Abstract
Free full text

Heat shock protein 90 (HSP90) contributes to cytosolic translocation of extracellular antigen for cross-presentation by dendritic cells
Associated Data
Abstract
In antigen (Ag) cross-presentation, dendritic cells (DCs) take up extracellular Ag and translocate them from the endosome to the cytosol for proteasomal degradation. The processed peptides can enter the conventional MHC I pathway. The molecules responsible for the translocation of Ag across the endosomal membrane into the cytosol are unknown. Here we demonstrate that heat shock protein 90 (HSP90) is critical for this step. Cross-presentation and -priming were decreased in both HSP90α-null DCs and mice. CD8α+ DC apoptosis mediated by translocation of exogenous cytochrome c to the cytosol was also eliminated in HSP90α-null mice. Ag translocation into the cytosol was diminished in HSP90α-null DCs and in DCs treated with an HSP90 inhibitor. Internalized Ag was associated with HSP90 and translocated to the cytosol, a process abrogated by the HSP90 inhibitor. Ag within purified phagosomes was released in an HSP90-dependent manner. These results demonstrate the important role of HSP90 in cross-presentation by pulling endosomal Ag out into the cytosol.
Cytosolic antigens (Ags) are degraded by the ubiquitin-proteasome (UP) pathway into short peptides and presented by MHC I molecules to CD8+ T cells (1). Extracellular Ags, on the other hand, once internalized into the endosome/phagosome of antigen presenting cells (APCs) can undergo two distinct fates. In the first “classical” pathway, the Ag is targeted from the endosome to the lysosome, where it is processed for MHC II-restricted presentation to CD4+ T cells. The second pathway involves translocation of the Ag from the endosome into the cytosol where it is degraded by the UP system, ultimately allowing its presentation by MHC class I molecules. This latter process is called cross-presentation (2–4) and is especially important to ensure effective host defense against viral infection or malignant transformation (5).
A perplexing issue related to cross-presentation has been the mechanism by which exogenous Ag is translocated across endosomal membranes into the cytosol. Misfolded proteins in the endoplasmic reticulum (ER) can be retrotranslocated to the cytosol for degradation by the proteasome, a process known as the ER-associated degradation (ERAD) (6, 7). An important component of this process is the ER-dislocon machinery, which consists of the Sec61 complex and the AAA-ATPase p97 (6, 7). Because these components are also present in the phagosome/endosomes (8), it is thought that the same dislocon machinery is also involved in the translocation of the Ag for cross-presentation (9–11). However, the cytosolic molecular entity providing the “driving force” that interacts with Ag as it translocates across the endosomal membranes remains unknown.
Heat shock protein 90 (HSP90) has been suggested to play a role in the endogenous MHC I antigen processing pathway (12–15). HSP90 associates with peptides as postproteasomal degradation products (14) or with newly synthesized polypeptides destined for degradation by the proteasome (13). Those peptides have been implicated as precursors of MHC class I ligands (13, 16). The HSP90-peptide/protein complex in Ag-donor cells seems to be prerequisite for cross-priming because cells treated with an HSP90-specific inhibitor or heat shock factor 1 (HSF-1)–deficient cells in which HSP90 is down-regulated, are less efficient in cross-priming ability (14, 17–19). In contrast to this view from Ag-donor cells, we recently performed experiments from the perspective of the Ag-accepting cells such as dendritic cells (DCs) and found that HSP90 within the DC plays a pivotal role in cross-presentation (20). Experiments using siRNA suggested that the inducible form of HSP90, HSP90α, plays a dominant role and HSP90β does to a lesser extent in cross-presentation by the DC-like cell line, DC2.4 (20).
Despite its known importance, the mechanism by which HSP90α is involved in cross-presentation is unclear. Moreover, the role of endogenous HSP90α in DC cross-priming has only been shown in vitro; its in vivo significance is completely unknown. To gain insight into these issues, we have generated HSP90α-null mice and found that translocation of extracellular Ag into the cytosol is diminished in HSP90α-null DCs and in DCs treated with a specific HSP90 inhibitor. We demonstrate here the indispensable role of cytosolic HSP90α in Ag translocation into the cytosol during cross-presentation.
Results
Cross-Presentation Ability Is Down-Regulated in Bone Marrow-Derived Dendritic Cells (BMDCs) Treated with HSP90 Inhibitors and in HSP90α-Deficient BMDCs.
Our initial experiments showed that, as predicted, the HSP90 inhibitors radicicol (Rad) and novobiocin (NB) blocked cross-presentation of a model antigen, ovalbumin (OVA) in the DC-like cell line, DC2.4 in a dose-dependent manner (Fig. 1A, Left) (20). Another inhibitor, geldanamycin (GA), when conjugated with FITC (GA–FITC) is cell-membrane impermeable, binding only cell surface HSP90 (Fig. 1B, Left) and inhibiting its functions but having no effect on cytosolic HSP90 (21, 22). We found that GA but not GA–FITC blocked cross-presentation (Fig. 1B, Right), indicating the involvement of cytosolic but not cell surface HSP90. Rad, NB, and GA did not affect presentation by DC2.4 cells pulsed with OVA257–264 peptide (Fig. 1A, Right), suggesting that the inhibitors did not influence antigen presentation on the cell surface.
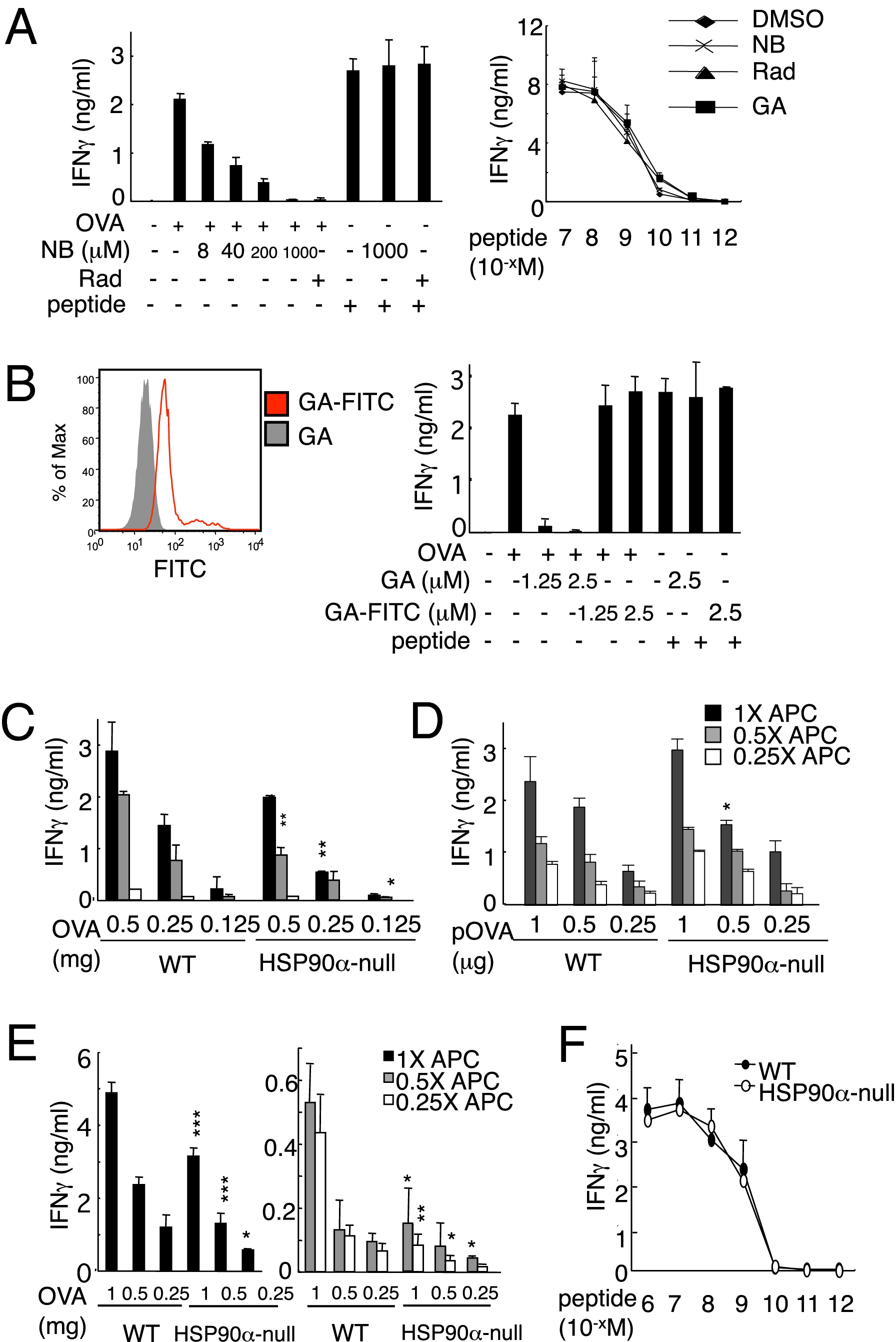
Cross-presentation depends on endogenous HSP90. (A) HSP90 inhibitors block cross-presentation of soluble OVA. DC2.4 cells were pretreated with HSP90 inhibitor NB at the indicated doses or with 25 μM of Rad and pulsed with 1 mg/mL of soluble OVA or 10−9 M peptide, then cocultured with OT-I CD8+ T cells (Left). (Right) DC2.4 cells were pretreated with NB (1,000 μM), Rad (25 μM), or GA (2.5 μM) and pulsed with 10−7 to 10−12M OVA peptide (OVA256–264) for 1 h, then cocultured with OT-I CD8+ T cells. The culture supernatants were collected for measurement of IFNγ. (B) Importance of intracellular HSP90 in cross-presentation. DC2.4 cells were pretreated with the general HSP90 inhibitor (GA) or the inhibitor of only cell surface HSP90 (GA–FITC) for 60 min, then analyzed by flow cytometry (Left). The pretreated DC2.4 cells were then used for the cross-presentation assay as APC (Right). (C, E, and F) BMDCs from wild-type (WT) or HSP90α-null mice were pulsed with graded doses of soluble OVA (C) or cell-associated OVA (E; described in Materials and Methods) or OVA peptide (F) for the cross-presentation assay. (D) BMDCs from WT or HSP90α-null mice were transfected with titrated doses of a plasmid, containing a cDNA encoding OVA60–386 and cocultured with OT-I CD8+ T cells for the Ag presentation assay. The values were compared between WT and HSP90α-null. Data are mean ± SD. *P < 0.05; **P < 0.01; ***P < 0.001. The results were confirmed in at least two independent experiments.
NB targets the C-terminal portion of the protein (23), which is critical for the formation of HSP90 dimers to exert its chaperone activity (24), whereas Rad and GA bind the N-terminal ATP-binding pocket to block the ATP-dependent chaperone cycling (25). On the basis of this information, we generated conditional HSP90α-null mice by floxing exons 9 and 10, which encode the C-terminal region of the protein (Fig. S1A). We crossed HSP90aa1neo mice with CAG-cre mice, which express cre recombinase under the control of the CAG promoter (a combination of the chicken β-actin promoter and cytomegalovirus immediate-early enhancer) (26) and obtained HSP90aa1+/− mice. We anticipated that HSP90aa1−/− would be embryonic lethal; however, surprisingly, the HSP90aa1−/− offspring were born at the expected Mendelian ratio and survived and grew normally. Homologous recombination and deletion of exons 9 and 10 were confirmed by PCR-based genotyping (Fig. S1 B and C). Western blot analysis verified the effectiveness of our gene targeting strategy; HSP90β but not HSP90α was readily detectable in multiple organs tested (Fig. S1E). We could detect mRNA encoding exons 3–11 (Fig. S1D) but no truncated forms of HSP90α, indicating that the mutant protein is rapidly degraded after synthesis, probably because it is unable to form dimers. (Exon 8 is spliced to exon 11, resulting in a frameshift with a premature termination codon that eliminates the region of the C terminus involved in dimer formation.) T-cell development and cell subpopulations in the lymphoid tissues of HSP90aa1−/− mice were equivalent to those of the wild-type (WT) mice (Fig. S2 A and B). Moreover, granulocyte macrophage colony-stimulating factor (GM-CSF)–dependent BMDCs with a normal phenotype could be generated from HSP90aa1−/− mice (Fig. S2C).
With these mice in hand, we could unequivocally define the direct and cross-presentation function of HSP90α in BMDCs. BMDCs from HSP90α-null and WT mice were pulsed with OVA protein as indicated (Fig. 1C) or transfected with a plasmid containing a cDNA (pOVA) encoding OVA60–386 (Fig. 1D). Cross-presentation was partially but significantly down-regulated in HSP90α-null BMDCs, whereas direct presentation was less affected (Fig.1 C and D and Fig.S3 A and B). Rad completely blocked residual cross-presentation activity of HSP90α-null BMDCs (Fig. S3A), indicating that, in addition to HSP90α, HSP90β is involved in cross-presentation. We examined internalization of FITC-OVA and found that it was essentially equivalent in WT and HSP90α-null BMDCs (Fig. S3C). To examine cross-presentation of cellular antigens, we used UV-irradiated transporter associated with antigen processing (TAP)1-deficient spleen cells that were osmotically loaded with OVA. BMDCs were pulsed with this cell-associated OVA and then incubated with OT-ICD8+ T cells. Here, again we found a defect in the HSP90α-null BMDCs, in this case manifest by a significant reduction in IFNγ production (Fig. 1E). This defect was not due to the inability of HSP90α-null BMDCs to activate T cells because pulsing the cells with the OVA257–264 epitope resulted in comparable T-cell activation by HSP90α-null and WT BMDCs (Fig. 1F). These results indicate that endogenous HSP90 is essential for cross-presentation.
Translocation of Internalized Ag into the Cytosol Is Dependent on Cytosolic HSP90 Function.
To better understand why HSP90 is required for cross-presentation, we used the ImageStream system (27, 28), which can detect subtle changes in signal distribution (Fig. S4). BMDCs were labeled with PKH67, a lipophilic general membrane-labeling reagent. The green-labeled BMDCs (PKH67_DC) were pulsed for 5 min with AF647 red-labeled OVA (AF647_OVA) and then washed, followed by ImageStream analysis. Once the OVA is internalized into endosomes, the AF647_OVA and PKH67_DC signals within the endosomes merge, resulting in a yellow emission, which was indicated as internalization (+)/colocalization (+). By contrast, AF647_OVA that was internalized but then translocated into the cytosol was red because PKH67 labels only membranes. Cells with this distribution were identified as internalization (+)/colocalization (−) (Fig. S5 B and D). AF647_OVA translocation to the cytosol was less frequent in the HSP90α-null DC compared with the WT DC, and Rad treatment further reduced the frequency in both types of DC (Figs. S4C and S5 A and C). Phagocytosis and internalization efficiency of both soluble and cell-associated OVA were comparable between WT and HSP90a-null BMDCs (Fig. S6). Therefore, the process of translocation of internalized Ags into the cytosol appears to be HSP90 dependent.
The ImageStream analysis was quite informative; however, one technical issue we had to consider was that because the optical z-axis stack of ImageStream is 4.0 μm, it has less focal depth compared with general confocal microscopy (0.1–0.2 μm). Therefore, we used an additional approach to confirm the ImageStream results by counting individual spots (endosome) to determine whether they were merged (yellow) or not (red) by fluorescence microscopy (Keyence; BZ-9000) analysis (0.3–0.4 μm in z-axis stack). We could detect clearly distinguishable countable spots in both DC2.4 cells (Fig. 2A) and BMDCs (Fig. 2D) internalizing OVA. More than 16,000 spots in 800 DC2.4 cells were identified in four independent experiments. Rad-treatment kept most of internalized OVA (red) within endosomes (green), thus resulting in yellow-colored vesicles (Fig. 2 B and C). It should be noted that the total number of vesicles per cell was equal, with or without Rad (Fig. 2C); thus, Rad treatment had no significant effect on internalization. The same experiments were performed with BMDCs from WT and HSP90α-null mice (Fig. 2D). We examined more than 50,000 vesicles in 1,200 cells and obtained similar results with DC2.4 cells (Fig. 2 E and F). Together with the ImageStream analysis, these results clearly indicate that the translocation of internalized Ag to the cytosol depends on HSP90α (or HSP90).
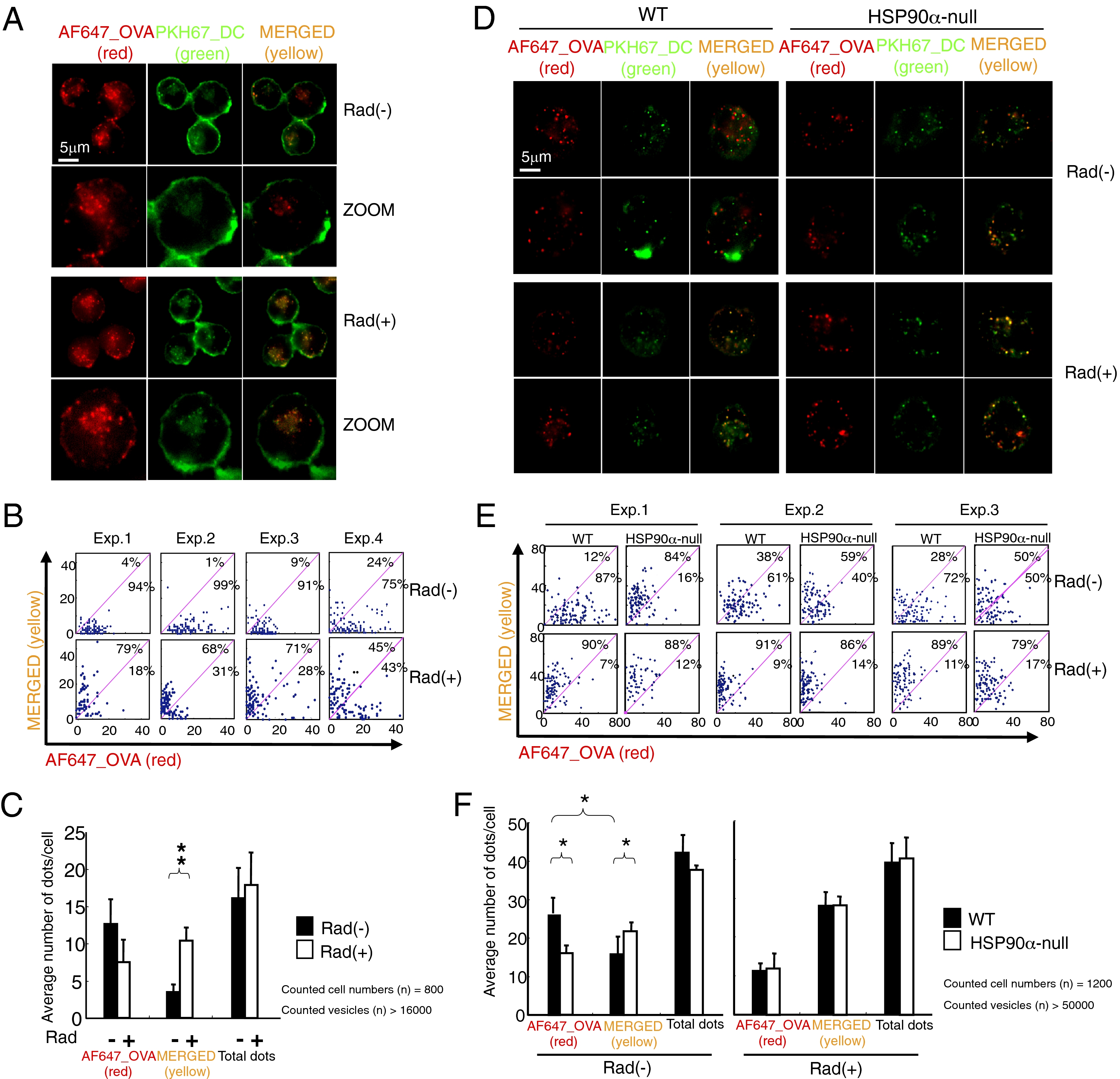
Quantitative analysis of the subcellular localization of pulsed OVA using fluorescence microscopy. DC2.4 cells (A–C) and BMDCs (D–F) were first cultured on glass bottom dishes for ~16 h and treated with MG115, with/without Rad for 15 min. The cells were then stained with PKH67 on ice for visualization of the membranes, then pulsed with AF647-labeled OVA for 5 min at 37 °C and fixed. Samples were analyzed by Keyence BZ-9000 fluorescence microscopy. (B and E) Individual spots (endosome) within a cell in A or D were analyzed to determine whether the color was red or yellow using an overlay program. Each dot in the panel was plotted according to the numbers of red and yellow spots. Each panel includes 100 dots, thus, 100 cells. (C and F) The results of B and E were summarized as bar graphs. Data are mean ± SD of three or four separate experiments. *P < 0.05, **P < 0.01.
To further evaluate the translocation of extracellular OVA to the cytosol, subcellular fractions of DC2.4 cells were prepared using a ProteoExtract Subcellular Proteome Extraction kit and then examined for the presence of internalized OVA. We first validated the assay by determining whether ER and lysosomal proteins remained membrane associated. Internalized OVA proteins were to be identified in the cytosolic and membrane fractions at 5 min after loading of the protein, so we extracted cytosolic proteins for 5, 10, and 30 min with solution I contained in the kit and did not observe any leakage of the ER-luminal proteins (KDEL-positive resident ER molecules) or a lysosomal protein, cathepsin D, into the cytosolic fraction (Fig. S7A). The efficiency of fractionation was assessed by tracking the location of several organelle-specific proteins as indicated (Fig. S7B). Rad reduced the amount of OVA in the cytosolic faction in a dose-dependent manner, whereas there was no effect on HSC70, a cytosolic protein, and again, no leakage of KDEL-positive molecules or cathepsin D into the cytosol was observed (Fig. 3A and Fig. S8 A and C). OVA appearance in the cytosol was not blocked by an inhibitor of the ubiquitin activator E1, UBEI-41 (Fig. S8D), although the same inhibitor completely blocked cross-presentation (20). As a further indication of the specificity of these observations, MG115 and Rad did not affect the uptake of FITC-OVA into DC2.4 cells measured at the same time point as the fractionation experiments (Fig. S8B). Similar experiments were performed using BMDCs and we found that the amount of OVA in the cytosol was much less in HSP90α-null than in WT BMDC; conversely, there was more OVA in the membrane/fraction in the HSP90α-null than in WT BMDCs (Fig. 3B).

HSP90 is required in translocation of internalized Ag to the cytosol. (A) DC2.4 cells were treated with the indicated dose of Rad and/or the proteasome inhibitor MG115 (10 μM) and pulsed with 3 mg/mL of OVA for 5 min. After extensive washing, the cells were fractionated using a subcellular fractionation kit (Calbiochem). The cytosol and membrane fractions were subjected to Western blot using the indicated antibodies. (B) BMDCs from WT or HSP90α-null mice were used for the translocation assay as in A. (C) DC2.4 cells were treated with the proteasome inhibitor MG115 (10 μM) with/without Rad (25 μM) or Exo A (5 μg/mL) and pulsed with biotin–OVA (1 mg/mL) for 10 min. The cells were lysed and immunoprecipitated with anti-HSP90 mAb or control IgG conjugated Dynabeads. After immunoprecipitation (IP), the samples were analyzed by immunoblotting with streptavidin (SA)–HRP. (D) DC2.4 cells were pulsed with biotin–OVA and separated into the cytosol and noncytosol fractions and subjected to IP. Representative results of at least three experiments are shown. *P < 0.05.
Importantly, anti-HSP90 mAb coprecipitated OVA from extracts of control DC2.4 cells but significantly less OVA was recovered from Rad-treated cells (Fig. 3C). By contrast, treatment with the ubiquitin E1 inhibitor did not affect the amount of coprecipitated OVA (Fig. S8E). Next, immunoprecipitation with anti-HSP90 mAb was performed with cytosolic and membrane fractions of DC2.4 cells after internalization of OVA. The association of HSP90 with internalized OVA was found to occur mainly in the membrane factions and not in the cytosol (Fig. 3D). We could exclude possible postlysis association of HSP90 with OVA on the basis of the following control experiments: The addition of a DC2.4 cell extract containing normal HSP90 to the extract of Rad-treated OVA-internalized DC2.4 cells significantly reduced the amount of OVA that coprecipitated with HSP90 compared with control Rad-untreated, OVA-internalized DC2.4 cells (Fig. S8F). Considered together, the results strongly imply that HSP90 is essential for translocation of OVA across or adjacent to the endosomal membranes and that Rad blocks this process.
To further test this hypothesis, we used an in vitro OVA translocation assay with phagosomes purified by the previously described latex beads (LB)-based method (29). Phagosomes purified from DC2.4 cells that internalized 0.8 μm LB and OVA for 5, 15, and 60 min contained OVA, LAMP1, HSP90, HSC70, and Sec61β (Fig. S9A). There was no release of OVA from phagosomes alone (Fig. S9 B and C), which validates the translocation assay we used. Notably, the addition of cytosol obtained from DC2.4 cells to phagosomes purified from DC2.4 cells that had internalized LB and OVA for just 5 min resulted in release of the OVA, a response that was significantly reduced in the presence of radicicol (Fig. 4A). By contrast, the cytosol from HSP90α-null DCs caused a weaker release of OVA (Fig. 4B). In other experiments, we used purified HSP90 instead of the cytosol in the OVA translocation assay. HSP90 but not the control protein BSA stimulated the translocation (Fig. S9D). The magnitude of the release was HSP90 dose (Fig. 4C) and temperature dependent, being abrogated at 4 °C (Fig. 4D). Pretreatment of HSP90 with radicicol inhibited OVA release in a dose-dependent manner (Fig. 4E). To ensure that the observed translocation was a broadly applicable HSP90 function, we analyzed another protein, Cryj1, an antigen of Japanese cedar pollen, and found that it too was also translocated to the cytosol in a HSP90-dependent manner after a 5-min internalization (Fig. 4F).

HSP90 is a translocator for engulfed extracellular antigen. (A) Ag transport assay. Purified phagosomes from DC2.4 cells containing OVA and/or latex beads were mixed with cytosol from DC2.4 cells treated with or without Rad. Thirty minutes after incubation at 37 °C, the samples were centrifuged and separated into pellet (P) and supernatant (S) for Western blot. (B) The cytosol from BMDCs of WT and HSP90α-null mice was used in the Ag transport assay. Thus, the cytosol was incubated with phagosomes from DC2.4 cells. (C) HSP90 was purified from Meth A cells and used as in A, instead of the cytosol. (D) Effects of temperature on antigen transport. (E) A total of 5 μg of HSP90 pretreated with Rad at 0, 25, 100, and 250 μM, respectively, was used. (F) Biotin–Cryj was used in the same assay. Representative results of at least three experiments are shown. *P < 0.05.
Cross-Presentation in Vivo Is Dependent on HSP90.
As the ultimate test of our hypothesis, we performed in vivo experiments to assess the cross-priming ability in HSP90α-null mice. The mice were immunized with Tap1−/− spleen cells osmotically loaded with OVA. One week later, the splenic CD8+T cells were stimulated with OVA257–264 epitope pulsed DC2.4 cells and IFNγ spots were counted (Fig. 5A). The number of cells producing IFNγ was significantly lower in HSP90α-null than in WT mice. Because there was no difference between HSP90α-null and WT mice in direct priming with the epitope pulsed DC2.4 (Fig. 5B), we concluded that HSP90α deficiency results in impairment in cross-priming ability. To analyze cross-presentation from a different perspective, we injected HSP90α-null and WT mice with cytochrome c, a very sensitive protocol that causes CD8α+ DCs to undergo apoptosis because the internalized cytochrome c is translocated into cytosol in this particular DC subset (30). We found that only the CD8α+ DC subset of WT, but not HSP90α-null mice, was significantly reduced by cytochrome c administration (Fig. 5 C and D). These results provide compelling confirmation of the physiological importance of HSP90α in cross-presentation.
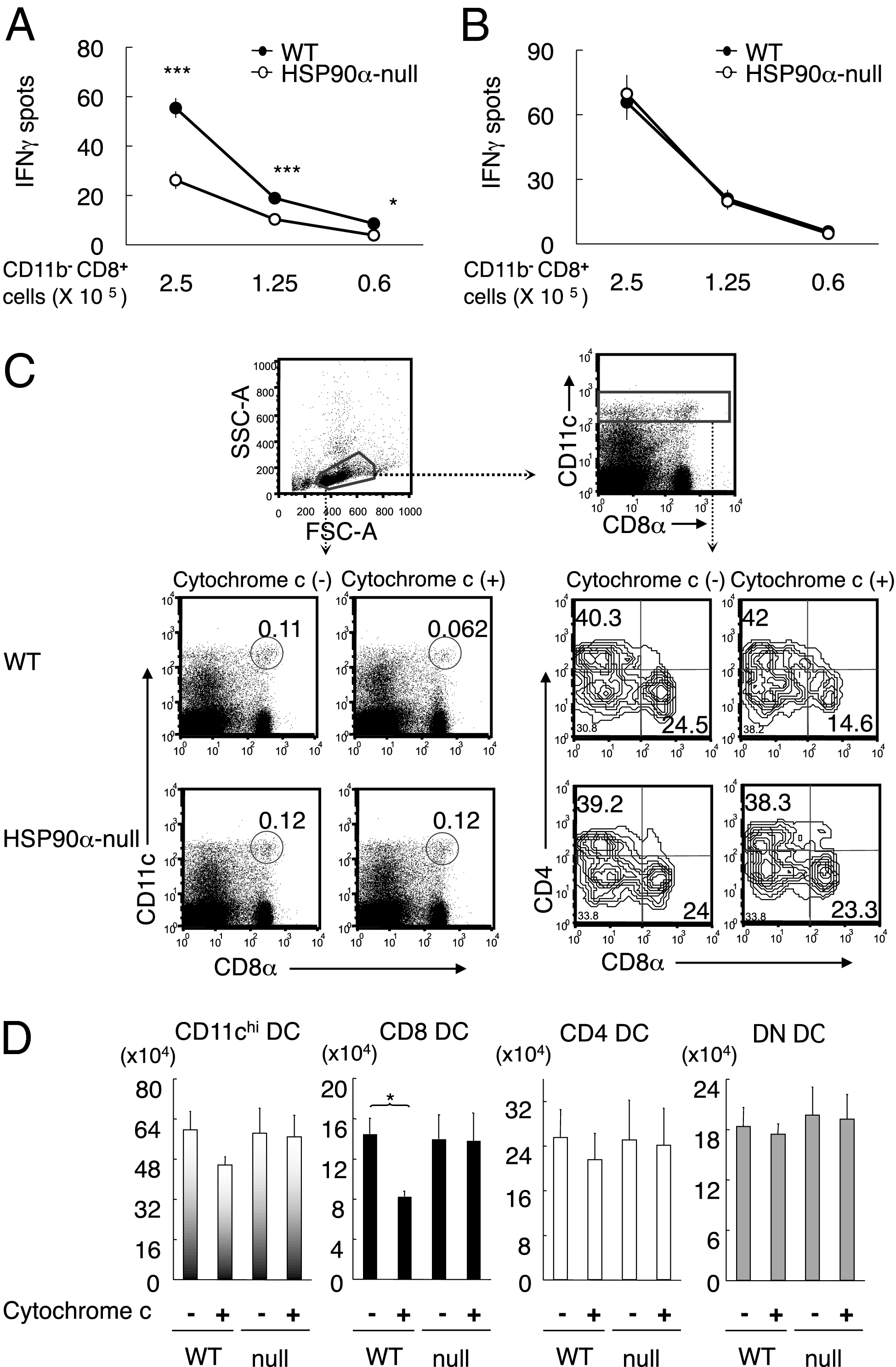
In vivo cross-presentation is decreased in HSP90α-null mice. (A and B) WT or HSP90α-null mice were immunized with cell-associated OVA (2 × 106 cells/mouse, A) or OVA257–264 pulsed DC2.4 (2 × 106 cells/mouse, B). Seven days later, the frequency of OVA-specific splenic CD8+ T cells was measured by IFNγ ELISPOT in response to peptide-pulsed DC2.4. Data are mean ± SE from four independent experiments including 10 mice in A and 4 mice in B. *P < 0.05, ***P < 0.001, two-tailed unpaired Student t tests. (C and D) Each experiment was performed with a pair of WT and HSP90α-null mice treated with/without 5 mg of cytochrome c. Splenic DCs were analyzed by FACS 24 h after treatment. The results in C are one of the representatives for three independent experiments. Numbers represent the % of cells within the gate (C). (D) Absolute number of CD11c high, CD8+ CD11chigh, CD4+ CD11chigh, and double negative (DN) CD11chigh cells per spleen. Mean ± SE are from C. *P < 0.05, two-tailed unpaired Student t tests.
Discussion
Using HSP90α-deficient mice, to our knowledge this is a unique report demonstrating that DC cytosolic HSP90 is essential for cross-priming of cell-associated Ag. Cross-presentation of both soluble and cell-associated Ag was less efficient in HSP90α-null than WT BMDCs. A twofold reduction in the magnitude of the immune response in HSP90α-null mice is not particularly striking (Fig. 5A). This is due to the effect of HSP90β in the HSP90α-null mice, which is able to play a significant role in translocation of extracellular antigen to the cytosol. The CD8α+ DC subset has been shown to mediate cross-presentation in vivo. Results of the sensitive in vivo cytochrome c-induced apoptosis assay, that apoptosis of CD8α+ DCs was eliminated in HSP90α-null mice, provided conclusive evidence for the importance of HSP90α in the translocation of internalized Ag into the cytosol.
In the subcellular fractionation study, the E1 inhibitor did not block the translocation of OVA to the cytosol (Fig. S8D), although it did block cross-presentation (20). This differential effect is relevant to a previously proposed mechanism whereby ubiquitin conjugation serves as a “ratchet” to dislocate ER-misfolded proteins into the cytosol, a model based on the fact that most of the proteins exported from the ER are polyubiquitinylated (31, 32). However, our results indicate that ubiquitination is essential for the proteasome-mediated processing of T-cell epitopes but is dispensable for the translocation of endosomal antigen into the cytosol. Intriguingly, a significant proportion of OVA exported from purified phagosomes after the addition of cytosol contained bands with a slower mobility than the native OVA (Fig. 4 A and B). By contrast, the mobility of the OVA and Cryj1 whose export was induced by purified HSP90 was exactly the same as the native proteins (Fig. 4 C–F). Polyubiquitination could account for the observed size increase, but this issue needs to be examined in future experiments. It is nonetheless possible to speculate that HSP90 itself acts as a cytosolic translocation factor for endosomal Ag and that other cytosolic factors then participate in its polyubiquitination.
Immunoprecipitation of HSP90 coprecipitated OVA from extracts of control DC2.4 cells but the OVA signal was weaker in Rad-treated cells (Fig. 3C). By contrast, the E1 inhibitor treatment had no effect on the amount of coprecipitated OVA (Fig. S8E). On the basis of studies showing that HSP90 can drive polypeptides into the mitochondria (33), we hypothesize that HSP90 binds to unfolded Ag, translocating it from the endosome and pulling it into the cytosol. As selective intracellular association of endogenous Ag with HSP90 is known to promote MHC II antigen presentation in B cells (34), it is possible that HSP90 governs the bidirectional Ag traffic between the cytosol and the endosomes, as a result regulating antigen presentation by both MHC I and II.
Pseudomonas aeruginosa exotoxin A (Exo A) was shown to inhibit the ERAD quality control system, preventing the retrotranslocation of ER-proteins/peptides into the cytosol for proteasomal degradation (35). In this case, the Sec61 complex (ER-dislocon) was implicated as the Exo A target molecule (35). On the other hand, Exo A was suggested to be an inhibitor of p97, which was identified as a cytosolic translocator for endosomal Ag (11). We observed that Exo A did not affect the association of OVA with HSP90 (Fig. 3C). Although ExoA suppresses cross-presentation (35), our data suggest that it may play a role downstream of HSP90 during Ag translocation. Elucidation of the entire pathway will require further study.
We demonstrated here that HSP90 is a cytosolic translocator for extracellular antigen in cross-presentation by DC. In addition, growth factors such as fibroblast growth factor (FGF)-1/2 (36) and certain protein toxins like diphtheria toxin appear to use HSP90 for their translocation into cytosol in non-APC (37, 38). Moreover, HSP90 was recently shown to mediate dislocation of the cholera toxin A1 (CTA1) subunit from the ER into the cytosol. ER-to-cytosol export of CTA1 requires association of HSP90 with CTA1, a process blocked by GA treatment (39). Thus, the Hsp90-mediated translocation system might have developed as a common mechanism in a variety of cell types, ultimately leading to an assortment of seemingly unrelated effects such as transcriptional activation (36), intoxication (38), and cross-presentation (this study).
Materials and Methods
Mice.
C57BL/6 (B6) mice and TAP1−/− mice (40) were purchased from Clea and The Jackson Laboratory, respectively. OT-I (H-2Kb restricted, anti-OVA TCR transgenic) mice (41) were kindly provided by W. R. Heath (Walter and Eliza Hall Institute, Melbourne, Victoria, Australia). All mice were maintained under specific pathogen-free conditions in the RIKEN RCAI animal facility according to the institutional guidelines.
Cells.
Bone marrow was harvested from B6 or HSP90α-null mice to generate BMDCs. BMDCs were cultured in 24-well plates at a density of 1 × 106 cells/mL with RPMI 1640 (Sigma) containing 10% FCS, 2 mM l-glutamine, 1 mM sodium pyruvate, 0.1 mM nonessential amino acid, penicillin-streptomycin, 2-mercaptoethanol, and 20 ng/mL GM-CSF (R&D Systems). Immature BMDCs were used in experiments on day 5. OT-I CD8+CD11b− T cells were purified from splenocytes by depletion of CD11b+ cells followed by positive selection of CD8+ cells by magnetic separation with the IMag system (BD Biosciences). The DC2.4 DC cell line was a kindly provided by K. L. Rock (University of Massachusetts Medical School, Worcester, MA).
Acknowledgments
We thank Mr. Sugahara for purification of HSP90 from mouse Meth A tumor cells and Dr. Kenneth Rock who kindly provided the DC2.4 cell line. This work was supported by Grant RIKEN RCAI and also by a Grant-in-Aid for Scientific Research Priority Areas from the Ministry of Education, Science, Sports, and Culture, Japan.
Footnotes
The authors declare no conflict of interest.
*This Direct Submission article had a prearranged editor.
This article contains supporting information online at www.pnas.org/lookup/suppl/10.1073/pnas.1108372108/-/DCSupplemental.
References
Articles from Proceedings of the National Academy of Sciences of the United States of America are provided here courtesy of National Academy of Sciences
Full text links
Read article at publisher's site: https://doi.org/10.1073/pnas.1108372108
Read article for free, from open access legal sources, via Unpaywall:
https://www.pnas.org/content/pnas/108/39/16363.full.pdf
Citations & impact
Impact metrics
Citations of article over time
Alternative metrics
Article citations
Previously unrecognized and potentially consequential challenges facing Hsp90 inhibitors in cancer clinical trials.
Cell Stress Chaperones, 29(5):642-653, 23 Aug 2024
Cited by: 0 articles | PMID: 39181529 | PMCID: PMC11402043
Review Free full text in Europe PMC
Heat shock proteins as hallmarks of cancer: insights from molecular mechanisms to therapeutic strategies.
J Hematol Oncol, 17(1):81, 04 Sep 2024
Cited by: 0 articles | PMID: 39232809 | PMCID: PMC11375894
Review Free full text in Europe PMC
HSP90 multi-functionality in cancer.
Front Immunol, 15:1436973, 01 Aug 2024
Cited by: 0 articles | PMID: 39148727 | PMCID: PMC11324539
Review Free full text in Europe PMC
All-Trans Retinoic Acid-Induced Cell Surface Heat Shock Protein 90 Mediates Tau Protein Internalization and Degradation in Human Microglia.
Mol Neurobiol, 20 Jun 2024
Cited by: 0 articles | PMID: 38900367
Introducing Molecular Chaperones into the Causality and Prospective Management of Autoimmune Hepatitis.
Dig Dis Sci, 68(11):4098-4116, 27 Sep 2023
Cited by: 0 articles | PMID: 37755606 | PMCID: PMC10570239
Review Free full text in Europe PMC
Go to all (100) article citations
Data
Data behind the article
This data has been text mined from the article, or deposited into data resources.
BioStudies: supplemental material and supporting data
Similar Articles
To arrive at the top five similar articles we use a word-weighted algorithm to compare words from the Title and Abstract of each citation.
Extracellular heat shock protein 90 plays a role in translocating chaperoned antigen from endosome to proteasome for generating antigenic peptide to be cross-presented by dendritic cells.
Int Immunol, 23(4):223-237, 18 Mar 2011
Cited by: 45 articles | PMID: 21421737
Essential role of endogenous heat shock protein 90 of dendritic cells in antigen cross-presentation.
J Immunol, 185(5):2693-2700, 28 Jul 2010
Cited by: 41 articles | PMID: 20668218
Heat shock protein magic in antigen trafficking within dendritic cells: implications in antigen cross-presentation in immunity.
Acta Med Okayama, 66(1):1-6, 01 Jan 2012
Cited by: 11 articles | PMID: 22358133
Review
Heat shock protein 90 targets a chaperoned peptide to the static early endosome for efficient cross-presentation by human dendritic cells.
Cancer Sci, 106(1):18-24, 15 Dec 2014
Cited by: 8 articles | PMID: 25414129 | PMCID: PMC4317773