Abstract
Purpose
Positron emission tomography (PET) with (89)Zr-ibritumomab tiuxetan can be used to monitor biodistribution of (90)Y-ibritumomab tiuxetan as shown in mice. The aim of this study was to assess biodistribution and radiation dosimetry of (90)Y-ibritumomab tiuxetan in humans on the basis of (89)Zr-ibritumomab tiuxetan imaging, to evaluate whether co-injection of a therapeutic amount of (90)Y-ibritumomab tiuxetan influences biodistribution of (89)Zr-ibritumomab tiuxetan and whether pre-therapy scout scans with (89)Zr-ibritumomab tiuxetan can be used to predict biodistribution of (90)Y-ibritumomab tiuxetan and the dose-limiting organ during therapy.Methods
Seven patients with relapsed B-cell non-Hodgkin's lymphoma scheduled for autologous stem cell transplantation underwent PET scans at 1, 72 and 144 h after injection of ~70 MBq (89)Zr-ibritumomab tiuxetan and again 2 weeks later after co-injection of 15 MBq/kg or 30 MBq/kg (90)Y-ibritumomab tiuxetan. Volumes of interest were drawn over liver, kidneys, lungs, spleen and tumours. Ibritumomab tiuxetan organ absorbed doses were calculated using OLINDA. Red marrow dosimetry was based on blood samples. Absorbed doses to tumours were calculated using exponential fits to the measured data.Results
The highest (90)Y absorbed dose was observed in liver (3.2 ± 1.8 mGy/MBq) and spleen (2.9 ± 0.7 mGy/MBq) followed by kidneys and lungs. The red marrow dose was 0.52 ± 0.04 mGy/MBq, and the effective dose was 0.87 ± 0.14 mSv/MBq. Tumour absorbed doses ranged from 8.6 to 28.6 mGy/MBq. Correlation between predicted pre-therapy and therapy organ absorbed doses as based on (89)Zr-ibritumomab tiuxetan images was high (Pearson correlation coefficient r = 0.97). No significant difference between pre-therapy and therapy tumour absorbed doses was found, but correlation was lower (r = 0.75).Conclusion
Biodistribution of (89)Zr-ibritumomab tiuxetan is not influenced by simultaneous therapy with (90)Y-ibritumomab tiuxetan, and (89)Zr-ibritumomab tiuxetan scout scans can thus be used to predict biodistribution and dose-limiting organ during therapy. Absorbed doses to spleen were lower than those previously estimated using (111)In-ibritumomab tiuxetan. The dose-limiting organ in patients undergoing stem cell transplantation is the liver.Free full text
Biodistribution, radiation dosimetry and scouting of 90Y-ibritumomab tiuxetan therapy in patients with relapsed B-cell non-Hodgkin’s lymphoma using 89Zr-ibritumomab tiuxetan and PET
Abstract
Purpose
Positron emission tomography (PET) with 89Zr-ibritumomab tiuxetan can be used to monitor biodistribution of 90Y-ibritumomab tiuxetan as shown in mice. The aim of this study was to assess biodistribution and radiation dosimetry of 90Y-ibritumomab tiuxetan in humans on the basis of 89Zr-ibritumomab tiuxetan imaging, to evaluate whether co-injection of a therapeutic amount of 90Y-ibritumomab tiuxetan influences biodistribution of 89Zr-ibritumomab tiuxetan and whether pre-therapy scout scans with 89Zr-ibritumomab tiuxetan can be used to predict biodistribution of 90Y-ibritumomab tiuxetan and the dose-limiting organ during therapy.
Methods
Seven patients with relapsed B-cell non-Hodgkin’s lymphoma scheduled for autologous stem cell transplantation underwent PET scans at 1, 72 and 144 h after injection of ~70 MBq 89Zr-ibritumomab tiuxetan and again 2 weeks later after co-injection of 15 MBq/kg or 30 MBq/kg 90Y-ibritumomab tiuxetan. Volumes of interest were drawn over liver, kidneys, lungs, spleen and tumours. Ibritumomab tiuxetan organ absorbed doses were calculated using OLINDA. Red marrow dosimetry was based on blood samples. Absorbed doses to tumours were calculated using exponential fits to the measured data.
Results
The highest 90Y absorbed dose was observed in liver (3.2±
1.8 mGy/MBq) and spleen (2.9
±
0.7 mGy/MBq) followed by kidneys and lungs. The red marrow dose was 0.52
±
0.04 mGy/MBq, and the effective dose was 0.87
±
0.14 mSv/MBq. Tumour absorbed doses ranged from 8.6 to 28.6 mGy/MBq. Correlation between predicted pre-therapy and therapy organ absorbed doses as based on 89Zr-ibritumomab tiuxetan images was high (Pearson correlation coefficient r
=
0.97). No significant difference between pre-therapy and therapy tumour absorbed doses was found, but correlation was lower (r
=
0.75).
Conclusion
Biodistribution of 89Zr-ibritumomab tiuxetan is not influenced by simultaneous therapy with 90Y-ibritumomab tiuxetan, and 89Zr-ibritumomab tiuxetan scout scans can thus be used to predict biodistribution and dose-limiting organ during therapy. Absorbed doses to spleen were lower than those previously estimated using 111In-ibritumomab tiuxetan. The dose-limiting organ in patients undergoing stem cell transplantation is the liver.
Introduction
Non-Hodgkin’s lymphomas (NHL) account for 3% of all cancers worldwide [1]. Not all patients are cured with standard induction treatment [2]. For patients with aggressive, relapsed or progressive disease after first-line (immuno-)chemotherapy, a second-line regimen consisting of reinduction chemotherapy and consolidation with high-dose chemotherapy (carmustine, etoposide, cytarabine, melphalan; BEAM) followed by autologous stem cell transplantation (auSCT) is standard therapy with curative intent. A significant number of these patients will not be able to meet response criteria before transplantation or will relapse after transplantation [3]. The addition of anti-CD20 monoclonal antibodies (mAb) has substantially improved response rates and overall survival [4–6]. The 90Y-labelled anti-CD20 mAb ibritumomab tiuxetan (Zevalin®) is approved for treatment of patients with relapsed and refractory NHL. In recent studies, radioimmunotherapy (RIT) was integrated into upfront treatment in indolent NHL [7] or added to high-dose chemotherapy followed by auSCT, showing significant benefit [8, 9]. In standard treatment, the maximum amount of 90Y-ibritumomab tiuxetan given to patients is 15 MBq/kg with a maximum of 1.2 GBq [10]. The dose-limiting toxicity of RIT is myelosuppression. The effect of myelosuppression is prevented in auSCT with stem cell support. In general, higher external radiation doses are associated with improved clinical outcomes [11, 12]. To optimize therapeutic amounts of activity in individual patients, one needs to know the biodistribution of 90Y-ibritumomab tiuxetan and the effective dose to dose-limiting organs.
Biodistribution studies with 111In-ibritumomab tiuxetan and 131I-ibritumomab tiuxetan in humans have been published [e.g. 13, 14]. These studies were performed using gamma cameras, which complicates quantification. Labelling of ibritumomab tiuxetan with the positron-emitting isotope 89Zr allows for better quantitative assessment of biodistribution of ibritumomab tiuxetan with positron emission tomography (PET). Perk et al. [15] used N-succinyldesferal for coupling of 89Zr to ibritumomab tiuxetan and showed that 89Zr-ibritumomab tiuxetan has a nearly identical biodistribution compared with 90Y-ibritumomab tiuxetan in mice, which allows for assessment of biodistribution of 90Y-ibritumomab tiuxetan using 89Zr-ibritumomab tiuxetan and PET. A scout scan procedure using 89Zr-ibritumomab tiuxetan to assess biodistribution of ibritumomab tiuxetan prior to therapy can then aid in selection of patients that can benefit from a potentially toxic therapeutic amount of 90Y-ibritumomab tiuxetan, as well as in optimizing the administered amount of 90Y-ibritumomab tiuxetan in the individual patient. However, even though 89Zr-ibritumomab tiuxetan is representative of 90Y-ibritumomab tiuxetan, a scout scan procedure with 89Zr-ibritumomab tiuxetan for this purpose is only valid when biodistribution during scout scan and therapy are similar, that is not dependent on the administered amount of labelled antibody.
The aim of this clinical, prospective study was to assess biodistribution and radiation dosimetry of 90Y-ibritumomab tiuxetan in humans using 89Zr-ibritumomab tiuxetan and to investigate whether pre-therapy scout scans with 89Zr-ibritumomab tiuxetan can be used to predict biodistribution during therapy, that is whether a co-injection of a therapeutic amount of 90Y-ibritumomab tiuxetan influences biodistribution of 89Zr-ibritumomab tiuxetan. A secondary aim was to determine the dose-limiting organ for therapy with 90Y-ibritumomab tiuxetan, in order to enable dose escalation and/or optimization with 90Y-ibritumomab tiuxetan in patients undergoing auSCT.
Materials and methods
Patients
Seven patients with relapsed or refractory aggressive B-cell (CD20-positive) NHL, who did not qualify for standard auSCT and were younger than 66, were included. All patients had previously been treated in first line with R-CHOP (cyclophosphamide, doxorubicin hydrochloride, vincristine and prednisolone, combined with rituximab). Second-line chemotherapy consisted of R-DHAP (cisplatin, cytarabine, dexamethasone combined with rituximab), R-VIM (etoposide, ifosfamide, methotrexate, combined with rituximab) and R-DHAP, which did not lead to at least partial remission as monitored by 18F-fluorodeoxyglucose (FDG) PET.
All patients had a WHO performance status of 0–2. Exclusion criteria were history of intolerance to exogenous protein administration, severe cardiac dysfunction [New York Heart Association (NYHA) functional classification class II–IV], severe pulmonary dysfunction (vital capacity or diffusion capacity<
70%), unless clearly related to NHL involvement, hepatic dysfunction, bilirubin or transaminase
≥
2.5 times the upper normal limit, renal dysfunction (serum creatinine
≥
180 μmol/l or clearance
≤
40 ml/min), prior treatment with radiation therapy, uncontrolled infections, human immunodeficiency virus (HIV) positive and NHL localization in the central nervous system. The study was approved by the Medical Ethics Committee of the VU University Medical Center and all patients signed a written informed consent prior to inclusion.
Synthesis of 89Zr-ibritumomab tiuxetan and 90Y-ibritumomab tiuxetan
Ibritumomab tiuxetan was obtained from Bayer Schering AG (Berlin, Germany). 90Y (18.5 GBq/ml, radioactive half-life T
1/2=
64.1 h) was obtained from IBA (Louvain-la-Neuve, Belgium) and 90Y-ibritumomab tiuxetan was prepared according to instructions of the supplier. 89Zr (≥0.15 GBq nmol−1, T
1/2
=
78.5 h) was produced in-house by a (p,n) reaction on natural 89Y and isolated with the use of a hydroxamate column [16]. Methods for radiolabelling were used as described previously [15]. 89Zr-ibritumomab tiuxetan conjugates were analysed by instant thin-layer chromatography (ITLC) for radiochemical purity, and by a cell binding assay for immunoreactivity, as described before [16]. Endotoxin levels were assessed by use of a limulus amebocyte lysate (LAL) test system licensed by the US Food and Drug Administration (FDA) according to the instructions provided by the supplier (Endosafe®-PTS, Charles River Laboratories, Wilmington, MA, USA). These procedures resulted in a sterile final product with endotoxin levels
<
5 EU/ml. The radiochemical purity was always >97% (mean 97.4
±
1.5%). The immunoreactive fraction of 89Zr-ibritumomab tiuxetan preparations ranged from 72 to 81% (mean 75.0
±
3.2%).
Scan protocol
Patients received standard treatment with 250 mg/m2 Rituxan 1 week before and on the same day prior to both 90Y- and/or 89Zr-ibritumomab tiuxetan administrations. They received approximately 68±
11 MBq 89Zr-ibritumomab tiuxetan 31 days before stem cell transplantation (Table 1) followed by three PET scans at 1, 72 and 144 h post-injection (p.i.) covering skull base to femur. Blood samples were drawn at 5, 10, 30 min and 1, 2, 72 and 144 h p.i. for measurement of absolute radioactivity concentrations in whole blood and plasma. In four patients, this was followed 14 days later by 15 MBq/kg or 30 MBq/kg of 90Y-ibritumomab tiuxetan, each in two patients, with a co-injection of 69
±
6 MBq 89Zr-ibritumomab tiuxetan, again followed by the same PET and blood sampling protocol. The first two patients were treated in hospital; therefore, all urine during the first 72 h after injection was collected and radioactivity in urine was measured. Patients 3 to 7 were treated in our outpatient department, until high-dose chemotherapy started 7 days before stem cell transplantation.
Table 1
Patient characteristics
No. | Sex | Age | Weight (kg) | 89Zr |
89Zr![]() ![]() | 89Zr scan 1 (MBq) | 89Zr scan 2 (MBq) | 90Y (MBq) |
---|---|---|---|---|---|---|---|---|
1 | F | 50 | 84 | 1, 72, 144 | 1, 72, 144 | 49.7 | 65.5 | 1,267.7 |
2 | F | 50 | 90 | 1, 72, 144 | 1, 72, 144 | 48.0 | 88.5 | 2,473.40 |
3 | M | 43 | 71 | 1, 72, 144 | 1, 72, 144 | 79.2 | 77.7 | 1,151.3 |
4 | M | 61 | 78 | 1, 72, 144 | 72, 144 | 74.7 | 64.8 | 2,378.8 |
5 | M | 46 | 82 | 1, 72, 144 | – | 68.4 | ||
6 | M | 64 | 76 | 72, 144 | – | 77.5 | ||
7 | F | 37 | 82 | 1, 72 | – | 75.8 |
PET scans were made on a dedicated full ring ECAT EXACT HR+ (CTI/Siemens, Knoxville, TN, USA) PET camera in 3-D acquisition mode. Whole-body scans consisted of 7-min emission and 3-min transmission scans per bed position. Data were normalized and corrected for randoms, scatter, attenuation and decay. Attenuation correction was based on a transmission scan with rotating 68Ge rod sources. Images were reconstructed to 128×128 pixels with dimensions 5.15×5.15×2.43 mm using an attenuation- and normalization-weighted ordered subsets expectation maximization (NAW-OSEM) algorithm with 2 iterations with 16 subsets, followed by post-smoothing of the reconstructed image using a 5-mm full-width at half-maximum Gaussian filter. This resulted in images with a spatial resolution of about 7 mm. Quantitative accuracy of 89Zr PET scans was previously confirmed using phantom studies and comparisons between PET-derived and sampled blood radioactivity concentrations, as well as between PET-derived and biopsy tumour radioactivity concentrations [17].
Volume of interest definition
The activity, percentage injected dose (%ID) and standardized uptake value (SUV) normalized to body mass in all organs that could be distinguished from background (lung, liver, spleen and kidneys) were determined using the mean activity concentration in volumes of interest (VOI) drawn over the entire organs using software developed in-house [18]. The activity in tumours was defined by drawing a 50% isocontour VOI. VOIs were drawn separately on all PET scans available for each patient. VOIs were drawn independently over each acquired PET image.
Dosimetry
For calculation of 90Y residence times and absorbed doses, organ and tumour radioactivity were corrected for 89Zr decay, i.e. multiplied by exp(ln(2)t/78.4), with t the time since injection and 78.4 the radioactive half-life of 89Zr in hours, and then uncorrected for decay applying the radioactive half-life of 90Y, i.e. multiplied by exp(−ln(2)t/64.1), with the 64.1 h half-life of 90Y. Residence times for 89Zr- and 90Y-labelled antibodies were calculated as the area under the curve of the organ time-activity data determined by piecewise exponential fitting and assuming only physical decay after the last measurement, divided by the amount of injected activity. Residence time for the red marrow was estimated by assuming a red marrow radioactivity concentration of 30% of the whole blood activity concentration [19]. The residence time of the remainder of the body was calculated as the maximum possible residence time [the radioactive half-life of the tracer divided by ln(2)] minus the sum of residence time of source organs including red marrow, assuming no excretion during the time course of the scans. Tumour absorbed doses were estimated assuming local deposition of all radiation emitted by 90Y. The area under the curve of the tumour time-activity curves was estimated using a single exponential fit to two measured data points at 72 and 144 h p.i. Absorbed doses were calculated using the OLINDA/EXM 1.0 software [20].
Statistical analysis
Total 89Zr-ibritumomab tiuxetan uptake in organs and tumours during the scout procedure and during treatment were compared using regression analysis.
Results
Patient characteristics are shown in Table 1. All patients tolerated 89Zr-ibritumomab tiuxetan well, with no adverse reactions noted. Three patients underwent all scans both prior to and during therapy. Two patients (1 and 3) received standard therapy activity of 90Y-ibritumomab tiuxetan and two patients (2 and 4) were treated with a higher 90Y-ibritumomab tiuxetan activity. Three patients (5–7) did not receive the co-injection of 89Zr- and 90Y-ibritumomab tiuxetan because of logistical problems. One patient (7) had to be excluded from further analysis because he did not undergo a third PET scan (144 h p.i.) because of scanner problems. In total, data from six patients could be used to assess biodistribution of 89Zr-ibritumomab tiuxetan at 72 and 144 h p.i. Calculation of residence times in the first three patients, both with and without the 1-h p.i. scans, revealed no significant differences due to exclusion of the first scan (Pearson correlation coefficient r=
0.99, linear regression with and without 1-h p.i. data point). Therefore, dosimetry data of patients 4 and 6 were included in the analysis and data from four patients could be used to compare biodistribution of 89Zr-ibritumomab tiuxetan prior to and during therapy.
Images at 1 h p.i. showed mainly blood pool activity and activity in heart, liver, spleen, bone marrow and kidneys. No activity was seen at tumour sites. The images at 72 and 144 h p.i. showed increasing activity on tumour sites and decreasing activity in source organs (Fig. 1). Negligible amounts of activity (<1% of administered activity) were found in urine.
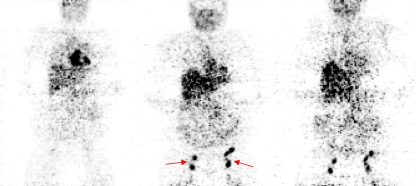
Typical coronal section 89Zr-ibritumomab tiuxetan images at 1, 72 and 144 h p.i. Arrows indicate tumour localizations
Figure 2 shows SUV as a function of time for all source organs. As shown in Fig. 3, a strong correlation between estimated absorbed doses in different organs with 89Zr-ibritumomab tiuxetan without 90Y-ibritumomab tiuxetan and 89Zr-ibritumomab tiuxetan with 90Y-ibritumomab tiuxetan was found (r=
0.97). The highest mean 90Y absorbed dose as calculated from the 89Zr-ibritumomab tiuxetan biodistribution was seen in the liver with 3.2
±
1.8 mGy/MBq (range 1.8–6.6 mGy/MBq, Table 2). However, absorbed dose to the spleen (2.9
±
0.7 mGy/MBq, range 1.8–3.8 mGy/MBq) was, although marginally, higher in five of six patients. The effective dose was 0.87
±
0.14 mSv/MBq. Table 3 shows effective half-lives of 90Y-ibritumomab tiuxetan for the 1–72 and 72–144 h intervals. As expected, no effect of the higher treatment activity of 90Y-ibritumomab tiuxetan on the biodistribution in two of the four patients was seen, although this could not be tested statistically due to the small patient groups.
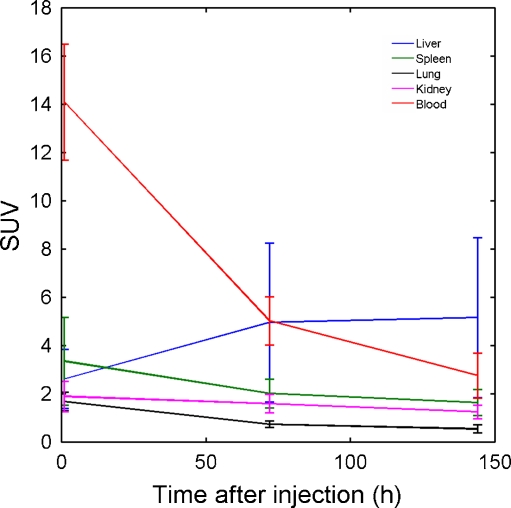
89Zr-ibritumomab tiuxetan SUV versus time after injection in liver, spleen, lung, kidneys and blood (mean ± SD, n=
6)
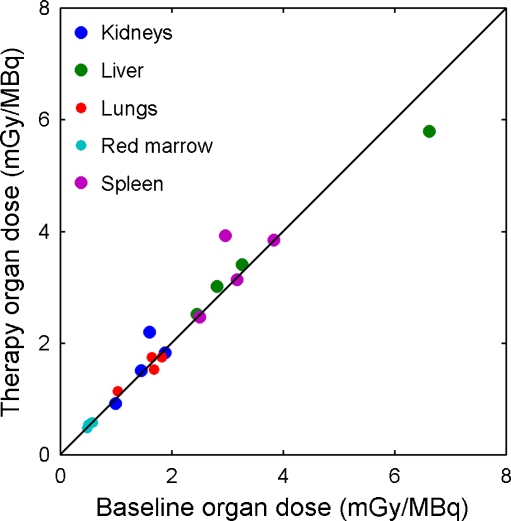
Absorbed organ doses estimated using a scout scan with 89Zr-ibritumomab tiuxetan prior to therapy versus those estimated using a simultaneous administration of 89Zr-ibritumomab tiuxetan and 90Y-ibritumomab tiuxetan. The solid line is the line of identity
Table 2
89Zr-ibritumomab tiuxetan and 90Y-ibritumomab tiuxetan organ residence times and absorbed doses as estimated using a scout scan with 89Zr-ibritumomab tiuxetan (n=
6)
89Zr-ibritumomab tiuxetan | 90Y-ibritumomab tiuxetan | |||
---|---|---|---|---|
Residence time | Absorbed dose | Residence time | Absorbed dose | |
Mean ± SD (h) | Mean ± SD (mGy/MBq) | Mean ± SD (h) | Mean ± SD (range) (mGy/MBq) | |
Liver | 11.6![]() ![]() | 1.36![]() ![]() | 9.9![]() ![]() | 3.2![]() ![]() |
Spleen | 1.08![]() ![]() | 1.04![]() ![]() | 0.95![]() ![]() | 2.88![]() ![]() |
Kidney | 0.93![]() ![]() | 0.754![]() ![]() | 0.81![]() ![]() | 1.46![]() ![]() |
Lung | 2.83![]() ![]() | 0.63![]() ![]() | 2.54![]() ![]() | 1.47![]() ![]() |
Red marrow | 0.59![]() ![]() | 0.460![]() ![]() | 0.48![]() ![]() | 0.520![]() ![]() |
Remaindera | 96![]() ![]() | 78![]() ![]() | ||
Effective dose (mSv/MBq) | 0.55![]() ![]() | 0.87![]() ![]() |
aBased on maximum residence time minus sum of source organ residence times
Table 3
Mean (± SD) effective half-life of 90Y-ibritumomab tiuxetan as estimated using a scout scan with 89Zr-ibritumomab tiuxetan (1–72 h: n=
5; 72–144 h: n
=
6)
Effective half-life (h) | ||
---|---|---|
1–72 h p.i. | 72–144 h p.i. | |
Liver | 185![]() ![]() | 74![]() ![]() |
Spleen | 43![]() ![]() | 54![]() ![]() |
Kidney | 57![]() ![]() | 51![]() ![]() |
Lung | 32![]() ![]() | 42![]() ![]() |
Blood | 42![]() ![]() | 66![]() ![]() |
Tumour absorbed dose estimates were made for the 4 patients that underwent 89Zr-ibritumomab tiuxetan scans both prior to and during therapy with 90Y-ibritumomab tiuxetan, with 13 tumour localizations in total. The average size of the lesion VOIs was 3.3 cm3 (range 0.8–11.3 cm3). Tumour absorbed doses ranged from 8.6 to 28.6 mGy/MBq (mean 14.9 mGy/MBq). Figure 4 shows estimated tumour absorbed doses. Correlation between absorbed doses estimated using a scout scan and during therapy was moderate (r=
0.75), with no significant differences between baseline and therapy absorbed dose estimates (p
=
0.09, two-tailed paired t test).

Absorbed tumour doses estimated using a scout scan with 89Zr-ibritumomab tiuxetan prior to therapy versus those estimated using a simultaneous administration of 89Zr-ibritumomab tiuxetan and 90Y-ibritumomab tiuxetan. The solid line is the line of identity
Figure 5 shows the correlation between SUV of 89Zr-ibritumomab tiuxetan and absorbed dose of 90Y-ibritumomab tiuxetan in the liver. Correlation was high for SUV at 72 h p.i. as well as 144 h p.i. (both r=
0.99), with a nearly identical relationship between SUV and absorbed dose for both time points (slope 0.50 and 0.49 mGy
MBq−1
SUV−1; intercept 0.85 and 0.89 mGy/MBq, respectively).
Discussion
To our knowledge, this is the first study describing the biodistribution and radiation dosimetry of 90Y-ibritumomab tiuxetan therapy as assessed by 89Zr-ibritumomab tiuxetan PET. All prior studies aiming to estimate 90Y-ibritumomab tiuxetan absorbed doses were performed using 111In-ibritumomab tiuxetan and single photon imaging. The advantage of using a positron-emitting isotope is that PET is inherently quantitative, whereas quantification with, especially planar, gamma camera imaging involves considerable uncertainties that can often surpass estimated activity concentrations themselves. Comparison of measured trues and random count rates as measured during scout scans and during scans with both 89Zr and therapeutic amounts of 90Y showed that the presence of 90Y had no effect on PET count rates and hence did not influence quantitative accuracy.
Although the results of the present work are generally consistent with previous studies using 111In-ibritumomab tiuxetan, there are a few important differences. The highest absorbed dose in the current work was found for the liver (3.2±
1.8 mGy/MBq, range 1.5–6.6 mGy/MBq) and the spleen (2.9
±
0.7 mGy/MBq, range 1.8–3.6 mGy/MBq). Wiseman et al. [13], using 111In-ibritumomab tiuxetan in a study population of follicular lymphomas and transformed B-cell NHL, found the highest absorbed dose in the spleen with a median absorbed dose of 7.30 mGy/MBq with a range of 3.50–26.0 mGy/MBq followed by liver (4.60, range 2.20–11.0 mGy/MBq), lungs (1.90 mGy/MBq, range 1.30–4.30 mGy/MBq), red marrow (0.65 mGy/MBq, range 0.26–1.10 mGy/MBq) and kidneys (0.20 mGy/MBq, range (0.01–0.65 mGy/MBq). Although Fisher et al. [14], with a study population of low- and intermediate-grade NHL, found an absorbed dose to the spleen of 4.7
±
2.3 mGy/MBq, closer to the results in the current study, the spleen was the organ with the highest absorbed dose, followed by liver (3.6
±
1.4 mGy/MBq), red marrow (2.7
±
0.9 mGy/MBq), kidneys (2.4
±
0.6 mGy/MBq) and lungs (0.8
±
0.8 mGy/MBq). Shen et al. [21], using 111In-ibritumomab tiuxetan as well, also found the spleen to be the organ receiving the highest absorbed dose with a median absorbed dose of 6.1 mGy/MBq (range 1.8–17.8 mGy/MBq). Absorbed dose estimates from all publications referred to above and from the Zevalin package insert are summarized in Table 4. The doses from the Zevalin package insert were estimated with 111In-Zevalin. In this study, the spleen is the organ with the highest absorbed dose as well, at 9.4 mGy/MBq (range 1.8–20.0 mGy/MBq). Shen et al. (study population of follicular or transformed CD20-positive B-cell NHL) administered 111In-ibritumomab tiuxetan with and without additional treatment with rituximab and found no difference in absorbed radiation dose before and during treatment except in the spleen where a significant decrease of residence time was found. They also analysed the tumour absorbed dose which was consistent with our data with a median absorbed dose of 18.1 mGy/MBq (range 4.7–98.9 mGy/MBq). Shen et al. showed a decrease in residence time and volume of the spleen after long-term rituximab treatment compared to a single dose of rituximab before 111In/90Y-ibritumomab tiuxetan. This could also apply to our study population and explain the difference from the four other studies where a single dose of rituximab was given prior to ibritumomab tiuxetan therapy. This observation is also in agreement with the study of Illidge et al. [22].
Table 4
Comparison of median absorbed doses of 90Y-ibritumomab tiuxetan for selected organs in mGy/MBq in different studies
Liver | Spleen | Kidney | Lung | Red marrow | Whole body (mSv/MBq) | |
---|---|---|---|---|---|---|
Current study | 3.2 (1.5–6.6) | 2.9 (1.8–3.6) | 1.46 (0.99–1.88) | 1.47 (1.07–1.82) | 0.52 (0.49–0.58) | 0.87 (0.70–1.06) |
Wiseman et al. [13] | 4.60 (2.20–11.0) | 7.30 (3.50–26.0) | 0.20 (<0.01–0.65) | 1.90 (1.30–4.30) | 0.65 (0.26–1.10) | 0.54 (0.46–0.78) |
Fisher et al. [14] | 3.1 (2.3–6.6) | 4.3 (0.98–9.0) | 2.4 (1.4–3.9) | 0.60 (0.31–1.6) | 2.4 (1.7–4.5) | 0.55 (0.44–0.81) |
Shen et al. [21] | 3.66 (2.11–11.62) | 6.14 (1.82–17.76) | 3.31 (1.95–4.65) | 1.10 (0.41–2.31) | 0.79 (0.32–1.22) | 0.48 (0.24–0.86) |
90Y-Zevalin® | 4.8 (2.9–8.1) | 9.4 (1.8–20.0) | 0.1 (0.0–0.3) | 2.0 (1.2–3.4) | 1.3 (0.6–1.8) | 0.5 (0.4–.07) |
Differences in biodistribution between 111In-mAb and 90Y-mAb conjugates have been observed before in vivo in tumour-bearing mice and in cancer patients [23–25]. Perk et al. [26] showed in their study that biodistribution of 89Zr-Df-cetuximab and 88Y-DOTA-cetuximab (88Y as a substitute for 90Y) is comparable for all observed organs, except differences in bone accumulation (sternum and thigh bone). What is more, the same was shown for 89Zr-ibritumomab tiuxetan and 90Y-ibritumomab tiuxetan in NHL-bearing mice [15]. Therefore, we can state that 89Zr-ibritumomab tiuxetan absorbed doses in liver and spleen are representative for 90Y-ibritumomab tiuxetan. Furthermore, the discrepancy between 111In-ibritumomab tiuxetan and 89Zr-ibritumomab tiuxetan uptake in liver and especially spleen has been described before when comparing 111In-DTPA-octreotide and 86Y-DOTA-octreotide as analogues of 90Y-DOTA-octreotide [27]. In this study 111In-DTPA-octreotide was shown to overestimate absorbed dose in kidneys and spleen, whereas absorbed dose to liver was underestimated in comparison to 86Y-DOTA-octreotide.
The effective dose found in the present study was generally higher than found in previous studies using 111In-ibritumomab tiuxetan (0.87±
0.14 mSv/MBq compared to
~
0.5 mSv/MBq). The remainder of the body residence times were based on the assumption of no excretion of radioactivity, which is likely to lead to conservative effective dose estimates in the current study. Using whole-body VOIs instead, which in the present work would not include the legs, would have resulted in somewhat lower effective doses (mean 0.72 mSv/MBq). However, because the energy of the radiation emitted by 90Y is deposited within the same organ only, this does not affect 90Y-ibritumomab tiuxetan organ absorbed doses.
Red marrow and liver absorbed doses were 26 and 9% of the threshold for nonstochastic effects (2 and 35 Gy, respectively) [28]. Since the liver is the dose-limiting organ in patients undergoing auSCT, this implies that a considerably higher amount than 30 MBq/kg can be given to these patients without resulting in deterministic effects. Clinically, no significant abnormalities were seen and no significant decreases of liver function or liver function abnormalities were seen in this particular group of patients. Use of blood data to estimate marrow absorbed doses may underestimate dosimetry results if there is any interaction of the targeting agent with the bone marrow. However, this is not relevant for the patient group discussed in the present work, since patients will undergo auSCT following therapy.
Relative to organ dosimetry, tumour dosimetry shows a moderate correlation between baseline and therapy scans. This is most probably due to the small volumes of the tumours comparing the organ volumes, resulting in increased uncertainty in measurement of radioactivity concentrations. Tumour VOIs were drawn as 50% isocontours independently on each image, as recommended in the European Association of Nuclear Medicine procedure guidelines for tumour imaging with 18F-FDG [29]. The size of these isocontour VOIs is dependent on the statistically uncertain values for the maximum pixel, and variations in tumour VOI sizes over time result in variations in partial volume errors, which further affect the accuracy of absorbed dose estimates. To minimize these effects, tumour VOIs could be based on anatomical information (e.g. coregistered CT or MRI scans, preferably acquired using PET/CT or PET/MRI scanners), and partial volume corrections could be applied based on the anatomical information. Since the data in the present study were acquired on a stand-alone PET scanner, VOIs could not be drawn on coregistered CT images and no attempts to further improve the quantitative accuracy of tumour measurements were made. In addition, the limited number of data points and resulting uncertainty in tumour uptake, especially during the first 24–48 h after injection, may contribute to the low tumour dose correlation.
As Fig. 5 shows, SUV of 89Zr-ibritumomab tiuxetan at either 72 or 144 h p.i. may be a good predictor of the absorbed radiation dose to the liver during 90Y-ibritumomab tiuxetan therapy, although this should be confirmed in a larger study. Therefore, a single PET scan of the liver at 3 or 6 days after injection of 89Zr-ibritumomab tiuxetan could be sufficient to estimate the maximum amount of 90Y-ibritumomab tiuxetan that can be administered to an individual patient who will also undergo auSCT. This strategy will lead to a therapy increasingly tailored to the individual patient. Although the present protocol requires administration of Rituxan prior to the pretreatment scan as well, this has limited influence on clinical feasibility since this is part of the routine treatment of the present patient group. Poor uptake in the tumour at the pre-therapy scan cannot be a reason to exclude patients from receiving this therapy. In tumour, not only the effect of RIT is important, but also the effect of Rituxan. Additional studies are needed for improved assessment of tumour dosimetry.
Conclusion
Biodistribution of 89Zr-ibritumomab tiuxetan is not influenced by simultaneous therapy with 90Y-ibritumomab tiuxetan. Therefore, a pre-therapy scan with 89Zr-ibritumomab tiuxetan can be used to accurately predict radiation dosimetry during treatment with 90Y-ibritumomab tiuxetan. Absorbed doses to the spleen were lower than those previously estimated using 111In-ibritumomab tiuxetan. The dose-limiting organ in patients undergoing stem cell transplantation is the liver. In the future, a single 89Zr-ibritumomab tiuxetan PET scan may be sufficient to optimize the administered amount of 90Y-ibritumomab tiuxetan RIT in the individual patient when combined with auSCT.
Acknowledgement
The authors thank the staff at the Department of Nuclear Medicine & PET Research for their assistance in performing the PET scans.
Conflicts of interest
None.
Open Access
This article is distributed under the terms of the Creative Commons Attribution Noncommercial License which permits any noncommercial use, distribution, and reproduction in any medium, provided the original author(s) and source are credited.
Footnotes
Saiyada N.F. Rizvi and Otto J. Visser contributed equally to this work.
References
Full text links
Read article at publisher's site: https://doi.org/10.1007/s00259-011-2008-5
Read article for free, from open access legal sources, via Unpaywall:
https://link.springer.com/content/pdf/10.1007/s00259-011-2008-5.pdf
Citations & impact
Impact metrics
Article citations
Radioimmunotheragnosis in Cancer Research.
Cancers (Basel), 16(16):2896, 20 Aug 2024
Cited by: 1 article | PMID: 39199666 | PMCID: PMC11352548
Review Free full text in Europe PMC
Advances and challenges in immunoPET methodology.
Front Nucl Med, 4:1360710, 19 Feb 2024
Cited by: 0 articles | PMID: 39355220 | PMCID: PMC11440922
Review Free full text in Europe PMC
Immuno-PET: Design options and clinical proof-of-concept.
Front Med (Lausanne), 9:1026083, 14 Oct 2022
Cited by: 5 articles | PMID: 36314010 | PMCID: PMC9613928
Review Free full text in Europe PMC
Clinical Applications of Immuno-PET in Lymphoma: A Systematic Review.
Cancers (Basel), 14(14):3488, 18 Jul 2022
Cited by: 2 articles | PMID: 35884548 | PMCID: PMC9316480
Review Free full text in Europe PMC
89Zr-PET imaging to predict tumor uptake of 177Lu-NNV003 anti-CD37 radioimmunotherapy in mouse models of B cell lymphoma.
Sci Rep, 12(1):6286, 15 Apr 2022
Cited by: 2 articles | PMID: 35428777 | PMCID: PMC9012778
Go to all (53) article citations
Similar Articles
To arrive at the top five similar articles we use a word-weighted algorithm to compare words from the Title and Abstract of each citation.
High-dose radioimmunotherapy with 90Y-ibritumomab tiuxetan: comparative dosimetric study for tailored treatment.
J Nucl Med, 48(11):1871-1879, 01 Nov 2007
Cited by: 20 articles | PMID: 17978355
Radiation dosimetry results and safety correlations from 90Y-ibritumomab tiuxetan radioimmunotherapy for relapsed or refractory non-Hodgkin's lymphoma: combined data from 4 clinical trials.
J Nucl Med, 44(3):465-474, 01 Mar 2003
Cited by: 113 articles | PMID: 12621016
Absorbed dose and biologically effective dose in patients with high-risk non-Hodgkin's lymphoma treated with high-activity myeloablative 90Y-ibritumomab tiuxetan (Zevalin).
Eur J Nucl Med Mol Imaging, 36(11):1745-1757, 20 May 2009
Cited by: 10 articles | PMID: 19455328
Yttrium-90-ibritumomab tiuxetan radioimmunotherapy: a new treatment approach for B-cell non-Hodgkin's lymphoma.
Drugs Today (Barc), 40(2):111-119, 01 Feb 2004
Cited by: 14 articles | PMID: 15045033
Review