Abstract
Free full text

Dynamic Antibody Specificities and Virion Concentrations in Circulating Immune Complexes in Acute to Chronic HIV-1 Infection
†
Abstract
Understanding the interactions between human immunodeficiency virus type 1 (HIV-1) virions and antibodies (Ab) produced during acute HIV-1 infection (AHI) is critical for defining antibody antiviral capabilities. Antibodies that bind virions may prevent transmission by neutralization of virus or mechanically prevent HIV-1 migration through mucosal layers. In this study, we quantified circulating HIV-1 virion-immune complexes (ICs), present in approximately 90% of AHI subjects, and compared the levels and antibody specificity to those in chronic infection. Circulating HIV-1 virions coated with IgG (immune complexes) were in significantly lower levels relative to the viral load in acute infection than in chronic HIV-1 infection. The specificities of the antibodies in the immune complexes differed between acute and chronic infection (anti-gp41 Ab in acute infection and anti-gp120 in chronic infection), potentially suggesting different roles in immunopathogenesis for complexes arising at different stages of infection. We also determined the ability of circulating IgG from AHI to bind infectious versus noninfectious virions. Similar to a nonneutralizing anti-gp41 monoclonal antibody (MAb), purified plasma IgG from acute HIV-1 subjects bound both infectious and noninfectious virions. This was in contrast to the neutralizing antibody 2G12 MAb that bound predominantly infectious virions. Moreover, the initial antibody response captured acute HIV-1 virions without selection for different HIV-1 envelope sequences. In total, this study demonstrates that the composition of immune complexes are dynamic over the course of HIV-1 infection and are comprised initially of antibodies that nonselectively opsonize both infectious and noninfectious virions, likely contributing to the lack of efficacy of the antibody response during acute infection.
INTRODUCTION
The major challenge to development of a successful human immunodeficiency virus type 1 (HIV-1) preventive vaccine is an incomplete understanding of the correlates of protective immunity to HIV-1 infection. A clear understanding of the early in vivo events following HIV-1 transmission, especially the short time window from transmission to the establishment of the latent pool of HIV-1-infected CD4+ T cells, is critical to the design of a protective vaccine (reviewed in reference 26). Details of the earliest host-pathogen interactions can provide insights into the challenges that the initial immune response may face during transmission and establishment of infection. We have previously reported that the first detectable B-cell response to HIV-1 in acute HIV-1 infection (AHI) is in the form of immunoglobulin (Ig)–HIV-1 virion immune complexes (ICs) approximately 8 days after the time of the first detectable plasma viral load (T0; 100 copies/ml). The appearance of plasma ICs is followed by the HIV-1-specific virus-free plasma antibody (Ab) against HIV-1 Env gp41, a median of 13 days post-T0 (44).
Antibodies induced by viral infections can contribute to antiviral immunity by directly neutralizing free virus particles, activating complement, mediating opsonization and phagocytosis, and facilitating Ab-dependent cellular cytotoxicity (ADCC) (42, 43). ICs in HIV-1 infection have numerous biological consequences that depend in part on the binding of the Fc region of IgG to Fc receptors on the surfaces of antigen-presenting cells (e.g., macrophages and dendritic cells) or natural killer (NK) cells and also depend on the antigen specificity of the antibody. FcγRIIa was associated with the progression of HIV-1 infection in vivo (13), and FcR alleles were associated with protection in a Vax004 vaccine trial (12), suggesting that Fc receptor-mediated activities do contribute to control of HIV-1. Opsonization of virions by complement may also be an important component of viral pathogenesis since CD21 on B cells can bind complement-coated virions and propagate infection of T cells (27), although HIV-1 virions also incorporate host complement inhibitor molecules during virion budding (37). Others have also found that antibody-opsonized HIV-1 without the presence of complement components could enhance HIV-1 infection in vitro (2, 3, 18).
The initial induced HIV-1-specific antibodies do not exhibit traditional neutralizing activity, do not mediate antibody-dependent cellular viral inhibition (ADCVI), do not drive HIV-1 Env escape mutations, and do not impact initial viral load dynamics (42). Thus, a critical question is whether the initial gp41 Env IgG response captures infectious virions, and if so, are a sufficient proportion of virions coated with Env antibody in AHI to potentially mediate an antiviral effect? To address these questions, we have quantified plasma IgG-virion ICs, determined the proportion of virions bound to IgG during AHI, determined the kinetics of the production of acute ICs, and determined the ability of acute HIV-1-purified IgG from AHI to bind infectious virions in vitro. We found that 89.5% of AHI subjects had plasma IgG-virion immune complexes. During acute infection, a median 22.1% of plasma viral RNA was opsonized by IgG (forming IgG-virion immune complexes), and the predominant IgG antibodies coating circulating plasma virions were anti-gp41 antibodies. Thus, circulating plasma virions in AHI are opsonized with antibodies that are of narrow specificity, of limited magnitude, and unable to control the initial spread of HIV-1.
MATERIALS AND METHODS
Ethics statement.
This CHAVI Acute and Chronic Cohorts study was reviewed and approved by the institutional review boards of Duke University Medical Center. All participants provided written informed consent for study participation.
Acute and chronic HIV-1 subjects.
A total of 209 samples (3 to 9 time points per subject) from 38 AHI subjects (plasma donors [ZeptoMetrix Corp. and SeraCare Life Sciences] and acute CHAVI 001 cohorts) and 10 chronic HIV-1 subjects from CHAVI 001 were examined. These cohorts have been previously described (15, 40, 44).
Plasma viral load.
Plasma viral RNA was measured by Quest Diagnostics (Lyndhurst, NJ) (HIV-1 RNA PCR Ultra). Plasma viral RNA for the quantification was also measured by in-house HIV-1 Gag real-time RT-PCR, as previously described (44).
Cells and replication-competent reporter viruses.
The 5.25.EGFP.Luc.M7 (M7-Luc) (provided by N. R. Landau, the Salk Institute for Biological Studies, La Jolla, CA) is a CEMx174 cell clone that was produced by retroviral vector transduction to express CCR5 (CD4 and CXCR4 are expressed naturally) and transfection to contain Tat-responsive luciferase (Luc) and green fluorescence protein (GFP) reporter genes. The cells were grown in RPMI 1640, supplemented with 12% fetal bovine serum (FBS), 50 μg/ml Geneticin (G418), 0.5 μg/ml puromycin, 1% Pen/strep, and 200 μg/ml hygromycin (8). Replication-competent virus stocks from infectious molecular clones (IMC) of Renilla luciferase (LucR) reporter viruses (designated NL-LucR.T2A-Env.ecto) (9) expressing envelope regions from lab-adapted NL4-3 or transmitted/founder viruses (CH040 and WITO) (19) were generated as described previously (9, 14). Briefly, proviral DNA was transfected into 293T cells by Fugene HD (Roche). Working stocks were amplified by passaging virus in human peripheral blood mononuclear cells (PBMCs) (American Red Cross). Virus supernatants were collected every 2 or 3 days and filtered through a 0.45-μm syringe filter, and titers were determined on TZM-bl cells (obtained through the NIH AIDS Research and Reference Reagent Program, Division of AIDS, NIAID, NIH, from John C. Kappes, Xiaoyun Wu, and Tranzyme, Inc.). Wild-type HIV-1 MN was amplified by the H9 cell line (obtained from the NIH AIDS Research and Reference Reagent Program, Division of AIDS, NIAID, NIH, from Robert Gallo).
HIV-1-specific binding antibody assay.
Plasma HIV-1-specific antibodies were measured by a custom HIV-1 binding antibody multiplex assay as previously described (44). HIV-specific Ab isotypes were detected with mouse-anti human IgG (Southern Biotech, Birmingham, AL), conjugated to phycoerythrin, at 4 μg/ml. Antibody measurements are acquired on a Bio-Plex instrument (Bio-Rad, Hercules, CA), and the readout is in mean fluorescent intensity (MFI) or μg/ml equivalents based on a 2F5 MAb (Polymune Scientific, Vienna, Austria) standard curve for gp41 IgG detection (5-PL curve fitting) using 21CFR part 11-compliant software. All assays were run under good clinical laboratory practice (GCLP)-compliant conditions, including tracking of positive controls by Levy-Jennings charts. Positivity criteria for antibody-antigen pairs were predetermined using a set of plasmas from 30 seronegative subjects (mean MFI + standard deviation [SD] of 3).
Quantitative IgG-HIV-1 virion immune complex assay.
IgG–HIV-1 virion (IC) were measured by protein G absorption. Briefly, plasma was centrifuged (10,000 × g) for 10 min to remove cellular debris and filtered in a 1.2-μm filter plate (Pall AcroPrep). The 150-μl filtered samples were loaded on each well in protein G HP MultiTrap 96-well plates (GE Healthcare, Inc.) and incubated 1 h with shaking. After incubation, the plate was centrifuged at 1,500 × g for 3 min. The flowthrough fraction was harvested. After washing to remove nonspecific bound material, the absorbed IgG-virion IC was lysed by 560 μl AVL lysis buffer from the QIAmp viral RNA minikit (Qiagen, Inc.) and was harvested by centrifuging 1,500 × g for 3 min. The viral RNA in the lysis buffer was purified with the QIAmp viral minikit according to the manufacturer's instructions. The viral RNA present in each fraction was measured by HIV-1 Gag real-time RT-PCR. Briefly, the one-step RT-PCR amplification reactions were performed in a MicroAmp optical 96-well plate (Applied Biosystems, Foster City, CA) in a 25-μl reaction mixture containing 1× one-step TaqMan RT-PCR master mix, 900 nM forward and reverse primers, 200 nM probe, and the template RNA in a final volume of 25 μl. Reverse transcription was performed at 48°C for 30 min and was followed by activation of TaqGold at 95°C for 10 min. Subsequently, 40 cycles of amplification were performed at 95°C for 15 s and 60°C for 1 min. Polyclonal HIV immunoglobulin (HIVIG) obtained from the NIH AIDS Research & Reference Reagent Program (ARRRP) and the HIV broadly neutralizing antibody 2G12 MAb (Polymune Scientific, Vienna, Austria) were utilized as positive controls. The negative controls, normal human serum (NHS) (Sigma), and virus-only control were utilized to establish the positivity cutoff. We utilized the mean ± 5 SD of the NHS negative control to calculate the positivity cutoff of 8.6% (NHS mean ± 5 SD).
Plasma IgG purification.
IgG was isolated from plasma using the protein G HP MultiTrap 96-well plates described above (GE Healthcare, Inc.). Briefly, plasma was 2-fold diluted with Tris-buffered saline (TBS) at pH 7.5, and 200 μl of diluted plasma was incubated in the plates for 1 h. Unbound fractions were removed by centrifugation at 1,500 × g for 3 min. Wells were then washed 3 times with 200 μl of TBS. IgG was eluted with 2.5% glacial acetic acid and immediately neutralized with 120 μl of 1 M Tris-HCl, pH 9.0. The eluted IgG fractions were concentrated and desalted using Amicon Ultra centrifugal filters (Millipore) with a 30,000 cutoff at 14,000 × g for 12 min. The IgG was then concentrated to the desired volume and assayed for protein concentration using a NanoDrop 1000 spectrophotometer (Thermo Fisher Scientific).
Protein G capture of virus particles and infectious HIV-1 virions.
Purified plasma IgG or monoclonal IgG was mixed with 1 × 107 RNA copies/ml NL-LucR.T2A viral stock at a final concentration of 10 μg/ml in 300 μl. The IgG and virion IC mixture were prepared in vitro and absorbed by a protein G MultiTrap 96-well plate as described in the measurement of endogenous IgG–HIV-1 virion IC described above. The virions in the flowthrough or captured fraction were measured by viral RNA with HIV-1 Gag real-time RT-PCR, and the 25-μl flowthrough or input was used to infect TZM-bl cells. Infection was measured by a firefly luciferase assay at 48 h postinfection as described previously (39). Briefly, 100 μl supernatant was removed, and 100 μl Britelite (Perkin Elmer) was added to each well. After 2-min incubation, the 150-μl lysis was used to measure HIV-1 replication as expressed as relative luciferase units (RLUs). Each sample was run in triplicate. The percentage of viral particles in the flowthrough or capture fraction was calculated as flowthrough or capture RNA/(flowthrough + capture) × 100%. The percentage of infectivity was calculated as flowthrough infectivity/input infectivity × 100%. The positive controls HIVIG and 2G12 MAb gave virus capture of 66.1 ± 3.7% at 0.83 mg/ml and 30.1 ± 1.4% at 10 μg/ml, respectively. The negative controls, NHS (Sigma), HIV-1− IgG (purified IgG from HIV-1-negative donors [Sigma]), G8 MAb (an IgG monoclonal antibody derived from an Epstein-Barr virus (EBV)-transformed B cell, provided by Kwan-Ki Hwang, Duke University [23]), and virus-only controls were utilized to establish the positivity cutoff. The means ± 5 SD of G8 MAb and virus only are 4.43% and 6.35%, respectively. We utilized the mean ± 5 SD of the NHS-negative control to calculate the positivity cutoff of 8.6% (NHS mean ± 5 SD).
Infectious virus capture assay.
To measure the captured infectious IC, we adopted the Ig-virus capture assay described previously (44). Briefly, microplates (Nunc) were coated overnight at 4°C with mouse monoclonal anti-human IgG (Southern Biotech, Birmingham, AL) at a concentration of 1 μg/ml diluted in PBS. After coating and washing, coated plates were blocked for 2 h with PBS supplemented with 5% goat serum, 5% milk, 0.05% Tween. The indicated concentration of antibodies was mixed with the viral stock containing 5 × 106 viral RNA and then centrifuged 90 min at 2,000 rpm. Then the mixture was centrifuged at 21,000 × g for 45 min at 4°C to remove the virus-free antibody (22), and the pellet was resuspended in the same volume of PBS. A total of 50 μl of the IC mixture was added to each coated well in triple wells for a 90-min incubation. Then, the wells were washed 4 times, and the indicator cell line (M7-luc or TZM-bl) was added. HIV-1 replication was assessed on day 5 after infection for M7-luc and on day 3 for TZM-bl. The infection was measured by the firefly luciferase assay and was expressed as RLU.
Antibody adsorption assay.
A total of 2.4 μg total-plasma-purified IgG was incubated with 0.36 × 106 carboxylated fluorescent beads conjugated with 1.5 μg HIV-1 MN gp41 (ImmunoDiagnostics, Woburn, MA), gp120 protein, or a blank (Luminex Corp, Austin, TX) for 1.5 h. After incubating for 1.5 h, beads were centrifuged for 2 min at 8,000 × g, and the supernatant was harvested. The absorption was repeated another two times. The supernatant was used to make the IgG-virion IC mixture in vitro, and the infectious IgG-virion IC captured by coated anti-human IgG was measured by an M7-luc assay as described above. The HIV-1 anti-gp41 IgG depletion efficiency of absorbed supernatant was confirmed to be removed of HIV-1-specific antibodies by the HIV-1-specific binding antibody assay as described above.
SPR.
Surface plasmon resonance (SPR) binding assays were performed on a BIAcore 3000 (BIAcore, Inc., Piscataway, NJ) instrument at 25°C, and data analyses were performed using BIAevaluation 4.1 software (BIAcore) as previously described (1). HIV-1 MN gp41 and a control protein, HIV-1 p66, were coupled covalently on individual flow cells from 5,000 to 7,000 RU on a BIAcore CM5 sensor chip as described previously (1). IgG samples (100 μg/ml) were injected at 10 μl/min for 2 min and allowed to dissociate in running buffer (PBS [pH 7.4], 0.005% P20) for 10 min. Bound complexes were regenerated by 10 μl glycine-HCl, pH 2.0, at 50 μl/min. Rate constants for HIV-1-specific IgG binding were measured using a 1:1 Langmuir dissociation model (1). The off rate (or dissociation rate) expressed as 1/Kd in seconds is reported.
Single-genome amplification and sequence analysis of the HIV env gene.
Viral RNA from the flowthrough or captured fraction was extracted using the PureLink viral RNA/DNA minikit (Invitrogen, Carlsbad, CA) and reverse transcribed into cDNA using Superscript III (Invitrogen, Carlsbad, CA) and primer 1.R3.B3R 5′-ACTACTTGAAGCACTCAAGGCAAGCTTTATTG-3′ (nucleotides [nt] 9611 to 9639 in HXB2) in a 50-μl volume. Single-genome amplification (SGA) was used to obtain rev-vpu-env cassettes as previously described (19, 21). The first-round PCR was carried out with primer 07for7 5′-CAAATTAYAAAAATTCAAAATTTTCGGGTTTATTACAG-3′ (nt 4875 to 4912 in HXB2) and antisense primer 2.R3.B6R 5′-TGAAGCACTCAAGGCAAGCTTTATTGAGGC-3′ (nt 9608 to 9636 in HXB2); the second-round PCR was carried out with sense primer VIF1 5′-GGGTTTATTACAGGGACAGCAGAG-3′ (nt 4900 to 4923 in HXB2) and antisense primer LOW2C 5′-TGAGGCTTAAGCAGTGGGTTCC-3′ (nt 9591 to 9612 in HXB2). All SGA amplicons were sequenced directly by cycle sequencing and dye terminator methods using an ABI 3730xl genetic analyzer (Applied Biosystems, Foster City, CA). Individual sequences were assembled and edited using the Sequencher program 4.7 (Gene Codes, Ann Arbor, MI). The env sequences were aligned using CLUSTAL W (41), and manual adjustment for optimal alignment was done using MASE (10). A phylogenetic tree was constructed with complete env gene sequences using the neighbor-joining method (38) and the Kimura two-parameter model (20). Highlighter analysis was performed through the LANL database website (http://www.hiv.lanl.gov/content/sequence/HIGHLIGHT/highlighter.html).
Quantity of HIV-1 antibodies in HIV-1 virion-IgG complexes during AHI.
To study the relationship between the concentration of anti-gp41 IgG and the percentage of viral load bound in the plasma of acutely infected patients, we assumed the following dynamics between antibodies and virions: A + V C. Antibodies A reversibly bind to free virions V to form an antibody-virion complex C. Since the binding is reversible, antibodies can dissociate from the complex. We assume that the binding kinetics are much faster than the changes in antibody concentration or viral load over time, leading to the following quasi-steady-state assumption: Kb·AV = Kd·C or C = K·AV, where Kb and Kd denote the binding and dissociation rate constants, respectively, and Kb/Kd = Ke, the (effective) affinity constant of the antibody studied. With VT = V + C defining the total viral load, we obtain:
If more than one antibody binds to a virus, an analogous equilibrium expression can be derived:
where f denotes the total number of antibodies than can simultaneously bind a virion, K is the affinity of an antibody for one gp41 on the virion (i.e., the true affinity), and Ci(t) is the concentration of virions with i antibodies bound at time t.
Statistical analysis.
The statistical significance between the mean values of the endogenous HIV-1 IgG-virion IC for the acute subjects and the chronic subjects was determined by an unpaired two-tailed t test. To determine the correlation between levels of HIV-1 plasma VL and the plasma HIV-1 IgG-virion IC, a linear regression analysis was performed, and the results were expressed as the correlation coefficient (r). A mixed-model analysis was performed to determine the relationship between the levels of HIV-1-specific anti-gp41 IgG and the plasma HIV-1 IgG-virion IC. Differences were considered significant if the P value was ≤0.05. All statistical analyses were performed in SAS v9.2 (SAS Institute, Cary, NC), using CORR, GLM, and MIXED procedures.
RESULTS
Plasma HIV-1 IgG-virion immune ICs during AHI.
We characterized plasma HIV-1 IgG-virion ICs during the initial stages of acute HIV-1 infection from 38 subjects from the CHAVI 001 and US plasma donor cohorts (167 samples, 3 to 9 time points per subject). The plasma donor cohort had frequent sampling for HIV antibody or viral RNA testing within the first 20 days before and after T0 (15, 40, 44), and the CHAVI 001 acute cohort had sampling near the initial VL peak with longitudinal follow-up samples. To measure the level of virions circulating in plasma that are coated in IgG, we utilized a quantitative IgG–HIV-1 virion immune complex assay. HIV-negative NHS and a control monoclonal antibody not specific for HIV-1 were used as negative controls, and polyclonal purified HIV-1 plus IgG (HIVIG) were used in every assay. Shown in Fig. 1 are 6 representative subjects of acute HIV-1 infection from the acute CHAVI 001 cohort that show the rise in the percentage of IgG-coated HIV-1 virion in plasma VL during the initial phase of AHI. The 6 subjects were enrolled in Fiebig stages I to IV according to the classification described by Fiebig et al. (11). In contrast, the plasma viral load declined during this same time period. We found ICs (comprised of the patient IgG and circulating plasma virus, ≥8.63%, the cutoff value, NHS + 5 SD) in 34 of the 38 AHI subjects studied (89.5% frequency of detection). The peak endogenous IgG-virion IC level ranged from 8.6 to 73.9%, (mean, 24.1%; median, 22.1%), indicating that a significant proportion of plasma virions was present in the IgG-bound IC during AHI. In four subjects (10.5%, 4/38), we could not detect IgG-virion ICs, although in the same specimens we could detect a significant quantity of viral RNA (7.24 × 105 to 2.82 × 106 copies/ml). Importantly, there were no significant differences in the viral loads between subjects who had detectable ICs and those that did not (IgG-virion IC-positive [IC+] group: 2.35 ± 2.51 × 106 copies/ml versus IgG-virion IC-negative [IC−] group: 1.62 ± 0.93 × 106 copies/ml) (P = 0.6, Student t test). We also determined whether there was a relationship between the level of immune complexes and plasma virus. There was no correlation between the plasma viral load (RNA copies/ml) and IgG ICs (percentage of IgG-bound plasma viral RNA) in each sample from 38 AHI subjects (r = 0.06, P = 0.41) (Fig. 2), indicating that the measurement of virus complexes is not reflective of overall viral load. Additionally, there was no correlation between the total measured plasma virus (RNA copies/ml) and the quantity of captured virions (RNA copies/ml) (peak response values are shown in Table S1 in the supplemental material).
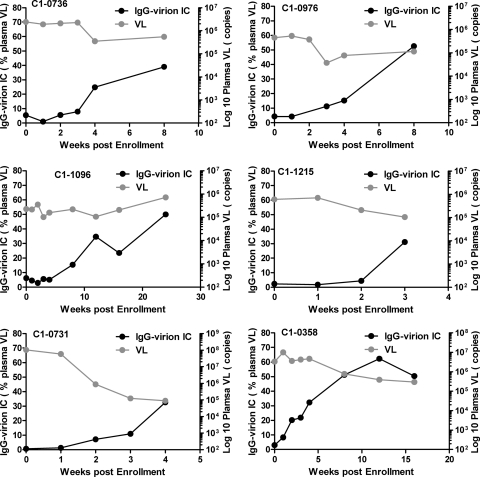
Increasing proportion of acute HIV-1 plasma virions are coated by IgG. Endogenous plasma IgG-virion immune complexes in plasma from 38 AHI subjects were measured by the use of protein G absorption. The IgG-virion immune complexes were detected in the plasma of 34 out of 38 subjects (IgG opsonized a range of 8.6 to 73.89% of plasma HIV-1 VL during AHI). Endogenous IgG-virion IC and plasma viral load for 6 representative subjects (enrolled at Fiebig stages I to IV) are shown.
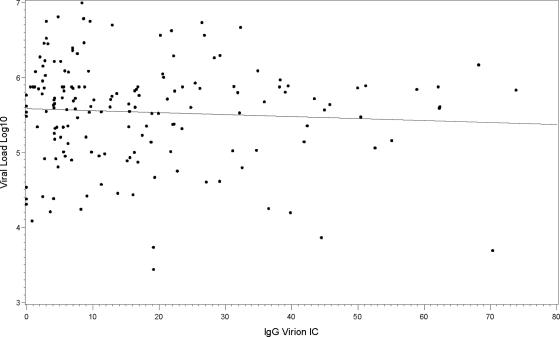
No correlation between HIV-1 plasma viral load and plasma endogenous IgG-virion IC in all samples from AHI. The plasma viral load was plotted against the level of the endogenous plasma IgG-virion IC (r = 0.06, P = 0.41).
Previous studies have demonstrated the presence of virion IC in chronic HIV-1 plasma (7, 29). Using the same assays as those used for AHI, we measured the plasma HIV-1 IgG-virion ICs in plasma from 10 chronically infected subjects. In contrast to AHI subjects, the IgG-virion ICs were detectable in all 39 plasma specimens tested from 10 subjects over a 24- to 60-week period (100% frequency of detection). The level of ICs in chronic plasma ranged from 17.7% to 66.9% with a median and mean of 47.8% and 46.8%, respectively. Unlike AHI, IgG-virion ICs in chronic subjects maintained stable levels over the time period examined. For example, with patient C1-0645, IgG-virion ICs were detectable in all samples from week 0 to week 24 poststudy. The level of ICs ranged from 45.7% to 59.1% over 24 weeks (Fig. 3A). The quantity of circulating virions relative to total viral RNA that were opsonized in IgG in chronic infection were significantly higher than acute infection, compared to plasma samples in Fiebig stages I to VI (P < 0.0001, unpaired two-tailed t test) (Fig. 3B) (11, 26). The absolute values for circulating immune complexes (measured as viral RNA) ranged from 132 to 6.62 × 106 viral RNA copies/ml in AHI (mean = 1.33 ×105) and 164 to 1.37 × 105copies/ml in chronic infection (mean = 1.9 × 104). Among those subjects who were positive for circulating HIV-1 complexes (greater than or equal to 8.6%, n = 37 AHI patients and n = 10 chronic patients), there was not a significant difference (mixed-model analysis of variance [ANOVA]) in the absolute amount of viral RNA in complexes between acute and chronic infection. Thus, although the absolute levels of circulating virions that are opsonized by IgG are similar in acute and chronic infection, the relative proportion of IgG-coated virions are significantly lower in acute infection.
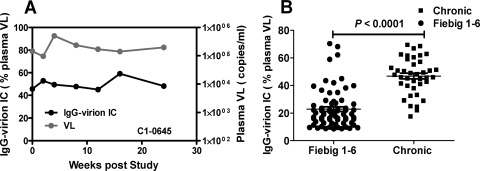
The plasma endogenous IgG-virion IC in chronic subjects. (A) Unlike AHI subjects, chronic subjects maintained a high level of endogenous IgG-virion IC over time. Approximately 50% plasma viral load was captured by IgG in the chronic subject C1-0645 over a 24-week study period. (B) Comparison of the endogenous IgG-virion IC in acute and chronic infection. The value of endogenous plasma IgG-virion IC in 39 samples from chronic infection (10 chronic subjects) compared to 69 samples from acute infection; Fiebig stages I to VI in 34 AHI subjects are shown here. The Fiebig stage was defined as described in reference 11. The samples from plasma donor cohort were assigned to Fiebig stages I to VI if they were less than 30 days post-T0. The line bar represents the mean value (P values, unpaired two-tailed Student t test).
Plasma IgG from AHI and HIV-1 chronically infected subjects capture infectious HIV-1 virions in vitro.
Since circulating plasma HIV-1 virions were opsonized by IgG in vivo, we also asked whether the purified antibody from plasma from the same subjects had the capacity to capture virions in vitro. Thus, in vitro ICs were made using infectious virus and purified AHI plasma IgG. The percentage of virus captured by purified plasma IgG was determined by separation of opsonized virions and nonopsonized virions using protein G columns. Both the percentage of virions bound and the infectious potential of IgG-bound virions were determined.
First, physical virions were measured in both the uncaptured (flowthrough) and the captured fraction (IgG bound) by real-time RT-PCR. The negative control G8 MAb and virus-only controls yielded capture of 3.5% and 2.9%, respectively (Fig. 4A and B). The positive controls included neutralizing polyclonal pooled IgG (HIVIG), the neutralizing antibody 2G12 MAb, and the nonneutralizing anti-gp41 monoclonal antibody 7B2 MAb (gp41 immunodominant region) (32). As shown in Fig. 4, these antibodies captured virions containing 2.77 × 106 to 4.60 × 106 copies of viral RNA at 10 μg/ml which accounted for 65.2%, 44.8%, and 71.2% of the input viral particles for HIVIG, 2G12 MAb, and 7B2 MAb, respectively. 7B2 MAb reflects a specificity of anti-gp41 antibody, which is among the first gp41 antibodies induced in AHI (44) and therefore serves as a good control to characterize the ability of this specificity of antibody to capture virions.
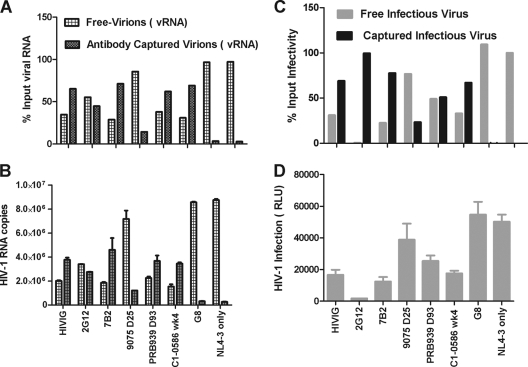
Plasma IgG from HIV-1 infection captures infectious or noninfectious HIV-1 viral particles. IgG-virion IC was prepared in vitro at final concentration of 10 μg/ml IgG, and IgG-virion IC mixture was absorbed by protein G. The viral RNA or infectivity in different fractions was measured by HIV-1 Gag real-time RT-PCR (Fig. 4A and B) or TZM-bl infection (Fig. 4C and D). The error bar represents the standard deviation. The percentage of virions (viral RNA) (Fig. 4A) or infectious viruses in each fraction (Fig. 4C) of the input was calculated as described in Materials and Methods and are shown here. vRNA, viral RNA.
We focused further characterization of plasma IgG capture of HIV-1 on three subjects (9075, PRB939, and C1-0586) that had detectable anti-gp41 IgG and detectable endogenous IgG-virion immune complexes (25.5% [25 days post-T0], 44.5% [93 days post-T0], and 55.3% [4 weeks postenrollment], respectively) and that represented two subjects from acute infection and one subject from chronic infection. Purified plasma IgG (10 μg/ml) from these three subjects were captured from 1.21 × 106 to 3.67 × 106 copies RNA of NL-LucR.T2A viral particles and accounted for 14.4%, 62.1%, and 69.1% of the input viral particles (Fig. 4A and B). Purified plasma IgG from subject PRB939 (93 days post-T0) and subject C1-0586 (4 weeks poststudy) demonstrated a similar ability to capture NL-LucR.T2A as HIVIG and the anti-gp41 monoclonal antibody, 7B2 MAb. The in vitro immune complex results correspond with the in vivo endogenous IgG-virion IC results, in that the ranking of the highest to the lowest quantity of immune complexes was the same: C1-0586 > PRB939 > 9075 (see Table S1 in the supplemental material). These in vitro IC formation data support our findings that significant fractions of circulating plasma virions can be coated with IgG in vivo.
Next, we examined whether IgG ICs contained infectious virions. Due to the inherent difficulties in accurately assessing the infectious nature of virus in patient plasma, either due to a small percentage of virions being infectious (4) or due to limitations in the in vitro assays (33), we determined the capacity of IgG purified from plasma following HIV-1 transmission to capture exogenously added infectious virions. We first tested the amount of infectious virus that could be captured by known neutralizing antibodies with HIV-1 specificities known to mediate ADCC and ADCVI. 7B2 MAb targets the immunodominant region of gp41 and is nonneutralizing in the classic HIV-1 neutralization assays but represents antibodies that could have virus inhibitory potential by other mechanisms (17). We found that 77.6% of the infectious virus was captured by MAb 7B2 (Fig. 4C and D). Similarly, for HIVIG, a polyclonal mixture of pooled IgG known to mediate both neutralization and ADCC, we found that 68.9% of the infectious virus could be captured (Fig. 4C and D). MAb 2G12, a broadly neutralizing anti-HIV-1 human MAb reactive with a high-mannose glycan cluster on the surface of glycoprotein gp120 (6, 46), selectively bound to virtually all infectious virions (Fig. 4). When 2G12 MAb-virion complexes were added to the column, there were no detectable infectious virions in the protein G-absorbed flowthrough as determined by TZM-bl infection; however, 55% of the input viral particles were detected in the flowthrough by measuring viral RNA. We confirmed that 2G12 MAb was not present in the flowthrough fraction (see Fig. S1A in the supplemental material), and the 2G12 flowthrough did not neutralize the infection (see Fig. S1B in the supplemental material). The purified plasma IgG from acute HIV-1 infection captured infectious virions at levels similar to those of MAb 7B2, which targets HIV-1 gp41 Env. Moreover, the rank order of infectious virus capture was similar to the results obtained by the virus capture assays that measured viral RNA: chronic C1-0586 > PRB939 > 9075. Plasma IgG from acute plasma subject PRB939 captured up to 51% infectious virus, similar to the levels captured by anti-gp41 nonneutralizing 7B2 MAb.
Nonselective binding of initial HIV-IgG to circulating plasma HIV-1 virions.
To address the question of whether the initial HIV-specific IgG selectively bound certain subpopulations of viral quasispecies, we obtained full-length HIV env gene sequences by SGA from the captured virions and compared the sequences to those of the uncaptured virions. Plasma samples collected 12 weeks postenrollment (the peak of the endogenous plasma IC) from subject C1-0358 were separated by the protein G column, and the env gene sequences from the captured and uncaptured (flowthrough) fractions were compared (23 and 20 env sequences from the uncaptured and the captured fractions, respectively). There was no distinction in virus sequence populations between the captured and uncaptured viruses by the phylogenetic tree and the highlighter plots (see Fig. S2A and B in the supplemental material). In some cases, the env sequences from the captured fraction were identical to the sequences from the flowthrough fraction. These results indicate that there are no genetic differences in the envelope sequences between the uncaptured (flowthrough) and IgG-captured viruses.
Anti-gp41 IgG is a component of plasma IgG-virion ICs.
Since the earliest detectable anti-Env IgG response after HIV-1 transmission targets gp41 and occurs close to the time of the first detectable endogenous HIV-1 IgG-virion IC, we investigated if this antibody specificity was a component of the initial IC (44). We found that the endogenous IgG-virion ICs were detected at the same time or later than the plasma anti-gp41 IgG in 76% (19/25) of IgG-virion IC+ subjects, and the two measurements had a corresponding increase in some subjects (Fig. 5A). The statuses of another 9 IgG-virion IC+ subjects were not able to be determined because the first available time point samples were both anti-gp41 antibody and IgG-virion IC positive. It is important to note that the presence of plasma IgG-virion IC arose before the appearance of anti-gp41 IgG in 24% of IgG-virion IC+ subjects (6/25). This indicates that natural cross-reactive antibodies to antigens present on HIV-1 virions or antibodies to host molecules that are incorporated in HIV-1 virions are likely a component of the specificities that comprise circulating immune complexes during AHI.
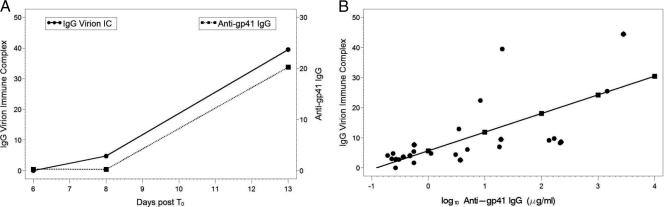
Circulating plasma IgG-virion immune complexes significantly correlate with the concentrations of anti-gp41 Env IgG during AHI. (A) IgG-virion IC and anti-gp41 IgG from one typical plasma donor subject (patient 9012) is presented here. (B) Levels of log10 anti-gp41 IgG (μg/ml) in plasma significantly correlate with the level of plasma IgG-HIV-1 virion IC (n = 8) (t = 4.88, P = 0.0001). Every log10 increase in anti-gp41 IgG correlates to a 6.2% increase in IgG-virion IC. Each color represents one individual subject.
Furthermore, we found that the complement component, C3d, also opsonized plasma virions in acute infection (see Fig. S3 in the supplemental material). For those samples collected within 30 days post-T0 (except PRB939-09, 93 days post-T0) and that had undetectable anti-gp120 plasma IgG in the study period (n = 8 subjects), we examined the correlation of gp41-specific antibodies with virion-IgG complexes. In these subjects, there was a significant positive correlation between anti-gp41 IgG (μg/ml) in plasma and endogenous IC (t = 4.88, P = 0.0001) (Fig. 5B). Furthermore, to determine whether the quality of anti-gp41 IgG was also associated with endogenous IgG-virion IC, we determined the dissociation rates (Kd) as one measure of avidity against Env gp41 of purified plasma IgG over time by surface plasmon resonance (SPR). In 4 AHI subjects, the increasing 1/Kd values suggested an increase in the avidity of anti-gp41 IgG to Env gp41 antigen and paralleled the plasma endogenous IgG-virion IC (Fig. 6A). IgG dissociation rates against HIV-1 MN gp41 were significantly correlated with the plasma endogenous IgG-virion IC (r2 = 0.76, P < 0.01) (Fig. 6B; see also Fig. S4 in the supplemental material). This indicates that the quality (as measured by the off rate) of HIV-1-specific IgG may play a role in the ability of gp41-specific IgG antibodies to opsonize virions. These data strongly suggest that HIV-1-specific anti-gp41 IgG is a component of the plasma IgG-virion IC in vivo.
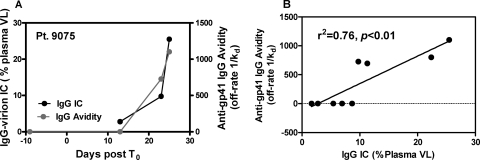
Endogenous plasma IgG-virion IC correlates with the dissociation rates (avidity maturation) of plasma anti-gp41 IgG. (A) The anti-gp41 IgG avidity (off rate,1/Kd in seconds) and the endogenous plasma IgG-virion IC are shown for a representative subject during acute infection. (B) Levels of IgG-virion IC and the anti-gp41 IgG dissociation rates significantly correlate (n = 4) (r2 = 0.76, P < 0.01). Dissociation rates (s−1) as a measure of avidity were calculated as described in Materials and Methods.
To further address the specificity of antibodies in the endogenous IgG-virion ICs, we measured the ability of purified plasma IgG from selected subjects, where endogenous IgG-virion ICs appeared at the same time or later than anti-gp41 IgG, to bind infectious virions over time. In subject PRB939, the purified plasma IgG from the later plasma time points (day 13 and day 93 post-T0) captured infectious lab-adapted HIV-1 NL-LucR.T2A, whereas the earlier sample obtained day 11 post-T0 that was negative for anti-gp41 IgG did not capture virus. In this subject, the levels of captured infectious NL-LucR.T2A corresponded with the increased levels of anti-gp41 IgG in plasma (Fig. 7A), and anti-gp41 was the only detectable specificity of antibody present in the plasma at this time point. We also assessed the ability of gp41 antibodies to capture virions expressing early transmitted/founder Env (19). The purified IgG from subject PRB939 (day 93 post-T0) captured NL-LucR.T2A-WITO at a level equal to an about 1:1,000 dilution of plasma (Fig. 7B). Similar results were obtained with another transmitted/founder virus (CH040) (not shown). The capture of HIV-1 NL-LucR.T2A virions by purified plasma IgG from subject PRB939 was also confirmed by measuring HIV-1 viral RNA (3.4 ×104 RNA copies/μg purified plasma IgG).
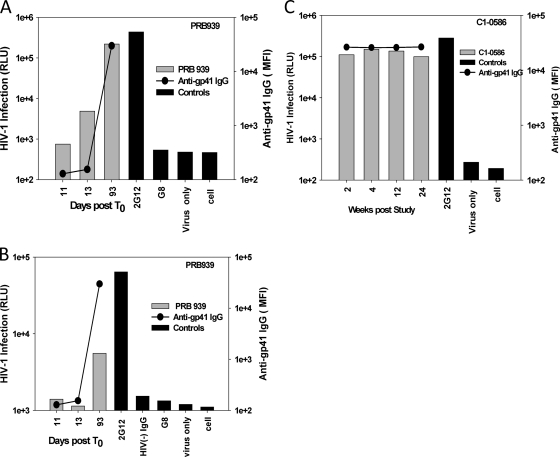
Purified plasma IgG from AHI (A, B) or chronic (C) subjects captures infectious HIV-1. A total of 50 μl IC mixture containing plasma IgG at 10 μg/ml and 5 × 106 RNA copies viral particles was added to each mouse anti-human IgG-coated well after incubation in vitro for 1.5 h. The infection of captured IgG IC was measured by multiple-round M7-luc assay for NL-LucR.T2A at 5 days postinfection (A and C) or by TZM-bl at 3 days postinfection for NL-LucR.T2A-WITO (B). The infectivity was measured by firefly luciferase assay and expressed as relative luciferase units (RLU). The gray bar represents the positive or negative controls. The gray bar represents the clinical samples. The levels of anti-gp41 IgG of plasma samples (solid line) were plotted together with the in vitro capture. The cutoff values were 3× background. All runs were performed in triplicate.
Unlike plasma from AHI, plasma from chronic infection had persistently high levels of endogenous IgG-virion ICs (Fig. 3) and anti-gp41-specific IgG. To determine the amount of infectious virus capture by antibodies in chronic infection, IgG was purified from a series of time points (2 to 24 weeks poststudy) from patient C1-0586 and assessed for the ability to capture infectious HIV. The level of HIV infection ranged from 9.9 × 104 to 1.5 × 105 RLU (Fig. 7C), and the kinetics of the appearance of antibodies capable of binding to virions were similar to those of the plasma IgG-virion ICs (Fig. 7C).
In order to further confirm that anti-gp41 IgG in plasma was responsible for the formation of HIV-1 virion ICs seen in vivo and in vitro in AHI, the anti-gp41 IgG in the purified polyclonal IgG of subjects PRB939 (day 93 post-T0) and 9075 (day 25 post-T0) were depleted first by anti-gp41 protein-conjugated microspheres, before detection of IgG-virion immune complexes. Depletion of gp41- or gp120-specific antibodies was confirmed by a quantitative HIV-1 binding antibody assay (>99% depletion by gp41-conjugated beads and 90% depletion by gp120-conjugated beads, respectively). For AHI 9075 and PRB939, after anti-gp41 antibody depletion by gp41-conjugated beads, the captured infectious NL-LucR.T2A was decreased by 96% and 66.7%, respectively, compared to blank bead control; however, the depleted IgG by gp120-conjugated beads reduced the captured infection only by 30% and 19%, respectively (Fig. 8). These results demonstrate that the initial anti-gp41 IgG elicited by HIV-1 transmission was the predominant antibody coating HIV-1 virions. In contrast, the total purified IgG from chronic patient C1-0586 (4 weeks poststudy) captured virions that were competed out predominantly by gp120 Env and not gp41 protein (Fig. 8). The depletion by gp120-conjugated beads resulted in a 77.5% decrease in the captured HIV-1 infection, but the depletion by gp41 beads demonstrated only a minor 5.3% decline in infection (Fig. 8). Similar results were found in plasma IgG depletion by HIV-1 Env-conjugated beads in chronic subject C1-0645. The infection of wild-type HIV-1 MN captured by the purified IgG of C1-0586 week 4 declined 94% after absorbed by HIV-1 MN Env gp120-conjugated beads; however, only a 19% decrease in the infection was seen after gp41-conjugated bead depletion. These results show that anti-gp120 but not anti-gp41 antibodies comprise a majority of the antibodies in virion-IgG immune complexes in these two subjects with chronic HIV-1 infection. In contrast, gp41 antibodies are the primary mediators of IC formation during AHI.
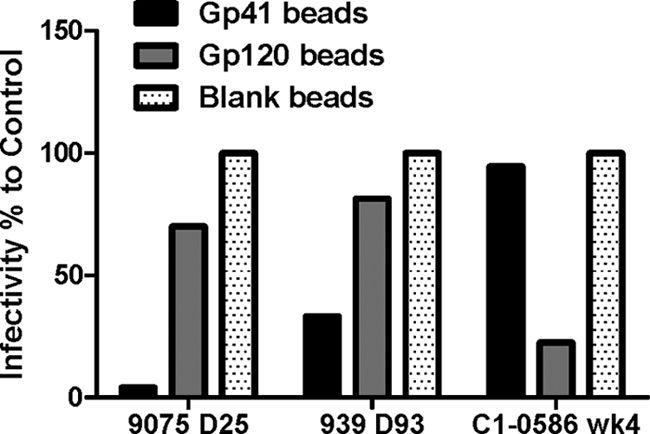
Different specificities of antibodies in immune complexes in acute and chronic infection. Purified plasma IgG from AHI subjects 9075 (day 25 post-T0) and PRB939 (day 93 post-T0) or chronic subject C1-0586 (week 4 poststudy) were absorbed by Env gp41 or Env gp120-conjugated, or blank microsphere beads. Then the absorbed supernatant was used to prepare IgG-virion IC mixture in vitro. The infectious IC was captured by plates coated with anti-human IgG antibody and was measured for infection in the M7-luc infection assay as described above. The percentage of infection compared to the control blank bead is shown here. The results represent at least 3 experiments.
Quantity of IgG bound to virions in AHI.
We measured the quantity of circulating virions captured by IgG antibodies in acute infection and identified that these IgG antibodies are specific for gp41. This raised the question of how many anti-gp41 antibodies coat virions during acute infection. As described in Materials and Methods, we derived some simple expressions for the equilibrium fraction of virions expected to be in antibody-virion complexes when antibody is present at concentration A(t) and has effective affinity K for gp41 on the virion. The analysis was restricted to those 8 plasma donor subjects that had endogenous IgG-virion ICs at the same time or later than anti-gp41 IgG. Figure 9 shows the data and the best fit predicted by equation 1 in Materials and Methods. The corresponding estimated affinity constants K are shown in Table S2 in the supplemental material. We also fitted equation 2 in Materials and Methods to the data to allow for multivalent binding, where HIV-1 was treated as an f-valent particle (Fig. 9; see also Table S2). In addition to f = 1, we examined two different values of f, f = 14, which corresponds to the average number of HIV envelope (Env) glycoprotein spikes on a virion, and f = 35, which corresponds to the maximum number of Env spikes (47). This approach did not improve the results significantly. As can be seen from Fig. 9, the fits with the assumption of multivalent binding do not differ from the results obtained by assuming only univalent binding. This, as well as the approximate linear nature of the curves in Fig. 9, suggests that most virions are bound by only one IgG antibody. This can also be shown formally using equation 2 and the estimated affinity constants given in Table S2.
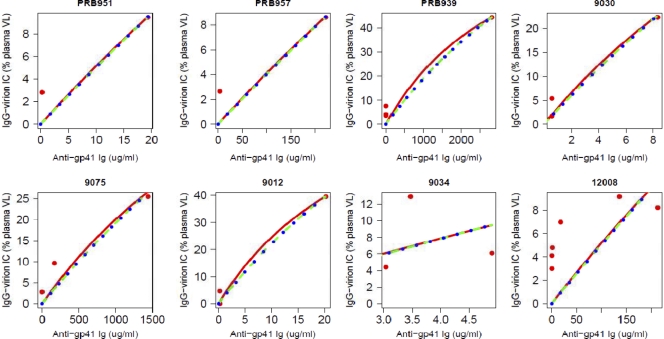
Percentage of virions bound in complexes at various antibody concentrations for 8 different patients. Red lines show the best fits to patient data for a model with univalent binding (red dots), given by equation 1. Blue and green lines assume HIV has binding sites with f values of 14 and 35, respectively. Estimates for the affinity constant K are given in Table S2 in the supplemental material. The aberrant fit of the model to the data from patient 12008 could be explained by an extension of the model that assumes that two different strains of HIV-1 with different affinities for anti-gp41 IgG are present in this patient (results not shown).
DISCUSSION
In this study we have shown that a median of 22% (range of 8.6% to 73.9%) of circulating plasma virions were coated by IgG in approximately 90% of AHI subjects. In 76% of IgG-virion IC+ AHI subjects, the initial HIV-1-specific anti-gp41 IgG elicited by HIV-1 infection accounted for the formation of IgG-virion ICs in vivo and was also able to capture infectious virions in vitro. However, in some acute HIV-1 subjects the differential kinetics of free anti-gp41 antibodies and immune complexes indicate that other antibodies are also likely to be involved in forming a minority of AHI ICs. In contrast to AHI, the plasma IgG-virion IC presented significantly higher levels in chronic plasma. Whereas gp41 antibodies predominated in AHI ICs, gp120 antibodies opsonize virions in plasma during chronic HIV-1 infection.
The first free plasma antibodies in AHI are directed to gp41 Env at 13 days post-T0 and are followed by Env gp120-specific antibodies at 1 month post-T0 (44). The initial HIV-1-elicited antibodies are nonneutralizing and ineffective in controlling virus replication during AHI. We have quantified the amount of circulating plasma virions that are opsonized in AHI (median of 22%). We have also demonstrated that there is no selection for particular HIV virions in subject C1-0358 (as determined by SGA). Furthermore, we identified that anti-gp41 antibodies are the predominant IgG antibody specificity comprising these initial virus complexes. However, mathematical modeling of the antibody-virion complexes suggests that a small number of antibodies are coating each virion compared to the number of potential binding sites on a virion.
One of the mechanisms whereby the host defends against incoming pathogens is to coat the pathogen with antibodies, forming immune complexes that opsonize and mediate immune clearance of the invading pathogen. Clearly, the initial antibodies in AHI do not clear HIV-1 virions after transmission. Whether the first antibodies could protect if present in sufficient quantities at the time of transmission is a key question. Since we and others have detected no neutralizing activity of AHI gp41 antibodies, if most virions could be opsonized, the anti-HIV-1 activity would have to be via nonneutralizing mechanisms, such as virion-inhibited movement across mucosal surfaces, antibody-mediated phagocytosis, or antibody-mediated cellular cytotoxicity (25). The results from the RV144 HIV-1 vaccine trial in Thailand have raised the hypothesis that virus inhibitory antibodies, other than traditional neutralizing antibodies, might provide some protection, especially in low-risk populations (36).
Our studies of immune complexes circulating in plasma during AHI are consistent with our in vitro results, wherein purified plasma IgG from selected patients captured up to 76% of infectious viruses. However, the IgG-bound virions likely represent a fraction of total circulating infectious virions in vivo. This leads to the question of whether the initial antibody response that forms ICs is ineffectual in controlling viral load because it fails to capture all infectious virions or because the initial nonneutralizing antibodies simply bind nonfunctional Env but do not bind functional trimers on the virion surface. Alternatively, the initial opsonization of virions may be ineffectual because they serve to promote infection by trafficking antibody-coated virions to lymph nodes where viral replication can be amplified. In acute simian immunodeficiency virus (SIV) infection, the ratio of infectious virions to total virions is highest in the ramp-up stage of plasma viremia (24, 45), suggesting that there might be virion or virion-associated factors that contribute to early SIV infectivity. Whether a similar phenomenon is present in HIV-1 AHI is not known. However, all evidence to date suggests that the initial antibody response to gp41 is not a factor in the initial control of viremia. Further work on the dynamics of infectious virions in acute HIV-1 is needed to understand how observations of acute SIV infection relate to acute HIV-1 infection.
Other studies have examined antibody-antigen complexes in plasma from subjects infected with HIV-1 (35) but have focused on HIV-1 antigens and not on whole-virion binding to antibody. We show in this report that the initial ICs in AHI were comprised primarily of gp41 IgG and that gp120 antibodies were not present in immune complexes during early AHI. Importantly, anti-gp120 antibodies are not found circulating in plasma during this time. The presence of gp41-specific antibodies in immune complexes in AHI indicates that gp41 is exposed on circulating virions in vivo. Thus, during AHI, the presence of a nonfunctional envelope on circulating virions could be the predominant antigen that helps to drive the initial nonneutralizing antibody response.
Several studies have characterized the ability of anti-Env monoclonal antibodies to capture both virions and infectious virions (5, 16, 30, 31, 34). Burrer et al. found that gp41 Env antibodies were responsible for virus capture in purified polyclonal plasma IgG (5). Poignard et al. demonstrated that the envelope expressed on HIV-1 virions is heterogeneous, such that both functional and nonfunctional envelope molecules are present (34). Thus, virus capture can be mediated by binding to the nonfunctional envelope spikes, leaving the infectious envelope spikes available for the measurement of infectious virus as we showed in this current study. Consistent with this earlier work (5, 28), we also found that the efficiency of virus capture by purified polyclonal plasma IgG did not correlate with the neutralizing activity, i.e., plasma that had no neutralizing activity robustly captured virions.
Like the prototypic nonneutralizing gp41 immunodominant region MAb, 7B2 (32), AHI anti-gp41 plasma IgG captured both infectious and noninfectious virions. This is likely due to the presence of nonfunctional Env protein on the surface of virions in the form of gp120/gp41 monomers or gp41 stumps (28). Anti-gp41 IgG can easily bind to gp41 stumps on the surfaces of infectious or noninfectious virions and may explain why the high percentage of plasma VL existed as circulating IgG-virion IC. Unlike anti-gp41 IgG and HIVIG, 2G12 MAb selectively captured infectious HIV-1, indicating that 2G12 MAb is selectively targeting the native functional Env spike that is presented on the surface of infectious virions.
In summary, circulating plasma IgG-virion ICs were frequently detected during AHI, accounted for a fraction of circulating virions in most subjects examined, and were comprised of Env gp41-specific antibodies. These IgG gp41 virion-binding antibodies either arise too late in the course of infection to control viremia, opsonize too few virions to mediate an antiviral effect, or alternatively may contribute to virus replication.
ACKNOWLEDGMENTS
This work was supported by the National Institutes of Health (NIH/NIAID/DAIDS) Center for HIV/AIDS Vaccine Immunology Grant (U01 AI067854). The funders had no role in study design, data collection and analysis, decision to publish, or preparation of the manuscript.
We are indebted to the CHAVI 001 clinical teams and patients for their numerous contributions that were central to this study. We also thank Kelly Soderberg and Jennifer Kirchherr for program support, Shelley Stewart, Judith T. Lucas, and Vicki C. Ashley for technical assistance, Hua-Xin Liao for HIV-1 gp140 envelope proteins, Cristine Cooper, SCHARP, for statistical support, and Michael Frank, Xiaoying Shen, Robert C. Schutte, and Genevieve Fouda for helpful discussions.
Footnotes
†Supplemental material for this article may be found at http://jvi.asm.org/.
Published ahead of print on 24 August 2011.
REFERENCES
Articles from Journal of Virology are provided here courtesy of American Society for Microbiology (ASM)
Full text links
Read article at publisher's site: https://doi.org/10.1128/jvi.05601-11
Read article for free, from open access legal sources, via Unpaywall:
https://europepmc.org/articles/pmc3194959?pdf=render
Citations & impact
Impact metrics
Article citations
Viral vector delivered immunogen focuses HIV-1 antibody specificity and increases durability of the circulating antibody recall response.
PLoS Pathog, 19(5):e1011359, 31 May 2023
Cited by: 1 article | PMID: 37256916 | PMCID: PMC10284421
Lower SARS-CoV-2-specific humoral immunity in people living with HIV-1 recovered from nonhospitalized COVID-19.
JCI Insight, 7(21):e158402, 08 Nov 2022
Cited by: 8 articles | PMID: 36136590 | PMCID: PMC9675463
Polyclonal Broadly Neutralizing Antibody Activity Characterized by CD4 Binding Site and V3-Glycan Antibodies in a Subset of HIV-1 Virus Controllers.
Front Immunol, 12:670561, 23 Dec 2021
Cited by: 3 articles | PMID: 35003053 | PMCID: PMC8733328
Validation of a Triplex Pharmacokinetic Assay for Simultaneous Quantitation of HIV-1 Broadly Neutralizing Antibodies PGT121, PGDM1400, and VRC07-523-LS.
Front Immunol, 12:709994, 24 Aug 2021
Cited by: 7 articles | PMID: 34504492 | PMCID: PMC8422903
A MUC16 IgG Binding Activity Selects for a Restricted Subset of IgG Enriched for Certain Simian Immunodeficiency Virus Epitope Specificities.
J Virol, 94(5):e01246-19, 14 Feb 2020
Cited by: 4 articles | PMID: 31776284 | PMCID: PMC7022352
Go to all (41) article citations
Data
Data behind the article
This data has been text mined from the article, or deposited into data resources.
BioStudies: supplemental material and supporting data
Similar Articles
To arrive at the top five similar articles we use a word-weighted algorithm to compare words from the Title and Abstract of each citation.
Antigenic properties of the HIV envelope on virions in solution.
J Virol, 88(3):1795-1808, 27 Nov 2013
Cited by: 37 articles | PMID: 24284318 | PMCID: PMC3911592
Concurrent Exposure of Neutralizing and Non-neutralizing Epitopes on a Single HIV-1 Envelope Structure.
Front Immunol, 10:1512, 05 Jul 2019
Cited by: 6 articles | PMID: 31338095 | PMCID: PMC6628914
Initial B-cell responses to transmitted human immunodeficiency virus type 1: virion-binding immunoglobulin M (IgM) and IgG antibodies followed by plasma anti-gp41 antibodies with ineffective control of initial viremia.
J Virol, 82(24):12449-12463, 08 Oct 2008
Cited by: 457 articles | PMID: 18842730 | PMCID: PMC2593361
Relevance of the antibody response against human immunodeficiency virus type 1 envelope to vaccine design.
Immunol Lett, 57(1-3):105-112, 01 Jun 1997
Cited by: 35 articles | PMID: 9232434
Review
Funding
Funders who supported this work.
NIAID NIH HHS (3)
Grant ID: U01 AI067854
Grant ID: P30 AI027767
Grant ID: P30 AI050410
NIH HHS (1)
Grant ID: R01 OD011095