Abstract
Free full text

Swatting flies: modelling wound healing and inflammation in Drosophila
Abstract
Aberrant wound healing can lead to a variety of human pathologies, from non-healing chronic wounds that can become dangerously infected, to exuberant fibrotic healing in which repair is accompanied by excessive inflammation. To guide therapeutic intervention, we need a better understanding of the fundamental mechanisms driving tissue repair; this will require complementary wound-healing studies in several model organisms. Drosophila has been used to model genetic aspects of numerous human pathologies, and is being used increasingly to gain insight into the molecular and genetic aspects of tissue repair and inflammation, which have classically been modelled in mice or cultured cells. This review discusses the advantages and disadvantages of Drosophila as a wound-healing model, as well as some exciting new research opportunities that will be enabled by its use.
Introduction
Wound healing is an essential homeostatic mechanism that maintains epithelial barrier integrity after tissue damage. All multicellular organisms can repair themselves to varying degrees but, to date, most of what we know about healing has come from a combination of in vivo experiments using mice and from tissue culture ‘scratch’ wound studies.
Immediately after wounding, a complex clotting cascade results in the formation of a protective scab. In the subsequent days to weeks, healthy tissues and cells migrate and proliferate beneath the scab to heal the wound (Gurtner et al., 2008; Martin, 1997). Epidermal cells collectively migrate across the wound bed until wound edges meet and fuse – a process known as re-epithelialisation. Beneath the migrating epidermal cells, a wound granulation tissue – consisting of migrating fibroblasts, contractile myofibroblasts and a dense capillary network – shrinks to close the wound. Tissue damage generates a large amount of cell and matrix debris, and provides a potential route for infection by invading pathogens. Such infections are dealt with by an army of recruited innate immune cells, first neutrophils (recruited within hours) and later macrophages, which clear neutrophils and release many signals that guide other cell lineages during the wound repair process.
Although we know in outline the main events involved in the healing of a wound, many of the underlying molecular mechanisms are still unclear. Understanding these molecular mechanisms is particularly important if we wish, for example, to develop strategies to intervene in or modulate the wound inflammatory response, or to enhance the migration of epidermal cells to heal chronic wounds that pose a health and economic burden.
Fruit flies provide a fantastic opportunity for studying these mechanisms because they are an ideal system in which to marry genetic techniques with live imaging of the repair process. In fact, researchers have been wounding insects for nearly a hundred years (e.g. Wigglesworth, 1937) to investigate patterning mechanisms and regenerative capacities of various tissues. However, only in the last 10 or so years have flies become recognised as a worthy model to investigate the genetics of tissue repair and the associated inflammatory response. In this Primer article, we review the progress made by a number of research groups that have begun to model the main aspects of mammalian tissue repair through studying Drosophila at various stages of development, from embryo to adult (Fig. 1). We also highlight the research opportunities in this area that can be addressed using Drosophila to guide our understanding of human wound healing (Box 1).
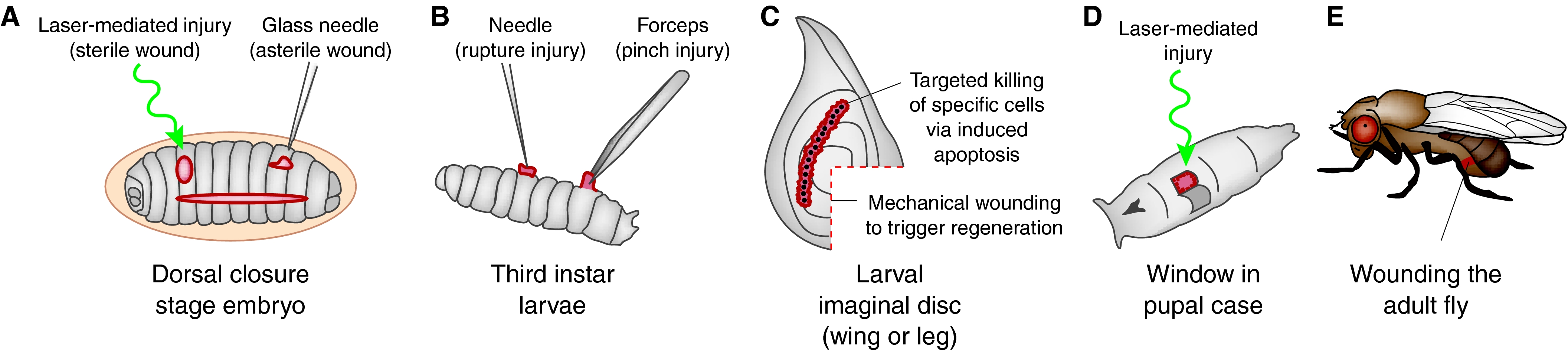
At all stages of development, from embryo to adult, Drosophila can and has been used as a wound-healing model. Wounded areas are shown in red. (A) Embryos can be wounded by hand with a glass needle, which punctures the vitelline membrane (creating asterile wounds), resulting in ragged wounds that vary in size. Laser-mediated wounding ablates a patch of epithelium, leaving a circular hole of reproducible size that gapes in the dorsoventral axis because of tensions within the epithelium. These laser-mediated wounds leave the vitelline membrane intact and are therefore sterile. Embryo wounding is generally performed at around stage 14, when dorsal closure (horizontal oval represents dorsal hole) is near completion, enabling comparisons between wound healing and morphogenesis. (B) Larval wounding (generally third instar) is mediated either with a needle, which breaks the epidermal layer and results in the formation of a melanin clot, or by ‘pinching’ with forceps, which damages the epidermis, but does not breach the barrier cuticle. (C) Within third instar larvae are imaginal discs, which have long been used as a model of pattern regeneration. Wounding was historically mediated by mechanical cutting (broken red line) and required grafting back into an adult host, but new studies use Gal4-UAS-targeted apoptotic killing of defined populations of cells in the wing and/or leg discs. (D) At pupal stages, fly tissues are translucent and are amenable to wounding and dynamic imaging, but only after a window has been cut in the pupal case. (E) Finally, adult flies can be wounded using a tungsten needle or with iridectomy scissors, by cutting between the tergites of the abdomen.
Actin machineries that drive wound re-epithelialisation
Studies of wound re-epithelialisation in Drosophila embryos have enabled live imaging of the actin machineries that drive wound closure. These machineries include a contractile actomyosin cable that closes the epithelial hole like a purse string, and dynamic filopodia (finger-like actin-rich membrane protrusions) that mainly function to knit the confronting epithelial edges together to finally seal the wound closed (Wood et al., 2002). The function of the actomyosin cable is dependent on a Rho GTPase, Rho1. rho1 mutant Drosophila embryos cannot assemble a wound actomyosin cable but compensate with extensive filopodial protrusions that ‘zipper’ neighbouring cells together and drag the wound closed (Wood et al., 2002).
We know that filopodia are required to knit together the sealing wound fronts because expression of a dominant-negative mutant version of the small Rho GTPase Cdc42 in the embryonic epidermis leads to inhibition of filopodia formation and a failure of the wound edges to seal (Wood et al., 2002). Several morphogenetic epithelial fusion episodes in fly (and also mammalian) development are dependent on similar filopodial ‘zippering’ processes (Li et al., 2003; Wood et al., 2002; Zenz et al., 2003). The epidermis of the Drosophila embryo is made up of striped domains that later define the pattern of the adult fly. By differentially labelling the cells in these domains with fluorescent proteins, it has been shown that filopodia, as well as providing the mechanism for knitting epithelial edges together, can also ‘sense’ matching opposing cells and sort these epithelial domains. Filopodia can even drag whole epithelial sheets into alignment during the developmental morphogenetic movement, dorsal closure and also during wound healing (Jacinto et al., 2002; Millard and Martin, 2008).
Despite the clear requirement for filopodia, most of the signalling mechanisms that mediate the sensing and final fusion of epithelial sheets during morphogenetic and wound healing episodes are still unclear. We do not yet know which Rho guanine nucleotide exchange factors (GEFs) are involved in wound-mediated activation of Rho1 and Cdc42, nor do we know which GTPase-activating proteins (GAPs) shut them off when wound healing is complete. In addition, primary activating cues following wounding probably involve growth factors and/or mechanical signals that have yet to be defined. In the embryo, the tyrosine kinase Stitcher has been implicated in actomyosin cable assembly (Wang et al., 2009) and, in larvae, it has been suggested that leakage and exposure of haemolymph components – in particular, of the growth factor PDGF- and VEGF-related factor (Pvf) – to wound epithelia can activate growth factor receptors and drive directed actin-based cell migration (Wu et al., 2009). These findings highlight the similarity between Drosophila and mammalian epidermal wound healing, in that both are regulated by receptor tyrosine kinases. We hope that studies with flies will uncover the components of this signalling machinery that are key for epithelial sealing and how they might be modulated in a therapeutic fashion.
The purse-string closure mechanism does not seem to operate after Drosophila embryonic stages, and re-epithelialisation mechanisms in larvae are more reminiscent of adult mammalian skin healing. Injury during this later stage of development results in the formation of a simple clot, and lamellae (thin, sheet-like membrane protrusions driven by actin treadmilling at the leading edge) drive Rho-GTPase- and myosin-II-dependent ‘crawling’ of front row cells to re-epithelialise the wound (Baek et al., 2010; Galko and Krasnow, 2004; Kwon et al., 2010). Curiously, this movement is coupled to syncytial fusion of neighbouring cells as the epithelium advances, in a process that does not seem to be mirrored in mammalian healing.
Why there is such a dramatic change in the mode of re-epithelialisation from purse-string healing (in Drosophila and vertebrate embryos) to lamellipodial migration (in Drosophila larvae and from the late stage vertebrate foetus into postnatal life) is not clear. Presumably, the genetic or mechanical changes (or both) that occur in these tissues during development must alter the balance of activation of the key Rho GTPases and favour the assembly of one actin-based machinery over another. Notably, small wounds are still closed by actomyosin purse strings in some mammalian adult tissues comprising simple epithelia, including the gut and cornea (Danjo and Gipson, 1998; Heath, 1996). It will be important to discover how these actin-based machineries control epidermal cell migration, especially the identity of the proteins involved, and to what extent these mechanisms are conserved from fly to mammalian wound healing.
Transcriptional signalling episodes at the wound edge
In addition to the activation of various small GTPases, at least two further signalling pathways have been shown to be activated after wounding fly embryos, larvae or adults and to go on to drive the expression of the wound transcriptome. These signalling pathways seem to be conserved and important during mammalian repair also.
Role of JNK signalling
JNK activity, which is required for mammalian wound healing (Martin and Nobes, 1992; Zenz et al., 2003), can be reported by the expression of Puckered in Drosophila. In both larval (Galko and Krasnow, 2004) and adult (Ramet et al., 2002) epithelial wound edges, Puckered expression is seen from as early as 4 hours after wounding. Wounding of larval imaginal discs also induces JNK activity, extending back several cell diameters from the wound edge (Bosch et al., 2005). The presence of JNK activity corresponds to the cells that will participate in the wound healing response and is thought to prepare the cells for entry into a regenerative response (Bosch et al., 2005). During all stages of Drosophila development thus far examined, impaired healing has been reported to result from expression of a dominant-negative form of Basket (Drosophila homologue of JNK) (Galko and Krasnow, 2004), expression of various interfering RNAs targeted against proteins in the JNK pathway (Lesch et al., 2010) or loss-of-function mutations in Kayak (Drosophila homologue of Fos) (Ramet et al., 2002).
In mammals, the formation of a fibrin clot at the wound site provides a source of stimulating cues for many cell types. This might also be the case in flies, although the coagulation cascade differs in invertebrates compared with vertebrates. There is no scab formed after wounding in the Drosophila embryo, but a plug composed of haemolymph and cell debris forms very rapidly after puncture wounding of later-stage Drosophila larvae (Karlsson et al., 2004); this plug subsequently becomes a melanised scab. Deletion of crystal cells (a haemocyte sub-lineage) impairs scab formation, resulting in retarded wound healing and a compensatory hyperinduction of the JNK response (Galko and Krasnow, 2004). How this might relate to mammalian wound healing is still unclear.
The role of Grainy head signalling
Grainy head signalling is required for both the establishment and healing of the barrier epidermal and cuticle layers of Drosophila larvae (Mace et al., 2005) and mammalian skin (Ting et al., 2005), providing a striking example of co-evolution. Recent studies have shown that several genes upregulated during wound healing are downstream of Grainy head signalling; some, but not all, of these are also JNK-AP1 target genes, suggesting that multiple signalling pathways that are induced during wound healing can activate the various components of the wound transcriptome (Mace et al., 2005; Pearson et al., 2009). Furthermore, one key target of Grainy head signalling, Stitcher, activates ERK (Wang et al., 2009), which in turn directly regulates Grainy head signalling through phosphorylation and enables expression of other Grainy head target genes in epidermal cells following wounding (Kim and McGinnis, 2011). Although Grainy head signalling is active at low levels under physiological conditions to maintain epidermal integrity in both mammals [grainyhead-like 3 (Grhl3)] and Drosophila, these studies suggest that its activity is upregulated to drive the wound healing response.
Overall, the close parallels already identified between fly and mammalian wound healing suggest that further studies in Drosophila will enable a fast-track understanding of fundamental wound-healing-associated signalling pathways and their regulation.
Other signalling pathways involved in the regeneration of larval imaginal discs
In addition to JNK and Grainy head signalling, several other pathways are now emerging as potential players in wound healing owing to their role in larval imaginal disc regeneration. For example, the bone morphogenetic protein (BMP; Decapentaplegic in Drosophila) pathway (Mattila et al., 2004), Hippo pathway (Grusche et al., 2011), Wnt (Wingless in Drosophila) pathway (Smith-Bolton et al., 2009) and retinoid signalling (Halme et al., 2010) are all active in regenerating tissue after mechanical wounding or in settings in which specific cell types have been genetically ablated through the expression of pro-apoptotic genes in specific regions of the disc. Although these pathways have not yet been shown to be essential for repair in fly models, it is clear that they play a role in growth and differentiation of regenerating tissue. In addition, several of them have been linked to vertebrate regeneration and/or repair (Halme et al., 2010; Masterson et al., 2011).
Role of cell proliferation in wound re-epithelialisation
Studies of mammalian skin healing have provided clear evidence that cell division occurs in the advancing wound epithelium (Werner et al., 1994). If a significant amount of tissue is lost through damage, it seems logical that new cells should be generated to replace it; however, studies have shown that cell division, per se, is not essential for healing. Indeed, in the fly embryo, small holes can heal rapidly at a stage of development when there is no cell division within the epithelium: rather, the gap is filled by cells changing shape and stretching in order to increase their surface area (Wood et al., 2002). Of course, this means that embryos are a poor model for investigating the regulation and role of cell division in wound re-epithelialisation. However, when larval imaginal discs are wounded, there is a surge of cell proliferation at the wound margin (Dale and Bownes, 1981), and this might play a key role in the regenerative process. Further studies of wounded imaginal discs might therefore shed light on the genetics that underlie this key aspect of the vertebrate wound response.
Recruitment of innate immune cells to wounds: studying the inflammatory response
Tissue damage triggers a robust inflammatory response to both fight infection and clear wound debris. Unfortunately, in performing these vital tasks, immune cells also induce fibrosis and scarring (for a review, see Stramer et al., 2007). Drosophila does not scar, as mammals do, because flies have a different connective tissue arrangement. However, Drosophila does have an innate immune cell lineage, known as the haemocyte, which is very amenable to live imaging and responds to tissue damage cues. Studying haemocytes in Drosophila models of wound healing can therefore be used to model some aspects of the immune cell responses that occur in mammalian wound healing.
Haemocytes are born at the anterior pole of the Drosophila embryo and become dispersed throughout the embryo by following developmental cues, including the growth factors Pvf2 and Pvf3 (for a review, see Wood and Jacinto, 2007). Only after this developmental dispersal can haemocytes respond to tissue damage attractant(s), including hydrogen peroxide, from the wound epidermis (Moreira et al., 2010). After their recruitment to the wound, haemocytes gorge themselves on damaged epithelial cells and bacteria, if present. The migration of haemocytes to wounds depends on phosphatidylinositol 3-kinase (PI3K) (Wood et al., 2006). This migration also requires, to various degrees, the small GTPase actin regulators Rho, Rac and Cdc42 (Stramer et al., 2005), Ena [a Vasp family member that regulates membrane protrusions (Tucker et al., 2011)], and orbit/clasp-mediated assembly of polarised microtubules (Fig. 2) (Stramer et al., 2010).
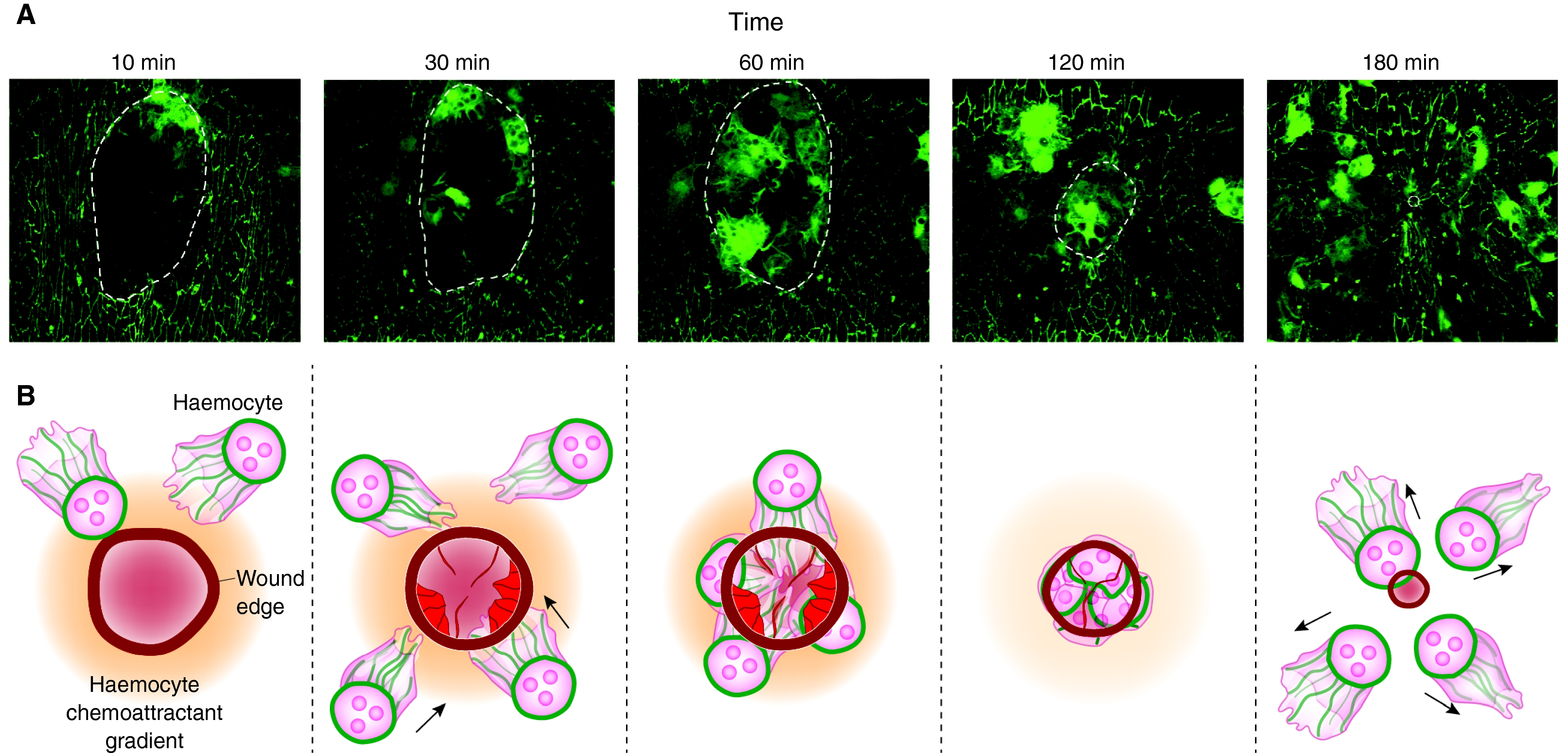
Drosophila embryonic macrophages (haemocytes) migrate to wounds in the epithelium. (A) Time course series revealing haemocyte recruitment to the wound within 10 minutes and continuing for 90 minutes before this inflammatory response begins to resolve. Wound recruitment only occurs from stage 15 of fly embryonic development; before this stage, haemocytes seem to integrate wound signals and developmental dispersal cues in ways that suggest molecular mechanisms that enable haemocytes to ‘prioritise’ various attractant cues over others. The closing wound edge is highlighted by the dotted line. Adapted from Stramer et al. (Stramer et al., 2005) with permission. (B) Schematic illustrating the time course of events imaged in A. Epithelial cells at the wound edge rapidly assemble an actomyosin cable and dynamic lamellae and filopodia (red webbed and finger-like structures). Wounding also induces the production of hydrogen peroxide (represented by orange gradient), which acts as a robust chemoattractant for haemocytes in embryos. Haemocytes become polarised and oriented towards the wound via bundled microtubules (green) and initially accumulate at the wound edge before filling the wound space. As the wound closes and the chemoattractant gradient fades, haemocytes return to their developmental positions.
In mammals, immune cells can exit from blood vessels into tissues in a process known as diapedesis; many aspects of the subsequent extravascular migration can be modelled in the Drosophila embryo studies described above (Moreira et al., 2010; Stramer et al., 2005; Tucker et al., 2011 Stramer et al., 2010). In addition, a recent study of haemocyte dispersal in the embryo during the early stages of development might provide another system for modelling mammalian immune cell migration, because haemocytes seem to penetrate the extended germ band using the same machinery as that used by mammalian blood cells to cross epithelial barriers (Siekhaus et al., 2010). By contrast, the wound immune response in Drosophila larvae is more reminiscent of mammalian pre-diapedesis events, when immune cells circulating in blood vessels become activated in response to local wound cues before passing through the vessel wall into the extravascular space. Although Drosophila has no blood vessels, activated haemocytes in larvae adhere to the damaged wound surfaces as they flow by in a manner that seems to resemble mammalian immune cell binding to activated blood vessel walls (Babcock et al., 2008).
What flies are best at: screening for new genes
Because of its short life cycle, relatively small genome and the availability of a large number of genetic tools, Drosophila is an ideal model in which to perform large-scale in vivo genetic screens that have the potential to reveal unidentified wound-healing genes and signalling pathways involved in tissue repair. Microarray comparisons and genetic screens have been undertaken by various laboratories to identify genes that drive wound healing and/or the wound inflammatory response in the embryo and in larvae (Stramer et al., 2008; Campos et al., 2010; Lesch et al., 2010). These screens are recent, and so the subsequent follow-up work has been limited, but already they have shown us that several aspects of the mammalian wound response are conserved in Drosophila. There is promise that many unidentified wound-healing genes will be uncovered by these screens that will guide further work for researchers interested in the more complex processes of mammalian tissue repair. Already, GADD45 (growth arrest and DNA damage-inducible gene 45) (Stramer et al., 2008), polycomb proteins (Campos et al., 2010) and many actin regulators (Lesch et al., 2010) have been identified as common to both mammalian and fly healing.
New opportunities for studies of wound healing in Drosophila
The field is ripe for investigation in several different directions, each with the potential to open up new therapeutic avenues. Now that the initial characterisation of the repair and inflammatory responses is done and the first broad screens completed, we can look forward to more advances as new imaging and genetic technologies are developed for Drosophila.
Live imaging of the signals and mechanical forces at play during wound repair
Increasingly, molecular probes will be used in Drosophila to provide readouts of signalling pathways (e.g. Ca2+ signalling) in vivo in real time as tissue repair proceeds. In situations in which translucency and immobility enable live imaging – in particular in the embryo and pupae – it will be possible to image events such as the translocation of fluorescently tagged transcription factors from cytoplasm to nucleus. It will also be possible to image actin remodelling with newer reporters of the actin cytoskeleton such as Lifeact (Riedl et al., 2008), and to carry out in situ observation of signalling pathways using fluorescence resonance energy transfer (FRET) (see Kamiyama and Chiba, 2009).
In addition to chemical signals that direct aspects of the wound response, mechanical signals are generated by the wound as the epidermis gapes open, and these could also impact on healing. Several recent studies have revealed that cells respond to ‘tugging’ forces during embryonic morphogenetic episodes. For example, the transcription factor Twist is upregulated in the Drosophila embryo in response to mechanical stress during gut morphogenesis (Farge, 2003), and translocation of fluorescently tagged Myocardin-related transcription factor (MAL-D) into the nucleus has been observed during migration of ‘follower’ border cells as they are dragged by ‘leader’ cells (Somogyi and Rorth, 2004). Such molecular ‘reporters’ that provide a readout of response to forces might enable us to image the early mechanical changes that occur after an epithelium is wounded, as the cells change their shape and shuffle their neighbour-neighbour relations to close the wound hole, in a manner similar to studies that have illustrated how force impacts on morphogenetic events such as dorsal closure (Hutson et al., 2003; Kiehart et al., 2000).
Investigating epigenetic regulation of the wound transcriptome
Epigenetic changes influence a multitude of cellular changes that might aid in tissue repair, including cell migration and cell fate decisions. The Drosophila larval imaginal disc has long been used as a model for regeneration, and studies of wounded imaginal discs have highlighted the importance of epigenetics in regulating regeneration in this context. Specifically, it seems that, during regeneration, the polycomb-mediated silencing machinery is shut down in cells at the leading edge of a regenerating blastema, which results in the transdifferentiation of some cells from leg to wing fate (Lee et al., 2005). Although it is not clear exactly how these epigenetic changes impact on the repair process, this study prompted further work that revealed similar observations during mammalian (Shaw and Martin, 2009) and fish (Stewart et al., 2009) healing. In these cases, polycomb repressive complex 2 (PRC2) proteins are downregulated in cells at the wound edge, whereas the counteracting histone demethylase enzymes UTX and Jmjd3 are upregulated. Thus, the fly embryo might provide good opportunities to test polycomb-mediated regulation of wound repair. Interestingly, hints from Drosophila embryonic screens suggest that polycomb, UTX and trithorax genes are all needed for wound healing (Campos et al., 2010).
Investigating how infection impacts on tissue repair
A great deal is known about how Drosophila combats the threat of invading microbes while possessing only an innate, and not an adaptive, immune response (for a review, see Lemaitre and Hoffmann, 2007). Notably, a key innate immune receptor, Toll, was discovered in the fly, and it has since been shown that there are close parallels between Drosophila Toll-mediated signalling and vertebrate Toll-like receptor (TLR)-mediated signalling following infection (for a review, see Valanne et al., 2011). To date, however, most studies of wound repair have not taken into account the presence of microbes; indeed, in laser wounding of embryos and pinching of larval epidermis, the outer barrier layer (the vitelline membrane in embryos and the cuticle in larvae) is not breached, negating the possibility of infection at the site of injury. Despite this sterile environment, several antimicrobial peptides are upregulated in these models (Stramer et al., 2008), suggesting that endogenous danger signals produced on wounding can activate innate immune responses in the absence of infection. It will be a simple step to compare infected wounds with what is known about the genetics of sterile tissue repair (as described above) so that Drosophila can become a driving force in investigations of how infection impacts on repair processes. The opportunity to carry out live imaging of immune cell engulfment and killing of microbes (Vlisidou et al., 2009) in real time in the in vivo healing wound will provide immediate rewards in pursuing this approach.
Conclusion
It is still early days for Drosophila wound-healing studies, but already it is clear that the genetic tractability of flies, combined with the various imaging opportunities available, means that it will become a more and more powerful contributor to our understanding of fundamental mechanisms that underpin wound repair. Drosophila screens will undoubtedly reveal unidentified genes and signalling pathways that are involved in repair and inflammation that can be further tested in mammals; indeed, the results of fly studies are already influencing how we approach questions about wound healing in vertebrates. Despite the strengths of Drosophila-based approaches, however, it is crucial that investigators are sensible about what aspects of mammalian healing flies can reasonably be used to study, and what aspects it cannot model (see Box 1). With this proviso, flies now look to be an important model for studying repair and inflammation, beautifully complementing research carried out in other species, including zebrafish, mice and humans.
Footnotes
COMPETING INTERESTS
The authors declare that they do not have any competing or financial interests.
REFERENCES
- Babcock D. T., Brock A. R., Fish G. S., Wang Y., Perrin L., Krasnow M. A., Galko M. J. (2008). Circulating blood cells function as a surveillance system for damaged tissue in Drosophila larvae. Proc. Natl. Acad. Sci. USA 105, 10017–10022 [Europe PMC free article] [Abstract] [Google Scholar]
- Baek S. H., Kwon Y. C., Lee H., Choe K. M. (2010). Rho-family small GTPases are required for cell polarization and directional sensing in Drosophila wound healing. Biochem. Biophys. Res. Commun. 394, 488–492 [Abstract] [Google Scholar]
- Bosch M., Serras F., Martin-Blanco E., Baguna J. (2005). JNK signaling pathway required for wound healing in regenerating Drosophila wing imaginal discs. Dev. Biol. 280, 73–86 [Abstract] [Google Scholar]
- Campos I., Geiger J. A., Santos A. C., Carlos V., Jacinto A. (2010). Genetic screen in Drosophila melanogaster uncovers a novel set of genes required for embryonic epithelial repair. Genetics 184, 129–140 [Europe PMC free article] [Abstract] [Google Scholar]
- Dale L., Bownes M. (1981). Wound healing and regeneration in the imaginal wing disc of Drosophila. Roux’s Arch. 190, 91–96 [Abstract] [Google Scholar]
- Danjo Y., Gipson I. K. (1998). Actin ‘purse string’ filaments are anchored by E-cadherin-mediated adherens junctions at the leading edge of the epithelial wound, providing coordinated cell movement. J. Cell Sci. 111, 3323–3332 [Abstract] [Google Scholar]
- Farge E. (2003). Mechanical induction of twist in the Drosophila foregut/stomodeal primordium. Curr. Biol. 13, 1365–1377 [Abstract] [Google Scholar]
- Galko M. J., Krasnow M. A. (2004). Cellular and genetic analysis of wound healing in Drosophila larvae. PloS Biol. 2, 1114–1126 [Europe PMC free article] [Abstract] [Google Scholar]
- Grusche F. A., Degoutin J. L., Richardson H. E., Harvey K. F. (2011). The Salvador/Warts/Hippo pathway controls regenerative tissue growth in Drosophila melanogaster. Dev. Biol. 350, 255–266 [Abstract] [Google Scholar]
- Gurtner G. C., Werner S., Barrandon Y., Longaker M. T. (2008). Wound repair and regeneration. Nature 453, 314–321 [Abstract] [Google Scholar]
- Halme A., Cheng M., Hariharan I. K. (2010). Retinoids regulate a developmental checkpoint for tissue regeneration in Drosophila. Curr. Biol. 20, 458–463 [Europe PMC free article] [Abstract] [Google Scholar]
- Heath J. P. (1996). Epithelial cell migration in the intestine. Cell Biol. Int. 20, 139–146 [Abstract] [Google Scholar]
- Hutson M. S., Tokutake Y., Chang M. S., Bloor J. W., Venakides S., Kiehart D. P., Edwards G. S. (2003). Forces for morphogenesis investigated with laser microsurgery and quantitative modeling. Science 300, 145–149 [Abstract] [Google Scholar]
- Jacinto A., Wood W., Woolner S., Hiley C., Turner L., Wilson C., Martinez-Arias A., Martin P. (2002). Dynamic analysis of actin cable function during Drosophila dorsal closure. Curr. Biol. 12, 1245–1250 [Abstract] [Google Scholar]
- Kamiyama D., Chiba A. (2009). Endogenous activation patterns of Cdc42 GTPase within Drosophila embryos. Science 324, 1338–1340 [Europe PMC free article] [Abstract] [Google Scholar]
- Karlsson C., Korayem A. M., Scherfer C., Loseva O., Dushay M. S., Theopold U. (2004). Proteomic analysis of the Drosophila larval hemolymph clot. J. Biol. Chem. 279, 52033–52041 [Abstract] [Google Scholar]
- Kiehart D. P., Galbraith C. G., Edwards K. A., Rickoll W. L., Montague R. A. (2000). Multiple forces contribute to cell sheet morphogenesis for dorsal closure in Drosophila. J. Cell Biol. 149, 471–490 [Europe PMC free article] [Abstract] [Google Scholar]
- Kim M., McGinnis W. (2011). Grainy head phosphorylation is essential for wound-dependent regeneration of an epidermal barrier but dispensable for embryonic barrier development. Proc. Natl. Acad. Sci. USA 108, 650–655 [Europe PMC free article] [Abstract] [Google Scholar]
- Kwon Y. C., Baek S. H., Lee H., Choe K. M. (2010). Nonmuscle myosin II localization is regulated by JNK during Drosophila larval wound healing. Biochem. Biophys. Res. Commun. 393, 656–661 [Abstract] [Google Scholar]
- Lee N., Maurange C., Ringrose L., Paro R. (2005). Suppression of Polycomb group proteins by JNK signalling induces transdetermination in Drosophila imaginal discs. Nature 438, 234–237 [Abstract] [Google Scholar]
- Lemaitre B., Hoffmann J. (2007). The host defense of Drosophila melanogaster. Annu. Rev. Immunol. 25, 697–743 [Abstract] [Google Scholar]
- Lesch C., Jo J., Wu Y. J., Fish G. S., Galko M. J. (2010). A targeted UAS-RNAi screen in Drosophila larvae identifies wound closure genes regulating distinct cellular processes. Genetics 186, 943–957 [Europe PMC free article] [Abstract] [Google Scholar]
- Li G. C., Gustafson-Brown C., Hanks S. K., Nason K., Arbeit J. M., Pogliano K., Wisdom R. M., Johnson R. S. (2003). c-Jun isessential fororganization of the epidermal leading edge. Dev. Cell 4, 865–877 [Abstract] [Google Scholar]
- Mace K. A., Pearson J. C., McGinnis W. (2005). An epidermal barrier wound repair pathway in Drosophila is mediated by grainy head. Science 308, 381–385 [Abstract] [Google Scholar]
- Martin P. (1997). Wound healing-Aiming for perfect skin regeneration. Science 276, 75–81 [Abstract] [Google Scholar]
- Martin P., Nobes C. D. (1992). An early molecular-component of the wound-healing response in rat embryos-induction of c-fos protein in cells at the epidermal wound margin. Mech. Dev. 38, 209–216 [Abstract] [Google Scholar]
- Masterson J. C., Molloy E. L., Gilbert J. L., McCormack N., Adams A., O’Dea S. (2011). Bone morphogenetic protein signalling in airway epithelial cells during regeneration. Cell. Signal. 23, 398–406 [Abstract] [Google Scholar]
- Mattila J., Omelyanchuk L., Nokkala S. (2004). Dynamics of decapentaplegic expression during regeneration of the Drosophila melanogaster wing imaginal disc. Int. J. Dev. Biol. 48, 343–347 [Abstract] [Google Scholar]
- Millard T. H., Martin P. (2008). Dynamic analysis of filopodial interactions during the zippering phase of Drosophila dorsal closure. Development 135, 621–626 [Europe PMC free article] [Abstract] [Google Scholar]
- Moreira S., Stramer B., Evans I., Wood W., Martin P. (2010). Prioritization of competing damage and developmental signals by migrating macrophages in the Drosophila embryo. Curr. Biol. 20, 464–470 [Abstract] [Google Scholar]
- Pearson J. C., Juarez M. T., Kim M., McGinnis W. (2009). Multiple transcription factor codes activate epidermal wound-response genes in Drosophila. Proc. Natl. Acad. Sci. USA 106, 2224–2229 [Europe PMC free article] [Abstract] [Google Scholar]
- Ramet M., Lanot R., Zachary D., Manfruelli P. (2002). JNK signaling pathway is required for efficient wound healing in Drosophila. Dev. Biol. 241, 145–156 [Abstract] [Google Scholar]
- Riedl J., Crevenna A. H., Kessenbrock K., Yu J. H., Neukirchen D., Bista M., Bradke F., Jenne D., Holak T. A., Werb Z., et al. (2008). Lifeact: a versatile marker to visualize F-actin. Nat. Methods 5, 605–607 [Europe PMC free article] [Abstract] [Google Scholar]
- Shaw T., Martin P. (2009). Epigenetic reprogramming during wound healing: loss of polycomb-mediated silencing may enable upregulation of repair genes. EMBO Rep. 10, 881–886 [Europe PMC free article] [Abstract] [Google Scholar]
- Siekhaus D., Haesemeyer M., Moffitt O., Lehmann R. (2010). RhoL controls invasion and Rap1 localization during immune cell transmigration in Drosophila. Nat. Cell Biol. 12, 605–610 [Europe PMC free article] [Abstract] [Google Scholar]
- Smith-Bolton R. K., Worley M. I., Kanda H., Hariharan I. K. (2009). Regenerative growth in Drosophila imaginal discs is regulated by Wingless and Myc. Dev. Cell 16, 797–809 [Europe PMC free article] [Abstract] [Google Scholar]
- Somogyi K., Rorth P. (2004). Evidence for tension-based regulation of Drosophila MAL and SRF during invasive cell migration. Dev. Cell 7, 85–93 [Abstract] [Google Scholar]
- Stewart S., Tsun Z. Y., Belmonte J. C. I. (2009). A histone demethylase is necessary for regeneration in zebrafish. Proc. Natl. Acad. Sci. USA 106, 19889–19894 [Europe PMC free article] [Abstract] [Google Scholar]
- Stramer B., Wood W., Galko M. J., Redd M. J., Jacinto A., Parkhurst S. M., Martin P. (2005). Live imaging of wound inflammation in Drosophila embryos reveals key roles for small GTPases during in vivo cell migration. J. Cell Biol. 168, 567–573 [Europe PMC free article] [Abstract] [Google Scholar]
- Stramer B. M., Mori R., Martin P. (2007). The inflammation-fibrosis link? A Jekyll and Hyde role for blood cells during wound repair. J. Invest. Dermatol. 127, 1009–1017 [Abstract] [Google Scholar]
- Stramer B., Winfield M., Shaw T., Millard T. H., Woolner S., Martin P. (2008). Gene induction following wounding of wild-type versus macrophage-deficient Drosophila embryos. EMBO Rep. 9, 465–471 [Europe PMC free article] [Abstract] [Google Scholar]
- Stramer B., Moreira S., Millard T., Evans I., Huang C. Y., Sabet O., Milner M., Dunn G., Martin P., Wood W. (2010). Clasp-mediated microtubule bundling regulates persistent motility and contact repulsion in Drosophila macrophages in vivo. J. Cell Biol. 189, 681–689 [Europe PMC free article] [Abstract] [Google Scholar]
- Ting S. B., Caddy J., Hislop N., Wilanowski T., Auden A., Zhao L. L., Ellis S., Kaur P., Uchida Y., Holleran W. M., et al. (2005). A homolog of Drosophila grainy head is essential for epidermal integrity in mice. Science 308, 411–413 [Abstract] [Google Scholar]
- Tucker P. K., Evans I. R., Wood W. (2011). Ena drives invasive macrophage migration in Drosophila embryos. Dis. Model. Mech. 4, 126–134 [Europe PMC free article] [Abstract] [Google Scholar]
- Valanne S., Wang J. H., Rämet M. (2011). The Drosophila Toll signaling pathway. J. Immunol. 186, 3323–3332 [Abstract] [Google Scholar]
- Vlisidou I., Dowling A. J., Evans I. R., Waterfield N., ffrench-Constant R. H., Wood W. (2009). Drosophila embryos as model systems for monitoring bacterial infection in real time. PLoS Pathog. 5, e1000518. [Europe PMC free article] [Abstract] [Google Scholar]
- Wang S. Q., Tsarouhas V., Xylourgidis N., Sabri N., Tiklova K., Nautiyal N., Gallio M., Samakovlis C. (2009). The tyrosine kinase Stitcher activates Grainy head and epidermal wound healing in Drosophila. Nat. Cell Biol. 11, 890–895 [Abstract] [Google Scholar]
- Werner S., Smola H., Liao X., Longaker M. T., Krieg T., Hofschneider P. H., Williams L. T. (1994). The function of KGF in morphogenesis of epithelium and reepithelialization of wounds. Science 266, 819–822 [Abstract] [Google Scholar]
- Wigglesworth V. B. (1937). Wound healing in an insect (Rhodnius prolixus hemiptera). J. Exp. Biol. 14, 364–381 [Google Scholar]
- Wood W., Jacinto A. (2007). Drosophila melanogaster embryonic haemocytes: masters of multitasking. Nat. Rev. Mol. Cell Biol. 8, 542–551 [Abstract] [Google Scholar]
- Wood W., Jacinto A., Grose R., Woolner S., Gale J., Wilson C., Martin P. (2002). Wound healing recapitulates morphogenesis in Drosophila embryos. Nat. Cell Biol. 4, 907–912 [Abstract] [Google Scholar]
- Wood W., Faria C., Jacinto A. (2006). Distinct mechanisms regulate hemocyte chemotaxis during development and wound healing in Drosophila melanogaster. J. Cell Biol. 173, 405–416 [Europe PMC free article] [Abstract] [Google Scholar]
- Wu Y., Brock A. R., Wang Y., Fujitani K., Ueda R., Galko M. J. (2009). A blood-borne PDGF/VEGF-like ligand initiates wound-induced epidermal cell migration in Drosophila larvae. Curr. Biol. 19, 1473–1477 [Europe PMC free article] [Abstract] [Google Scholar]
- Zenz R., Scheuch H., Martin P., Frank C., Eferl R., Kenner L., Sibilia M., Wagner E. F. (2003). c-Jun regulates eyelid closure and skin tumor development through EGFR signaling. Dev. Cell 4, 879–888 [Abstract] [Google Scholar]
Articles from Disease Models & Mechanisms are provided here courtesy of Company of Biologists
Citations & impact
Impact metrics
Citations of article over time
Alternative metrics

Discover the attention surrounding your research
https://www.altmetric.com/details/102386268
Smart citations by scite.ai
Explore citation contexts and check if this article has been
supported or disputed.
https://scite.ai/reports/10.1242/dmm.006825
Article citations
The Enigmas of Tissue Closure: Inspiration from Drosophila.
Curr Issues Mol Biol, 46(8):8710-8725, 09 Aug 2024
Cited by: 0 articles | PMID: 39194731 | PMCID: PMC11352826
Review Free full text in Europe PMC
Deep learning for rapid analysis of cell divisions in vivo during epithelial morphogenesis and repair.
Elife, 12:RP87949, 23 Sep 2024
Cited by: 2 articles | PMID: 39312468 | PMCID: PMC11419669
Wound Repair of the Cell Membrane: Lessons from Dictyostelium Cells.
Cells, 13(4):341, 14 Feb 2024
Cited by: 0 articles | PMID: 38391954 | PMCID: PMC10886852
Review Free full text in Europe PMC
On the origin of the functional versatility of macrophages.
Front Physiol, 14:1128984, 23 Feb 2023
Cited by: 6 articles | PMID: 36909237 | PMCID: PMC9998073
Wound Healing Insights from Flies and Fish.
Cold Spring Harb Perspect Biol, 14(11):a041217, 01 Nov 2022
Cited by: 2 articles | PMID: 35817511 | PMCID: PMC9620851
Review Free full text in Europe PMC
Go to all (57) article citations
Data
Similar Articles
To arrive at the top five similar articles we use a word-weighted algorithm to compare words from the Title and Abstract of each citation.
Crawling wounded: molecular genetic insights into wound healing from Drosophila larvae.
Int J Dev Biol, 62(6-7-8):479-489, 01 Jan 2018
Cited by: 15 articles | PMID: 29938760 | PMCID: PMC6352908
Review Free full text in Europe PMC
Identification and functional analysis of healing regulators in Drosophila.
PLoS Genet, 11(2):e1004965, 03 Feb 2015
Cited by: 5 articles | PMID: 25647511 | PMCID: PMC4315591
Why mammalian wound-healing researchers may wish to turn to Drosophila as a model.
Exp Dermatol, 23(8):538-542, 21 Jul 2014
Cited by: 3 articles | PMID: 24942366
JNK-mediated Slit-Robo signaling facilitates epithelial wound repair by extruding dying cells.
Sci Rep, 9(1):19549, 20 Dec 2019
Cited by: 8 articles | PMID: 31863086 | PMCID: PMC6925126
Funding
Funders who supported this work.
Wellcome Trust (1)
Drosophila embryonic macrophages as a model system for studying migration and bacterial infection in real time.
Prof Will Wood, University of Bath
Grant ID: 090899