Abstract
Free full text

Can Consuming Flavonoids Restore Old Microglia to their Youthful State?
Abstract
Microglial cells, resident macrophages in the central nervous system, are “primed” in the aged brain and are hypersensitive to messages emerging from immune-to-brain signaling pathways. Thus, in old individuals with an infection, microglia over react to signals from the peripheral immune system and produce excessive levels of cytokines causing behavioral pathology including serious deficits in cognition. Importantly, recent studies indicate dietary flavonoids have anti-inflammatory properties and are capable of mitigating microglial cells in the brain of aged mice. Thus, dietary or supplemental flavonoids and other bioactives have potential to restore the population of microglial cells in the old brain to its youthful state. This review briefly describes the immune-to-brain signaling pathways, consequences of microglial cell priming, and the potential of flavonoids to mitigate brain microglia and cognitive deficits induced by inflammatory cytokines.
Introduction
Although the immune system and brain constantly communicate, exchange of information increases during peripheral infection. When stimulated, the innate immune system conveys information to the brain where microglial cells react and produce inflammatory cytokines to coordinate a behavioral response that is normally adaptive1. Excessive production of inflammatory cytokines in the brain, however, can produce severe behavioral deficits and promote neurotoxicity. A good example is the murine ME7 model of prion disease (a chronic neurodegenerative disease) where stimulation of the peripheral innate immune system with lipopolysaccharide (LPS) induced an aggressive inflammatory cytokine response by brain microglial cells, excessive sickness behavior, and accelerated progression of the disease.2-3 The elements unique to the neurodegenerative disease provide a priming or sensitizing stimulus for microglia, while subsequent signals from peripheral immune stimulation provide a secondary triggering stimulus.4 The result of combining the two stimuli is an overall response that is greater in magnitude than the sum of the responses to individual stimuli alone. This interaction is thought to explain why infection is a risk factor for relapse in multiple sclerosis patients and dementia in patients with Alzheimer's disease.5-6
Similar to chronic neurodegenerative diseases, aging was recently proposed to prime microglial cells.7-9 Specifically, MHC class II, a marker for activated microglia, was shown to be increased in brain of healthy aged mice 9 and intraperitoneal (i.p.) administration of LPS resulted in an exaggerated inflammatory cytokine response in the aged brain.9-10 Similar findings have been reported in older rats inoculated with Escherichia coli,11 and the discordant cytokine response in the brain is not due to an amplification of the peripheral immune signals, because intracerebroventricular injection of LPS elicited the same discordant response in aged mice.12 Consistent with an exaggerated inflammatory cytokine response in the brain, aged mice exhibited more severe anorexia, depressive-like behaviors, and deficits in hippocampal-dependent learning and memory compared with younger cohorts after i.p. LPS injection.9-10, 13 These observations from rodent studies are noteworthy because acute cognitive impairments are common in elderly humans and often occur in association with peripheral infection.14-15 For example, demented elderly patients are routinely screened for bladder infections because of the recognized association of infection and cognitive disorders. It is also interesting that elderly people with pneumonia often present symptoms consistent with delirium.16 Thus, an inflammatory response initiated during infection that is normally adaptive may elicit severe behavioral deficits in elderly individuals, ultimately leading to prolonged recovery periods.
Together these examples highlight the need for pragmatic strategies to mitigate microglial cell activity in aged individuals. Interestingly, recent evidence suggests that ingestion of flavonoids is associated with improved cognitive function and a lower risk for neurodegenerative disease, suggesting the beneficial effects of flavonoids may be conferred through an anti-inflammatory pathway. Thus, dietary factors may directly or indirectly affect microglial cell activity and prevent behavioral and neurological pathology in certain situations. The goal of this review is to discuss the evidence that suggests flavonoids modulate microglia, reduce inflammation, and improve cognitive function in the aged. First, however, we provide a brief overview of how the immune system “talks” to the brain so readers can appreciate how flavonoids or other dietary constituents may influence neuroimmune interactions.
Immune-to-brain signaling
Cells associated with the peripheral innate immune system (e.g., macrophages and monocytes) produce inflammatory cytokines such as interleukin (IL)-1β, IL-6, and tumor necrosis factor-α (TNFα) that facilitate communication between periphery and brain during infection. Although there is clear evidence that inflammatory cytokines can be actively transported from blood into the brain,17-21 peripheral cytokines need not enter the brain to elicit behavioral changes. This is because inflammatory stimuli in the periphery (e.g., LPS and inflammatory cytokines) induce transcripts for IL-1β, IL-6, and TNFα in discrete brain areas.22-23 For example, inflammatory stimuli in the periphery can bind receptors on blood-brain barrier endothelial cells24 and induce perivascular microglia to express cytokines.25-26 Furthermore, cytokines in the periphery can convey a message to the brain via the vagus nerve. After LPS challenge, dendritic cells and macrophages that are closely associated with the abdominal vagus have been shown to express IL-1β protein27; IL-1 binding sites have been identified in several regions of the vagus as well.28 When activated by cytokines, the vagus can activate specific neural pathways that are involved in sickness behavior. However, activation of the vagus also appears to stimulate microglia in the brain to produce cytokines via the central adrenergic system.29-31 The neural pathways activated in the brain by the vagus nerve for rapid immune-to-brain signaling have been described in some detail.32 These pathways appear to be responsible for activating the hypothalamic-pituitary-adrenal axis and depressing behavior in response to infection. Other mechanisms that involve induction of prostaglandin synthesis are also important for fever. A slower immune-to-brain signaling mechanism based on volume transmission has also been proposed.33 In this method of immune-to-brain communication, production of IL-1β by the brain first occurs in the choroid plexus and circumventricular organs—brain areas devoid of an intact blood-brain barrier. The cytokines then slowly diffuse throughout the brain by volume transmission, along the way activating neurons and neural pathways that induce sickness behavior and inhibit cognition. This pathway may be more important for maintaining sickness behavior than inducing it 33. Nonetheless, it appears all pathways involved in immune-to-brain signaling lead to production of inflammatory cytokines by brain microglia, a commonality that will likely prove useful in mitigating exaggerated microglial responses.
For reasons not well understood, microglia in the brain of old, but otherwise healthy mice appear to be primed.34 Microglia from aged brain typically show a reactive phenotype (Figure 1A) including increased expression of cell-surface receptors like MHC class II, scavenger receptors, and complement receptors. Microglial expression of complement receptor-335 and the macrosialin (CD68) scavenger receptor36 are also elevated in the brain of aged rodents. In humans, increased HLA-DR, a MHC class II marker, was found on hippocampal microglia of older individuals.37 However, this reactive phenotype was more prevalent in patients with inflammatory disease or injury.38-40 In old healthy subjects, the primed microglia produce markings of a low-grade inflammatory response. For example, Gavilan et. al. (2007) observed a shift in balance between inflammatory and anti-inflammatory mediators in aged hippocampus, showing significantly increased mRNA expression of IL-1β, TNFα and iNOS.41 In addition, a microarray study that compared steady-state gene expression in brain of young adult and aged mice discovered 38 genes associated with inflammation that were differentially expressed: 34 were up-regulated and only 4 were down-regulated in the aged (Figure 1B).9 Another study suggested inflammatory markers begin to appear in middle-age.42 Godbout et. al. (2005) also confirmed elevated steady-state levels of inflammatory cytokines in the normal healthy aging brain, which lead to prolonged sickness behavior after activation of the peripheral innate immune system.9 This increased vulnerability to infection, which increases age-associated neurobehavioral impairments and increases the risk for neurodegenerative diseases,43-44 may be related to chronic microglial activation and the subsequent elevated levels of inflammatory cytokines.4 The question remains as to whether microglial activity or function can be altered (e.g. via diet) to positively influence the aging brain.
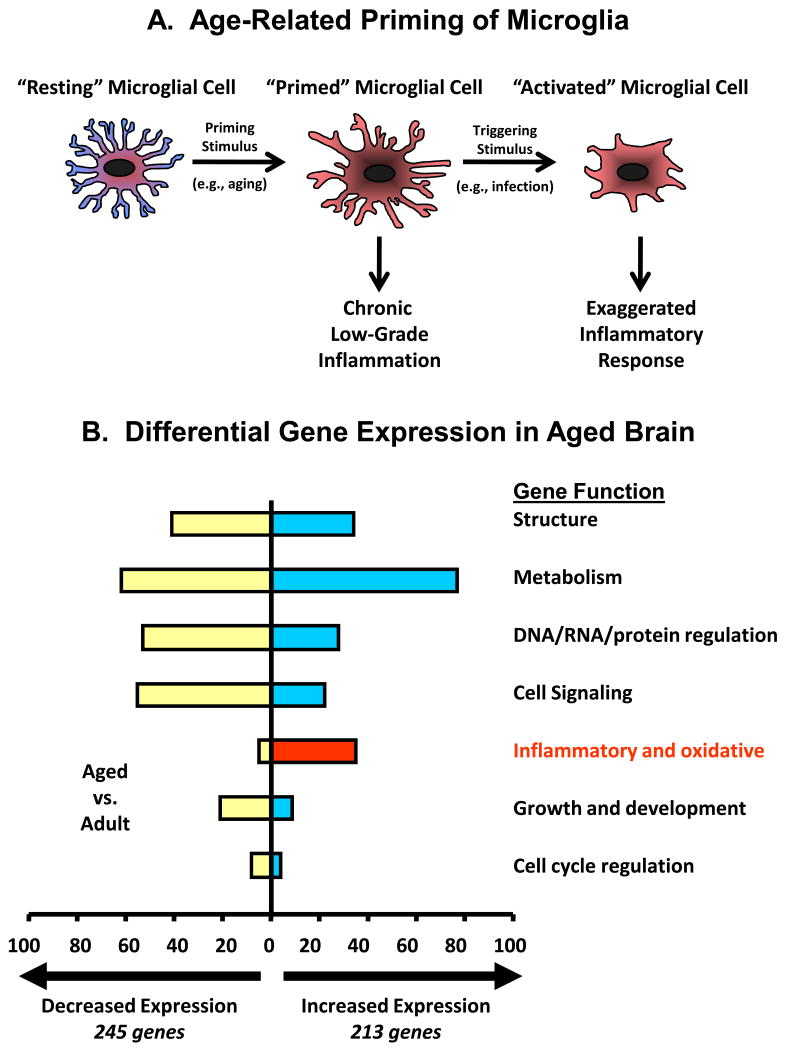
(A) Microglia are normally quiescent but in old age they transition to a “primed” phenotype. Evidence suggests primed microglia cause low-grade inflammation. Further, primed microglia are hypersensitive to signals emerging from immune-to-brain signaling pathways, producing excessive levels of inflammatory cytokines and causing behavioral pathology including deficits in cognition (adapted from Dilger and Johnson, 2008). (B) Microarray analysis revealed a number of differentially expressed genes in brains of old mice compared to young adults. Nearly all of the inflammatory genes that were differentially expressed were up regulated in older mice (adapted from Godbout et al., 2005).
Can flavonoids regulate microglial cell activity?
So what evidence is there that flavonoids may regulate microglial cells? A number of epidemiological studies suggest that consuming diets rich in fruits and vegetables containing high levels of phytochemicals reduces the risk of various pathologic conditions ranging from diabetes to cardiovascular disease.45-47 Note that these, and almost all chronic diseases have a major inflammatory component. These observations prompted the recommendation of two cups of fruit and two and a half cups of vegetables per day for a 2,000 calorie daily intake (Dietary Guidelines for Americans (2005)).48 Flavonoids represent the most common and widely distributed group of plant phenolics and are found in fruits, vegetables, nuts, grains, and tea. Over 6000 different flavonoids have been identified49 and can be categorized in 6 classes by structure: flavonoles, flavones, isoflavones, flavanoles, flavanones and anthocyanidins. Flavonoids possess a number of physiological properties including antioxidant, antibactericidal, antiviral, antimutagenic, anticancer, and yes, anti-inflammatory.50 A number of epidemiological studies observed health benefits of flavonoids.51-52 Hertog et al. (1995) observed that the average intake of flavonoids was inversely associated with coronary heart disease.51 In the Zutphen study, a longitudinal population-based study in the Netherlands an inverse association between dietary flavonoid consumption and incidence of stroke was observed.52 The effect of flavonoids on cancer has also been examined. Significant inverse associations of flavonoid intake and incidence of lung cancer has also been observed by analyzing dietary data from 541 individuals with lung cancer and 540 hospitalized controls.53 A cohort study from Finland of nearly 10,000 men and women reported an inverse association between the intake of flavonoids and the incidence of all cancers combined.54
Flavonoids exist as glycosides and aglycones forms in plant-derived foods. After oral ingestion, flavonoids are extensively conjugated and metabolized during absorption in the small intestine and then again in the liver.55-57 The intact form of flavonoid and respective metabolites derived from flavonoid biotransformation in the gastrointestinal tract and in the liver are the forms which enter the circulation and ultimately reach to the brain58-60. Investigations into the effects of flavonoids on cognition have mainly focused on synaptic plasticity and neurogenesis. However, the role of flavonoids on other brain cells, which affect neuronal function, has received much less attention. Microglia are the major cell type in the brain to produce inflammatory molecules such as cytokines, superoxide, and nitric oxide. Previous studies reported that inhibition of these molecules is beneficial for mitigating neurodegenerative disorders 61. In the inflammatory response, NF-κB and AP-1 are important transcription factors and MAPK (ERK1/2, p38 and c-Jun-N-terminal kinase (JNK)) pathways are involved in regulating inflammatory gene expression. Several studies have demonstrated that flavonoids significantly suppressed the activation of NF-κB and AP-1 as well as MAPK pathways in activated microglia, resulting in an attenuation of the production of inflammatory molecules.62-63 Recently, luteolin, a flavonoid present in celery and green peppers, was shown to suppress LPS-induced IL-6 protein and mRNA expression by inhibiting AP-1 activation and phosphoryolation of JNK in murine microglial cell line, BV-2 cells.62 Moreover, when mice were provided drinking water supplemented with luteolin before treatment with LPS, plasma IL-6 and IL-6 mRNA in the hippocampus was reduced compared to those not receiving luteolin.62 In another study, luteolin affected the microglial transcriptome leading to an anti-inflammatory, anti-oxidative, and neuroprotective phenotype 64. Apigenin and luteolin also suppressed microglia TNFα and IL-6 production stimulated by interferon-gamma in the presence of CD40 ligation.65 Quercetin suppressed LPS-induced NO production and inducible nitric oxide synthase (iNOS) gene transcription by inhibiting activation of IκB kinase (IKK), NF-κB and AP-1.63
Furthermore, studies have shown that flavonoids exert neuroprotection through inhibiting microglia activation and the subsequent release of various inflammatory molecules (Table 1). For example, pretreatment with luteolin attenuated inflammatory mediators (IL-1β, TNFα, NO, and prostaglandin E2) produced by LPS-stimulated microglia.66 Supernatant from LPS-stimulated microglia caused discernible death in Neuro.2a cells (a neuroblastoma cell line). However, treating microglia with luteolin prior to LPS reduced neuronal cell death caused by conditioned supernatants.66 Incubating Neuro.2a cells with luteolin did not protect them from supernatants from LPS-stimulated microglia, indicating luteolin protects neurons by acting exclusively on microglia.66 Zheng et al also reported that fisetin decreased TNFα and NO production and significantly suppressed nuclear transclocation of NF-κB and phosphorylation of p30 MAPKinase in the LPS-stimulated BV-2 microglia cells.67 In addition, fisetin reduced cytotoxicity of LPS-stimulated microglia toward B35 neuroblastoma cells in a co-culture system.67 A flavonoid in soy, genistein, inhibited LPS-induced microglia activation and production of TNFα, NO and superoxide and attenuated the LPS-induced decrease in dopamine uptake and loss of tyrosine hydroxylase-immunoreactive neurons in rat mesencephalic neuron-glia cultures.68 Wogonin also inhibited NO production by suppressing inducible NO synthase (iNOS) induction and NF-κB activation in microglia.69 Inhibition of microglia by wogonin reduced cytotoxicity when co-cultured with PC12 cells, differentiated neuronal cells from neuroendocrine cells, supporting a neuroprotective role for wogonin in vitro69. Other studies have shown that resveratrol, quercetin, or genistein diminished neuronal cell death induced by microglial activation.68, 70 Taken together, these results indicate that flavonoids may be important bioactives for attenuating microglia activation and neuronal cell damage by inflammatory conditions.
Table 1
Flavonoid | Experimental model | Action | Reference |
---|---|---|---|
Luteolin | BV-2 | Decreased IL-1β, TNFα, NO, and PGE2; Attenuated neuronal cell death | Jang et al. (2010) 66 |
BV-2 | Caused global changes in microglial transcriptome leading to an anti-inflammatory and neuroprotective phenotype | Dirsherl et al. (2010)64 | |
Fisetin | BV-2 | Decreased TNFα and NO; reduced cytotoxicity of LPS-stimulated microglia toward neuroblastoma cells | Zheng et al. (2008)67 |
Apigenin | BV-2 | Inhibited NO and PGE2 | Ha et al. (2008)94 |
Resveratrol and quercetin | N9 | Diminished apoptotic neuronal cell death induced by microglial activation | Bureau et al. (2008)70 |
Blueberry extract | BV-2 | Inhibited LPS-induced NO, TNFα and IL-1β | Lau et al. (2007)95 |
Isoflavone metabolites | Primary microglia BV-2 | Decreased LPS-induced NO and TNFα | Park et al. (2007)96 |
Genistein | Primary glia | Inhibited LPS-induced microglia activation (OX-42) and protect dopaminergic neurons | Wang et al. (2005)68, Park et al. (2007)96 |
Silymarin | BV-2 | Reduced LPS-induced nitrite and iNOS | Wang et al. (2002)97 |
Do flavonoids reduce microglial cell activity and inhibit cognitive aging?
An important question is if the aforementioned in vitro studies translate to the in vivo setting. Epidemiological studies provide evidence suggesting beneficial effects of flavonoids on cognitive deficits in old persons or demented patients.71-73 Letenneur et al. (2007) reported an inverse relationship between flavonoid intake and cognitive decline in older persons (n=1,640 at baseline).72 They showed that flavonoid intake was associated with better cognitive performance at baseline as well as with a better evolution of the performance over time. Commenges et al. (2002) also reported that the relative risk of dementia was inversely related to the mean flavonoid intake in a cohort of 1367 subjects above 65 years of age.73 Although many studies focus on flavonoid's anti-oxidant properties, it is important to note that the primary transcription factors that regulate inflammatory genes (e.g., NF-κB and AP-1) are redox sensitive. Together, these studies show that flavonoids have beneficial effects on age-related cognitive decline in humans.
So what is the connection between inflammatory cytokines and cognition? As already described, peripheral infection leads to the synthesis of inflammatory cytokines in the brain. Inflammatory cytokines like IL-1β disrupt hippocampal-dependent cognitive processing during infection.74-79 Specifically, IL-1β inhibits memory consolidation making it more difficult to learn new tasks. Elevated hippocampal levels of IL-1β are associated with impaired synaptic plasticity in the CA1 region of the hippocampus and deficits in long-term potentiation (LTP), which is thought to be a biological substrate for learning and memory.80 In rats, peripheral administration of LPS caused deficits in contextual fear conditioning which was blocked when IL-1ra was administered immediately following LPS.77 Similarly, animals infected with the Gram negative bacterium Legionella pneumophila showed significantly longer latencies and distances to find the platform in the Morris water maze compared to non-infected animals, but when neutralizing antibodies against IL-1β were administered, these deficits were eliminated.81
The inflammatory cytokine IL-6 may also play a role in modulating cognition during neurodegenerative disease and aging. The severity of dementia in Alzheimer's disease has been linked to high levels of IL-6 in plaques,82 and IL-6 levels in plasma were correlated with cognitive impairments in humans.83 In transgenic mice whose astrocytes over expressed IL-6, deficits in avoidance learning and a reduction in dendritic complexity in the hippocampus were evident.84-85 Further, mice deficient in IL-6 also had significantly lower levels of LPS-induced IL-1β and TNFα in the neuronal layers of the hippocampus compared to similarly treated wild type mice.86 After peripheral immune stimulation, mice deficient in IL-6 performed similarly to saline injected mice in the spatial working memory version of the water maze in which mice had to integrate new information with existing memories, while wild type mice were impaired. This study suggests that IL-6 is an important mediator of cognitive deficits, and collectively, these experiments provide evidence that inflammatory cytokines combine to cause deficits in learning and memory.
Evidence also has emerged showing beneficial effects of dietary intervention with flavonoids on neuroinflammation and cognitive decline in animal models (Table 2). For example, mice fed control diet from 22-24 months of age exhibited deficits in spatial working memory and expression of inflammatory markers in the hippocampus indicative of increased microglial cell activity, whilst luteolin consumption improved spatial working memory and restored expression of inflammatory markers in the hippocampus to that seen in young adults.62 In addition, aged rats supplemented with flavonoid-rich fruits and vegetables such as spinach, blueberry, or strawberry extracts exhibited faster learning and better memory retention than non-supplemented animals.87 Furthermore, long-term administration of green tea catechins suppressed morphologic and functional regression of the brain in SAMP10 mice, a mouse strain that ages prematurely.88 Catechins were further shown to improve spatial learning in rats.89 A neuroprotective effect of flavonoids was also observed in a study in which chronic administration of apigenin-7-glucoside and quercetin reversed the age- and LPS-induced retention deficits in passive avoidance and elevated plus-maze tasks.90 In addition, chronic Ginkgo biloba, a flavonoid-rich antioxidant treatment blocked spatial memory deficits in a transgenic mouse model of Alzheimer's disease.91 Taken together, these reports indicate that flavonoid intake may improve cognitive function by inhibiting neuroinflammation.
Table 2
Flavonoid | Experimental model | Action | Dose | Reference |
---|---|---|---|---|
Luteolin | Aged mice | Decreased pro-inflammatory cytokines in hippocampus and improved spatial working memory | 6 mg/kg diet; 4 wks | Jang et al. (2010)66 |
Grape seed extract | Transgenic mouse model of AD | Decrease IL-1β, TNFα, NO, and PGE2 | 10 – 50 μmol/L | Wang et al. (2009)98 |
Onion extract and quercetin | Gerbil | Attenuated gliosis and protected pyramidal neurons of the hippocampal CA1 region from ischemic damage | 100 mg.kg onion extract and 20 mg/kg quercetin for 15 days | Hwang et al. (2009)99 |
EGCG | Transgenic mouse model of ALS | Increased number of motor neurons; diminished microglial activation (CD11b); reduced immunohistochemical reaction of NF-κB | 10 mg/kg, p.o. for 80 days | Xu et al. (2006)100 |
Green tea catechin | Rats | Improved spatial learning | 0.1%, 0.5% of green tea catechins in water;p.o.; 26 wks | Haque et al. (2006)89 |
Apigenin-7-glucoside and quercetin | Young and aged mice | Reversed the age-induced and LPS-induced retention deficits in passive avoidance and elevated plus-maze tasks | apigenin-7-glucoside (5-20 mg/kg i.p.) and quercetin (25-100 mg/kg i.p.); 7 days | Patil et al. (2003)90 |
Ginkgo biloba | Transgenic mouse model of AD | Blocked spatial memory deficits | 70 mg/kg/day in water; 6 months | Stackman et al. (2003)91 |
Spinach, blueberry or strawberry extracts | Aged rat | Improved learning and memory retention | 1.48% strawberry, 0.91% spinach, or 1.86% blueberry (w/v) for 8 wks | Joseph et al. (1999)87 |
Conclusion
Food for thought
In light of the recent evidence suggesting microglial cells become dysregulated due to aging, it is an interesting prospect to think dietary flavonoids and other bioactives can be used to restore the population of this cell to its youthful state. But how could flavonoids impart this anti-inflammatory effect? Although in vitro studies clearly indicate that several flavonoids can act directly on microglial cells to restrict the inflammatory response, results from in vivo studies thus far do not prove that dietary flavonoids access the brain to interact with microglia in a meaningful way. This is a complicated question to dissect because flavonoids reduce inflammation in the periphery and microglia seem to act like an “immunostat,” detecting and responding to signals emerging from immune-to-brain signaling pathways. Thus, whether dietary flavonoids enter the brain and impart an anti-inflammatory effect on microglia is an interesting question but one that is more theoretical than practical because what is most important is how the immunostat is adjusted whether that is via a direct or indirect route (Figure 2). Because flavonoids are detectable in brain.58, 92-93 they are most likely to regulate microglia both directly and by dampening immune-to-brain signaling.
Acknowledgments
Funding. This work was supported by National Institutes of Health (NIH) grants AG16710 and MH069148.
Contributor Information
Saebyeol Jang, Integrative Immunology and Behavior Program and Division of Nutritional Sciences, University of Illinois, 1207 W. Gregory Dr., Urbana, IL 61801.
Rodney W. Johnson, Division of Nutritional Sciences, Integrative Immunology and Behavior Program, and Department of Animal Sciences, University of Illinois, 1207 W. Gregory Dr., Urbana, IL 61801.
References
Full text links
Read article at publisher's site: https://doi.org/10.1111/j.1753-4887.2010.00336.x
Read article for free, from open access legal sources, via Unpaywall:
https://europepmc.org/articles/pmc3058829?pdf=render
Subscription required at ingentaconnect
http://openurl.ingenta.com/content?genre=article&issn=0029-6643&volume=68&issue=12&spage=719
Citations & impact
Impact metrics
Article citations
Dissecting the antidepressant effect of troxerutin: modulation of neuroinflammatory and oxidative stress biomarkers in lipopolysaccharide-treated mice.
Naunyn Schmiedebergs Arch Pharmacol, 29 Jun 2024
Cited by: 0 articles | PMID: 38951153
Anti-Inflammatory and Immunomodulatory Effects of the Grifola frondosa Natural Compound o-Orsellinaldehyde on LPS-Challenged Murine Primary Glial Cells. Roles of NF-κβ and MAPK.
Pharmaceutics, 13(6):806, 28 May 2021
Cited by: 6 articles | PMID: 34071571 | PMCID: PMC8229786
Lifestyle-dependent microglial plasticity: training the brain guardians.
Biol Direct, 16(1):12, 05 Aug 2021
Cited by: 14 articles | PMID: 34353376 | PMCID: PMC8340437
Review Free full text in Europe PMC
Microglia Specific Drug Targeting Using Natural Products for the Regulation of Redox Imbalance in Neurodegeneration.
Front Pharmacol, 12:654489, 13 Apr 2021
Cited by: 20 articles | PMID: 33927630 | PMCID: PMC8076853
Review Free full text in Europe PMC
Natural Phytochemicals as Novel Therapeutic Strategies to Prevent and Treat Parkinson's Disease: Current Knowledge and Future Perspectives.
Oxid Med Cell Longev, 2021:6680935, 25 May 2021
Cited by: 20 articles | PMID: 34122727 | PMCID: PMC8169248
Review Free full text in Europe PMC
Go to all (19) article citations
Similar Articles
To arrive at the top five similar articles we use a word-weighted algorithm to compare words from the Title and Abstract of each citation.
Feeding the beast: can microglia in the senescent brain be regulated by diet?
Brain Behav Immun, 43:1-8, 30 Oct 2014
Cited by: 28 articles | PMID: 25451610 | PMCID: PMC4258457
Review Free full text in Europe PMC
Aging, microglial cell priming, and the discordant central inflammatory response to signals from the peripheral immune system.
J Leukoc Biol, 84(4):932-939, 01 Oct 2008
Cited by: 223 articles | PMID: 18495785 | PMCID: PMC2538600
Review Free full text in Europe PMC
Microglia across the lifespan: from origin to function in brain development, plasticity and cognition.
J Physiol, 595(6):1929-1945, 29 May 2016
Cited by: 248 articles | PMID: 27104646
Review
Healthy Brain Aging Modifies Microglial Calcium Signaling In Vivo.
Int J Mol Sci, 20(3):E589, 30 Jan 2019
Cited by: 29 articles | PMID: 30704036 | PMCID: PMC6386999
Funding
Funders who supported this work.
NIA NIH HHS (5)
Grant ID: R01 AG016710
Grant ID: AG16710
Grant ID: R01 AG023580-05
Grant ID: R01 AG016710-09
Grant ID: R01 AG023580
NIMH NIH HHS (2)
Grant ID: MH069148
Grant ID: R01 MH069148