Abstract
Free full text

IL-7 IN HUMAN HEALTH AND DISEASE
1. Introduction
The same mechanisms and pathways utilized by the immune system to respond to infection, lymphopenia and cancer can also predispose to autoimmunity and can be hijacked by cancer cells to support their uncontrolled proliferation. IL-7’s essential properties as a required factor for lymphocyte development and modulator of T cell homeostasis make it an attractive candidate for modulating immunodeficiency and augmenting immune based therapies, but also render it a plausible culprit in human disease. Indeed, dysregulated IL-7 signaling is a co-factor in several autoimmune diseases and in lymphoid cancers, while treatment with recombinant human IL-7 (rhIL-7) is showing promise in several clinical settings. This review will discuss known roles for IL-7 in human health (sections 2 & 3) and disease (sections 4, 6 & 7), and review results of ongoing clinical trials with rhIL7 (section 5).
2. IL-7 in Human Lymphocyte Development
2.1 IL-7 in T-Cell Development
Humans born with loss of function mutations in IL7R (IL7Rα, CD127), IL2RG (γc, CD132), or JAK3, present during infancy with a syndrome of severe combined immunodeficiency (SCID), characterized by opportunistic infection, rash, diarrhea and failure to thrive[1–4]. Without bone marrow transplantation or gene replacement therapy, the condition is fatal[1, 5]. Immunodeficiency associated with IL7R mutations results in an absence of T cells with relatively normal numbers of NK cells and B cells (T–B+NK+ SCID)[2], in contrast to SCID caused by IL2RG or JAK3 mutations, which results in a T−B+NK− phenotype, due to concomitantly impaired IL15 signaling[1]. Hypomorphic IL7R mutations result in severe T cell deficiency, whereas hypomorphic mutations in IL2RG result in a milder phenotype[6], emphasizing the essential and non-redundant role for IL7R mediated signaling in human T cell development.
2.2 IL-7 in B-Cell Development
In contrast to the severe T cell deficiency, infants with IL7R mutations have normal numbers of circulating B cells[1, 7]. The observation that IL-7 is dispensable for human B cell development has been a point of consternation, since IL-7 is required for B cell development in mice[8, 9]. Despite this clear evidence for cytokine redundancy in utero and in infancy, several lines of evidence implicate IL-7 as an important contributor to normal human B cell development. IL-7Rα expression is highly regulated during human B cell developmental stages, expressed on common lymphoid progenitors and pre-B cells, then rapidly down regulated on pro-B cells and remaining absent on mature B cells. Ex vivo cultures demonstrate potent effects of IL-7 on human early B cell progenitors[10–12], including suppression of premature Ig light-chain rearrangement in the early stages of B cell development[13]. Interestingly, IL-7 independent B cell development in a fully human ex vivo model can be accomplished using umbilical cord blood, but not when progenitors are derived from adult bone marrow[12], raising the prospect that the cytokine redundancy uncovered by congenital deficiency may not necessarily translate into a capacity for IL-7 independent generation of B cells throughout life.
Further evidence implicating a role for IL-7 during normal human B cell development comes from clinical studies where individuals are exposed to supraphysiologic levels of IL-7. In both HIV infection and idiopathic CD4+ lymphopenia, increased levels of immature/transitional B cells are observed in the peripheral blood[14, 15], at levels that are quantitatively correlated with the degree of physiologic elevations in IL-7 present. The most direct evidence for IL-7’s role in human B cell development comes from rhIL-7 induced expansion of immature/transitional B cells observed during a Phase I trial of rhIL-7[16]. RhIL-7 treated patients showed significant increases in CD19+CD10+ B cells in the peripheral blood, but no significant change in the number of mature B cells. Several patients also showed dramatic polyclonal expansion of early pre-B cells in the bone marrow, which rapidly normalized following completion of the rhIL-7 therapy. Thus, IL-7 is not absolutely required for primary B cell development in humans, but a substantial body of evidence implicates IL-7 in normal human B cell development and increased IL-7 signaling can potently expand early B cell progenitors. Not surprisingly therefore, emerging evidence implicates activating mutations in IL-7Rα in B cell precursor acute lymphoblastic leukemia (discussed in section 7.2).
3. IL-7 As a Modulator of Human T Cell Homeostasis
In addition to IL-7’s critical roles in primary lymphocyte development, murine and human studies also implicate IL-7 as an essential modulator of peripheral T cell homeostasis. Receptors for most γc cytokines are induced upon T cell activation, but the IL-7R complex is expressed continuously on most resting human T cells, with high level expression on naïve and central memory cells, lower level expression on regulatory CD4+FOXP3+ cells[17, 18], and low to absent expression on activated effectors and terminally differentiated senescent cells. Since IL-7 is continuously produced by stromal cells and circulates in picomolar concentrations[19], most human T cells are continuously exposed to IL-7 at levels sufficient to induce signaling. Murine studies demonstrate that this “trophic” IL-7 signaling provides requisite survival signals for T cell survival as adoptive transfer of cells into IL-7 deficient hosts results in severely impaired T cell survival[20]. Limitations of studies in the human model system prevent formal proof of a similar role in humans, but a substantial amount of evidence implicates IL-7 as a major modulator of peripheral T cell homeostasis in humans. The IL-7R complex is also widely expressed on a family of cells termed “innate lymphoid cells” that include populations responsible for secondary lymphoid organ development[21] as well as recently described CD4+ and CD8+ stem cell memory cells, which possess superior proliferative and renewal capacity compared to other effector/memory populations[22].
4. IL-7 during lymphopenia
Following a lymphopenia inducing insult or in the setting of congenital or acquired immunodeficiency associated with lymphopenia, patients experience elevated circulating and tissue levels of IL-7, with strong inverse correlations between circulating IL-7 levels and CD4+ T cell numbers[19, 23, 24]. Circulating IL-7 levels in healthy humans typically range from 2–8 pg/ml, but levels as high as 60 pg/ml can be observed during lymphopenia. Paradoxically, IL-7 production is actually decreased during lymphopenia due to a regulatory feedback loop mediated by IL7Rα on stromal cells, and therefore lymphopenia induced elevations result from decreased utilization[25]. Animal models demonstrate that increased IL-7 availability that occurs during lymphopenia plays a major role in mediating the enhanced cycling of naïve and memory T cells, and the increased responses to cognate and low affinity antigens seen in this setting[26–29]. Following recovery of CD4+ T cell populations, elevated IL-7 levels are cleared via IL7Rα receptor-mediated clearance and circulating levels return to normal[19, 30]. Similar inverse relationships between growth factor levels and target cell numbers are observed between erythropoietin and red blood cells[31], thrombopoietin and platelets[32] and granulocyte colony-stimulating factor (G-CSF) and neutrophils[33].
5. RhIL-7 in Clinical Trials
Direct evidence that supraphysiologic levels of IL-7 mediate alterations in human T cell homeostasis comes from studies of the effects of rhIL-7 administered in early phase clinical trials. Four Phase I trials have administered increasing doses of rhIL-7 (range 10–60 mcg/kg) to patients with refractory cancer or HIV infection[16, 34–37]. Thus far, the agent has been well tolerated, inducing only mild constitutional symptoms and no evidence for the capillary leak syndrome observed with rhIL-2 therapy [32, 33]. In each of the studies, rhIL-7 induced dose dependent increases in circulating T cell populations, with preferential expansion of recent thymic emigrants, naïve T cells and central memory T cells compared to other subsets. These changes in peripheral blood T cells reflect changes in total body T cell numbers, since FDG positron emission tomography demonstrates increased metabolic activity within secondary lymphoid organs and CT scanning demonstrates increased size of both spleen and lymph nodes at the time of peak peripheral blood expansions[35].
Patients treated with rhIL-7 show diversification of their TCR repertoire, primarily due to intense, preferential cycling of recent thymic emigrants and naïve cells, although augmentation of thymopoiesis may also contribute in some circumstances[35, 37]. The enhanced cell cycling of T cell subsets is notable. Whereas under normal conditions only approximately 5% of circulating T cells are dividing (as evidenced by Ki-67 expression), more than 50% of the circulating T cell pool becomes Ki-67+ following rhIL-7 therapy[37]. Interestingly, repetitive dosing leading to very high levels of rhIL-7 results in widespread down regulation of IL7Rα, which limits ongoing T cell cycling and provides a theoretical safety mechanism against “overdose”. RhIL-7 therapy mediates prolonged effects, likely due to both a relatively long T1/2, (range ~7–23 hours, with wide variability) as well as pharmacodynamic effects that remain well past clearance of the cytokine[16, 36]. Although cycling rates return to baseline relatively rapidly following IL7Rα down regulation and/or clearance of the cytokine, rises in circulating CD4+ and CD8+ T cell numbers remain for weeks to months after completion of rhIL-7 therapy[37].
Unlike naïve and central memory cells, some T cell subsets do not undergo substantial expansion with rhIL-7 therapy. Notably, CD4+FOXP3+ regulatory T cells show only minimal expansion, and as a result, rhIL-7 treated patients experience substantial decreases in the relative frequency of regulatory T cells within the peripheral T cell pool[34, 35]. Effector memory cells and senescent populations also show minimal expansion with rhIL-7 therapy, raising the prospect that rhIL-7 therapy could enhance T cell reactivity to chronic infection by diminishing the frequency of senescent cells.
As of 2011, at least 15 ongoing studies of rhIL-7 therapy were underway[38]. Investigators are seeking to utilize rhIL-7 to enhance viral clearance in the setting of chronic viral infection, including hepatitis B, hepatitis C and HIV infection. RhIL-7 is being tested for its capacity to augment immune reconstitution following chemotherapy and/or bone marrow transplantation and in the setting of idiopathic CD4+ lymphopenia. Trials have been initiated to test its efficacy as a vaccine adjuvant in aged populations and trials are planned to determine whether rhIL-7 therapy will augment the efficacy of tumor vaccines and adoptive T cell therapy for cancer. Together, the bulk of murine data and the emerging clinical data suggest that the IL-7 may prove clinically useful as a recombinant cytokine for a variety of immune indications, spanning immunodeficiency, chronic viral infection and cancer.
6. IL-7 as a Co-factor in Autoimmunity and Alloimmunity
Over 30 years ago, investigators identified the seemingly paradoxical coexistence of autoimmunity and immunodeficiency by demonstrating autoantibodies in a large proportion of patients with immunodeficiency[39]. It is now widely appreciated that lymphopenia often coexists with human autoimmune disease[40–42], and the hypothesis that lymphopenia predisposes hosts to autoimmunity generated by these correlative studies has been confirmed in murine models ranging from autoimmune colitis, to gastritis to diabetes[43–46]. Given the direct role that IL-7 plays in augmenting reactivity to self-antigens during lymphopenia[26, 29], it is not surprising that IL-7 has emerged as a cofactor in the development of autoimmune disease in general and that elevated IL-7 levels found in lymphopenic hosts could predispose to autoimmunity. Beyond the role that IL-7 is recognized to play in the predisposition to autoimmunity, animal models and human studies also provide evidence for IL-7 in perpetuating autoimmune inflammation. For instance, inflamed joints in rheumatoid arthritis show increased IL-7 production, perhaps as a result of TNF-α and IL-1β induced regulation[47]. Similarly, IL-7 plays a direct role in perpetuating murine colitis[48] and depletion of IL7Rα+ cells can abrogate established disease[49].
6.1 IL7R Polymorphisms and Autoimmunity
Recently, polymorphisms in IL7R, the gene encoding IL7Rα, have been shown to modulate the risk of developing several autoimmune conditions including multiple sclerosis[50, 51], ulcerative colitis[52], sarcoidosis[53] and primary biliary cirrhosis[54]. Some studies suggest a link to type 1 diabetes, but the statistical significance of this association is not strong enough to be definitive in a genome wide study [55, 56]. The risk of developing acute GVHD[57] and the risk of treatment related mortality[58] after allogeneic stem-cell transplantation has also shown some correlation with donor IL7R genotype. While all of these associations show only modest influences on disease susceptibility (RR 1.07–1.37, Table 1), taken together they provide strong evidence for an important role for IL-7 in modulating susceptibility to human autoimmune and potentially alloimmune disease.
Table 1
Several IL7R single nucleotide polymorphisms (SNPs) have been associated with autoimmune conditions in case control genome wide association studies. All associations have low odds ratios and are in strong linkage disequilibrium with the functional SNP rs6897932, suggesting they are due to a common mechanism. Percentages of co-carriage of the studied allele with the MS predisposing allele rs6897932*C and LD scores were calculated based on Hapmap data of a Utah population with northern and western European ancestry (CEU).
Disease | SNP | Risk Allele | Odds Ratio | % of haplotypes including risk allele and rs6897932*C | LD (D’) with rs6897932 |
---|---|---|---|---|---|
Multiple Sclerosis | rs6897932 | C | 1.37 | 100% | 1 |
Type 1 Diabetes | rs6897932 | C | 1.07 | 100% | 1 |
Primary Biliary Cirrhosis | rs860413 | A | 1.33 | 98.7% | 0.93 |
Ulcerative colitis | rs3194051 | G | 1.07 | 100% | 1 |
Sarcoidosis | rs10213865 | A | 1.32 | 100% | 1 |
In the genetic studies localizing IL7R susceptibility alleles, Single Nucleotide Polymorphisms (SNPs) were utilized to tag for larger genomic regions. This approach is based on the fact that SNP alleles inherited at one locus influence alleles inherited at a proximal locus, a property known as linkage disequilibrium (LD). Knowledge of LD generated through the Hapmap project has been used as the basis for Genome Wide Association studies (GWAS), as it allows a finite number of SNPs to be used as representatives for the entire genome. Which SNP is chosen as a marker for a certain region varies based on the study design and gene chip manufacturer. All of the SNPs in the IL7R region that have been associated with autoimmunity and risk after stem cell transplantation are in strong linkage disequilibrium with rs6897932, a functional SNP located in exon 6 of IL7R. The genotype that is now clearly associated with an increased susceptibility to multiple sclerosis contains a cytosine at rs6897932 (rs6897932*C), whereas a thymine base in the same location is protective (odds ratio ~0.8), and heterozygosity at this allele renders a risk intermediate between the two (odds ratio ~1.0). Rs6897932 is a non-synonymous SNP affecting whether amino acid 244 in IL7Rα is transcribed as a threonine (rs6897932*C genotype) or an isoleucine (rs6897932*T genotype). Amino acid 244 is located in exon 6, at the exact location where splicing of the full-length protein occurs during posttranslational processing and the IL7R genotype impacts the efficiency of exon 6 splicing. As a result, individuals with a CC genotype at Rs6897932 (and thus increased susceptibility to autoimmune disease) show higher levels of the spliced IL7R isoform compared to individuals with a TT genotype. Moreover, individuals with a CC genotype have been reported to have higher levels of circulating IL7Rα, consistent with a model wherein splicing efficiency determines levels of circulating soluble IL7Rα[51, 59, 60].
Despite findings in numerous studies demonstrating modulation of susceptibility to autoimmune disease based upon IL7R polymorphisms, the mechanisms whereby these genetic variations alter risk remain to be elucidated. Most hypotheses rest upon the presumption that modulation in autoimmunity risk is a result of modulation in soluble IL7Rα levels, although this has not been definitively demonstrated. Recent work using a recombinant fusion protein consisting of the IL7Rα extracellular domain linked to a human IgG1 Fc chain showed lowered IL-7 induced STAT5 phosphorylation in human T-cells in vitro in the presence of the fusion protein[61, 62]. The authors suggested that the fusion protein is an accurate mimic of soluble receptor, which if true, would suggest that increased levels of circulating IL7Rα primarily serve to inhibit IL-7 signaling. In order for such a regulator to predispose to autoimmunity, one would have to invoke regulatory effects of IL-7, which have been demonstrated[63], but have not been considered the major effect of IL-7 signaling and occur simultaneously with activating aspects on T cells. An alternative hypothesis is that IL-7 signaling is increased or potentiated in the presence of circulating IL7Rα, similar to the immune potentiating effects of soluble IL15Rα and soluble IL2Rα on cytokine signaling[64, 65].
Given the immune stimulatory properties of IL-7 discussed above, one could easily envision a scenario wherein potentiation of IL-7 signaling could promote increased survival and peripheral expansion of autoreactive T-cell clones leading to increased susceptibility to autoimmune disease. Indeed, recent studies showing potentiation of IL-7 induced antitumor effects when IL-7 is administered as an IL-7:IL7Rα extracellular domain complex are consistent with this model [57]. We await future studies that more clearly delineate the biology of soluble IL7Rα and shed more light on the biology responsible for these intriguing genetic observations. Regardless of the exact biology at play, the fact that the allele associated with increased autoimmunity is a common variant, raises the prospect of hereditary pressure against the opposite, protective allele. It is plausible that genetic mechanisms within the IL7 axis that increase the risk of autoimmunity may have provided an evolutionary benefit in fighting infections or cancer.
6.2 IL-7 in Graft Versus Host Disease
Graft-versus-host disease represents one of the major morbidities complicating hematopoietic stem cell transplantation (HSCT). The alloantigens that drive pathology in this setting are generally stronger stimuli than the self-antigens that drive autoimmune disease, but there is significant overlap between the pathophysiology of GVHD and autoimmunity. Patients undergoing hematopoietic HSCT universally experience lymphopenia and elevated IL-7 levels as part of the procedure, raising the prospect that IL-7 could contribute to morbidity in this setting[19, 66]. Further, murine models demonstrate that IL-7 is required for the development of GVHD[67], that neutralization of IL-7 can prevent GVHD[68] and that administration of rhIL7 lowers increases the risk for developing GVHD[69]. It is perhaps not surprising therefore that those genetic and/or environmental variables, which modulate IL-7 biology, might also modulate risk in this setting. Indeed, the risk of GVHD is increased with higher IL-7 levels post-SCT[70, 71]. Furthermore, some groups have noted that IL7R polymorphisms correlate with the risk of GVHD and treatment related mortality following stem cell transplantation[58, 72], although this has not been confirmed in other studies. Despite that an effect of the exon 6 SNP (rs6897932) was not observed in these studies, the polymorphisms that showed a positive association are in strong LD with rs6897932. Thus, further studies are required to clarify whether sIL7Rα contributes to the risk of GVHD or whether the genetic association observed relate to an entirely different mechanism.
Despite the potential for IL-7 to mediate adverse effects in the setting of GVHD, it is important to note that the potent immunorestorative effects of this agent could also potentially mediate benefit. IL-7 therapy potently augments immune reconstitution in the context of syngeneic or T cell depleted HSCT[73, 74], where GVHD is much less likely and impaired immune reconstitution remains is a major cause of morbidity and mortality. Because of this, a clinical trial is currently underway to determine whether rhIL-7 can augment immune reconstitution and diminish morbidity following stem cell transplantation.
7. IL7R and CRLF2 as Oncogenes
IL7Rα’s involvement in oncogenesis is closely linked to CRLF2 (also known as TSLPR), therefore both receptors will be discussed in this section. Signaling of IL-7 and TSLP is complex and the details continue to be elucidated. Briefly, IL7Rα associates with IL-2Rγ (CD132) to form the IL-7 receptor complex, and IL7Rα associates with CRLF2 to form the thymic stromal lymphopoietin receptor complex. IL7Rα associates with JAK1, IL- 2Rγ associates with JAK3, while CRLF2 associates with JAK2[75]. As a result, IL-7 signals via JAK1 and JAK3 to mediate phosphorylation of STAT1, STAT3 and STAT5, while TSLP signals via JAK1 and JAK2 to phosphorylate STAT5. Given the important roles of IL-7 and TSLP in survival and proliferation of developing B cells and T cells, it is not surprising that the potent growth pathways mediated by signaling through these receptors would be hijacked by malignancies comprising these populations.
7.1 CRLF2 mutations in BCP-ALL
B cell precursor acute lymphoblastic leukemia (BCP-ALL) is the most common malignancy of childhood, and results from clonal expansion of early pre-B cells. IL7Rα is constitutively expressed in BCP-ALL cells, as IL7Rα is also expressed on the normal counterpart. IL-7 stimulates proliferation of BCP-ALL leukemia cells in vitro and in vivo, and IL-7 signaling reverses rapamycin induced growth inhibition of BCP-ALL cells[76]. Recently several mutations in TSLP and IL-7 signaling pathways have been discovered in BCP-ALL (Figure 1A). The first to be reported were mutations in JAK1 and JAK2[77–80], with a higher frequency of mutations reported in JAK2, which associates with CRLF2[75]. Subsequently, translocations and mutations in CRLF2 itself were discovered[81–84]. CRLF2 mutations often involved the substitution of a cysteine for a phenylalanine at amino acid 232 (F232C), resulting in constitutive CRLF2 dimerization and cytokine independent growth (Figure 1A, II)[81, 82]. JAK2 and CRLF2 mutations are mutually exclusive, providing evidence for involvement in the same oncogenic pathway[81, 82]. Both F232C CRLF2 mutations and JAK2 mutations are associated with CRLF2 over expression, which can be detected by flow cytometry. In total, CRLF2 is over expressed in approximately 10–15% of adult and pediatric BCP-ALL, with a higher frequency in patients with high-risk disease and in BCP-ALL associated with Down’s syndrome[81, 82]. Because patients with JAK2 and CRLF2 mutations have more aggressive disease and inferior survival, it is possible that screening for this mutation, perhaps via identification of overexpression of CRLF2 expression, could become an important component in assessing prognosis and/or stratifying therapy for BCP-ALL (Figure 1A, I). Moreover, because both groups demonstrate over expression of CRLF2, it is possible that therapies could be developed for these high-risk groups based upon targeting of CRLF2.
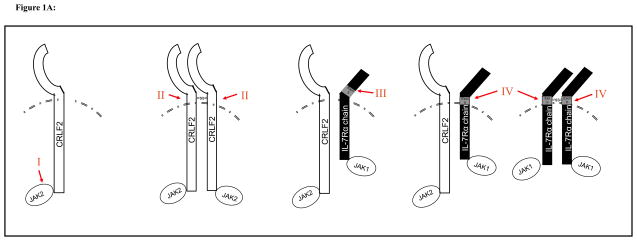
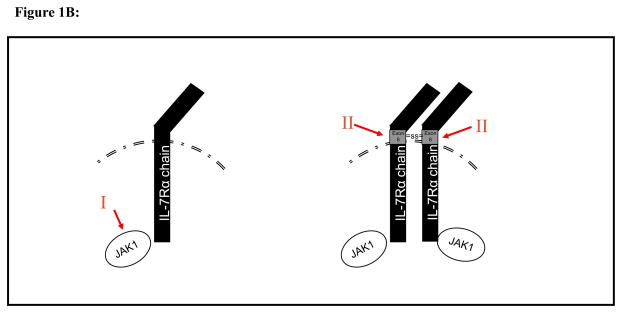
Figure 1A. BCP-ALL: CRLF2/IL7R axis mutations occurs in 10–15% of cases, is associated with CRLF2 overexpression and a poor prognosis
I. Activating JAK2 mutations are found in approximately 8% and are always associated with over expression of CRLF2.
II. CRLF2 mutations, most commonly F232C or complex insertions and deletions, encode for an unpaired cysteine in the juxtamembrane domain, leading to homodimerization and CRLF2 activation.
III. IL7R S185C mutations found in the juxtamembrane domain (exon 5), are typically associated with overexpression of CRLF2.
IV. IL7R insertion and/or deletion mutations in the transmembrane domain (exon 6) encode for an unpaired cysteine, leading to homodimerization and IL7R activation. In BCP-ALL, this mutation is typically associated with overexpression of CRLF2.
Figure1B: T cell-ALL: IL7R axis mutations occurs in ~25% of cases, is not associated with CRLF2 expression and does not impact on prognosis.
I. Activating JAK1 mutations leading to ligand independent signaling ( ~15% of cases)
II. IL7R mutations in exon 6 encoding for an upaired cysteine leads to homodimerization and ligand-, gc- and CRLF2-independent signaling. (~10%)
7.2 IL7R mutations in B-ALL
In addition to an emerging role for CRLF2 signaling in BCP-ALL pathogenesis, gain of function mutations in IL7R have also recently been found in BCP-ALL[85]. Shochat et al. screened 286 BCP-ALL bone marrow samples for IL7R mutations. Among leukemias with CRLF2 over expression, IL7R mutations were found in 6% vs. 0.6% in those without CRLF2 over expression. Two types of gain-of-function mutations were identified in IL7R. Four patients exhibited substitution of a cysteine for serine at position 185 (S185C) in the juxtamembranous portion of the extracellular domain in exon 5 (Figure 1A, III), while five patients showed of in-frame insertions and/or deletions which included the addition of an unpaired cysteine within the transmembrane domain encoded by exon 6 (Figure 1A, IV). To study the function of the mutated IL7R, the S185C mutant IL7R was introduced into BAF3, an IL-3 dependent mouse pro-B cell line, generating cell lines that expressed wild type or mutant IL7Rα with or without CRLF2. S185C IL7R mediated constitutive, ligand independent activation via homodimerization involving disulfide bonds resulting from cysteine-cysteine interactions. Constitutive activation was abrogated when the cysteine was replaced by a glycine. The addition of a cysteine in the juxtamembranous region of a cytokine receptor as a mechanism for generating constitutive activation has also been observed in CRLF2 (discussed above) as well as other leukemias (e.g. erythropoietin receptor), raising the prospect that this is a common mechanism for mutational activation of receptor tyrosine kinases in leukemia[86, 87].
Interestingly, while nearly all of the BCP-ALL mutations were associated with co-expression of CRLF2, and CRLF2 over expressing BCP-ALL cells continued to respond to TSLP by phosphorylating STAT5, studies thus far do not demonstrate response to exogenous IL-7[88]. Furthermore, Shochat et al found that S185C IL7R mediated cytokine independent growth only when expressed with CRLF2. Thus, IL7R mutations occur in a small fraction of all cases of BCP-ALL, and appear to mediate IL-7 independent signaling. IL7R mutations found in BCP-ALL are typically associated with CRLF2 over expression, which appears to retain the capacity to respond to CRLF2 and likely cooperates with the mutant IL7R in BCP-ALL oncogenesis.
7.3 IL7R mutations in T-ALL
T cell acute lymphoblastic leukemia (T-ALL), a common leukemia of children and young adults, is comprised of clonal IL7Rα cells derived from transformation of triple negative thymocytes. Previous work demonstrating that T-ALL cells respond to IL-7 in vitro[89–91] and JAK1 mutations occur in >15% of cases of adult T-ALL (Figure 1B, I), had implicated the IL7R axis in this disease[92]. Recently, two groups sequenced IL7R genes in several T-ALL cohorts and a found a mutation rate approximating 10%[85, 93]. Similar to the IL7R mutations found in BCP-ALL, nearly all encoded for a mutant, unpaired cysteine in the transmembrane domain of exon 6 (Figure 1B, II). Unlike the results in BCP-ALL however, the vast majority of T-ALL samples were not associated with CRLF2 expression. Furthermore, the T-ALL IL7R gain-of-function mutations were capable of transforming cells, regardless of the presence or absence of CRLF2[85] or IL-2Rγ and JAK3[93]. Consistent with this, mutant IL7R expressing T cells show activation of JAK1 and STAT5, without activation of JAK2 or JAK3. Pharmacological inhibition of the JAK1 and STAT5 induced tumor cell death in vitro and diminished tumor growth rate in vivo, raising the prospect that agents targeting IL7Rα, JAK1 or STAT5 could be used for specific therapy of these tumors[93]. In summary, mutant IL7R is found not uncommonly in T-ALL, where it functions independently of CRLF2 and IL-2Rγ to induce transformation.
8. Conclusion
Since the discovery of IL-7 over 20 years ago, a substantial body of basic research has elucidated fundamental physiologic roles for this cytokine, including non-redundant roles in lymphocyte and lymph node development and peripheral T cell homeostasis. More recently, the IL-7 axis has been implicated in the pathophysiology of a variety of diseases, including effects of germline variation and somatic mutations in IL7Rα in autoimmunity and cancer. This knowledge base paves the way for current and future translational and clinical studies, including clinical trials of rhIL-7 that are currently underway in cancer, HIV, stem cell transplantation and chronic viral infection as well as anticipated studies that seek to inhibit IL7R signaling in the context of cancer and autoimmune disease.
Footnotes
Publisher's Disclaimer: This is a PDF file of an unedited manuscript that has been accepted for publication. As a service to our customers we are providing this early version of the manuscript. The manuscript will undergo copyediting, typesetting, and review of the resulting proof before it is published in its final citable form. Please note that during the production process errors may be discovered which could affect the content, and all legal disclaimers that apply to the journal pertain.
References
Full text links
Read article at publisher's site: https://doi.org/10.1016/j.smim.2012.02.005
Read article for free, from open access legal sources, via Unpaywall:
https://europepmc.org/articles/pmc3358500?pdf=render
Citations & impact
Impact metrics
Article citations
Navigating the Modern Landscape of Sepsis: Advances in Diagnosis and Treatment.
Int J Mol Sci, 25(13):7396, 05 Jul 2024
Cited by: 0 articles | PMID: 39000503
Review
Lrp10 suppresses IL7R limiting CD8 T cell homeostatic expansion and anti-tumor immunity.
EMBO Rep, 25(8):3601-3626, 02 Jul 2024
Cited by: 1 article | PMID: 38956225 | PMCID: PMC11315911
TEMPORAL CHANGES IN INNATE AND ADAPTIVE IMMUNITY DURING SEPSIS AS DETERMINED BY ELISPOT.
Shock, 62(2):255-264, 03 May 2024
Cited by: 0 articles | PMID: 38754032
Causal relationship between immune cells and prostate cancer: a Mendelian randomization study.
Front Cell Dev Biol, 12:1381920, 19 Mar 2024
Cited by: 4 articles | PMID: 38566827 | PMCID: PMC10985200
Identification and validation of immune-related genes in osteoarthritic synovial fibroblasts.
Heliyon, 10(7):e28330, 24 Mar 2024
Cited by: 0 articles | PMID: 38571590 | PMCID: PMC10988018
Go to all (92) article citations
Data
Data behind the article
This data has been text mined from the article, or deposited into data resources.
BioStudies: supplemental material and supporting data
SNPs (4)
- (7 citations) dbSNP - rs6897932
- (1 citation) dbSNP - rs860413
- (1 citation) dbSNP - rs3194051
- (1 citation) dbSNP - rs10213865
Similar Articles
To arrive at the top five similar articles we use a word-weighted algorithm to compare words from the Title and Abstract of each citation.
IL-7 therapy dramatically alters peripheral T-cell homeostasis in normal and SIV-infected nonhuman primates.
Blood, 101(6):2294-2299, 31 Oct 2002
Cited by: 155 articles | PMID: 12411295
Interleukin-7: Fuel for the autoimmune attack.
J Autoimmun, 45:40-48, 04 Jul 2013
Cited by: 56 articles | PMID: 23831438
Review
Interleukin-7 Biology and Its Effects on Immune Cells: Mediator of Generation, Differentiation, Survival, and Homeostasis.
Front Immunol, 12:747324, 02 Dec 2021
Cited by: 71 articles | PMID: 34925323 | PMCID: PMC8674869
Review Free full text in Europe PMC
Soluble IL7Rα potentiates IL-7 bioactivity and promotes autoimmunity.
Proc Natl Acad Sci U S A, 110(19):E1761-70, 22 Apr 2013
Cited by: 88 articles | PMID: 23610432 | PMCID: PMC3651437
Funding
Funders who supported this work.
Intramural NIH HHS (2)
Grant ID: Z01 BC011069-01
Grant ID: Z01 BC011069