the mdx mouse using high-resolution melt polymerase chain reaction.
Muscle Nerve, 39(5):603-608, 01 May 2009
Cited by: 6 articles | PMID: 19347923
A genotyping method combining primer competition PCR with HRM analysis to identify point mutations in Duchenne animal models.
Sci Rep, 10(1):17224, 14 Oct 2020
Cited by: 3 articles | PMID: 33057138 | PMCID: PMC7560699
What do animal models have to tell us regarding Duchenne muscular dystrophy?
Acta Myol, 24(3):172-180, 01 Dec 2005
Cited by: 14 articles | PMID: 16629050
Review
Funding
Funders who supported this work.
NIAMS NIH HHS (3)
Grant ID: R01 AR049419
Grant ID: AR-49419
Grant ID: R01 AR049419-08