Abstract
Free full text

Erythropoietin and hypoxia increase erythropoietin receptor and nitric oxide levels in lung microvascular endothelial cells
Abstract
Acute lung exposure to low oxygen results in pulmonary vasoconstriction and redistribution of blood flow. We used human microvascular endothelial cells from lung (HMVEC-L) to study the acute response to oxygen stress. We observed that hypoxia and erythropoietin (EPO) increased erythropoietin receptor (EPOR) gene expression and protein level in HMVEC-L. In addition, EPO dose- and time- dependently stimulated nitric oxide (NO) production. This NO stimulation was evident despite hypoxia induced reduction of endothelial NO synthase (eNOS) gene expression. Western blot of phosphoe-NOS (serine1177) and eNOS and was significantly induced by hypoxia but not after EPO treatment. However, iNOS increased at hypoxia and with EPO stimulation compared to normal oxygen tension. In accordance with our previous results of NO induction by EPO at low oxygen tension in human umbilical vein endothelial cells and bone marrow endothelial cells, these results provide further evidence in HMVEC-L for EPO regulation of NO production to modify the effects of hypoxia and cause compensatory vasoconstriction.
1. Introduction
Erythropoietin (EPO) is a hypoxia-inducible cytokine required for production of mature erythrocytes. EPO acts by binding to and inducing expression of the erythropoietin receptor (EPOR). Beyond erythropoiesis, EPO and EPOR elicit response in numerous tissues, particularly in endothelial cells [1,2]. The ability for EPO to stimulate cell proliferation and chemotaxis in endothelial cells was first demonstrated in vitro and then in vivo in the chick chorioallantoic membrane and mouse uterine endometrium [3,4]. The pleiotrophic nature of EPO proliferative and survival response is observed in a variety of non-hematopoietic cells including tumor cells [5,6]. Moreover, EPO in combination with low oxygen tension can increase the endothelial capacity to produce nitric oxide (NO) by induction of both EPOR and endothelial nitric oxide synthase (eNOS) [7]. EPO activation of NO production in murine erythrocytes has also been reported [8]. EPO stimulated production of NO may compensate for the enhanced NO scavenging in the presence of cell-free hemoglobin followed by haptoglobin binding to facilitate clearance, especially with reduced oxygen availability [9,10]. Additionally, EPO induced neovascularisation through endothelial progenitor cells recruitment from the bone marrow associated with coronary heart disease and possibly acting in conjunction with stimulated VEGF production by cardiomyocytes [11,12]. Several in vivo studies have demonstated EPO protective effect to endothelial cells in diverse models of vascular disease [13,14].
Endothelial cells exibited specific response in adaptation to low oxygen tension in lung [15]. Acute exposure to hypoxia resulted in vasoconstriction of pulmonary arteries, increased pulmonary arterial pressure and redistribution of blood flow from basal to the apical level of lung. In chronic hypoxic lung disease dysfunction of mechanisms regulating vascular tone and remodelling of the pulmonary vasculature contribute to the development of sustained pulmonary hypertension [16]. Chronic hypoxia stimulated polycytemia results in increased blood viscosity and pulmonary hypertension.
NO has a central role in modulation of pulmonary vascular tone. Three isoforms of the enzyme responsible for the NO production are endothelial, inducible (iNOS) and neuronal (nNOS) [17,18]. All three isoforms are increased in chronic hypoxic pulmonary hypertension [19]. eNOS and nNOS are constitutively expressed NOS isoforms and require calcium for activation, while iNOS is calcium independent but inducible by cytokines. In the normal murine pulmonary circulation nNOS does not modulate tone, whereas eNOS-derived NO is the principle mediator of endothelium-dependent vasodilation in the pulmonary circulation, and both eNOS and iNOS play a role in modulating basal tone chronically [20]. eNOS is the principal isoform expressed in the normal pulmonary vasculature [21].
To clarify the response of lung endothelial cells to EPO stimulation, we examined the levels of EPOR and eNOS/iNOS expression in cultured endothelial cells at normoxia and low oxygen tension. We found that EPO increased expression of EPOR, particularly at low oxygen tension. EPO had little effect on NO production at normoxia. At hypoxia, concomitant with the increase in EPOR expression, EPO increased production of NO up to 4 hr. This EPO induction of NO was NOS dependent and evident despite hypoxia induced down regulation of eNOS mRNA and protein expression. The activity of eNOS is regulated primarily by post-translational mechanisms including phosphorylation at multiple sites, but one of the most studied sites is the activation of serine 1177. However, ratio for phosphorylated eNOS at serine 1177 and eNOS, showed increased in there activity at low oxygen but not after EPO stimulation. In contrast, Western blotting showed that iNOS increased at low oxygen tension and even further when combined with EPO treatment. These data suggest that the low level of NO induced at low oxygen tension by EPO stimulation in lung endothelial cells is NOS dependent and concomitant with increase in the low level of iNOS protein expression but decrease in eNOS activity.
2. Materials and methods
2.1 HMVEC-L cell culture
Human microvascular endothelial cells from lung (HMVEC-L; Clonetics Endothelial Cell System, Lonza, Walkersville, MD) were cultured in endothelial basal media (EGM-2MV) containing 5% FBS and cytokines under 5%CO2 with balanced 95% room air. Before exposure to 2% oxygen, cells were washed in HEPES buffer (N-2-hydroxyethylpiperazine-N’-2-ethanesulfonic acid) and plated in EBM-2 containing 1% FBS and cytokines. Incubator (Forma Scientific, Marietta, OH) with low oxygen control capability was used to generate reduced oxygen tension.
2.2 Real-time quantitative RT-PCR
At third passage, HMVEC-L were plated at cell density of 3000 cells in 35 mm culture dishes at normal and reduced oxygen tensions with and without EPO (5U/ml). After two days, the cells were harvested, RNA was isolated using STAT 60 (Tel-TEST, Friendswood, TX) and treated with RNase-Free DNase (Promega, Madison, WT). Total RNA (1mg) of each sample was used for cDNA synthesis using murine leukemia virus (MuLV) reverse transcriptase (RT) and oligo d(T)16 (Applied Biosystems, Foster City, CA). Quantitative real-time RT-PCR analysis was performed using the ABI PRISM 7700 Detection System (Applied Biosystems). The conditions and Taqman oligonucleotide probes (Applied Biosystems) were used as previously described [7]. β-actin was used as an internal control for the total amount of RNA analyzed.
2.3 Protein analysis
HMVEC-L cells were plated in EBM-2 media with and without 5 U/ml EPO. After 48 hours, cells were washed with HEPES buffer, treated with lysis buffer and detached from the plate. The cell lysate was centrifuged for 10 minutes and the supernatant was transferred to a new tube. For EPOR analysis, 1 mg of protein were incubated with EPOR antibody (Santa Cruz Biotechnology, Santa Cruz, CA) overnight. The complex was captured with protein A-Agarose (Santa Cruz Biotechnology) for 2 hours at 4°C, washed 2 times and incubated at 70°C for 10 minutes. The proteins were separated by sodium dodecyl sulfate-polyacrylamide gel electrophoresis (SDS-PAGE) and transfered to a nitrocellulose membrane. The membrane was blocked with 5% milk-0,1% Tween 20 for 1 hour at room air and probed with primary antibody specific for EPOR antibody (Santa Cruz Biotechnology), 1:1000 at 4°C overnight. Hyperfilm was developed to visualize the secondary antibody by enhanced chemilumniscence (ECL; Amersham Pharmacia Biotech, Piscataway, NJ). For Western blotting using anti-eNOS (BD Transduction Laboratories, San Jose, CA), anti-phospho-eNOS (Ser1177), and anti-iNOS (Cell Signaling Technology, Inc) antibodies, HMVEC-L were treated with EPO (5 U/ml) for 48 hours. Cells were washed with HEPES, treated with lysis buffer and scraped from the plate. The proteins were separated by SDS-PAGE gel electrophoresis and transfered to a nitrocellulose membrane, blocked with primary antibody overnight at 4°C and visualized by chemiluminescence. The membrane was stripped for one hour, washed, blocked and incubated with the β-actin antibody (Sigma, St Louis, MO) overnight at 4°C and then visualised. The Java image-processing program inspired by NIH image was used for quantitative analysis.
2.4 Ozone-based chemiluminescent determination of nitrite/nitrate in supernatant of cell culture
For only nitrite measurement, confluent HMVEC-L in 6-well plates were washed once with HEPES buffer (BioWhittaker Inc.) and EBM-2 was added (total volume 2ml). HMVEC-L were pretreated for 30 minutes with 1 mM NG-Nitro-l-arginine-methyl ester (L-NAME, Alexis Biochemicals). The cells were exposed to EPO (1, 5, 10 U/ml) in the presence of l-arginine (Sigma-Aldrich). After incubation period, the supernatants of treated cells were injected in reductants in line with the Sievers Model 280 NO analyser (Sievers, Boulder, CO). NO was measured by nitrite reduction in acidified KI: 7 ml of glacial acetic acid, 2ml of distilled water, 50 mg of KI, a crystal of iodine was added to yield a concentration of 6–20 mmol/l (Sigma-Aldrich). Helium was bubbled through the reaction mixture. For combined nitrite/nitrate measurement, confluent HMVEC-L in small flasks were washed once with HEPES buffer and EBM-2 was added (total volume 5ml). After EPO treatment (5 U/ml) and 4 hours of incubation, nitrite levels were measured as previously described, whereas nitrate levels were measured using vanadium (III) chloride in hydrochloric acid at 90°C with the Sievers Model 280 NO analyser.
2.5 Statistical analysis
The one way analysis of variance (ANOVA) and Dunnett’s post tests were applied using Prism 4 software (GraphPad Software Inc., San Diego, CA).
3. Results
3.1 EPOR expression in HMVEC-L
Our previous results on EPO activity in endothelial cells indicated that the optimal concentration of EPO stimulation of EPOR expression is 5 U/ml [7]. To investigate EPOR expression in HMVEC-L, cells were exposed to normal (21% O2) and low (2% O2) oxygen tension for 48 hours. We observed that EPO (5 U/ml) induced EPOR mRNA in lung endothelial cells at normal oxygen tension (Figure 1A). The increase of EPOR mRNA was also observed at 2% O2 (P<0.01) and adding EPO to the culture media further doubled EPOR expression (Figure 1A). Western blotting showed that EPO treatment of HMVEC-L, under hypoxic condition, induced the EPOR protein more than three fold compared to cultures at 21% O2 with and without EPO (Figure 1B). Therefore, while EPO had no effect on EPOR protein level at normoxia, quantification of the EPOR bands from Western blotting (Figure 1C) showed significant EPOR induction after EPO stimulation at low oxygen tension (P<0.01). EpoR appeared to increase with hypoxia, but this increase is not significant. These results demonstrated stimulative effects of EPO and hypoxia on total EPOR levels in HMVEC-L.
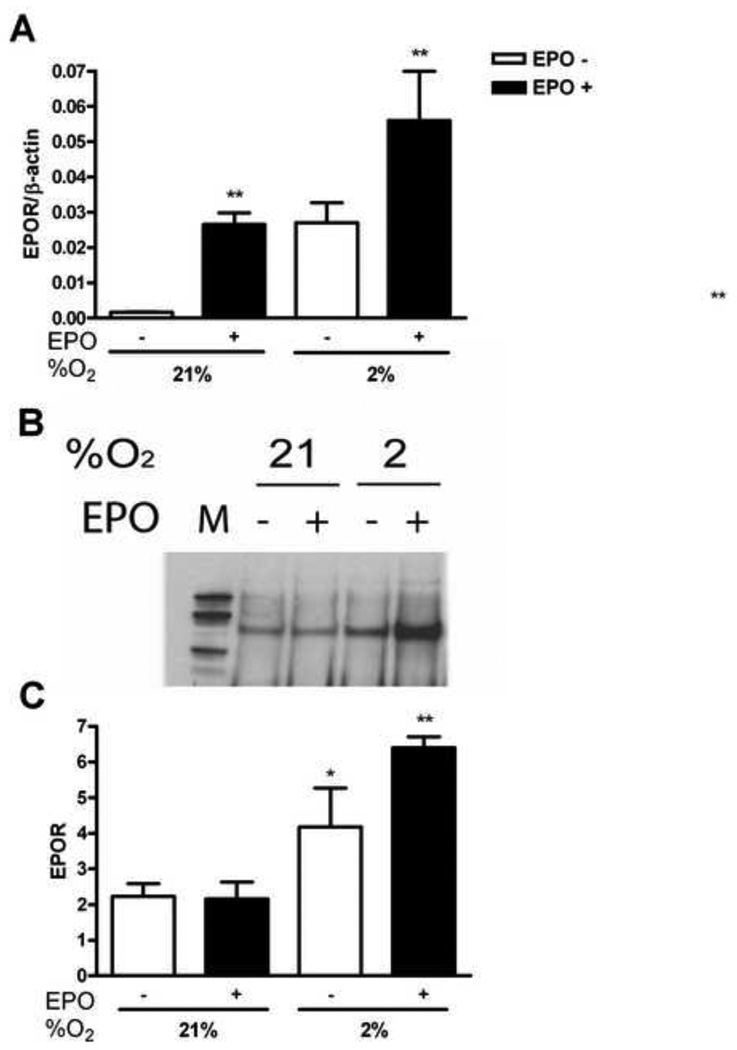
(A) EPOR expression was determined by quantitative real-time PCR at 21% and 2% O2, with (black bars) and without (open bars) EPO (5 U/ml) treatment. EPOR gene expresion was induced with EPO after 48 hours at 21% and 2% O2. Results were normalized to β-actin expression. Values are mean ± SEM (n=3). **P < 0.01 compared to nontreated cells. (B) EPOR protein induction by EPO in normoxia vs. hypoxia as measured by Western blotting. (C) Quantification of EPOR Western blotting showed significant induction up to three fold at 2% O2 after EPO treatment. Open bars demonstrate 21% and 2% of oxygen and black bars cells treated with EPO. Values are mean ± SEM (n=3). **P < 0.01 compared to samples under normoxia.
3.2 NO production in HMVEC-L
We previously found that EPO stimulated NO production in selected endothelial cells through eNOS activation that was reflected in increased eNOS expression and phosphorylation [7]. HMVEC-L were treated with different concentrations of EPO (1 U/ml, 5 U/ml and 10 U/ml) at 21% and 2% oxygen. The NO production after EPO stimulation was mesured at 30 minutes (Figure 2), 1 hour (Figure 3) and 4 hours (Figure 4). EPO did not induce statistically significant changes in NO production at 21% oxygen at all time points (Figure 2B, ,3B3B and and4B).4B). L-NAME treatment in combination with EPO decreased NO production compared with EPO alone.
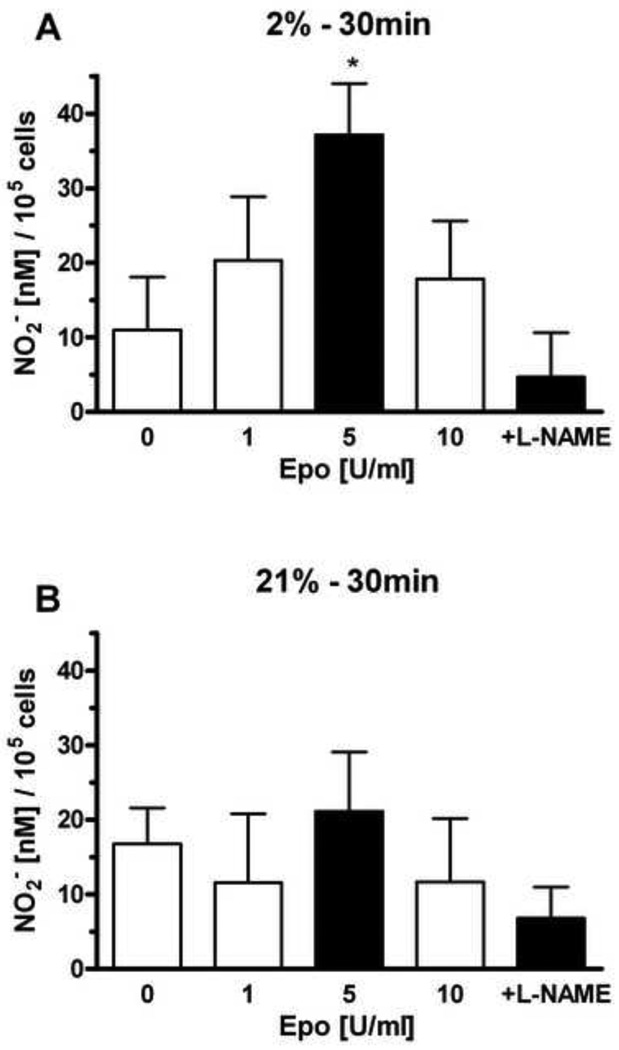
Effects of 1, 5 and 10 U/ml of EPO stimulation on NO2− production after 30 minutes were determined at 2% O2 (A) and 21% oxygen (B). The effect of EPO treatment at 5U/ml on NO2− production is shown as a shaded bar. NO production was measured as released nitrite in the supernatant per 105 cells. 1mM L-NAME was added in the presense of 5 U/ml of EPO. Values are mean ± SEM (n=3). *P < 0.05 compared to control and L-NAME treatment.
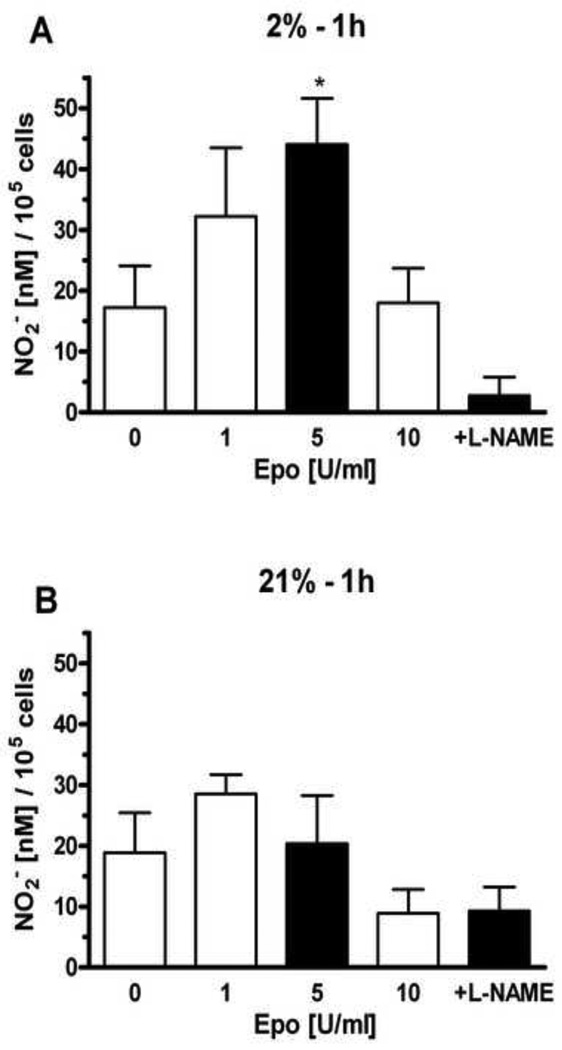
Cells were treated with 1, 5 and 10 U/ml of EPO on NO2− production after 1 hour were deteremined at 2% (A) and 21% oxygen (B). Shaded bar with 5U/ml of EPO represents the optimum effect on NO2− production. NO production was measured as released nitrite in the supernatant per 105 cells. 1 mM L-NAME suppresed NO induction stimulated by 5U/ml of EPO. Values are mean ± SEM (n=3). *P < 0.05 compared to control and L-NAME treatment.
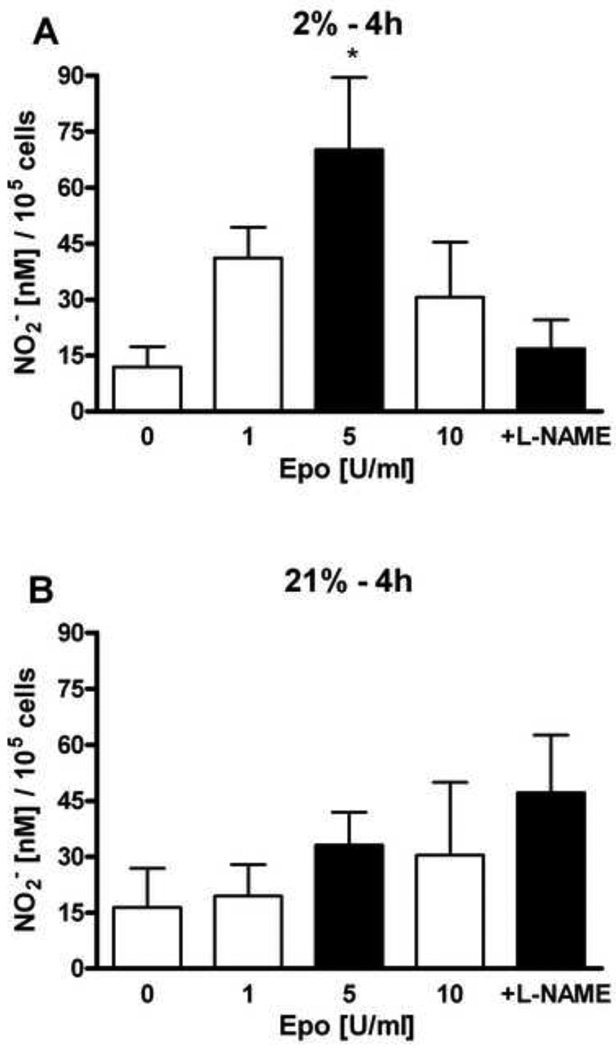
Cells were treated with 1, 5 and 10 U/ml of EPO on NO2− production after 4 hours were deteremined at 2% (A) and 21% oxygen (B). Shaded bar with 5U/ml of EPO represents the optimum effect on NO2− production. NO production was measured as released nitrite in the supernatant per 105 cells. 1 mM L-NAME suppresed NO induction stimulated by 5U/ml of EPO. Values are mean ± SEM (n=3). *P < 0.05 compared to control and L-NAME treatment.
HMVEC-L cultured at 2% oxygen exhibited a greater response to EPO, consistent with the up regulation of EPOR expression (Figure 1). The optimum for NO production was observed with 5 U/ml of EPO at 30 minutes, 1 hour and 4 hours (Figure 2A, ,3A3A and and4A).4A). NO induction increased with time and appeared greatest after 4 hours. Addition of NOS inhibitor L-NAME, decreased the EPO induction of NO at all three time points at 2% oxygen.
3.3 Nitrite and nitrate production in HMVEC-L
Endothelial NO synthesis is important in controlling vascular tone and blood pressure [22]. Under normal physiological conditions, nitrite, along with NO and nitrate, is part of a complex biological cycle in mammals [23]. NO itself is short-lived, as it is rapidly oxidized to nitrite in aqueous solutions, and directly oxidized to nitrate in the presence of superoxide or oxyhemoglobin [24]. Inorganic nitrate synthesis results from NO oxidation and may be an important storage form of reactive nitrogen oxides, which can be reduced back to nitrite and then NO under certain physiological and pathological conditions [23]. In HMVEC-L released NO was monitored using nitrate and nitrite levels as an index of NO production.
After 4 hours of HMVEC-L stimulation by EPO (5 U/ml) nitrite levels were significantly induced at low oxygen tension (Figure 5A). There was about the same production of nitrite in HMVEC-L cultured at 21% and 2% of oxygen. However, overall nitrate production in cells was as expected higher in comparison to nitrite levels and significantly stimulated by hypoxya itself (Figure 5B). EPO remarkably stimulated nitrate production both at normal and low oxygen tension (Figure 5B), suggesting that nitrite rapidly converts to nitrate.
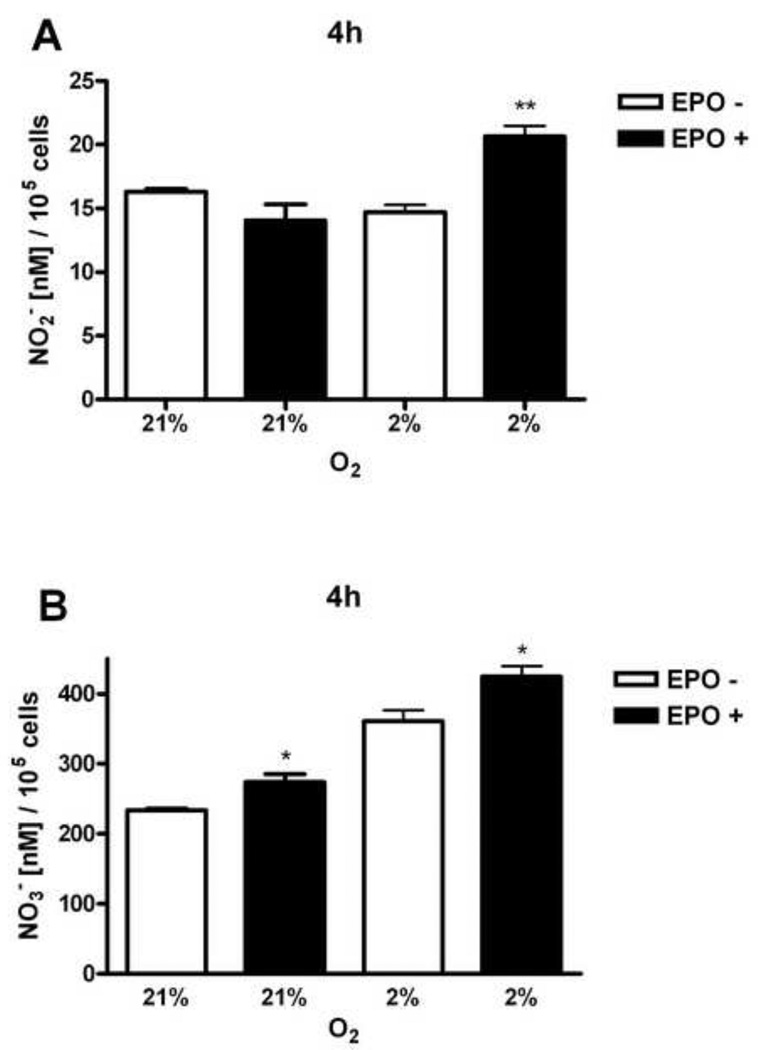
Cells were cultured at 21% and 2% oxygen with (black bars) and without (open bars) 5 U/ml of EPO for 4 hours. (A) Nitrite levels (NO2−) showed the induction after EPO stimulation at 2% oxygen. (B) Nitrate levels (NO3−) were induced with EPO at normal and low oxygen tension. Values are mean ± SEM (n=3). *P < 0.05 compared to at normoxia. **P < 0.01 compared to cells at normoxia.
3.4 eNOS, phosphoNOS and iNOS expression in HMVEC-L
The three known isoforms of NOS were identified in the lung, two constitutive NOS isoforms are neuronal (nNOS) and endothelial (eNOS), they are regulated by calcium and calmodulin ions and release NO in small (nanomolar) quantities. However, this quite limited NO production by constitutive NOS are sufficient to keep the extremely vasodilated baseline systemic vascular tone stable, inhibit the interaction of leukocytes with the endothelium, inhibit platelet aggregation and control cell proliferation [25]. The third isoform of NOS is inducible iNOS isoform, independent of calcium and calmodulin ions, which promotes the release of greater (micromolar) amounts of NO. It is known that iNOS isoform can be induced by inflammatory stimuli [26].
Expression of eNOS and iNOS were examined in HMVEC-L. Cells were cultured with and without EPO for 48 hours and we found that expression of eNOS mRNA was significantly decreased at 2% oxygen (P<0.01, Figure 6A). This change in eNOS expression is analogous to the 2- to 4-fold decrease we previously observed in primary endothelial cells from human umbilical vein (HUVEC) and artery (HUAEC) when cells were cultured at reduced oxygen tension [7]. Adding EPO (5 U/ml) did not affect expression of eNOS mRNA in HMVEC-L (data not shown). Western blotting of eNOS showed that expression was reduced up to 2-fold at 2% O2. Moreover, antibody specific for eNOS phosphorylated at serine 1177 followed the decrease in protein and mRNA expression of eNOS (Figure 6B,C). However, when we examined the ratio phospho eNOS to eNOS we found statisticaly significant changes in expression but only at low oxygen (Figure 6D). The reduction of eNOS expression at the mRNA and protein levels by hypoxia, not significantly reversed by EPO treatment, may account for the relatively low EPO induction of NO production in HMVEC-L in comparison to HUVEC or HUAEC where NO production was an order of magnitude greater following EPO treatment [7]. Interestingly, iNOS protein was induced by hypoxia and even more in combination with EPO stimulation. These data suggest that small amounts of NO produced in HMVEC-L under low oxygen tension could be accompanied with induction of iNOS protein with hypoxia and more with EPO, but not eNOS in lung microvascular cells.
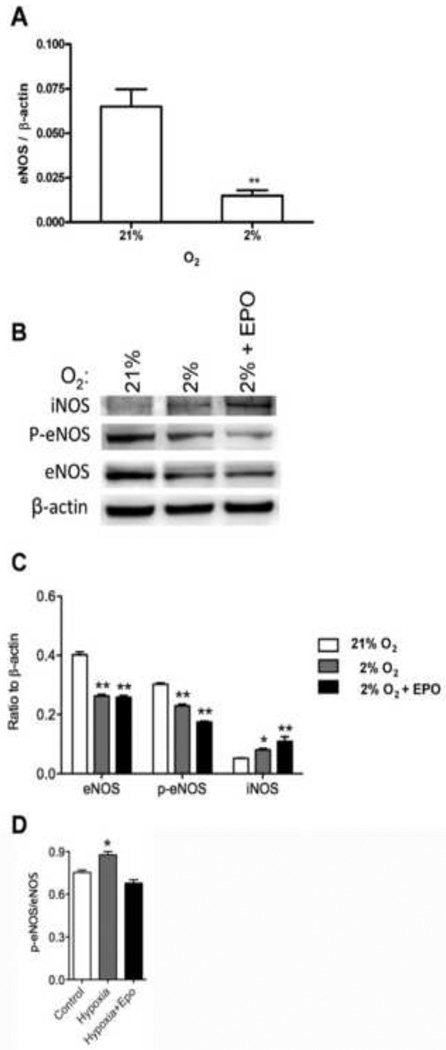
(A) eNOS expression was determined by quantitative real-time RT-PCR at 21% and 2% O2. There was a significant decrease of eNOS expression at 2% O2. Results were normalized to β-actin expression. Values are mean ± SEM (n=3). **P < 0.01 compared to cells at normoxia. (B) Western blotting analysis confirmed the decreased expression of eNOS and p-eNOS at 2% O2 that persisted with EPO stimulation. iNOS expression was induced at 2% oxygen and even more with 5 U/ml of EPO. (C) Quantification of eNOS, p-eNOS and iNOS in relation to β-actin. (D) Quantification of p-eNOS/eNOS ratio from Western blotting. *P < 0.05 compared to at normoxia. **P < 0.01 compared to cells at normoxia.
4. Discussion
Hypoxia increased EPOR mRNA and protein levels in HMVEC-L that are further induced by EPO particularly at low oxygen tension. Induction of EPOR mRNA by EPO is regulated in part through activation of GATA transcription factors as observed in erythroid, myoblast and neuronal cells [27,28,29]. In addition, EPOR reporter gene assays show a direct response of EPOR promoter activity to reduced oxygen tension as well as increased NO production in neuronal cells [30], providing insight on the induction of EPOR expression by EPO and hypoxia. Hypoxia reduced eNOS mRNA and protein levels at 2% O2. This change in phospho-eNOS/eNOS is small and reduction in eNOS protein is considerably larger so that is very likely that there is an overall reduction in eNOS activity. EPO stimulated NO production in HMVEC-L at low oxygen tension, although the level of NO production was markedly lower than the level of EPO induced NO in HUVEC reflecting the overall reduction of eNOS by hypoxia [7]. EPO stimulated nitrite and even more nitrate production at normal and low oxygen tension, suggesting that nitrite rapidly converts to nitrate. In contrast to eNOS, iNOS was induced with low oxygen and EPO stimulation. Compared with previous reports of EPO stimulation of endothelial NO production, these data indicate variation in endothelial response in EPO-induced NO at hypoxia consistent with endothelial cell diversity in tissue specific response.
The pulmonary endothelium is important for the maintenance of the blood flow in the pulmonary vasculature. When the lungs are exposed to low oxygen, pulmonary arteries exhibit vasoconstriction and redistribution of blood flow [15]. Chronic hypoxia decreases the luminal diameter of the small pulmonary arteries and increases vascular resistence, contributing to the development of hypertension [31]. Also, chronic hypoxia stimulates production of erythrocytes and polycythemia, resulting in increased blood viscosity and can contibute to pulmonary hypertension [32]. Beyond excessive erythrocytosis and/or polycythemia, a variety of other factors have been implicated in the mechanisms of pulmonary hypertension such as hypoxia, vascular remodeling and NO. In transgenic mice with constitutively overexpressed human EPO gene, pulmonary artery pressure was increased in vivo but was reduced in blood-free perfused lungs [33]. These mice have polycythemia but normal blood pressure, heart rate and cardiac output and in pulmonary vasculature elevated prostacyclin production, eNOS expression and reduced vascular smooth muscle thickness. Since these mice have elevated pulmonary hypertension in vivo but not in vitro, it suggests that their pulmonary hypertension is due to the increased blood viscosity. With respect to tissue hypoxia, our results showed that eNOS expression was decreased when HMVEC-L were exposed to 2% O2 for 48 hours, suggesting that the long incubation and low oxygen tension dramatically changed eNOS expression localy in the pulmonary tissue. Stimulation with 5 U/ml of EPO did not reverse the decreased eNOS expression, in contrast to EPO induction of eNOS in HUVEC [7]. The iNOS isoform can be induced by inflammatory stimuli [26]. Recent evidence has revealed that NO production is increased in lung diseases accompanied by intense inflammation, such as acute respiratory distress syndrome (ARDS) and that NO derived from iNOS might aggravate such diseases [34]. It has been observed that patients with ARDS present elevated lung concentrations of NO metabolites (nitrite and nitrate) and 3-nitrotyrosine [35]. Because of this findings it is proposed that selective iNOS inhibition can have beneficial effects in the treatment of lung diseases accompanied by inflammation and pulmonary arterial hypertension, but not yet clinically validated. In case of HMVEC-L the level of iNOS was low compare to eNOS. But after 48 hours iNOS protein increased as oxygen decreased to 2% and further more after EPO stimulation. Of note, the amount of iNOS protein is only one of several factors that regulate iNOS generated NO production [36]. However, these data suggest that acute exposure of HMVEC-L to low oxygen may produce NO via a mechanism independent of eNOS activation, but is insuficient to protect cells form hypoxic stress, and consequently leads to vasoconstriction.
The differential response of various endothelial beds to EPO may explain in part the role of EPO in modulating endothelial response related to the pulmonary vasculature. Several reports suggest that EPO may serves as a cytokine that elicits mobilisation of endothelial progenitor cells promoting the repair process of the injured pulmonary endothelium [14]. EPOR-null mutant mice expressing EPOR exclusevly in the erythroid lineage (EPOR−/− rescued mice) showed accelerated pulmonary hypertension and pulmonary vascular remodeling, illustrating the importance of EPO and EPOR for pulmonary endothelial function [14]. Our data suggest that primary endothelial cells from lung after stimulation with EPO at low oxygen tension induced EPOR and increased NO production, but did not significantly affect the inhibition of eNOS expression, while iNOS began to induce within 48 hours. In EPOR−/− rescued mice, when bone-marrow derived endothelial progenitor cells were intravenously infused, incorporation of endothelial cells was significantly impaired comparing to wild type animals, again suggesting the significance EPO and EPOR in endothelial cells. In wild-type mice after three weeks exposure to 10% oxygen, endothelial cells were incorporated into the pulmonary endothelium and EPOR and eNOS were induced [14]. On the other hand, NO is also a reactive radical capable of tissue injury either directly or after reacting with other radicals. There is evidence that when rats are exposed to 10% oxygen, NO production is increased during the first days of hypoxia, mostly by the iNOS isoform [36]. It was suggested that transient iNOS induction in the pulmonary vascular wall at the beginning of chronic hypoxia participates later in the pathogenesis of pulmonary hypertension [37]. Our data also suggest that after 48 hours of exposure to 2% oxygen, there was an increase in iNOS protein that increased further with EPO treatment.
Taken together, our data suggest that the increase of plasma EPO level in response to oxygen stress results in upregulated EPOR in lung endothelial cells accompanied by the NOS dependent small induction of NO that may be insufficient to counteract hypoxic vasoconstriction.
Acknowledgments
This research was supported by the Intramural Research Program of the National Institute of Diabetes and Digestive and Kidney Diseases and by grant from the Serbian Ministry of Science and Technological Development [175053].
Footnotes
Publisher's Disclaimer: This is a PDF file of an unedited manuscript that has been accepted for publication. As a service to our customers we are providing this early version of the manuscript. The manuscript will undergo copyediting, typesetting, and review of the resulting proof before it is published in its final citable form. Please note that during the production process errors may be discovered which could affect the content, and all legal disclaimers that apply to the journal pertain.
References
Full text links
Read article at publisher's site: https://doi.org/10.1016/j.cyto.2011.01.015
Read article for free, from open access legal sources, via Unpaywall:
http://rimi.imi.bg.ac.rs/bitstream/123456789/341/1/338.pdf
Citations & impact
Impact metrics
Citations of article over time
Alternative metrics
Smart citations by scite.ai
Explore citation contexts and check if this article has been
supported or disputed.
https://scite.ai/reports/10.1016/j.cyto.2011.01.015
Article citations
Neuronal nitric oxide synthase required for erythropoietin modulation of heart function in mice.
Front Physiol, 15:1338476, 02 Apr 2024
Cited by: 0 articles | PMID: 38628440 | PMCID: PMC11019009
Beauty and the beast: host microRNA-155 versus SARS-CoV-2.
Hum Cell, 36(3):908-922, 27 Feb 2023
Cited by: 9 articles | PMID: 36847920 | PMCID: PMC9969954
Review Free full text in Europe PMC
Erythropoietin receptor regulates tumor mitochondrial biogenesis through iNOS and pAKT.
Front Oncol, 12:976961, 16 Aug 2022
Cited by: 4 articles | PMID: 36052260 | PMCID: PMC9425774
Low serum erythropoietin levels are associated with fatal COVID-19 cases at 4,150 meters above sea level.
Respir Physiol Neurobiol, 292:103709, 02 Jun 2021
Cited by: 10 articles | PMID: 34087493 | PMCID: PMC8169280
Altitude and COVID-19: Friend or foe? A narrative review.
Physiol Rep, 8(24):e14615, 01 Jan 2021
Cited by: 32 articles | PMID: 33340275 | PMCID: PMC7749581
Review Free full text in Europe PMC
Go to all (31) article citations
Similar Articles
To arrive at the top five similar articles we use a word-weighted algorithm to compare words from the Title and Abstract of each citation.
Erythropoietin and hypoxia stimulate erythropoietin receptor and nitric oxide production by endothelial cells.
Blood, 104(7):2073-2080, 17 Jun 2004
Cited by: 190 articles | PMID: 15205261
Nitric oxide and hypoxia stimulate erythropoietin receptor via MAPK kinase in endothelial cells.
Microvasc Res, 92:34-40, 08 Feb 2014
Cited by: 16 articles | PMID: 24518819 | PMCID: PMC3982609
Endothelial cell regulation of nitric oxide production during hypoxia in coronary microvessels and epicardial arteries.
J Cell Physiol, 182(3):359-365, 01 Mar 2000
Cited by: 35 articles | PMID: 10653602
Erythropoietin action in stress response, tissue maintenance and metabolism.
Int J Mol Sci, 15(6):10296-10333, 10 Jun 2014
Cited by: 70 articles | PMID: 24918289 | PMCID: PMC4100153
Review Free full text in Europe PMC
Funding
Funders who supported this work.
Intramural NIH HHS (1)
Grant ID: ZIA DK025061-35
National Institute of Diabetes and Digestive and Kidney Diseases
Serbian Ministry of Science and Technological Development (1)
Grant ID: 175053