Abstract
Free full text

Cytokine production by primary human macrophages infected with highly pathogenic H5N1 or pandemic H1N1 2009 influenza viruses
Abstract
Highly pathogenic H5N1 avian influenza viruses have caused infection in humans, with a high mortality rate, since 1997. While the pathogenesis of this infection is not completely understood, hypercytokinaemia and alveolar macrophages are thought to play a role. To gain further insight into the cytokine-mediated pathogenesis of this infection in humans, we measured various cytokines produced by primary human macrophages infected with H5N1, pandemic H1N1 or seasonal influenza viruses. We found that many cytokines were produced at higher levels on infection with the H5N1 strains tested compared with seasonal influenza viruses. Interestingly, the extent of cytokine induction varied among the H5N1 strains and did not correlate with replicative ability in macrophages. Further, a pandemic H1N1 virus induced higher levels of several cytokines compared with seasonal viruses and some H5N1 strains. Our results demonstrate that high cytokine induction is not a universal feature of all H5N1 viruses.
Highly pathogenic H5N1 avian influenza virus infection of humans has continued since 1997, with a mortality rate of nearly 60% (Abdel-Ghafar et al., 2008; Claas et al., 1998). The mechanism underlying the pathogenesis of these infections is not fully understood, although hypercytokinaemia has been linked to the high pathogenicity (de Jong et al., 2005, 2006; Peiris et al., 2004; To et al., 2001; Uiprasertkul et al., 2005).
Alveolar macrophages are central players in both innate and adaptive immune responses to respiratory infection. In studies in vitro, human primary macrophages infected with H5N1 viruses produce higher levels of cytokines compared with those infected with seasonal viruses (Cheung et al., 2002; Guan et al., 2004; Lee et al., 2009; Mok et al., 2009; Woo et al., 2010). However, the H5N1 viruses examined in these studies were isolated before 2005, and the number of cytokines studied was limited.
In 2009, a novel swine-origin H1N1 influenza virus (2009 pandemic H1N1 virus; pdm H1N1) caused a pandemic (Khan et al., 2009). During the first phase of this pandemic, 9% of patients were hospitalized with pneumonia, respiratory failure or acute respiratory distress syndrome (Dawood et al., 2009; Louie et al., 2009). The pdm H1N1 virus induced cytokine production and gene expression in dendritic cells and primary human macrophages at levels similar to or below those induced by seasonal viruses (Osterlund et al., 2010; Woo et al., 2010).
To gain further insight into the cytokine-mediated pathogenesis of influenza virus infection in humans and to assess the possible relationship between hypercytokinaemia and the pathogenicity of influenza viruses in humans, we measured 48 different cytokines in culture supernatants of primary human macrophages infected with H5N1 viruses of various clades or subclades, a pdm H1N1 virus and seasonal influenza viruses.
First, we prepared primary human macrophages from PBMCs, which were separated from the buffy coat of five different donors, and incubated them with granulocyte-macrophage colony stimulating factor (GM-CSF) (5 ng ml−1). Our research protocol was approved by the Research Ethics Review Committee of the Institute of Medical Science, University of Tokyo (approval numbers 18-15-0129 and 19-24-0430). Macrophage differentiation was confirmed by using FACS analysis for CD68 high and CD14 low (Peng et al., 1999; Shimizu et al., 2007; Taylor et al., 2005). The phenotype of the human macrophages derived from the peripheral blood monocytes used in this study is similar to that of human alveolar macrophages (Shimizu et al., 2007; Taylor et al., 2005), suggesting that this is a useful model with which to study the dynamics of influenza virus infection in the human lung.
To evaluate the susceptibility of primary human macrophages to avian H5N1 and human influenza viruses, we examined whether α-2,3- and α-2,6-linked sialic acids, which are avian and human virus receptors, respectively, were present on the cells by using lectins specific for these sialo-oligosaccharides: Sambucus nigra agglutinin (SNA) for α-2,6-linked and Maackia amurensis agglutinin II (MAAII) (Vector laboratories) for α-2,3-linked sialic acids. Cells were incubated with biotinylated lectins and then conjugated with streptavidin–Alexa-Fluor 488 (Invitrogen). FACS analysis showed a right-shift compared with controls without lectins, indicating that both α-2,6- and α-2,3-linked sialic acids were expressed on the primary human macrophages, as they were on Madin–Darby canine kidney (MDCK) cells, but not chicken embryo fibroblasts (CEF), which predominantly express MAAII-positive oligosaccharides (Fig. 1a).
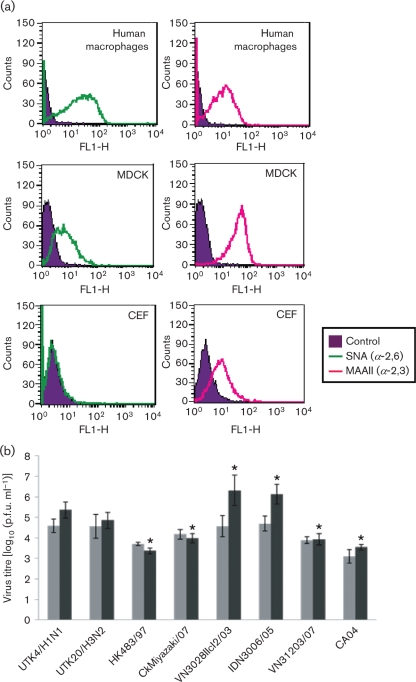
Expression of α-2,6- and α-2,3-linked sialic acids on the surface of human macrophages (a) and viral replication in macrophages (b). (a) To detect α-2,6- and α-2,3-linked sialic acids on human macrophages, biotinylated lectins (SNA, specific for α-2→6 linkages, and MAAII, specific for α-2→3 linkages) were incubated with cells and with streptavidin–Alexa-Fluor 488 conjugate and then analysed by FACS. MDCK cells and CEF served as controls. The profile shows the number of cells as a function of the log fluorescence intensity of α-2,6- and α-2,3-specific lectin-reactive oligosaccharides on the cell surface. (b) Primary human macrophages were infected with various influenza viruses at an m.o.i. of 2. At 12 and 36 h p.i., supernatants were collected and virus was titrated by using plaque assays in MDCK cells. Light-shaded bars show titres at 12 h p.i. and dark-shaded bars show titres at 36 h p.i. Data were obtained independently with macrophages derived from five individuals. The results are expressed as means±sd. *, P<0.01, significant difference compared with seasonal virus (UTK4/H1N1 and UTK20/H3N2); one-way ANOVA.
Since our receptor analysis indicated the replicative potential of both avian and human viruses in human macrophages, we infected the cells with H5N1, seasonal influenza or pdm H1N1 viruses at an m.o.i. of 2 and measured the virus output in infected-cell supernatants by means of a plaque assay with MDCK cells. Virus replication was assessed in primary human macrophages derived from five different donors, and the means±sd for macrophages from the five individuals are presented in Fig. 1(b). At 12 h post-inoculation (p.i.), we found no significant difference in titres among the virus strains. However, at 36 h p.i., some H5N1 strains, such as A/Vietnam/UT3028II/2003 clone2 (clade 1; VN3028IIcl2/03) and A/Indonesia/UT3006/2005 (clade 2.1.3; IDN3006/05), grew significantly better than did the seasonal viruses A/Kawasaki/UTK-4/2009 (H1N1; UTK4) and A/Kawasaki/UTK-20/2008 (H3N2; UTK20), whereas other H5N1 strains, such as A/Hong Kong/483/97 (clade 0; HK483/97), A/chicken/Miyazaki/K11/2007 (clade 2.2; CkMiyazaki/07) and A/Vietnam/UT31203A/2007 (clade 2.3.4; VN31203/07), as well as the pdm H1N1 virus, A/California/04/2009 (CA04), replicated less efficiently than did the seasonal viruses. Thus, although all of the viruses tested could infect human macrophages, levels of replication differed even among the H5N1 viruses.
We then measured 48 different cytokines from the culture supernatants of primary human macrophages upon virus infection at an m.o.i. of 2 by using Bio-Plex human 27-plex and 21-plex panels (Bio-Rad). To aid in the visualization of the cytokine arrays, we generated a heat-map by analysing the means of data individually obtained with macrophages from the different individuals (Fig. 2). The individual macrophage data are shown in Supplementary Fig. S1, available in JGV Online.
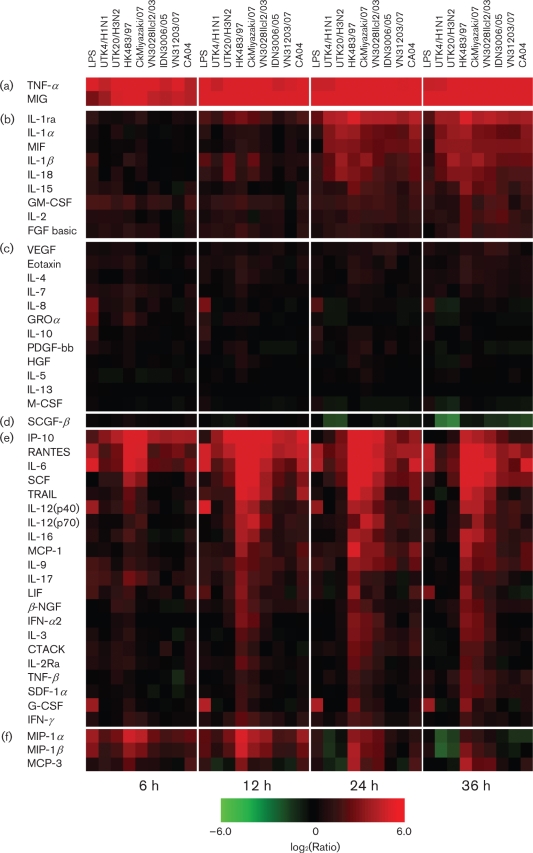
Cytokine production by human macrophages infected with influenza virus. Heat-map shows relative cytokine production on infection with seasonal viruses, H5N1 viruses and pdm H1N1 virus at different time points. Cytokine production levels at 6, 12, 24 and 36 h p.i. were normalized to mock-infection values and are shown on a log2 scale. Data presented are means of five independent experiments with macrophages from five individuals; 2−6 to 26 ranges were visualized by using Spotfire software (tibco). Cytokines are divided into six groups (a–f) according to their expression patterns.
We observed marked upregulation of two cytokines, tumour necrosis factor (TNF)-α and monokine-induced by interferon (IFN)-γ (MIG), throughout the time-course of the experiment (6–36 h p.i.) with all influenza viruses tested (Fig. 2a). Similarly, the cytokines shown in Fig. 2(b) were upregulated to various degrees upon infection with most of the test strains at the late phase (24 and 36 h p.i.). Of these, the anti-inflammatory cytokine interleukin (IL)-1 receptor antagonist (ra) was produced at the highest level. Upregulation of the IL-1 family (IL-1α, IL-1β and IL-18) and of macrophage migration inhibit factor (MIF) was also notable. By contrast, changes in the levels of the cytokines shown in Fig. 2(c) were limited. Interestingly, stem-cell growth factor (SCGF)-β was strongly downregulated by many of the influenza viruses tested at 36 h p.i., especially the seasonal viruses (Fig. 2d).
Several of the cytokines shown in Fig. 2(e) were upregulated upon infection with most of the H5N1 strains tested compared with the seasonal influenza viruses (UTK4/H1N1 and UTK20/H3N2), although the levels of expression varied among the cytokines (Fig. 2e); three proinflammatory cytokines, including chemokines [IFN-γ-inducible protein (IP)-10, regulated upon activation normal T-cell expressed and secreted (RANTES) and IL-6] and stem-cell factor (SCF), were upregulated strongly by infection with all of the viruses, but more so with the H5N1 strains, especially during the late phase of infection. However, the remaining 17 cytokines shown in Fig. 2(e), for example, IL-12, IL-16 and IL-9, which activate cell-mediated immunity, were induced markedly by some H5N1 virus strains, such as HK483/97 (clade 0) and CkMiyazaki/07 (clade 2.2), induced somewhat by H5N1 strain VN3028IIcl2/03 (clade 1) and upregulated only slightly or not by other H5N1 strains, IDN3006/05 (clade 2.1.3) and VN31203/07 (clade 2.3.4), or by seasonal viruses. Therefore, the former H5N1 viruses can be defined as high-cytokine inducers and the latter as low-cytokine inducers. Notably, the chemokines macrophage inflammatory protein (MIP)-1α and -1β (Fig. 2f) were also upregulated by all of the viruses, but more so by the high-inducer H5N1 strains, whereas these chemokines were downregulated by seasonal viruses during the late phase of infection. Another chemokine, monocyte chemotactic protein (MCP)-3, was upregulated by the high-inducers, but not by the seasonal strains.
The 2009 pandemic H1N1 virus, CA04, induced several cytokines at higher levels than those induced by the seasonal viruses; in particular, the expression of IL-6, TRAIL, MCP-1, IL-9 and MCP-3 was enhanced. Interestingly, these levels of expression were even higher than those induced by low-cytokine-inducing H5N1 viruses (Fig. 2e, f). However, since we did not confirm that this cytokine production profile is common to all pandemic H1N1 viruses, further studies may be needed to establish whether this profile is a universal trait.
In this study, we examined the cytokine profiles of influenza viruses by using the means of data obtained independently with macrophage preparations from five different individuals (Supplementary Fig. S1). This approach was essential to obtain general cytokine responses upon virus infection, since the levels of cytokine production upon influenza virus infection, especially H5N1 virus infection, varied among the donors (although the overall trend was similar). For example, IL-6 was induced strongly in two donors, but weakly in another, even for the cytokine high-inducer H5N1 strains. Similarly, the induction levels of other cytokines, such as IL-1ra and MIP-1α, with cytokine high-inducer H5N1 strains varied among blood donors. Induction levels of TNF-α also varied among the donors upon seasonal UTK20/H3N2 virus infection. These variations may contribute to the different clinical progression and disease outcomes observed in patients infected with seasonal, pdm H1N1 or H5N1 viruses.
Since the clinical presentation of patients infected with an H5N1 virus resembles that of septic shock, with a cytokine storm caused by the lipopolysaccharide (LPS) of Gram-negative bacteria (Bozza et al., 2007; Kellum et al., 2007), we also examined cytokine production by human macrophages upon LPS treatment, a well-known stimulus for cytokine production by these cells. We found that the range and kinetics of cytokine production upon LPS treatment (10 ng for 60 min at 37 °C) were substantially different from those seen on influenza virus infection. LPS-treated human macrophages produced several proinflammatory cytokines at high levels (Fig. 2), as was observed with H5N1 virus infection. However, IL-8 and GROα were induced specifically by LPS, which explains the neutrophil activation in septic shock. A broader range of cytokines was upregulated in H5N1 virus infection than upon LPS treatment, indicating that H5N1 viruses may activate the host immune system in a more complicated manner than occurs during septic shock.
The cytokine production profiles with the influenza viruses obtained here (Fig. 2) did not correlate with the viral replication levels in human macrophages (Fig. 1b). These data suggest that cytokine upregulation upon H5N1 virus infection may be regulated by factors other than virus replicative ability in these cells. To address this issue, we measured cytokine induction in macrophages incubated with VN3028IIcl2/03 virus that was inactivated by 0.1% (final) β-propiolactone (Logrippo & Hartman, 1955; Smith & Srb, 1951) for 16 h at 4 °C. We confirmed that cells treated with β-propiolactone alone, at the concentration in the inactivated virus preparation, did not affect the cytokine response (not shown). We found that almost all cytokines were produced at the same or only slightly higher levels than those of the mock infection (Fig. 3), indicating that virus replication is required for cytokine induction in human macrophages.
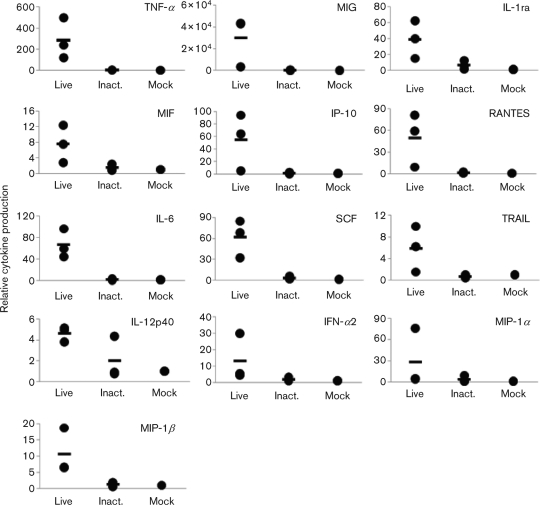
Cytokine induction profiles of inactivated virus. VN3028IIcl2/03 (Live) and its β-propiolactone-inactivated counterpart (Inact.) were respectively inoculated at an m.o.i. of 2 or the equivalent dose. At 24 h p.i., supernatants were collected and cytokine levels were measured. Mock-infected controls are also shown. Each data point is shown (filled circles), and the mean cytokine response is presented (bar).
Here, we found that many cytokines were produced at high levels upon infection with H5N1 strains (although the levels of expression varied among strains) in comparison with infection with seasonal influenza viruses, confirming, at least in part, the hypercytokinaemia that is often observed in patients infected with H5N1 viruses. The patients from whom HK483/97, VN3028IIcl2/03 and IDN3006/05 were isolated died, whereas the patient infected with VN31203/07 recovered (Le et al., 2010). Notably, the IDN3006/05 strain that killed its victim was a cytokine-low inducer. However, since the cytokine levels of the patient infected with this virus were not available, we cannot speculate as to whether the patient’s death involved cytokine-independent pathogenesis. Nonetheless, we would argue that clinical outcomes of H5N1 virus infection in humans may be affected not only by hypercytokinaemia, but also by other, as-yet unknown factors. We also found that a pandemic H1N1 2009 virus induced higher levels of several cytokines than those induced by seasonal viruses and even some H5N1 strains. These results suggest that, although hypercytokinaemia may play a part in the severe disease progression and ultimate death of patients with H5N1 virus infection, induction of high levels of cytokines may not be a feature common to all H5N1 viruses.
Acknowledgements
We thank Susan Watson for editing the manuscript. This work was supported by a Grant-in-Aid for Specially Promoted Research, by a contract research fund for the Program of Founding Research Centers for Emerging and Reemerging Infectious Diseases from the Ministry of Education, Culture, Sports, Science, and Technology (Japan), by grants-in-aid from the Ministry of Health (Japan), by ERATO (Japan Science and Technology Agency), by research grants from the National Institute of Allergy and Infectious Diseases Public Health Service and by an NIAID-funded Center for Research on Influenza Pathogenesis (CRIP, HHSN266200700010C). S.S. is supported by JSPS Research Fellowships for Young Scientists.
Footnotes
†Present address: Department of Veterinary Microbiology, Graduate School of Agriculture and Life Sciences, University of Tokyo, 1-1-1 Yayoi, Bunkyo-ku, Tokyo 113-8657, Japan.
A supplementary figure is available with the online version of this paper.
References
- Abdel-Ghafar A. N., Chotpitayasunondh T., Gao Z., Hayden F. G., Nguyen D. H., de Jong M. D., Naghdaliyev A., Peiris J. S., Shindo N., et al. (2008). Writing Committee of the Second World Health Organization Consultation on Clinical Aspects of Human Infection with Avian Influenza A (H5N1) Virus. Update on avian influenza A (H5N1) virus infection in humans. N Engl J Med 358, 261–273 10.1056/NEJMra0707279. [Abstract] [CrossRef] [Google Scholar]
- Bozza F. A., Salluh J. I., Japiassu A. M., Soares M., Assis E. F., Gomes R. N., Bozza M. T., Castro-Faria-Neto H. C., Bozza P. T. (2007). Cytokine profiles as markers of disease severity in sepsis: a multiplex analysis. Crit Care 11, R49 10.1186/cc5783. [Europe PMC free article] [Abstract] [CrossRef] [Google Scholar]
- Cheung C. Y., Poon L. L., Lau A. S., Luk W., Lau Y. L., Shortridge K. F., Gordon S., Guan Y., Peiris J. S. (2002). Induction of proinflammatory cytokines in human macrophages by influenza A (H5N1) viruses: a mechanism for the unusual severity of human disease? Lancet 360, 1831–1837 10.1016/S0140-6736(02)11772-7. [Abstract] [CrossRef] [Google Scholar]
- Claas E. C., Osterhaus A. D., van Beek R., De Jong J. C., Rimmelzwaan G. F., Senne D. A., Krauss S., Shortridge K. F., Webster R. G. (1998). Human influenza A H5N1 virus related to a highly pathogenic avian influenza virus. Lancet 351, 472–477 10.1016/S0140-6736(97)11212-0. [Abstract] [CrossRef] [Google Scholar]
- Dawood F. S., Jain S., Finelli L., Shaw M. W., Lindstrom S., Garten R. J., Gubareva L. V., Xu X., Bridges C. B., et al. (2009). Novel swine-origin influenza A (H1N1) virus investigation team. Emergence of a novel swine-origin influenza A (H1N1) virus in humans. N Engl J Med 360, 2605–2615 10.1056/NEJMoa0903810. [Abstract] [CrossRef] [Google Scholar]
- de Jong M. D., Bach V. C., Phan T. Q., Vo M. H., Tran T. T., Nguyen B. H., Beld M., Le T. P., Truong H. K., et al. (2005). Fatal avian influenza A (H5N1) in a child presenting with diarrhea followed by coma. N Engl J Med 352, 686–691 10.1056/NEJMoa044307. [Abstract] [CrossRef] [Google Scholar]
- de Jong M. D., Simmons C. P., Thanh T. T., Hien V. M., Smith G. J., Chau T. N., Hoang D. M., Chau N. V., Khanh T. H., et al. (2006). Fatal outcome of human influenza A (H5N1) is associated with high viral load and hypercytokinemia. Nat Med 12, 1203–1207 10.1038/nm1477. [Europe PMC free article] [Abstract] [CrossRef] [Google Scholar]
- Guan Y., Poon L. L., Cheung C. Y., Ellis T. M., Lim W., Lipatov A. S., Chan K. H., Sturm-Ramirez K. M., Cheung C. L., et al. (2004). H5N1 influenza: a protean pandemic threat. Proc Natl Acad Sci U S A 101, 8156–8161 10.1073/pnas.0402443101. [Europe PMC free article] [Abstract] [CrossRef] [Google Scholar]
- Kellum J. A., Kong L., Fink M. P., Weissfeld L. A., Yealy D. M., Pinsky M. R., Fine J., Krichevsky A., Delude R. L., Angus D. C., GenIMS Investigators (2007). Understanding the inflammatory cytokine response in pneumonia and sepsis: results of the Genetic and Inflammatory Markers of Sepsis (GenIMS) Study. Arch Intern Med 167, 1655–1663 10.1001/archinte.167.15.1655. [Europe PMC free article] [Abstract] [CrossRef] [Google Scholar]
- Khan K., Arino J., Hu W., Raposo P., Sears J., Calderon F., Heidebrecht C., Macdonald M., Liauw J., et al. (2009). Spread of a novel influenza A (H1N1) virus via global airline transportation. N Engl J Med 361, 212–214 10.1056/NEJMc0904559. [Abstract] [CrossRef] [Google Scholar]
- Le Q. M., Ito M., Muramoto Y., Hoang P. V., Vuong C. D., Sakai-Tagawa Y., Kiso M., Ozawa M., Takano R., Kawaoka Y. (2010). Pathogenicity of highly pathogenic avian H5N1 influenza A viruses isolated from humans between 2003 and 2008 in northern Vietnam. J Gen Virol 91, 2485–2490 10.1099/vir.0.021659-0. [Europe PMC free article] [Abstract] [CrossRef] [Google Scholar]
- Lee S. M., Gardy J. L., Cheung C. Y., Cheung T. K., Hui K. P., Ip N. Y., Guan Y., Hancock R. E., Peiris J. S. (2009). Systems-level comparison of host-responses elicited by avian H5N1 and seasonal H1N1 influenza viruses in primary human macrophages. PLoS ONE 4, e8072 10.1371/journal.pone.0008072. [Europe PMC free article] [Abstract] [CrossRef] [Google Scholar]
- Logrippo G. A., Hartman F. W. (1955). Antigenicity of beta-propiolactone-inactivated virus vaccines. J Immunol 75, 123–128 [Abstract] [Google Scholar]
- Louie J. K., Acosta M., Winter K., Jean C., Gavali S., Schechter R., Vugia D., Harriman K., Matyas B., et al. (2009). Factors associated with death or hospitalization due to pandemic 2009 influenza A(H1N1) infection in California. JAMA 302, 1896–1902 10.1001/jama.2009.1583. [Abstract] [CrossRef] [Google Scholar]
- Mok K. P., Wong C. H., Cheung C. Y., Chan M. C., Lee S. M., Nicholls J. M., Guan Y., Peiris J. S. (2009). Viral genetic determinants of H5N1 influenza viruses that contribute to cytokine dysregulation. J Infect Dis 200, 1104–1112 10.1086/605606. [Europe PMC free article] [Abstract] [CrossRef] [Google Scholar]
- Osterlund P., Pirhonen J., Ikonen N., Rönkkö E., Strengell M., Mäkelä S. M., Broman M., Hamming O. J., Hartmann R., et al. (2010). Pandemic H1N1 2009 influenza A virus induces weak cytokine responses in human macrophages and dendritic cells and is highly sensitive to the antiviral actions of interferons. J Virol 84, 1414–1422 10.1128/JVI.01619-09. [Europe PMC free article] [Abstract] [CrossRef] [Google Scholar]
- Peiris J. S., Yu W. C., Leung C. W., Cheung C. Y., Ng W. F., Nicholls J. M., Ng T. K., Chan K. H., Lai S. T., et al. (2004). Re-emergence of fatal human influenza A subtype H5N1 disease. Lancet 363, 617–619 10.1016/S0140-6736(04)15595-5. [Europe PMC free article] [Abstract] [CrossRef] [Google Scholar]
- Peng Z., Zhang Z., Xu Y. (1999). [Effect of Angelica sinensis injection on CD11c and CD14 expression in alveolar macrophage membrane of chronic bronchitis patients]. Zhongguo Zhong Xi Yi Jie He Za Zhi 19, 282–285 (in Chinese). [Abstract] [Google Scholar]
- Shimizu T., Fujimori F., Shimaoka Y., Narita J., Takada T., Tajima S., Moriyama H., Terada M., Suzuki E., Gejyo F. (2007). Isolation and immunophenotyping of mononuclear cells from human lung tissue. Intern Med 46, 163–169 10.2169/internalmedicine.46.1857. [Abstract] [CrossRef] [Google Scholar]
- Smith H. H., Srb A. M. (1951). Induction of mutations with beta-propiolactone. Science 114, 490–492 10.1126/science.114.2967.490. [Abstract] [CrossRef] [Google Scholar]
- Taylor P. R., Martinez-Pomares L., Stacey M., Lin H. H., Brown G. D., Gordon S. (2005). Macrophage receptors and immune recognition. Annu Rev Immunol 23, 901–944 10.1146/annurev.immunol.23.021704.115816. [Abstract] [CrossRef] [Google Scholar]
- To K. F., Chan P. K., Chan K. F., Lee W. K., Lam W. Y., Wong K. F., Tang N. L., Tsang D. N., Sung R. Y., et al. (2001). Pathology of fatal human infection associated with avian influenza A H5N1 virus. J Med Virol 63, 242–246 10.1002/1096-9071(200103)63:3<242::AID-JMV1007>3.0.CO;2-N. [Abstract] [CrossRef] [Google Scholar]
- Uiprasertkul M., Puthavathana P., Sangsiriwut K., Pooruk P., Srisook K., Peiris M., Nicholls J. M., Chokephaibulkit K., Vanprapar N., Auewarakul P. (2005). Influenza A H5N1 replication sites in humans. Emerg Infect Dis 11, 1036–1041 [Europe PMC free article] [Abstract] [Google Scholar]
- Woo P. C., Tung E. T., Chan K. H., Lau C. C., Lau S. K., Yuen K. Y. (2010). Cytokine profiles induced by the novel swine-origin influenza A/H1N1 virus: implications for treatment strategies. J Infect Dis 201, 346–353 10.1086/649785. [Europe PMC free article] [Abstract] [CrossRef] [Google Scholar]
Articles from The Journal of General Virology are provided here courtesy of Microbiology Society
Citations & impact
Impact metrics
Citations of article over time
Alternative metrics
Smart citations by scite.ai
Explore citation contexts and check if this article has been
supported or disputed.
https://scite.ai/reports/10.1099/vir.0.030346-0
Article citations
The role of alveolar macrophages in viral respiratory infections and their therapeutic implications.
Biochem Biophys Rep, 40:101826, 17 Sep 2024
Cited by: 0 articles | PMID: 39324036 | PMCID: PMC11422589
Review Free full text in Europe PMC
Human B cells and dendritic cells are susceptible and permissive to enterovirus D68 infection.
mSphere, 9(2):e0052623, 23 Jan 2024
Cited by: 0 articles | PMID: 38259063 | PMCID: PMC10900886
Severe influenza infection is associated with inflammatory programmed cell death in infected macrophages.
Front Cell Infect Microbiol, 13:1067285, 16 Feb 2023
Cited by: 6 articles | PMID: 36875528 | PMCID: PMC9980436
Human influenza virus infection elicits distinct patterns of monocyte and dendritic cell mobilization in blood and the nasopharynx.
Elife, 12:e77345, 08 Feb 2023
Cited by: 8 articles | PMID: 36752598 | PMCID: PMC9977282
A Glu-Glu-Tyr Sequence in the Cytoplasmic Tail of the M2 Protein Renders Influenza A Virus Susceptible to Restriction of the Hemagglutinin-M2 Association in Primary Human Macrophages.
J Virol, 96(18):e0071622, 13 Sep 2022
Cited by: 1 article | PMID: 36098511 | PMCID: PMC9517718
Go to all (47) article citations
Similar Articles
To arrive at the top five similar articles we use a word-weighted algorithm to compare words from the Title and Abstract of each citation.
Systems-level comparison of host-responses elicited by avian H5N1 and seasonal H1N1 influenza viruses in primary human macrophages.
PLoS One, 4(12):e8072, 14 Dec 2009
Cited by: 81 articles | PMID: 20011590 | PMCID: PMC2788213
Comparison of pro-inflammatory cytokine expression and cellular signal transduction in human macrophages infected with different influenza A viruses.
Med Microbiol Immunol, 200(1):53-60, 24 Sep 2010
Cited by: 40 articles | PMID: 20865277
Mammalian innate resistance to highly pathogenic avian influenza H5N1 virus infection is mediated through reduced proinflammation and infectious virus release.
J Virol, 86(17):9201-9210, 20 Jun 2012
Cited by: 24 articles | PMID: 22718824 | PMCID: PMC3416141
Pathogenesis of emerging avian influenza viruses in mammals and the host innate immune response.
Immunol Rev, 225:68-84, 01 Oct 2008
Cited by: 133 articles | PMID: 18837776
Review
Funding
Funders who supported this work.
NIAID NIH HHS (1)
Grant ID: HHSN266200700010C