Abstract
Free full text

Advances in Liver Cancer Antibody Therapies: A Focus on Glypican-3 and Mesothelin
Abstract
Liver cancer is one of the most common malignancies worldwide. Hepatocellular carcinoma (HCC) and cholangiocarcinoma (CCA) are the two most common primary liver cancers, yet there have been no significant advances in effective therapeutics. There is an urgent need to identify molecular targets for the development of novel therapeutic approaches. In this review, glypican-3 (GPC3) and mesothelin are discussed, with a focus on their potential as targets for antibody therapy in liver cancer. GPC3 and mesothelin are glycosylphosphatidylinositol-anchored proteins present on the cell surface. They are attractive candidates for liver cancer therapy given that GPC3 and mesothelin show high expression in HCC and CCA, respectively. Antibody drugs targeting GPC3 or mesothelin have shown anti-cancer activity in mice. Humanized or chimeric IgG molecules based on first-generation murine monoclonal antibodies against these antigens are being evaluated in clinical studies. Recently, fully human monoclonal antibodies against GPC3 and mesothelin have been isolated by antibody phage display technology that may provide opportunities for novel cancer therapy.
1. Introduction
Liver cancer is the fifth most common malignant cancer worldwide. According to the American Cancer Society (www.cancer.org), hepatocellular carcinoma (HCC) accounts for approximately 75% of liver cancer cases. Cholangiocarcinoma (CCA) is the second most common primary liver malignant tumor arising from cholangiocytes, and CCA accounts for approximately 10% of liver cancer cases. In 2010, 24,120 new cases of liver cancer were found and 18,910 patients died in the United States. Surgery is the standard treatment for liver cancer. Liver cancer does not respond to most chemotherapy drugs and there is an urgent need to develop new drugs with different mechanisms of action. Monoclonal antibody (mAb) therapy represents a new promising approach but remains a challenge mainly due to a lack of tumor-specific targets. Approximately 40 trials of antibody therapies for advanced HCC have been registered on the ClinicalTrials.gov website. The mAbs (and their antigens) include bevacizumab/Avastin® (vascular endothelial growth factor A [VEGF-A]), IMC-1121B (VEGF receptor 2), cetuximab/Erbitux® (epidermal growth factor receptor), cixutumumab (insulin-like growth factor 1 receptor [IGF-1R]), AVE1642 (IGF-1R), BIIB022 (IGF-1R), MEDI-575 (platelet-derived growth factor receptor), CT-011 (Programmed Death-1), CP 675,206/tremelimumab (cytotoxic T Lymphocyte antigen 4), RO5323441 (placenta growth factor or PGF), and HGS1012/mapatumumab (TRAIL-R1). GC33 (glypican-3[GPC3]) is the only mAb targeting a HCC-specific tumor antigen. No mAb specific for CCA is being evaluated in clinical studies.
GPC3 is a cell surface protein that is highly expressed in HCC; therefore, it has been suggested as an attractive candidate for liver tumor-specific therapy [1]. The GPC3 gene encodes a 70 kDa precursor core protein, which can be cleaved by furin to generate a 40 kDa amino (N)-terminal protein and a 30 kDa membrane-bound carboxyl (C)-terminal protein. There are two heparan sulphate (HS) glycan chains attached to the C-terminus (Figure 1). The GPC3 protein is attached to the cell membrane by a glycosylphosphatidylinositol (GPI) anchor.
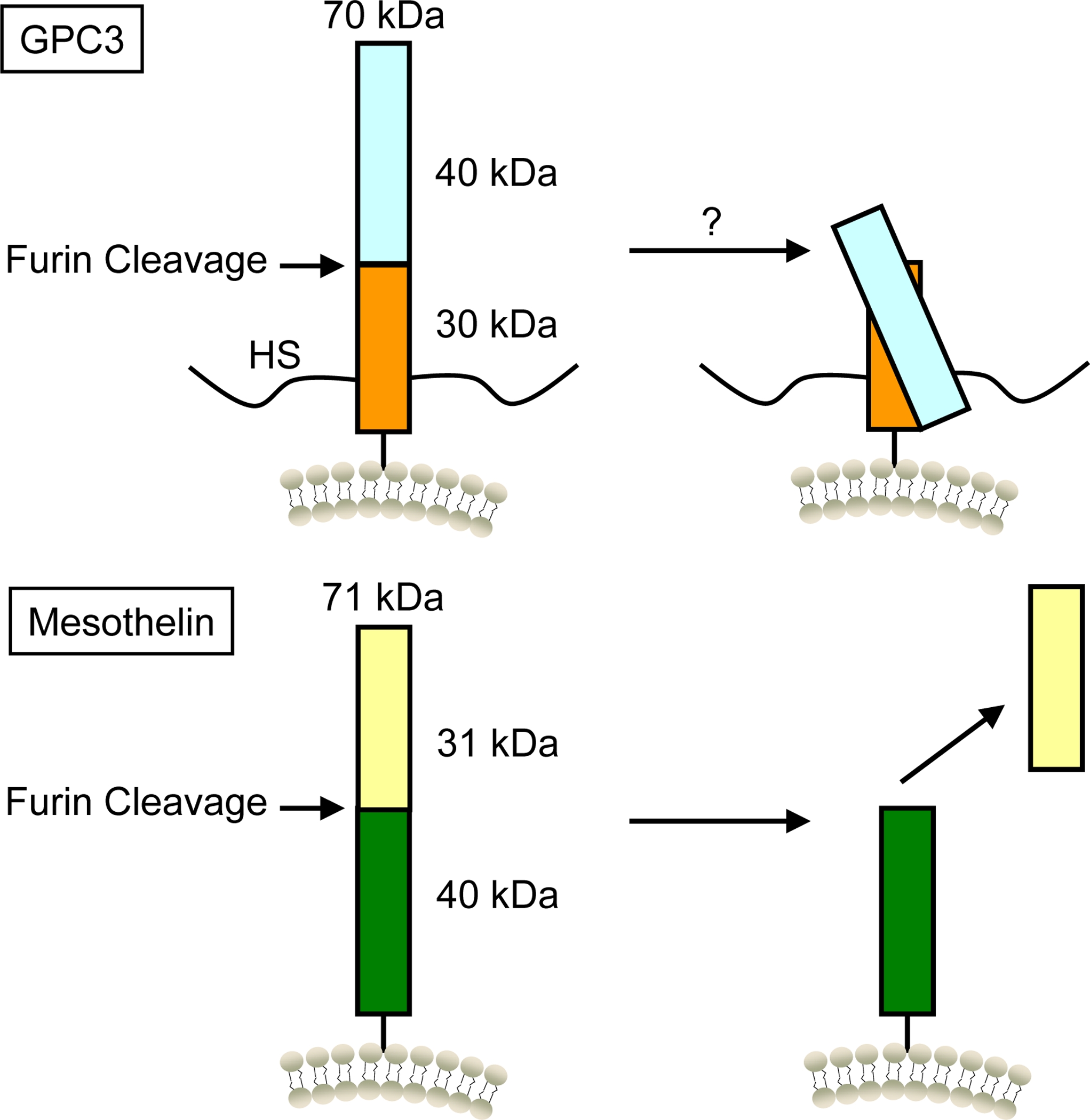
Products of the glypican-3 (GPC3) and mesothelin (MSLN) genes. The primary product of the GPC3 gene is a 70 kDa glycoprotein with two HS glycan chains. The N-terminus and C-terminus of GPC3 may be linked by intramolecular disulfide bonds after potential furin cleavage. The MSLN gene is a 71 kDa precursor protein. This protein is cleaved by furin to release its 31 kDa N-terminal megakaryocyte potentiating factor (MPF) and is displayed as mature mesothelin on the cell surface.
The mesothelin (MSLN) gene encodes a precursor protein of 71 kDa that is processed to a 31 kDa released protein called megakaryocyte potentiating factor (MPF) [2] and a 40 kDa fragment, mesothelin [3], that is attached to the cell membrane by a GPI anchor (Figure 1). Mesothelin is highly expressed in mesothelioma and several other human cancers, including pancreatic adenocarcinomas, ovarian cancers and lung adenocarcinomas [4]. It has been suggested that mesothelin is highly expressed in CCA tissues [5, 6]. My laboratory recently analyzed the mesothelin protein in CCA by immunohistochemistry (IHC), Western blot, and flow cytometry, and found that SS1P, a recombinant anti-mesothelin immunotoxin currently being evaluated in clinical trials for mesothelioma, had remarkable single agent activity against CCA [6]. This finding indicates that mesothelin may be a therapeutic candidate in CCA and SS1P may be a novel agent for targeted therapy of CCA.
This review focuses on GPC3 and mesothelin as potential targets in primary liver cancer and summarizes the available mAbs currently used in translational research as well as ongoing clinical studies.
2. Discovery of Glypican-3 (GPC3)
GPC3 was first identified as the product of a gene designated OCI-5 in 1988 [7]. The OCI-5 gene was later named GPC3 based on its homology with other members of the glypican family. The GPC3 gene is located on human X chromosome (Xq26) where the most common gene (Isoform 2, GenBank Accession No.: NP_004475) encodes a 70 kDa core protein with 580 amino acids. Three variants have been identified in GenBank that encode alternatively spliced forms termed Isoform 1 (NP_001158089), Isoform 3 (NP_001158090) and Isoform 4 (NP_001158091) [1]. The distribution and functional significance of these GPC3 isoforms have not been reported. The protein core of GPC3 consists of two subunits, where the N-terminal subunit is 40 kDa and the C-terminal subunit is 30 kDa. Based on the GPC3 protein sequence, we predicted serine 560 as a cleavage site in GPC3 for GPI anchorage [1]. Furin cleavage between Arg358 and Ser359 is important in GPC3’s modulation of cell survival and Wnt signalling in zebrafish [8], but it is not important in HCC cell growth [9]. Like other members in the glypican family, GPC3 has 14 conserved cysteine residues, which may form intramolecular disulphide linkages to link the N terminus and C-terminus, even after furin cleavage [8]. This hypothesis is also supported by enzyme-linked immunosorbent assay (ELISA) analysis of serum GPC3 using the mAbs specific for the N- [10] or C-terminus [11]. The sequence R355-Q356-Y357-R358 in GPC3 fits the convertase recognition motif. While experimental results suggest that furin is involved in GPC3 processing [8], the efficiency of furin cleavage is not fully evaluated in tumors. We postulate that the furin cleavage in GPC3 may not be highly efficient, based on an early study on furin cleavage specificity by Wells and colleagues [12]. Y357 at the P2 position is not a common residue (K, R or P) in the convertase recognition motif. Therefore, it is possible that the N-terminus and C-terminus remain together due to insufficient furin cleavage in tumors. Our Western blot analysis shows the presence of dominant full-length GPC3 proteins, indicating poor furin cleavage [13]. Future studies are necessary to determine the GPC3 cleavage efficiency of furin in HCC and other GPC3-expressing tumors in addition to and characterizing the biochemical structure of serum GPC3. Up to now, the three-dimensional (3D) structure of GPC3 or any other glypican has not been resolved.
3. Functional mechanisms of GPC3
Normal biologic functions of GPC3 have been studied in humans and GPC3 knockout mice. Mutations in GPC3 result in Simpson-Golabi-Behmel syndrome (SGBS), an X-linked condition characterised by pre- and postnatal overgrowth [14]. GPC3-deficient mice exhibit the clinical hallmarks of SGBS patients [15]. However, the roles of GPC3 in tumor pathogenesis remain elusive. In general, HS proteoglycans (HSPGs) may act as co-receptors or storage sites for growth factors. It has been suggested that GPC3 is involved in cell proliferation and survival due to its interaction with IGF-2. However, biochemical and genetic studies [15–19], with the exception of one [20], have shown that GPC3 does not regulate IGF signalling. GPC3 knockout mice exhibit alterations in Wnt signalling. GPC3 can form a complex with Wnt molecules and promote the growth of HCC by stimulating canonical Wnt signalling [21]. Although GPC3 can form a complex with Wnt, but interestingly, the HS chains are not essential for the activation of Wnt signalling. These results indicate that GPC3 and Wnts may be part of a glypican-Wnt/growth factor complex where other proteins may be involved as co-receptors for either GPC3 or Wnts. It has also been established in genetic experiments that GPC3 regulates developmental growth by interacting with hedgehog (Hh) signalling [22, 23]. In addition, the HS chains may interact with other basic growth factors for cell growth. There is evidence that the HS chains can interact with with fibroblast growth factor-2 (FGF-2) and modulate growth factor activity [16]. An interaction between GPC3 and fibroblast growth factor (FGF-2) has also been found in HCC cells [24]. Roberts’ group at the Mayo Clinic investigated the role of sulfatase (SULF2), an enzyme with 6-O-desulfatase activity on HSPGs, in HCC pathogenesis. They found that overexpression of SULF2 was associated with increased expression of GPC3 and binding of GPC3 to FGF2 [25]. They also showed how the effects of SULF2 can upregulate GPC3 expression and tumor growth in nude mouse xenografts. Interestingly, they showed that GPC3 stimulated the Wnt/β-catenin signalling pathway [26]. SULF2 increased the cell surface expression of GPC3 and Wnt3a, and stabilized β-catenin in HCC cells. Moreover, nude mouse xenografts established from SULF2-overexpressing HCC cells showed enhanced GPC3, Wnt3a, and β-catenin expression levels. These results indicate that SULF2 may be part of the glypican-Wnt/growth factor complex. In the current model (i) GPC3 may store Wnts on the HS chains; (ii) SULF2 may release Wnts from the HS chains of GPC3; and (iii) Wnts bind Frizzled and activate Wnt/Frizzled signaling. However, it is unclear whether Frizzled is part of the complex or how SULF2 is regulated and activated. Filmus’ group at the University of Toronto showed that HCC cells infected with lentivirus expressing soluble GPC3 (sGPC3) had a lower cell proliferation rate [27]. My laboratory at the US National Cancer Institute (NCI) produced a recombinant sGPC3ΔGPI protein in human embryonic kidney (HEK)-293 cells (residues: Q25–H559) without the GPI anchor. We found that recombinant sGPC3ΔGPI directly inhibited the growth of GPC3-expressing HCC in vitro [13]. Recently, we generated a recombinant sGPC3ΔGPI-human IgG constant fragment (Fc) fusion protein and confirmed that it inhibited the growth of HCC (unpublished data). Our observations indicate that recombinant sGPC3ΔGPI protein may function as a dominant-negative form to block the key molecular interactions and signaling by competing with endogenous GPC3 for its associated extracellular proteins in the glypican-Wnt/growth factor complex on HCC cells. These studies clearly show the important roles of GPC3 in HCC cell growth and proliferation. Further studies will be necessary to reveal the molecular mechanisms underlying the role of GPC3 in the glypican-Wnt/growth factor complex and HCC pathogenesis, particularly tumor growth and proliferation.
4. GPC3 expression in Hepatocellular Carcinoma
There is a growing body of evidence that GPC3 is a new tumor marker for HCC. Hsu et al. [28] first detected the GPC3 mRNA in 143 of 191 (74.8%) primary and recurrent HCCs taken from 154 patients in 1997. GPC3 expression was also found in the HCC cell lines HepG2, Hep3B and HuH-7 [24] and in more than 70% of HCC tumors, but not in normal liver tissue when using a rabbit polyclonal antibody [29] or with mAb 1G12 [11]. Many studies have shown high GPC3 protein expression in HCC but not in normal liver tissue [30–32]. We showed the absence of GPC3 protein expression in CCA, indicating that GPC3 may be potentially used as a diagnostic marker to distinguish between these two primary liver cancers [1]. Because of the finding that GPC3-positive HCC patients have a significantly lower 5-year survival rate than GPC3-negative HCC patients, GPC3 expression is correlated with poor prognosis in HCC [30].
GPC3 is also expressed to a lesser degree in melanoma, ovarian clear-cell carcinomas, and other tumors [1]. However, the significance of GPC3 as a diagnostic tool for tumors other than HCC is unclear. On the other hand, GPC3 is silenced in breast cancer, mesothelioma, epithelial ovarian cancer, and lung adenocarcinoma [1].
5. GPC3-targeted antibody therapy
Given the high expression in HCC, the usefulness of GPC3 as a target for both antibody- and cell-based immunotherapies has been explored [1]. This review focuses on antibody therapies. Table I summarizes the available mAbs specific for GPC3. In 2003, Midorikawa et al. reported a mAb against a GPC3 peptide consisting of 17 residues (residues: 355–371) to study the interaction of GPC3 and FGF-2 [24]. Capurro et al. described a mAb called 1G12 specific for the last 70 amino acids of the C-terminus of the GPC3 core protein [11] and used it to detect serum GPC3 in HCC patients. Hippo et al. made two mAbs, M18D04 and M19B11, specific for the N-terminus of GPC3 (residues: 25–358) [10]. Although these two groups used mAbs that recognized two different domains, surprisingly, they found a similar proportion of GPC3-positive sera in HCC patients, suggesting the linkage of the N-terminus and C-terminus of serum GPC3 by intramolecular disulfide bonds. However, we cannot rule out the possibility that full-length GPC3 may actually be inefficiently cleaved by furin in some HCC cells. In addition, Yamauchi et al. made a form of GPC3 protein lacking the GPI anchor region in Chinese hamster ovary (CHO) cells and used the recombinant protein as an immunogen to obtain two mAbs: A1836A for the N-terminus of GPC3 and GPC3-C02 for the C-terminus. These mAbs were used as reagents for IHC analysis [31].
Table I
Summary of available ant-glypican-3 (GPC3) monoclonal antibodies (mAbs)
mAb | Species | Epitope | Applications | References |
---|---|---|---|---|
Anti-GPC3 | Mouse | The junction of N- and C-termini (residues: 355–371) | IHC, Western blot | [24] |
1G12 | Mouse | The last 70 amino acids of C-terminus | ELISA (serum GPC3), IHC, Western blot, flow cytometry | [11] |
M18D04 and M19B11 | Mouse | N-terminus (residues: 25–358) | ELISA (serum GPC3), Western blot | [10] |
A1836A | Mouse | N-terminus | IHC, Western blot | [31] |
GPC3-C02 | Mouse | C-terminus | IHC, Western blot | [31] |
GC33 | Mouse | C-terminus (residues: 524–563) | Preclinical studies, Western blot, ELISA, flow cytometry | [33, 34] |
hGC33 | Humanized GC33 | C-terminus (residues: 524–563) | Phase I clinical studies | [35, 37] |
ELISA = enzyme-linked immunosorbent assay; IHC = immunohistochemistry.
The first therapeutic mAb against GPC3 has been recently described [33, 34]. Its epitope is located at the C-terminus (residues: 524–563). The mAb, designated GC33 (IgG2a, κ), induces antibody-dependent cellular cytotoxicity (ADCC) and exhibits tumor growth inhibition of subcutaneously transplanted HepG2 and HuH-7 xenografts in mice. Humanized GC33 (hGC33) mAb is as effective as murine GC33 against the HepG2 xenograft [35]. The ADCC anti-tumor activity of GC33 is mainly due to natural killer cells [33]. On the other hand, Takai et al. found that macrophages may also play an important role in the anti-tumor activity of the GC33 mAb by unknown mechanisms [36]. They found that the GC33 mAb did not directly inhibit the proliferation of GPC3-positive cancer cells. It has been suggested that an anti-GPC3 antibody may increase the susceptibility of HCC to chemotherapeutic agents [37]. The anti-tumor activity of the hGC33 mAb combined with standard chemotherapy agents has recently been evaluated. The combination of hGC33 and sorafenib is more potent in inhibiting tumor growth than sorafenib alone in the HepG2 xenograft model. The hGC33 mAb is currently being evaluated in two phase I clinical studies in patients with advanced or metastatic HCC. In one study (2008–2011), the hGC33 mAb is used as a single agent (http://clinicaltrials.gov/ct2/show/NCT00746317), while in the other study (2009–2012), the hGC33 mAb is used in combination with sorafenib to treat patients (http://clinicaltrials.gov/ct2/show/NCT00976170).
All currently available mAbs against GPC3 are murine variable fragment (Fv)- based, which could limit their use because of the increased likelihood of immunogenicity compared with human mAbs. Given their lower immunogenicity in patients, a fully human mAb is the most desirable antibody format for clinical applications. In May 2011, we first reported human mAbs against GPC3 at the Protein Engineering Summit (PEGS) Antibodies for Cancer Therapy symposium in Boston [38]. The mechanistic and translational studies using these novel anti-GPC3 human mAbs are ongoing at the NCI.
6. Discovery of mesothelin
Pastan and colleagues [39] at the NCI identified the mesothelin cDNA and protein using the mAb K1 that was generated by the immunisation of mice with human ovarian carcinoma (OVCAR)-3 cells in 1992. The MSLN gene encodes a 71 kDa precursor protein that is processed to a 40 kDa protein termed mesothelin, which is a GPI-anchored glycoprotein present on the cell surface [3]. The human MSLN gene encodes a precursor protein of 622 amino acids. Upon translocation into the endoplasmic reticulum, the N-terminal signal peptide (residues: 1–33) and the C-terminal GPI anchor addition signal (predicted cleavage site: Ser598) are removed and the latter is replaced with a GPI anchor. The precursor protein is cleaved into two products, MPF (residues: Ser34–Arg286) [2] and the GPI-anchored, membrane-bound, mature mesothelin starting from Glu296 [3]. There is a predicted furin cleavage site at Arg295. Unlike GPC3, mesothelin has a common Arg residue at the P2 position (294) of the furin cleavage motif (R292-F293-R294-R295) for what may be potentially efficient cleavage [12]. We sequenced the N terminus of soluble mesothelin by automated Edman degradation and confirmed the predicted furin cleavage site, indicating highly efficient furin-mediated cleavage [40]. Mesothelin is overexpressed in a variety of cancers, including mesothelioma, ovarian cancer, pancreatic cancer and lung cancer [3, 4, 39]. Research done by Hellstrom’s group and ours has shown that mesothelin is shed from tumor cells [40, 41], and antibodies specific for mesothelin are elevated in the sera of patients with mesothelioma and ovarian cancer [42, 43]. Shed serum mesothelin has been approved by USA FDA as a new diagnostic tumor biomarker to monitor mesothelioma. Pastan and colleagues developed an immunotoxin (SS1P) with a Fv for mesothelin [44]. Two phase I clinical trials were completed at the NCI [45, 46], and SS1P is currently being evaluated in phase II clinical trials to treat mesothelioma. A chimeric antibody (MORAb-009 or amatuximab) containing the mouse SS1 Fv for mesothelin was also developed, and a phase I clinical trial for ovarian cancer, mesothelioma and pancreatic cancer has been completed [47]. Currently, phase II clinical trials of MORAb-009 (amatuximab) combined with chemotherapy are ongoing.
7. Functional mechanisms of mesothelin
The normal biologic function of mesothelin remains elusive since mesothelin knockout mice do not show any detectable phenotype in growth or reproduction [48]. In recent years, the role of mesothelin in tumor pathogenesis has been studied. One aspect that has been investigated is the mesothelin-MUC16 interaction. MUC16 is a heavily glycosylated cell surface mucin with an average molecular weight between 2.5 and 5 million Da [49], which is shed into the serum. CA125 is a repeating peptide epitope of MUC16 and is used to monitor the response to therapy in ovarian cancer [50]. Rump and colleagues were the first to show that binding of MUC16 to membrane-bound mesothelin mediates cancer cell adhesion [51]. Their results suggest that mesothelin is a novel MUC16 binding protein and that the mesothelin-MUC16 interaction might lead to intraperitoneal dissemination of tumors. We, along with collaborators, analyzed the biochemical basis of this interaction between mesothelin and MUC16. The study by Patankar’s group found that the N-glycans on MUC16 were required for binding to the core protein of mesothelin [52]. In another study, my group used truncated mutagenesis and alanine replacement techniques to identify the functional domain (residues: 296–359) called IAB that is necessary and sufficient for the binding of mesothelin to MUC16 [53]. We have also shown that the SS1 Fv recognises this MUC16-binding domain and blocks the mesothelin-MUC16 interaction on cancer cells. We recently generated an immunoadhesin, HN125, against MUC16 that consists of IAB and the Fc portion of a human IgG1 antibody [54]. We showed that HN125 has high and specific affinity for MUC16-expressing cancer cells and has the ability to disrupt the heterotypic cancer cell adhesion mediated by the MUC16-mesothelin interaction. Moreover, HN125 elicits strong ADCC against MUC16-positive ovarian cancer cells in vitro. Our results suggest that HN125 merits evaluation as a potential therapeutic agent for preventing or treating MUC16-expressing malignant tumors.
Another aspect that has been explored is the regulation of mesothelin by Wnt and other major signalling pathways. Prieve and Moon first showed that mesothelin is upregulated by Wnt-1 in a single mouse epithelial cell line [55]. A 3-fold increase in mesothelin RNA is observed in cells treated with Wnt-1. Mesothelin RNA expression is also induced by Li+, an inhibitor of glycogen synthase kinase (GSK)-3β that mimics Wnt-1. Uehara and colleagues found that overexpression of mesothelin in human breast cancer cell lines (MCF-7, T47D and MDA-MB-231) promotes cancer cell survival under anchorage-independent conditions [56]. Cancer cells expressing high levels of mesothelin exhibit resistance to anoikis, a type of apoptosis induced by detachment from the surrounding extracellular matrix. Yao’s group observed a proliferative effect of mesothelin in pancreatic cancer cells. They found that expression of mesothelin increased pancreatic tumor cell proliferation and migration, and increased tumor volume in the nude mouse xenograft model [57]. Silencing of mesothelin inhibited cell proliferation and migration in cancer cells, and tumor progression in vivo. They further found that overexpression of mesothelin in pancreatic cancer cells led to constitutive activation of Stat3 and increased expression of cyclin E and cyclin E/cyclin-dependent kinase 2 complex formation, as well as increased G1-S transition [58]. They also discovered that mesothelin expression correlated with interleukin-6 (IL-6) in pancreatic cancer cells [59]. Overexpression of mesothelin in pancreatic cells led to higher IL-6 production. Conversely, silencing mesothelin reduced the IL-6 level. The ‘mesothelin/IL-6 axis’ may play an important role in pancreatic cancer and other mesothelin-expressing tumors. The correlation between mesothelin and IL-6 may provide a new rationale for combination therapy targeting both mesothelin and IL-6 for effective control of pancreatic cancers and other mesothelin-overexpressing cancers (e.g. CCA).
8. Mesothelin expression in cholangiocarcinoma
Mesothelin is highly expressed in epithelial malignant mesothelioma [3, 39, 60]. Mesothelin overexpression has been noted in other human cancers, such as epithelial ovarian cancer and pancreatic cancer [4]. Although earlier studies using the mAb K1 showed no expression of mesothelin in lung adenocarcinomas [60], Ordonez found mesothelin expression in 14 of 34 (41%) lung adenocarcinomas using the 5B2 mAb[5]. We analyzed mesothelin expression in lung cancer [61]. Using the NCI-60 cell line panel, we detected mesothelin mRNA in seven of nine (78%) lung cancer cell lines. Cell surface mesothelin expression was detected by flow cytometry. In addition, mesothelin mRNA was present in 10 of 12 (83%) lung adenocarcinoma samples obtained from patients. We have observed by IHC that one unusual feature of lung cancer is an increase in intracellular mesothelin reactivity compared with what is seen in mesotheliomas, ovarian cancer, or pancreatic cancer [61].
Ordonez first reported mesothelin expression in primary liver cancer by IHC and found that 37% (7 of 19) of CCA showed mesothelin expression and that no HCC exhibited mesothelin expression [5]. We recently characterized mesothelin expression in CCA by IHC, western blot and flow cytometry [6]. We showed high mesothelin expression in 33% of the surgically resected CCA specimens and three (OZ, KMBC and KMCH) of six CCA cell lines. No mesothelin staining was found in HCC or normal liver tissue. Mesothelin was primarily localised to the cellular plasma membrane, and the mature form (molecular weight: ~40 kDa) was found at a high level in CCA tissues. Interestingly, the precursor form of mesothelin (molecular weight: 71 kDa), as shown in lung adenocarcinomas [61], was not detectable in any of the CCA specimens. In CCA, furin cleavage of mesothelin is efficient. Moreover, 22% of CCA specimens had a high mesothelin expression level, which was comparable to the CCA cell line models. Interestingly, the anti-mesothelin SS1P immunotoxin showed very high and specific growth inhibition of tumor cells when added to mesothelin-expressing CCA cells. These findings indicate the potential use of SS1P in the novel immunotherapeutic treatment of CCA.
9. Mesothelin-targeted antibody therapies
The limited expression of mesothelin on normal human tissues and high expression in CCA and several human cancers makes mesothelin an attractive candidate for antibody therapy. Antibodies that are being evaluated in clinical trials include SS1P and MORAb-009. Table II summarizes all available mAbs specific for mesothelin currently being evaluated in translational research for cancer therapy or diagnosis.
Table II
Summary of available anti-mesothelin monoclonal antibodies (mAbs)
mAb | Species | Epitope | Applications | References |
---|---|---|---|---|
K1 | Mouse | C-terminus | ELISA, IHC, Western blot | [3, 39, 60] |
OV569 and 4H3 | Mouse | C-terminus | MESOMARK kit: ELISA (serum mesothelin) | [74] |
SS and SS1 (improved SS) | Mouse Fv | C-terminus | Phase I immunotoxin therapy, Western blot, flow cytometry | [62, 63] |
MORAb-009 (amatuximab) | Mouse/human chimeric | C-terminus | Phase II clinical studies, IHC, flow cytometry | [47, 71] |
MN and MB | Mouse | C-terminus | ELISA (serum mesothelin), IHC, Western blot, flow cytometry | [75, 76] |
MPF25 and MPF44 | Mouse | N-terminus | ELISA (serum MPF), Western blot | [77] |
7E7 | Mouse | N-terminus | ELISA (serum MPF) | [78] |
m912 | Human | C-terminus | Preclinical studies, ELISA, flow cytometry, Western blot | [72] |
HN1 | Human | C-terminus | Preclinical studies, flow cytometry, ELISA | [73] |
ELISA = enzyme-linked immunosorbent assay; Fv = variable fragment; IHC = immunohistochemistry; MPF = megakaryocyte potentiating factor.
SS1P is a recombinant immunotoxin consisting of an anti-mesothelin Fv linked to a truncated Pseudomonas exotoxin that mediates cell killing [62, 63]. After binding to mesothelin, the immunotoxin is internalized and undergoes processing in the endocytic compartment, and the immunotoxin fragment containing the adenosine diphosphate-ribosylation domain is transported to the endoplasmic reticulum and then translocated to the cytosol, where it inhibits elongation factor-2, leading to inhibition of protein synthesis and ultimately cell death [44]. Pre-clinical studies have shown that SS1P is cytotoxic to cell lines expressing mesothelin and causes complete regression of mesothelin expressing tumor xenografts in nude mice [4]. We recently studied the efficacy of SS1P in newly established in vitro and in vivo tumor models. We examined how the tumor microenvironment affects the penetration and killing activity of SS1P in a new in vitro, 3D spheroid, tumor model cultured using the human mesothelioma cell line (NCI-H226) and two primary cell lines isolated from the ascites of malignant mesothelioma patients [64]. Mesothelioma cells grown as monolayers or as spheroids expressed comparable levels of mesothelin; however, spheroids were at least 100-fold less affected by SS1P. We used confocal microscopy to examine the time course of SS1P penetration within spheroids. The penetration was limited after 4 hours. Interestingly, we found a significant increase in the number of tight junctions in the core area of spheroids by electron microscopy. Expression of E-cadherin, a protein involved in the assembly and sealing of tight junctions and highly expressed in malignant mesothelioma, was significantly increased in spheroids compared with monolayers. Moreover, we found that small interfering RNA silencing and antibody inhibition targeting E-cadherin enhanced SS1P immunotoxin therapy in vitro. In a new in vivo tumor model, we fluorescently labelled the NCI-H226 cell line using a lentiviral vector harbouring a luciferase-green fluorescent protein (Luc/GFP) fusion gene [65]. A new cell line (named LMB-H226-GL) that stably expresses high levels of Luc/GFP was obtained by single cell cloning. LMB-H226-GL can consistently form solid tumors in the peritoneum of mice. The model exhibited the pathologic hallmarks consistent with the clinical progression of malignant mesothelioma in terms of tumor growth, and spread inside the peritoneal cavity. All of the tumor-bearing mice had a significant response to SS1P treatment.
Two phase I clinical trials of SS1P were completed at the NCI [45, 46]. In one study, SS1P given as a 30-minute intravenous infusion every other day for six or three doses was administered to 34 patients with advanced mesothelioma (n = 20), or ovarian (n = 12) or pancreatic (n = 2) cancer [45]. Of the 33 evaluable patients, 4 had minor responses, 19 had stable disease (including 2 with resolution of ascites), and 10 had progressive disease. In another study of SS1P, 24 patients, 5 with peritoneal mesothelioma, 9 with pleural mesothelioma, 2 with pleural-peritoneal mesothelioma, 7 with ovarian carcinoma, and 1 with pancreatic carcinoma, received 4, 8, 12, 18, and 25 µg/kg/d for 10 days [46]. One patient had a partial response; other responses included cessation of ascites and independence from paracentesis, resolution of masses by positron emission tomography, and improved pain and range of motion. Based on phase I clinical studies showing the safety of SS1P and its anti-tumor activity, a clinical trial of SS1P in combination with chemotherapy is currently ongoing. The combination of SS1P with chemotherapy is based on laboratory work showing marked synergy between SS1P and chemotherapy in mouse models [66–69]. Our tumor spheroid results indicate that poor penetration is a major mechanistic factor for the resistance to SS1P in mesothelioma [64]. Antibody-based drugs enter into solid tumors mainly by a slow process of diffusion. Because of the fact that immunotoxins have a relatively short life in circulation (20 minutes in mice and 2–8 hours in humans) [70], the time that a tumor is exposed to high immunotoxin concentrations is relatively short. Hence, improving the penetration of immunotoxins is believed to have an important impact on their therapeutic effect.
MORAb-009 (amatuximab) is a chimeric (mouse/human) monoclonal IgG1/κ based on the SS1 Fv. MORAb-009 prevents adhesion of mesothelin-bearing tumor cells to MUC16-positive cells and can elicit ADCC on mesothelin-bearing tumor cells [71]. Treatment of MORAb-009 in combination with chemotherapy led to a marked reduction in tumor growth of mesothelin-expressing tumors in nude mice compared to chemotherapy or MORAb-009 treatment alone. A phase I clinical trial of MORAb-009 has been recently completed [47]. A total of 24 subjects were treated, including 13 mesothelioma, 7 pancreatic cancer, and 4 ovarian cancer patients. Eleven subjects had stable disease. Phase II studies of MORAb-009 in mesothelin-expressing cancers are ongoing.
In recent years, we and intramural collaborators at the NCI have pursued the generation of human mAbs for mesothelin. Dimitrov’s group at NCI-Frederick reported the first human mAb, m912 [72]. The m912 mAb binds cell surface-associated mesothelin and lyses A431/H9 [42] cells engineered to express mesothelin in the presence of peripheral blood mononuclear cells (PBMC) isolated from healthy donors, most likely by ADCC [72]. The m912 mAb does not induce ADCC in mesothelin-negative A431 cells. In another study, we isolated a high affinity human single-chain Fv (named HN1) specific for mesothelin from a naïve human single-chain Fv (scFv) phage display library [73]. To investigate the HN1 antibody as a potential therapeutic agent, we generated a fully human IgG1κ and an immunotoxin by fusing the HN1 scFv to a truncated Pseudomonas exotoxin A. The HN1 IgG kills cancer cells with very strong ADCC. The HN1 mAb binds a unique conformation-sensitive epitope in cell surface-associated human mesothelin with high affinity. The HN1 epitope is different from that of SS1. The HN1 mAb can functionally block the interaction of mesothelin and MUC16 on cancer cells. In addition, the HN1 immunotoxin kills mesothelin-expressing cancer cells with high cytotoxic activity. We believe that the HN1 mAb has significant potential for mesothelin-expressing cancer treatment and diagnosis.
10. Conclusions
This review has evaluated recent advances in liver cancer antibody therapies with a focus on GPC3 and mesothelin. GPC3 and mesothelin are attractive candidates for targeted therapies of liver cancer as they show high expression in HCC and CCA, respectively. First-generation murine Fv-based antibody drugs are currently undergoing clinical trials, which will help define the utility of GPC3 and mesothelin as targets for cancer therapy. The recent development of fully human mAbs may provide opportunities for novel cancer therapy. Future work may lead to the generation of mAbs directed to various functional domains of the targets and examine whether a mAb can directly inhibit tumor growth and metastasis by blocking the key ligand receptor interactions involved in tumor pathogenesis.
Acknowledgements
This research is supported by the Intramural Research Program of the NIH, National Cancer Institute (NCI), Center for Cancer Research. Dr Mitchell Ho is also supported by an Ovarian Cancer Research Fund Individual Investigator Award, a Mesothelioma Applied Research Foundation Grant in Honor of Craig Kozicki, a Zi-jiang Lecture Professorship from East China Normal University (Shanghai, China), and the NCI Director's Intramural Innovation Award for Principal Investigators. Dr Ho is a co-inventor on patents assigned to the US, as represented by the Department of Health and Human Services, for the investigational products. The funders have no role in study design, data collection and analysis, decision to publish, or preparation of the manuscript. The content of this publication does not necessarily reflect the views or policies of the Department of Health and Human Services, nor does mention of trade names, commercial products or organizations imply endorsement by the US Government.
Footnotes
The author has no conflict of interest directly relevant to the content of this review.
References
Full text links
Read article at publisher's site: https://doi.org/10.2165/11595360-000000000-00000
Read article for free, from open access legal sources, via Unpaywall:
https://europepmc.org/articles/pmc3198870?pdf=render
Citations & impact
Impact metrics
Citations of article over time
Alternative metrics
Smart citations by scite.ai
Explore citation contexts and check if this article has been
supported or disputed.
https://scite.ai/reports/10.2165/11595360-000000000-00000
Article citations
Oncofetal protein glypican-3 is a biomarker and critical regulator of function for neuroendocrine cells in prostate cancer.
J Pathol, 260(1):43-55, 14 Mar 2023
Cited by: 3 articles | PMID: 36752189 | PMCID: PMC10273879
Inhibition of Glypican-3 Cleavage Results in Reduced Cell Proliferation in a Liver Cancer Cell Line.
J Surg Res, 282:118-128, 19 Oct 2022
Cited by: 4 articles | PMID: 36272230 | PMCID: PMC10893758
Role of glypican-1 in regulating multiple cellular signaling pathways.
Am J Physiol Cell Physiol, 321(5):C846-C858, 22 Sep 2021
Cited by: 28 articles | PMID: 34550795 | PMCID: PMC8616591
Review Free full text in Europe PMC
Identification of a Glypican-3 Binding Peptide From a Phage-Displayed Peptide Library for PET Imaging of Hepatocellular Carcinoma.
Front Oncol, 11:679336, 04 Jun 2021
Cited by: 1 article | PMID: 34150644 | PMCID: PMC8212053
T-Cell Receptor Mimic Antibodies for Cancer Immunotherapy.
Mol Cancer Ther, 20(9):1533-1541, 25 Jun 2021
Cited by: 17 articles | PMID: 34172530 | PMCID: PMC8419142
Review Free full text in Europe PMC
Go to all (32) article citations
Other citations
Wikipedia
Data
Data behind the article
This data has been text mined from the article, or deposited into data resources.
BioStudies: supplemental material and supporting data
Clinical Trials (2)
- (1 citation) ClinicalTrials.gov - NCT00746317
- (1 citation) ClinicalTrials.gov - NCT00976170
Similar Articles
To arrive at the top five similar articles we use a word-weighted algorithm to compare words from the Title and Abstract of each citation.
Glypican-3: a new target for cancer immunotherapy.
Eur J Cancer, 47(3):333-338, 26 Nov 2010
Cited by: 136 articles | PMID: 21112773 | PMCID: PMC3031711
Review Free full text in Europe PMC
Therapeutic potential of targeting glypican-3 in hepatocellular carcinoma.
Anticancer Agents Med Chem, 11(6):543-548, 01 Jul 2011
Cited by: 19 articles | PMID: 21554204 | PMCID: PMC3843004
Review Free full text in Europe PMC
Glypican-3: A promising biomarker for hepatocellular carcinoma diagnosis and treatment.
Med Res Rev, 38(2):741-767, 16 Jun 2017
Cited by: 160 articles | PMID: 28621802
Review
Exploring Glypican-3 as a Molecular Target in Hepatocellular Carcinoma: Perspectives on Diagnosis and Precision Immunotherapy Strategies.
Front Biosci (Landmark Ed), 29(7):268, 01 Jul 2024
Cited by: 0 articles | PMID: 39082348
Review
Funding
Funders who supported this work.
Intramural NIH HHS
NCI NIH HHS (1)
Grant ID: Y99 CA999999