Abstract
Free full text

Downregulation of MiR-199a Derepresses Hypoxia-Inducible Factor-1α and Sirtuin 1 and Recapitulates Hypoxia Preconditioning in Cardiac Myocytes
Abstract
MicroRNAs are posttranscriptional gene regulators that are differentially expressed during various diseases and have been implicated in the underlying pathogenesis. We report here that miR-199a is acutely downregulated in cardiac myocytes on a decline in oxygen tension. This reduction is required for the rapid upregulation of its target, hypoxia-inducible factor (Hif)-1α. Replenishing miR-199a during hypoxia inhibits Hif-1α expression and its stabilization of p53 and, thus, reduces apoptosis. On the other hand, knockdown of miR-199a during normoxia results in the upregulation of Hif-1α and Sirtuin (Sirt)1 and reproduces hypoxia preconditioning. Sirt1 is also a direct target of miR-199a and is responsible for downregulating prolyl hydroxylase 2, required for stabilization of Hif-1α. Thus, we conclude that miR-199a is a master regulator of a hypoxia-triggered pathway and can be exploited for preconditioning cells against hypoxic damage. In addition, the data demonstrate a functional link between 2 key molecules that regulate hypoxia preconditioning and longevity.
MicroRNAs (miRNAs) are ≈ 20-nt oligoribonucleotides that specifically target mRNA and inhibit translation or induce its degradation. Each has the potential to regulate a set of specific genes that are involved in a common cellular function. For example, an array of growth-promoting genes are targeted by miR-1, and require its downregulation at the onset of cardiac hypertrophy.1 Similarly, when John et al analyzed the predicted targets for gene ontology terms, the authors found that miR-208 targets were biased toward “transcription factor,” whereas miR-105 had a preponderance of the term “small GTPase mediated signal transduction.”2 Thus, miRNAs offer a mechanism whereby functionally related genes are coregulated. Because miRNA levels are posttranscriptionally regulated,3–5 they, therefore, have the potential to elicit an immediate and specific change in translation by attaching to, or detaching from, mRNA targets.
Early ischemia (IPC) or hypoxia (HPC) preconditioning is an immediate cellular reaction to brief hypoxia/reoxygenation cycles that involves de novo protein, but not mRNA, synthesis.6 It was first described by Murry et al as a mechanism that protected the heart against subsequent prolonged ischemia- or ischemia/reperfusion-induced damage.7 It is mediated, at least in part, by adenosine, which is produced on hydrolysis of ATP and released from the cell to stimulate a surface receptor.8 Central to early preconditioning effects is the protection of mitochondria against hypoxic damage, mainly through inhibiting the opening of MPTP.9 Protein kinase Cε has been shown to interact with the MPTP proteins and inhibit mitochondrial swelling,10 possibly through a GSK3β-mediated effect.11
Hypoxia inducible factor (Hif)-1α is a well-established transcription factor that is rapidly induced by hypoxia through a posttranscriptional mechanism, in all tested cell types.12 It accounts for the transcription of 89% of genes that are upregulated during hypoxia.13 In the heart, overexpression of Hif-1α during hypoxia resulted in a smaller infarct size following ischemia/reperfusion and was associated with higher capillary density, vascular endothelial growth factor, and inducible nitric oxide synthase (iNOS), in the periinfarct zone.14 This suggested that Hif-1α plays a role in late IPC. Recently, though, Cai et al showed that mice heterozygous for Hif-1α fail in early preconditioning,15 whereas Eckle et al reported that knockdown of Hif-1α abolished the effect of early ischemia preconditioning.16 In this report, we describe the role of miR-199a in regulating Hif-1α and Sirtuin (Sirt)1 during HPC in cardiac myocytes.
Materials and Methods
Cell Cultures and Adenovirus Infection
Neonatal cardiac myocytes were prepared from Sprague–Dawley rat hearts as previously described, using both preplating and Percoll gradients for enriching of myocytes.17 Adult cardiac myocytes were prepared as previously described.18
All ribosomal DNA was delivered to the myocytes via adenovirus vectors using 10 to 20 multiplicity of infection.
Construction of Adenoviruses
Recombinant adenoviruses were constructed, amplified and titered, as previously described by Graham and Prevec.19
Constructs
See the expanded Materials and Methods section in the online data supplement at http://circres.ahajournals.org.
Northern Blotting
Northern blotting was performed as previously described.20
Cellular Fractionation and Western Blotting
Mitochondria were isolated using ProteoExtract Cytosol/Mitochondria Fractionation Kit (Calbiochem), according to the protocol of the manufacturer. Fifteen micrograms of protein were separated on a 4% to 20% gradient SDS-PAGE (Criterion gels, Bio-Rad) and transferred onto TransBlot Transfer membrane (Bio-Rad).
Antibodies
See the online data supplement.
Hypoxia and HPC
Cultured myocytes were subjected to hypoxia in a hypoxic chamber (Billups-Rothenberg Inc, Del Mar, Calif). The chamber was filled with gas mixture of 95% N and 4.8% with or without 0.2% CO2 (Inhalation Therapy, Clifton, NJ) at 7 psi/12 000 kPa filling pressure for 15 minutes. The chamber was then placed in a 37°C incubator. For HPC, cultured myocytes were subjected to 4 cycles of 1 hour of hypoxia followed by 1 hour of reoxygenation.
Luciferase Assay
A concatamer of miR-199a–predicted target sequence within the HIF1A 3′ (untranslated region) UTR (GTTGGTTATTTTTGGACACTGGT)×3, the SIRT1 3′UTR (GGACAGTTAACTTTTTAAACACTGG)×3, or a mutant sequence lacking any complementarity with miR-199a seed sequence, as previously described,20 were cloned in the 3′UTR of the luciferase gene, generating Luc.Hif13′UTR, Luc.Sirt13′UTR, and Luc.control vectors, respectively. Myocytes were transfected with these constructs, using Lipofectamine (Invitrogen), in the presence or absence of virally delivered miR-199a. After 24 hours, luciferase activity was assayed using an Lmax multiwell luminometer.
Caspase Assay
Caspase-3 activity was measured using ApoTarget Caspase-3 Pro-tease Assay (Biosource, Invitrogen), as recommended by the manufacturer. The activity was normalized to total protein content.
Immunocytochemistry
Immunocytochemistry was performed previously described.20 The Antibodies used were as follows: anti–Hif-1α (Novus Biologicals, Littleton, Colo), anti-p53 (Genscript, Piscataway, NJ), and anti–myosin heavy chain (Hybridoma Bank, University of Iowa, Iowa City).
Monitoring Mitochondrial Membrane Potential
Mitochondrial membrane potential was monitored using JC-1 cationic dye (Molecular Probes, Invitrogen) as recommended by the manufacturer. Briefly, the cells were incubated with JC-1 (0.35 μg/mL) for 20 minutes at 37°C. The cells were then washed with 1× PBS and imaged live.
Cardiac Ischemia in C57Bl/6 Mice
Through a left third intercostal thoracotomy, the pericardial sac is opened and an 8-0 nylon suture is passed under the left anterior descending coronary artery 2 to 3 mm from the tip of the left auricle. Then a nontraumatic silicone tubing is placed on top of the vessel and a knot tied on top of the tubing to occlude the coronary artery and to induce a permanent occlusion. The procedure was approved by the Institutional Animal Care and Use Committee at the New Jersey Medical School.
Early IPC of Porcine Hearts
IPC (first window) was induced by 2 cycles of 10 minutes coronary artery occlusion, followed by 10 minutes of reperfusion, as previously described by Shen et al, and approved by the Institutional Animal Care and Use Committee at the New Jersey Medical School.21
Statistical Analysis
Calculation of significance between 2 groups was performed using an unpaired, 2-tailed t test.
Results
Downregulation of MiR-199a During Hypoxia Is Required for Induction of Proapoptotic Genes
Our studies regarding differentially expressed miRNA in the heart revealed that mature miR-199a was reduced to undetectable levels during cardiac ischemia, whereas its precursor continued to accumulate (Figure 1a). A time course revealed that this occurred as early as 30 minutes after ischemia (Figure 1b). To investigate its function, we overexpressed it in myocytes exposed to hypoxia. Western blot analysis revealed that miR-199a resulted in complete inhibition of hypoxia-induced caspase-3, -6, -9, and -12 and FasL, AIF, and Bnip1 (Figure 1c). Northern blot analysis showed that miR-199a, but not miR-199a* or miR-21, was completely abolished by hypoxia and that the adenoviral delivered construct was able to rescue this downregulation (Figure 1d). This suggested that miR-199a downregulation is required for upregulation of hypoxia-induced apoptotic genes.

Downregulation of miR-199a is required for hypoxia-induced proapoptotic genes. a, C57Bl/6 mice were subjected to left coronary artery occlusion for 16 hour. The ischemic and remote regions of the left ventricle and the sham-operated ventricle were isolated and total RNA was extracted and analyzed by Northern blotting (n = 3). b, Mice were subjected to left coronary artery occlusion for 0.5, 3, and 6 hours and analyzed as in a. c, Myocytes were infected with a control or miR-199a–expressing adenoviruses before exposure to hypoxia for 24 hours in complete culture medium with serum (where marked by +). Protein was extracted and analyzed by Western blotting (n = 3). d, Myocytes were treated as in c. Total RNA was extracted and analyzed by Northern blotting (n = 3).
MiR-199a Targets and Inhibits Hif-1α
Computational prediction of targets by TargetScanS software22 identified Hif-1α as a miR-199a target. Figure 2a shows the alignment between miR-199a and a highly conserved region within the 3′UTR of mouse Hif-1α, which represents a putative target sequence that can confer inhibition of translation by miR-199a. Inclusion of this sequence within the 3′UTR of a luciferase gene rendered it a target of miR-199a, as demonstrated by the inhibition of its activity on overexpression of miR-199a (Figure 2b). For further confirmation, we cloned the Hif-1α cDNA with or without a deletion of its miR-199a recognition site. The deletion resulted in ≈4 times higher expression of the Hif-1α protein in cardiac myocytes, but not in HEK293 cells that are devoid of endogenous miR-199a (Figure 2c). The data demonstrate that miR-199a directly targets and inhibits miR-199a.
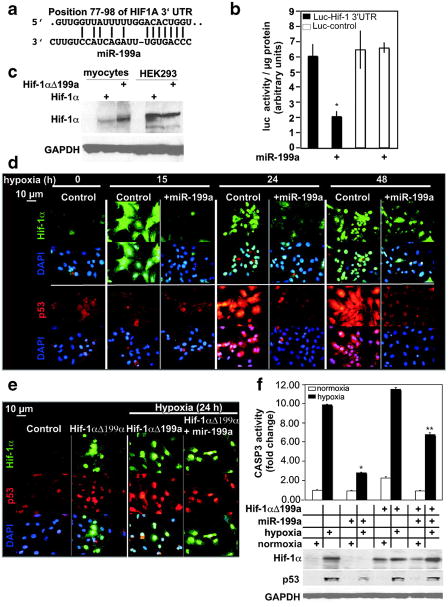
MiR-199a targets and inhibits Hif-1α. a, The alignment between Mus musculus miR-199a and the 3′UTR of HIF1A, identified by TargetscanS software. b, The miR-199a target region, or a mutant, was cloned into the 3′UTR of a luciferase gene (represented in the graph by black and white bars, respectively). These constructs were introduced into myocytes, in addition to exogenous miR-199a (where marked by +) or a control virus (n = 6). After 24 hours, luciferase activity was measured, averaged, and plotted. The y axis represents arbitrary luciferase activity normalized to micrograms of protein content. Error bars represent SEM. *P<0.01, miR-199a–treated luciferase-Hif-1α 3′UTR target vs control. c, Wild-type Hif-1αcDNA or a mutant lacking miR-199a target site (Hif-1αΔ199a) were delivered to cardiac myocytes or HEK293 cells. After 24 hours, protein was extracted and analyzed by Western blotting (n = 2). d, Myocytes were treated with a control or a miR-199a overexpressing virus for 24 hours before subjecting them to various periods of hypoxia, as indicated. Parallel slides were stained separately with anti–Hif-1α (green) or anti-p53 (red) antibodies and DAPI (blue) (n = 4). e, Myocytes were treated with a control or Hif-1αΔ199a virus, in the absence or presence of a control or miR-199a virus for 24 hours. Cells were then exposed to hypoxia for 24 hours where indicated, before immunostaining with anti-Hif-1α (green), anti-p53 (red), and DAPI (blue) (n = 3). f, Myocytes were treated as in e. Protein was extracted and either assayed for caspase 3 activity (graph, n = 6) or analyzed by Western blotting (n = 3). The treatments are indicated in the grid below the graph by + signs and each aligned with its Western blot results. Results were averaged, normalized to protein content, and plotted as fold change after adjusting basal levels to 1. Error bars represent SEM, *P<0.001, miR-199a–treated vs untreated cells during hypoxia; **P<0.01, miR-199a–treated plus Hif-1aΔ199a vs miR-199a–treated.
Hif-1α mediates hypoxia-induced apoptosis23 through stabilization of p53.24 Thus, to determine the effect of miR-199a on endogenous Hif-1α, its stabilization of p53, and myocyte apoptosis during hypobaric conditions, we subjected the myocytes to hypoxia in the absence or presence of excess miR-199a. Figure 2d shows that Hif-1α is robustly induced within 15 hours of oxygen deprivation. Initially Hif-1α is seen throughout the cell, but with longer periods of hypoxia, it becomes more restricted to the nucleus and coincides with the increase in p53 after 24 hours. Overexpression of miR-199a completely abolished Hif-1α and p53 during the first 24 hours of hypoxia but started losing effectiveness after 48 hour. The results suggest that downregulation of miR-199a during hypoxia is required for upregulation of Hif-1α and stabilization of p53.
Unlike Hif-1α, p53 is not a direct target of miR-199a, but has been shown to require Hif-1α for its stabilization during hypoxia.24 To test this possibility in cultured myocytes, we supplemented myocytes with Hif-1α lacking the miR-199a responsive element (Hif-1αΔ 199a, Figure 2e). This sustained the levels of Hif-1α during hypoxia after overexpression of miR-199a and completely rescued the downregulation of p53 (Figure 2e and 2f). The results confirm that p53 is not a direct target of miR-199a and that it requires Hif-1α for its stability during prolonged periods of hypoxia. The expression levels of p53 positively correlated with caspase 3 activity in these cells, which was dramatically reduced by miR-199a, but partially rescued by Hif-1αΔ199a (Figure 2f). Therefore, the results suggest that downregulation of miR-199a is required for induction of hypoxia-induced apoptosis, at least partly, through the Hif-1α-p53 pathway.
Knockdown of MiR-199a Recapitulates HPC
We next questioned whether knockdown of miR-199a during normoxia is sufficient for induction of Hif-1α. Figure 3a shows that abrogation of miR-199a with an antisense miR-199a expression vector (miR-199a eraser) resulted in the upregulation of Hif-1α. Interestingly, its distribution favored the cytosol, where it was punctate in appearance, similar to that observed during HPC, and in contrast to its predominant nuclear localization seen during hypoxia. Moreover, HPC or miR-199a knockdown inhibited hypoxia-induced Hif-1α transport to the nucleus, as well as upregulation of p53. Coimmunostaining of cells with α-myosin heavy chain revealed the predominance of myocytes versus nonmyocytes in these cultures and proved that miR-199a is intrinsic in both (Figure I in the online data supplement).
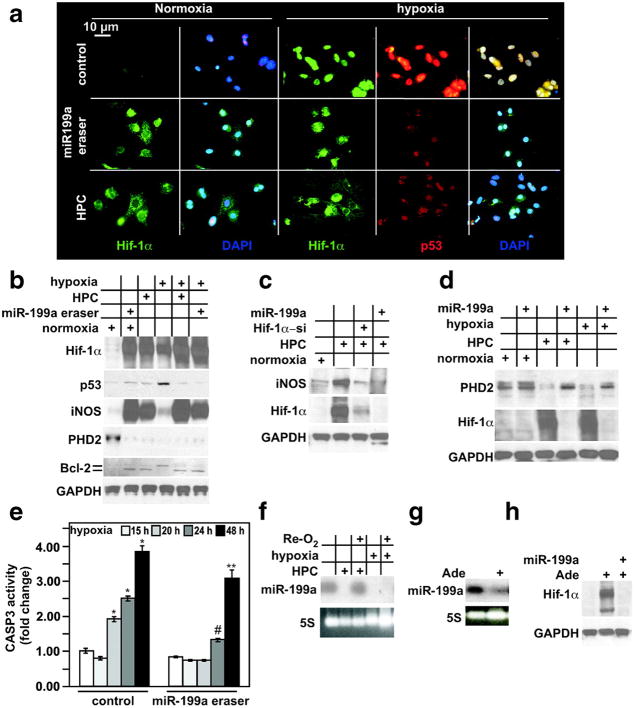
Knockdown of miR-199a induces upregulation of Hif-1α, iNOS, and down-regulation of PHD2, mimicking HPC. a, Cardiac myocytes were treated with a control or miR-199a eraser–expressing adenovirus for 24 hours, or HPC, as indicated on the left. A parallel set of myocytes were similarly treated and subsequently subjected to hypoxia for 24 hours, as indicated on the top. Myocytes were then fixed and costained with anti–Hif-1α (green), anti-p53 (red), and DAPI (blue) (n = 5). b, Myocytes were treated as described in a and as indicated in the grid by + signs. Protein was extracted and analyzed by Western blotting (n = 3). c, Myocytes were subjected to HPC before or after pretreatment with a control, miR-199a, and Hif-1α short interfering RNA (Hif-1α-si)-expressing adenoviruses for 24 hours, where indicated by + signs. Protein was extracted and analyzed by Western blotting for the molecules indicated on the left. d, Myocytes were subjected to 24 hours of hypoxia or HPC, before or after treatment with a control or miR-199a–expressing virus for 24 hours where indicated by + signs. Protein was extracted and analyzed by Western blotting for the molecules indicated on the left. e, Myocytes were subjected to 15, 20, 24, or 48 hours of hypoxia before or after treatment with a control or miR-199a eraser for 24 hours, as indicated. Protein was extracted and assayed for caspase 3 activity (n = 6). Results were averaged, normalized to protein content, and plotted as fold change, after adjusting basal levels to 1. Error bars represent SEM. *P<0.01, hypoxia vs normoxia; #P<0.01, miR-199a eraser–pretreated, 24-hour hypoxic, vs control-treated, 24-hour hypoxic, myocytes; **P<0.5, miR-199a eraser–pretreated, 48-hour hypoxic, vs control-treated, 48-hour hypoxic, myocytes. f, Myocytes were subjected to HPC or 24 hours of hypoxia with or without reoxygenation (Re-O2) for 12 hours as indicated with the + sign. Total RNA was then extracted and analyzed by Northern blotting for the miRNA indicated on the left (n = 2). g, Myocytes were stimulated with 100 μmol/L adenosine for 16 hours. Total RNA was then extracted and analyzed by Northern blotting for the miRNA indicated on the left (n = 2). h, Myocytes were treated as in (g). Protein was extracted and analyzed by Western blotting (n = 2).
Results of the immunostaining were confirmed by Western blot analysis (Figure 3b). In addition, we show that HPC and miR-199a knockdown, but not hypoxia, was associated with robust upregulation of iNOS and significant upregulation of Bcl-2. Pretreatment of cells with HPC or miR-199a eraser provided cells with iNOS during hypoxia and inhibited upregulation of p53 and the unmodified form of Bcl-2 (faster migrating band). The slower migrating form of Bcl-2 seen during hypoxia has been shown to be attributable to phosphorylation, which inhibits its antiapoptotic activity.25 iNOS expression was dependent on downregulation of miR-199a and upregulation of Hif-1α, because it was abolished by overexpression of miR-199a during HPC or by Hif-1α knockdown (Figure 3c).
MiR-199a eraser–induced upregulation of Hif-1α during normoxia suggested that it might be associated with inhibition or downregulation of prolyl hydroxylase (PHD)2. Indeed, PHD2 was reduced more than 90% in eraser-treated cells and during HPC or hypoxia (Figure 3b). This decrease was reversed by overexpression of miR-199a, suggesting that it requires downregulation of the miRNA under these conditions (Figure 3d). Not only did the miR-199a eraser elicit a gene expression pattern that mimicked HPC, but it also retarded the increase in caspase-3 activity induced by hypoxia (Figure 3e).
MiR-199a was reversibly downregulated during HPC but was irreversible after prolonged hypoxia (Figure 3f). Moreover, adenosine, which is an established mediator of IPC,26,27 induced miR-199a downregulation (Figure 3g). This was associated with upregulation of Hif-1α that was blocked by overexpression of miR-199a (Figure 3h). The data suggest that HPC requires downregulation of miR-199a.
Hif-1α Associates With and Protects Mitochondria During HPC
As noted earlier, during preconditioning of cells with hypoxia or miR-199a eraser, Hif-1α exhibited a punctate appearance in the cytosol. Because mitochondrial protection is central to preconditioning, we questioned whether Hif-1α might associate with this organelle. The results revealed that Hif-1α copurifies with mitochondria during HPC or miR-199a eraser treatment of cells but was undetectable in that fraction after 24 hours of hypoxia (Figure 4a). On the other hand, there was more nuclear Hif-1α during the latter condition than was observed during preconditioning (Figure 4b).
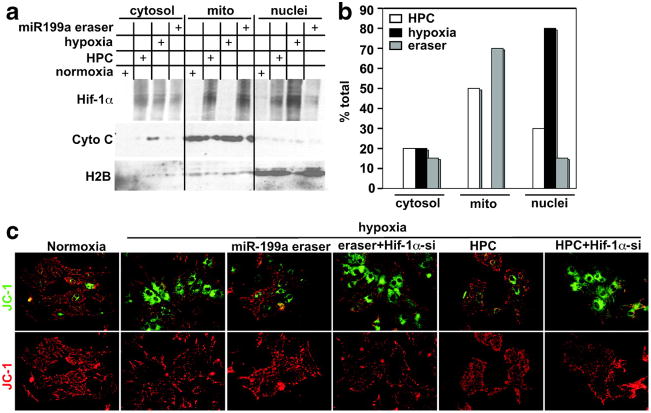
Hif-1α associates with mitochondria and is required for HPC-mediated protection. a, Cardiac myocytes were subjected to HPC, 24 hours of hypoxia, or treated with a control or the miR-199a eraser–expressing virus for 24 hours, as indicated in the grid by + signs. Cells were fractionated into cytosol, mitochondria, and nuclei and analyzed by Western blotting for the proteins indicated on the left (n = 3). b, The Hif-1α signal shown in a was quantitated in all fractions, for each treatment, and the percentage of total was calculated and plotted (n = 3). c, Cardiac myocytes were plated on gelatin-coated glass chamber slides. Cells were treated with a control or a Hif-1α short interfering RNA (Hif-1α-si)-expressing adenovirus for 48 hours before applying miR-199a eraser or HPC. They were then exposed to hypoxia for 24 hours. Following that, JC-1 dye was applied and the cells were imaged live (n = 4).
To determine whether miR-199a eraser treatment protects against hypoxia-induced mitochondrial damage and whether it requires Hif-1α, we monitored mitochondrial integrity using the JC-1 dye. Figure 4c shows that hypoxia-induced mitochondrial damage was rescued by HPC or miR-199a eraser pretreatment. This is reflected by low levels of green florescent monomeric dye in the cytosol and higher levels of red florescent aggregates in intact healthy mitochondria and vice versa during hypoxia. Knockdown of Hif-1α abrogated the mitochondrial protective effect of preconditioning. Thus, Hif-1α is required for mitochondrial protection during preconditioning, plausibly mediated through a mechanism that involves a direct interaction.
MiR-199a Targets Sirt1
Intriguingly, Sirt1, a class III histone deacetylase and a longevity gene, is another miR-199a predicted target. Figure 5a shows a conserved alignment between the 2 molecules. Inclusion of this target sequence within the 3′UTR of a luciferase gene rendered it a target of miR-199a, as demonstrated by the inhibition of its activity on overexpression of miR-199a, relative to a mutant sequence (Figure 5b). In concordance, overexpression of miR-199a reduced endogenous Sirt1 by 50%, whereas its knockdown enhanced its expression 2.2 times (Figure 5c). This suggested that Sirt1 should increase during HPC as a result of the reduction in miR-199a. We found that this was indeed the case, where Sirt1 was upregulated 9 times after HPC and was completely reversed by replenishing miR-199a. However, unlike Hif-1α, there was no increase in Sirt1 during prolonged hypoxia (see Figure 5d and 5g). An increase in Sirt1 by resveratrol was also inhibited by overexpression of miR-199a and was associated with upregulation of Hif-1α. The results suggest that Sirt1 plays a role during HPC but not prolonged hypoxia.
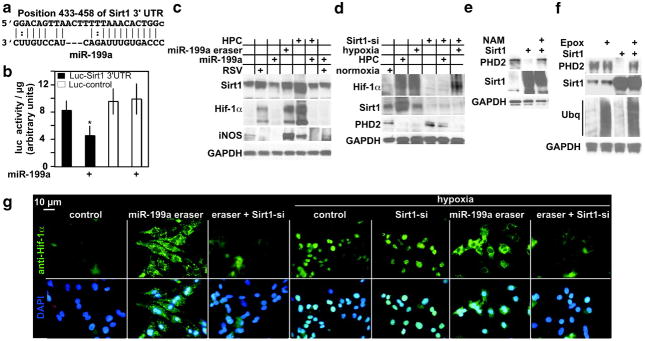
Sirt1 is a direct target of miR-199a, is upregulated during HPC, and is required for downregulation of PHD2. a, The alignment between M musculus miR-199a and a 3′UTR region of Sirt1. b, The miR-199a target site, or a mutant, was cloned into the 3′UTR of a luciferase gene (represented in the graph by black and white bars, respectively). These constructs were delivered to myocytes via adenovirus, in addition to exogenous miR-199a (where marked by +) or a control virus (n = 6). After 24 hours, luciferase activity was measured, averaged, and plotted. The y axis represents arbitrary luciferase activity normalized to micrograms of protein content. Error bars represent SEM. *P<0.01, miR-199a–treated, luciferase-Sirt13′UTR target vs control. c, Myocytes were treated with 40 μmol/L resveratrol (RSV) for 24 hours or HPC, with or without exogenous miR-199a for an additional 24 hours, or with miR-199a eraser for 24 hours, where indicted by + signs (n = 3). Protein was then extracted and analyzed by Western blotting. d, Myocytes were treated with Sirt1 short interfering RNA (Sirt1-si) adenovirus for 48 hours. These cells were then exposed to hypoxia for 24 hours or HPC, where indicated by +signs. Protein was then extracted and analyzed by Western blotting (n = 3). e, Myocytes were treated with a control or Sirt1-overexpressing virus in the absence or presence of 20 mmol/L nicotinamide (NAM). Protein was extracted and analyzed by Western blotting (n = 3). f, Myocytes were treated with a control or Sirt1-overexpressing virus in the absence or presence of 0.1 μmol/L epoxomicin (Epox) for 24 hours. Protein was extracted and analyzed by Western blotting (n = 3). g, Myocytes were plated on gelatin-coated glass chamber slides. Cells were treated with a control, miR-199a eraser, or a Sirt1 short interfering RNA (Sirt1-si)-expressing adenovirus for 48 hours, followed miR-199a eraser. A parallel set of similarly treated slides was then exposed to 24 hours of hypoxia, as indicated above. Cells were then fixed and stained with anti-Hif-1α (green) and DAPI (blue) (n = 3).
Sirt1-Induced Downregulation of PHD2 Is Required for Hif-1α Accumulation
To examine the role of Sirt1 during HPC, we used a loss-of-function approach. Unexpectedly, knockdown of Sirt1 resulted in loss of Hif-1α (Figure 5d). This led us to speculate that Sirt1 may be regulating Hif-1α expression through regulating PHD2. Western blot analysis shows that the downregulation of PHD2 during HPC was blocked by the loss of Sirt1. On the other hand, Sirt1 did not increase during prolonged hypoxia nor did its knockdown influence upregulation of Hif-1α or downregulation of PHD2. Thus, Sirt1 is necessary for ablation of PHD2, but only during HPC. To determine whether it is sufficient, we overexpressed wild-type Sirt1 in myocytes. The results of this experiment show >90% knockdown of PHD2 that was reversed by 20 mmol/L nicotinamide, which inhibits the NAD-dependent deacetylase activity of Sirt1 (Figure 5e) and by the proteasomal inhibitor epoxomicin (Figure 5f). In addition, Sirt1 knockdown inhibited eraser-induced Hif-1α (Figure 5g). Conversely, hypox-ia-induced Hif-1α, which is predominantly nuclear, was unaffected, except when the cells were pretreated with miR-199a eraser first. Thus, Sirt1 is necessary during HPC, and sufficient, for downregulating PHD2, and the effect is dependent on its deacetylase activity and proteasomal degradation.
MiR-199a Is Downregulated During IPC in Porcine Hearts
Lastly, we examined whether miR-199a, Hif-1α, and Sirt1 are regulated during early IPC in vivo. For that purpose, we induced IPC in porcine hearts and analyzed the tissue by Northern and Western blots. Figure 6a shows that miR-199a was reduced to undetectable levels in the preconditioned area of the heart, whereas the remote area exhibited modest downregulation of miR-199a, relative to a sham-operated heart. This was associated with upregulation of Hif-1α and Sirt1, as predicted. Moreover, when knocked down in isolated adult rat myocytes, miR-199a derepressed Hif-1α and Sirt1 expression, proving that miR-199a is intrinsic to adult myocyte (Figure 6b).

MiR-199a is downregulated during IPC in porcine hearts and is associated with upregulation of Hif-1α and Sirt1. a, Porcine hearts were preconditioned via 2×10-minute cycles of ischemia/reperfusion of the left ventricle (n = 3). A second set of animals was subjected to a sham operation. The IPC area of the left ventricle, remote zone, and sham-operated ventricles were immediately dissected (early/first window IPC) and analyzed by Northern and Western blotting. The top 2 gels are the results of a Northern blot, and the lower 3 gels are Western blots. b, Cultured adult rat cardiac myocytes were treated with miR-199a eraser for 24 hours or HPC. Protein was extracted and analyzed by Western blotting for the molecules indicated (n = 3).
Discussion
The results unveil a unique aspect of miRNA function, serving as molecular switches that trigger an immediate change in gene expression in response to a stimulus. Here, we show that miR-199a is sensitive to low oxygen levels and is rapidly reduced to undetectable levels, thereby releasing mRNA targets from its inhibitory effect. We concluded that this was a posttranscriptional event, because it did not affect miR-199a*, which is expressed from the same stem-loop precursor. We also show that it was not a generalized effect, because we did not observe any changes in miR-1. Moreover, after longer periods of hypoxia or ischemia, miR-199a precursor started to accumulate, suggesting that its transcription and primary transcript processing were unaffected by hypoxia. On the other hand, processing of the stem–loop precursor was inhibited. There is indeed accumulating evidence that miRNAs are widely regulated by posttranscriptional events.3–5 Our data further suggest that selective miRNA stability and processing of the stem–loop are subject to regulation in response to external stimuli.
Hif-1α is the “master transcriptional regulator” of hypoxiainduced gene expression. It is regulated by a posttranscriptional oxygen-sensitive mechanism that triggers its prompt expression on a drop in oxygen levels. PHDs hydroxylate Hif-1α during normoxia,28 which allows von Hippel–Lindau to bind and ubiquitinate Hif-1α, marking it for proteasomal degradation.29 This process is inactivated during hypoxia, thus, permitting rapid accumulation of Hif-1α. Our results introduce miR-199a as an obligatory regulator of this process. We show that miR-199a directly targets and inhibits translation of Hif-1α mRNA during normoxia. This not only ensures suppression of Hif-1α during normoxia but also circumvents the need for perpetual energy consumption required for its proteosomal degradation. Conversely, downregulation of miR-199a is required for upregulation of Hif-1α during hypoxia or HPC. However, when we knocked down miR-199a during normoxia, we did not expect that it would be sufficient for inducing Hif-1α expression, because this would also require inhibition of PHD2. Surprisingly, we observed a robust increase in its protein, which indicated that miR-199a effects were mediated through a broader range of targets.
PHD2 is the primary PHD family member that hydroxylates Hif-1α during normoxia.30 PHDs in general require O2, 2-oxoglutarate, and ascorbic acid for their full catalytic activity, and, thus, the availability of these factors regulates their function. On the other hand, the regulation of PHD2 protein availability during hypoxia has not been reported. In cardiac myocytes the level of PHD2 during hypoxia remains unexamined. Our results show that HPC or hypoxia induces downregulation of PHD2 in cardiac myocytes, which is dependent on the reduction in miR-199a levels. Unexpectedly, we discovered that Sirt1 is a direct target of miR-199a and mediates downregulation of PHD2 during HPC, through a NAD-dependent deacetylase function. Although there are no previous reports on its involvement in hypoxia or HPC, its activator, resveratrol, was reported to mediate preconditioning of the heart,31 brain,32 and kidney,33 against hypoxic damage.
Hif-1α and its targets are generally considered mediators of late preconditioning versus early preconditioning in the heart. This idea was supported by earlier findings that showed that de novo protein synthesis was not required for IPC.34 These results have since been challenged by other studies that demonstrated an opposite outcome.6,35 In concordance, Cai et al recently showed that mice heterozygous for Hif-1α fail to exhibit early preconditioning,15 whereas Eckle et al reported that knockdown of Hif-1α abolished the effect of early ischemia preconditioning.16 But the mechanism for Hif-1α–mediated early preconditioning remains obscure. Because early preconditioning occurs immediately after brief episodes of hypoxia/reoxygenation, it is unlikely that it involves transcriptional events. Indeed, Rowland et al showed that de novo mRNA synthesis is not required for IPC.6 Interestingly, immunostaining of the myocytes for Hif-1α revealed its preferential localization to the cytosol in a punctate appearance, but only during HPC or miR-199a eraser treatment. We, thus, predicted, and, later, confirmed that it associates with mitochondria under these conditions. Although it is unclear what its role there may be, we do know that it is required for HPC-mediated mitochondrial protection (Figure 4b). It is worth mentioning that Hif-1α mediates adaptive mitochondrial autophagy through a Bnip3-dependent mechanism.36 Thus, it would be of interest to examine whether the direct association of Hif-1α with mitochondria plays a role in this process.
Acknowledgments
Sources of Funding
This study was supported in part by NIH grants 2R01 HL057970 and R01 HL081381 (to M.A.); P01HL069020 to (D.E.V. and S.F.V.); and 1R01AG028787 (to J.S.).
References
Full text links
Read article at publisher's site: https://doi.org/10.1161/circresaha.108.193102
Read article for free, from open access legal sources, via Unpaywall:
https://www.ahajournals.org/doi/pdf/10.1161/CIRCRESAHA.108.193102
Free after 12 months at intl-circres.ahajournals.org
http://intl-circres.ahajournals.org/cgi/content/full/104/7/879
Free to read at intl-circres.ahajournals.org
http://intl-circres.ahajournals.org/cgi/content/abstract/104/7/879
Free after 12 months at intl-circres.ahajournals.org
http://intl-circres.ahajournals.org/cgi/reprint/104/7/879.pdf
Citations & impact
Impact metrics
Citations of article over time
Alternative metrics
Smart citations by scite.ai
Explore citation contexts and check if this article has been
supported or disputed.
https://scite.ai/reports/10.1161/circresaha.108.193102
Article citations
miRNA in the Diagnosis and Treatment of Critical Limb Ischemia.
Biomedicines, 12(9):2026, 04 Sep 2024
Cited by: 0 articles | PMID: 39335540 | PMCID: PMC11428243
The role of deacetylase SIRT1 in allergic diseases.
Front Immunol, 15:1422541, 16 Jul 2024
Cited by: 1 article | PMID: 39081309 | PMCID: PMC11286408
Review Free full text in Europe PMC
Physiological and cellular mechanisms of ischemic preconditioning microRNAs-mediated in underlying of ischemia/reperfusion injury in different organs.
Mol Cell Biochem, 13 Jul 2024
Cited by: 0 articles | PMID: 39001984
Review
MicroRNAs as Regulators of Radiation-Induced Oxidative Stress.
Curr Issues Mol Biol, 46(7):7097-7113, 06 Jul 2024
Cited by: 0 articles | PMID: 39057064 | PMCID: PMC11276491
Review Free full text in Europe PMC
Cardiovascular Disease and miRNAs: Possible Oxidative Stress-Regulating Roles of miRNAs.
Antioxidants (Basel), 13(6):656, 27 May 2024
Cited by: 0 articles | PMID: 38929095
Review
Go to all (406) article citations
Data
Data behind the article
This data has been text mined from the article, or deposited into data resources.
BioStudies: supplemental material and supporting data
Similar Articles
To arrive at the top five similar articles we use a word-weighted algorithm to compare words from the Title and Abstract of each citation.
miR-21 contributes to renal protection by targeting prolyl hydroxylase domain protein 2 in delayed ischaemic preconditioning.
Nephrology (Carlton), 22(5):366-373, 01 May 2017
Cited by: 12 articles | PMID: 27030384
Minocycline ameliorates hypoxia-induced blood-brain barrier damage by inhibition of HIF-1α through SIRT-3/PHD-2 degradation pathway.
Neuroscience, 304:250-259, 23 Jul 2015
Cited by: 40 articles | PMID: 26211444
An antagonism between the AKT and beta-adrenergic signaling pathways mediated through their reciprocal effects on miR-199a-5p.
Cell Signal, 22(7):1054-1062, 01 Mar 2010
Cited by: 58 articles | PMID: 20193759 | PMCID: PMC2872486
Hypoxia-inducible factor as a therapeutic target for cardioprotection.
Pharmacol Ther, 136(1):69-81, 16 Jul 2012
Cited by: 75 articles | PMID: 22800800
Review
Funding
Funders who supported this work.
NHLBI NIH HHS (12)
Grant ID: 2R01 HL057970
Grant ID: P01 HL069020
Grant ID: R01 HL057970-07
Grant ID: R01 HL057970-11
Grant ID: R01 HL057970-09
Grant ID: R01 HL057970
Grant ID: R01 HL057970-06A1
Grant ID: R01 HL057970-08
Grant ID: R01 HL057970-13
Grant ID: R01 HL081381
Grant ID: P01HL069020
Grant ID: R01 HL057970-12
NIA NIH HHS (2)
Grant ID: 1R01AG028787
Grant ID: R01 AG028787