Abstract
Free full text

A Short Segment of the HIV-1 gp120 V1/V2 Region Is a Major Determinant of Resistance to V1/V2 Neutralizing Antibodies
Abstract
Antibody PG9 is a prototypical member of a class of V1/V2-directed antibodies that effectively neutralizes diverse strains of HIV-1. We analyzed strain-specific resistance to PG9 using sequence and structural information. For multiply resistant strains, mutations in a short segment of V1/V2 resulted in gain of sensitivity to PG9 and related V1/V2 neutralizing antibodies, suggesting both a common mechanism of HIV-1 resistance to and a common mode of recognition by this class of antibodies.
TEXT
Monoclonal antibodies (MAbs) capable of effectively neutralizing diverse strains of HIV-1 have been isolated from a number of HIV-1-infected individuals. One recently identified class of such antibodies recognizes an epitope primarily in the V1/V2 region of HIV-1 gp120, requires an N-linked glycan at residue 160, and generally binds with much higher affinity to membrane-associated trimeric forms of Env than to monomeric forms of gp120 (4, 15). Members of this class include PG9 and the somatically related PG16, as well as antibodies CH01 to CH04 and PGT141 to PGT145 from two other donors (1, 14, 15). Antibody PG9 is one of the most broadly cross-reactive members of the class and neutralizes 70 to 80% of diverse HIV-1 isolates (3, 7, 15). The structure of PG9 in complex with scaffolded forms of V1/V2 has been determined: when bound by PG9, V1/V2 adopts a 4-stranded β-sheet structure, with PG9 interacting with two glycans (at residues 156 and 160) and with one β-strand (strand C, at the sheet edge) (7). The free-antibody structures of PG9 as well as other antibodies from this class (PG16, CH04, and PGT145) are also known and suggest a common mode of Env recognition mediated primarily by the long anionic complementarity-determining region (CDR) H3 loops of these antibodies (7, 9, 10).
Previous studies indicated that virus neutralization sensitivity to PG9 might correlate with V2 length, the number and positioning of potential N-linked glycosylation sites in V1, V2, and V3, and net charge of the PG9-interacting strand C (2, 7, 11). Additionally, residues outside the structure-identified epitope—in V1/V2 as well as in V3—were found to affect PG9 and PG16 neutralization (8, 13, 15). Resistance conferred by an N160K mutation was described as a defining attribute for this class, but this residue does not account for all instances of resistance (15, 16). To gain a more complete understanding of the mechanism of naturally occurring viral resistance to PG9 and other MAbs of the class, we performed a combination of sequence and structural analyses to predict gain-of-sensitivity mutations among PG9-resistant strains. The effects of the mutations on resistance to PG9 and five other members of the V1/V2 antibody class were then assessed.
Among a panel of 172 HIV-1 Env pseudoviruses, 38 strains (22%) were found to be resistant to PG9 (3, 7). Examination of strain sequences indicated that 16 were missing the N-linked glycan at position 160, leaving a total of 134 sensitive and 22 resistant strains to be analyzed for protein sequence-based resistance signatures (Fig. 1). Initially, we focused on residues 154 to 184 of V1/V2 (HXB2-relative residue numbering), a region that spans β-strands B and C, is relatively conserved (with few insertions/deletions), and includes the entire PG9 epitope (7). Specifically, based on sequence alignments, we searched for amino acids that were preferentially found among PG9-resistant versus -sensitive strains for a given residue position (Fig. 2A). A number of such amino acids at positions at or near the PG9 interface (as observed in the crystal structure of scaffolded V1/V2) were selected for gain-of-sensitivity mutations (Fig. 2B). Each of the selected residues was mutated to amino acids commonly observed among PG9-sensitive sequences (Fig. 2A). This sequence analysis was able to identify candidate mutations for 11 of the PG9-resistant strains. However, since the selected mutations were primarily in the short segment between residues 166 and 173, which overlaps strand C of V1/V2, we swapped that 8-residue segment in 10 additional strains, as well as in five of the strains identified by the sequence analysis, with the corresponding segment from CAP45, a sensitive strain used for the PG9 crystal structure (7) (Fig. 2B). Additionally, analysis of potential N-linked glycosylation sites (PNGS) revealed that residue 128 was the location of a PNGS in the PG9-resistant strain CNE4 but not in any of the other strains in the neutralization panel. Since glycans may create substantial steric hindrance, PNGS 128 in CNE4 was also selected for gain-of-sensitivity experiments, despite a more distal position with respect to the PG9 interface in the scaffolded V1/V2 structures (Fig. 3).
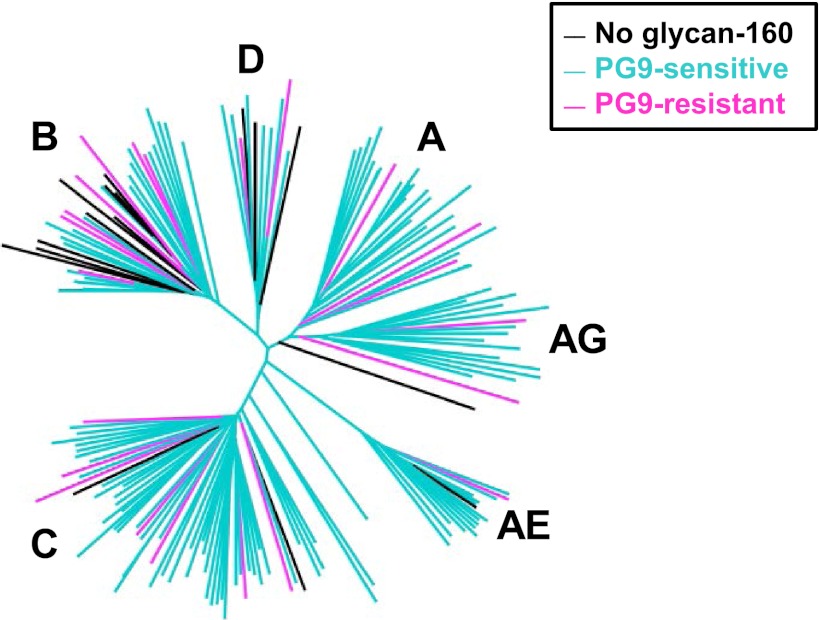
PG9 neutralization sensitivity and resistance. Neighbor-joining dendrogram constructed from full gp160 sequences of 172 virus strains representing the major HIV-1 genetic subtypes (labeled branches). Neutralization sensitivity of each Env pseudovirus is indicated by the color of the branch: PG9-resistant strains not containing a PNGS at residue 160 are in black, PG9-sensitive strains are in aqua, and all other PG9-resistant strains are in pink.
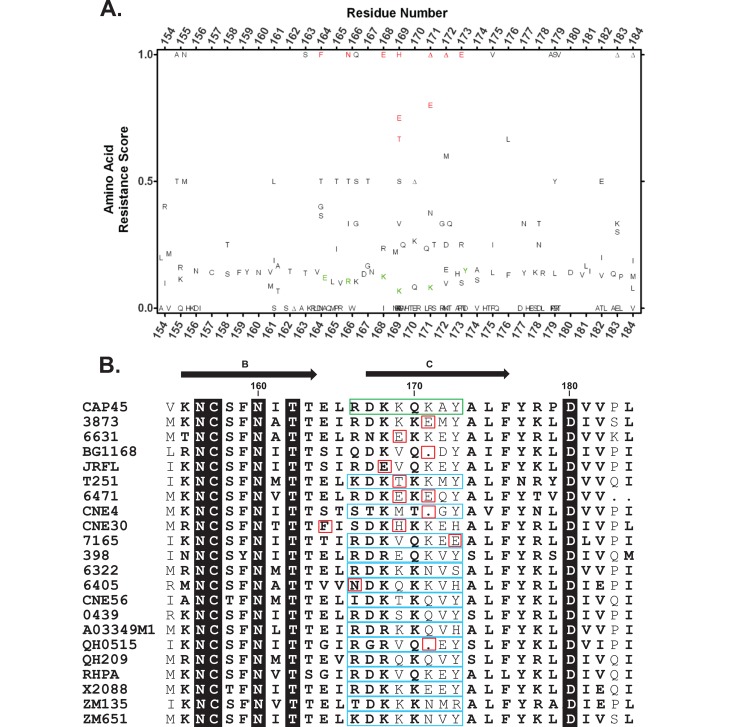
Design of gain-of-sensitivity mutants among PG9-resistant strains. (A) V1/V2 amino acid frequency analysis. Symbols correspond to the respective amino acids, with representing sequence gaps at the given position. For each residue at positions 154 to 184 (HXB2-relative numbering), the resistance score for a given amino acid (or a gap) was defined as the ratio of its number of occurrences in resistant sequences to its overall number of occurrences for the given residue position. A higher score indicates that the amino acid was preferentially found among resistant sequences, with a score of 1 indicating that the amino was found only among resistant sequences. Residues highlighted in red were selected for gain-of-sensitivity studies and were mutated to the amino acid types shown in green for the specified residue positions. (B) PG9-resistant strains selected for gain-of-function experiments, with highlighted residues selected for point-mutations (red) and/or swaps (cyan). The PG9-sensitive CAP45 sequence, used to determine the atomic structure of V1/V2, is shown as a reference, with the residue segment used in the swaps with the resistant strains highlighted in green. Strands B and C of V1/V2 shown at the top of the figure are based on the CAP45 structure. Residue positions with no variation are shown in white font on black background, while conserved residue positions are shown in bold.

Structure-based explanation of gain-of-sensitivity results for V1/V2-directed broadly neutralizing antibodies. The structure of scaffolded V1/V2 from the CAP45 strain of HIV-1 (green ribbon with labeled strands and molecular surfaces of glycans 156 and 160) is shown in complex with PG9 (yellow, heavy chain; blue, light chain). The side chains of V1/V2 residues selected for gain-of-sensitivity mutation are shown as sticks and labeled by residue number; side chains of proximal interacting residues in PG9 CDR H3 are shown as yellow sticks and labeled.
In total, 21 PG9-resistant HIV-1 isolates (three from clade A, six from B, seven from C, two from D, and one each from AE, AG, and G) were analyzed by mutagenesis and neutralization assays (Table 1; also, see Table S1 in the supplemental material). The point mutations and strand C swaps were generated by site directed mutagenesis (GeneImmune LLC, New York, NY) on Env expression plasmids (5, 6, 15, 17). Parental and mutant Envs were used to construct pseudoviruses for the single round of infection neutralization assays using TZM-bl target cells as described previously (12, 17). Each pair of parental and mutant viruses was tested against six members of the V1/V2-directed class of broadly neutralizing antibodies, isolated from three different donors: PG9 and PG16 (15), CH01 and CH04 (1), and PGT141 and PGT145 (14). In each case, the parental virus was resistant to PG9 at a 50% inhibitory concentration (IC50) of >50 μg/ml, although several were sensitive to other V1/V2 MAbs. MAbs to other epitopes (MAbs VRC01, F105, 17b, PGT128, and 4E10) were included as controls to assess the impact of the mutations on overall Env conformation and neutralization sensitivity.
Table 1
Neutralization IC50s for 21 PG9-resistant HIV-1 Env pseudoviruses and their corresponding gain-of-function mutants
Clade | Virusa | IC50 (μg/ml)b | ||||||||||
---|---|---|---|---|---|---|---|---|---|---|---|---|
V1/V2 MAbs | Control MAbsc | |||||||||||
PG9 | PG16 | CH01 | CH04 | PGT141 | PGT145 | VRC01 | F105 | 17b | PGT128 | 4E10 | ||
C | 3873 | >50 | >50 | >50 | >50 | 0.011 | 0.009 | 0.475 | >50 | >50 | 0.006 | 6.43 |
3873-E171K | 0.018 | 0.004 | 0.161 | 0.385 | 0.003 | 0.004 | 0.071 | >50 | >50 | 0.006 | 2.75 | |
C | 6631 | >50 | >50 | >50 | >50 | >50 | >50 | >50 | >50 | >50 | 4.07 | >50 |
6631-E169K | 0.225 | 0.047 | 1.66 | 0.759 | 12.3 | >50 | >50 | >50 | >50 | 4.52 | >50 | |
B | BG1168 | >50 | >50 | >50 | >50 | >50 | >50 | 0.203 | >50 | >50 | >50 | 1.84 |
BG1168-ins171K | 4.99 | 0.276 | >50 | >50 | >50 | 0.517 | 0.670 | >50 | >50 | >50 | 5.15 | |
B | JRFL | >50 | >50 | >50 | >50 | >50 | 8.77 | 0.029 | >50 | >50 | 0.01 | 6.16 |
JRFL-E168K | 0.009 | <0.003 | 0.122 | 0.095 | 0.11 | 0.017 | 0.023 | >50 | >50 | 0.006 | 4.04 | |
AG | T251 | >50 | 5.23 | 13.6 | 29.5 | >50 | 0.316 | 3.03 | >50 | >50 | >50 | 10.3 |
T251-T169K | 0.056 | 0.022 | 2.51 | 3.67 | >50 | 0.059 | 2.85 | >50 | >50 | >50 | 7.01 | |
T251-Cd | 0.210 | 0.104 | >50 | >50 | 0.009 | 0.038 | 3.35 | >50 | >50 | >50 | 16.8 | |
C | 6471 | >50 | >50 | >50 | >50 | >50 | >50 | >50 | >50 | >50 | >50 | >50 |
6471-E169K/E171K | 0.045 | 0.012 | 1.32 | 1.32 | 0.043 | 0.080 | >50 | >50 | >50 | >50 | 8.57 | |
B | CNE4 | >50 | >50 | >50 | >50 | >50 | >50 | 0.352 | 17.9 | 18.1 | 0.546 | 0.822 |
CNE4-N128T/T130D | >50 | >50 | >50 | >50 | >50 | >50 | 0.165 | 0.554 | 15.6 | 2.02 | 0.17 | |
CNE4-ins171K | 0.312 | >50 | >50 | >50 | >50 | >50 | 0.266 | 0.236 | 0.214 | >50 | 0.043 | |
CNE4-C | 0.049 | 15.9 | 36.5 | >50 | >50 | >50 | 0.321 | 0.338 | 0.198 | >50 | 0.135 | |
C | CNE30 | >50 | >50 | >50 | >50 | >50 | >50 | 0.979 | >50 | >50 | 0.19 | 4.70 |
CNE30-F164E | >50 | >50 | >50 | >50 | >50 | >50 | 0.714 | >50 | >50 | 0.417 | 13.4 | |
CNE30-F164E/H169K | 2.37 | 0.648 | >50 | >50 | >50 | >50 | 0.686 | >50 | >50 | 0.255 | 9.51 | |
B | 7165 | >50 | >50 | >50 | >50 | 2.15 | 0.063 | 26.6 | >50 | >50 | 0.004 | 1.79 |
7165-E173Y | 0.603 | 0.045 | 1.07 | 2.45 | 0.151 | 0.029 | >50 | >50 | >50 | 0.004 | 3.21 | |
7165-C | 0.020 | 0.008 | 0.209 | 0.608 | >50 | 0.031 | >50 | >50 | >50 | <0.003 | 1.75 | |
A | 398 | >50 | >50 | 0.266 | 0.463 | >50 | >50 | 0.144 | >50 | >50 | <0.003 | 8.56 |
398-C | 0.004 | 0.003 | 0.059 | 0.095 | 0.144 | 0.38 | 0.081 | >50 | >50 | <0.003 | 0.329 | |
C | 6322 | >50 | >50 | >50 | >50 | 0.013 | 0.105 | >50 | >50 | >50 | >50 | 11.4 |
6322-C | 0.019 | 0.008 | 0.696 | 1.14 | <0.003 | <0.003 | >50 | >50 | >50 | >50 | 7.10 | |
D | 6405 | >50 | >50 | >50 | >50 | >50 | >50 | 1.53 | >50 | >50 | 3.86 | 9.10 |
6405-N166R | >50 | >50 | >50 | >50 | 1.27 | >50 | 1.39 | >50 | >50 | 2.82 | 4.17 | |
6405-C | 0.245 | 0.195 | 2.05 | 6.39 | 0.004 | 0.117 | 0.815 | >50 | >50 | 3.64 | 1.34 | |
AE | CNE56 | >50 | >50 | >50 | >50 | >50 | 2.25 | 0.186 | >50 | >50 | 7.36 | 0.960 |
CNE56-C | 0.165 | 0.033 | 2.79 | 2.93 | 0.128 | 0.807 | 0.341 | >50 | >50 | 2.03 | 0.219 | |
A | 0439 | >50 | >50 | >50 | >50 | 3.91 | 2.00 | 0.170 | >50 | >50 | 2.57 | 6.70 |
0439-C | 4.19 | >50 | >50 | >50 | 1.54 | 2.76 | 0.116 | >50 | >50 | 2.58 | 2.50 | |
D | A03349M1 | >50 | >50 | >50 | >50 | >50 | 2.71 | 2.83 | >50 | >50 | 0.01 | 4.60 |
A03349M1-C | 1.18 | 0.181 | >50 | >50 | 0.937 | 0.059 | 0.637 | 10.4 | >50 | 0.012 | 1.27 | |
B | QH0515 | >50 | >50 | >50 | >50 | >50 | >50 | 0.11 | >50 | >50 | >50 | 3.07 |
QH0515-ins171K | >50 | >50 | >50 | >50 | >50 | >50 | 0.769 | >50 | >50 | >50 | 3.59 | |
QH0515-C | 4.58 | >50 | >50 | >50 | >50 | >50 | 1.19 | >50 | >50 | >50 | 1.65 | |
A | QH209 | >50 | >50 | >50 | >50 | >50 | 0.015 | 0.017 | >50 | >50 | >50 | 5.27 |
QH209-C | 0.39 | 0.124 | >50 | >50 | >50 | >50 | 0.025 | >50 | >50 | >50 | 4.81 | |
B | RHPA | >50 | 0.816 | >50 | 34.4 | 0.064 | 0.044 | 0.035 | >50 | >50 | 0.010 | 5.66 |
RHPA-C | 0.603 | 0.101 | 7.78 | 38.5 | >50 | 2.48 | 0.031 | >50 | >50 | 0.008 | 5.03 | |
G | X2088 | >50 | >50 | >50 | >50 | >50 | >50 | >50 | >50 | >50 | >50 | >50 |
X2088-C | 0.11 | 0.694 | >50 | >50 | >50 | >50 | >50 | >50 | >50 | >50 | >50 | |
C | ZM135 | >50 | >50 | >50 | >50 | >50 | >50 | 0.237 | >50 | >50 | 3.92 | 0.136 |
ZM135-C | 0.099 | 0.03 | >50 | >50 | 0.004 | 0.148 | 1.19 | >50 | >50 | 5.68 | 0.746 | |
C | ZM651 | >50 | >50 | >50 | >50 | >50 | 7.56 | 0.558 | >50 | >50 | 0.101 | 0.462 |
ZM651-C | 22.5 | >50 | >50 | >50 | 2.93 | 8.22 | 1.3 | >50 | >50 | >50 | 1.38 |
Mutations that changed the glutamic acid (E) to lysine (K) at position 168, 169, or 171 had the most dramatic effects on sensitivity to the V1/V2 MAbs (Table 1; also, see Table S1 in the supplemental material). For viral strains 3873, 6631, BG1168, JRFL, and T251, a single point mutation at one of these three sites was sufficient to confer sensitivity to multiple V1/V2 MAbs. For resistant strain 6471, the double mutation E169K/E171K resulted in neutralization sensitivity to all six V1/V2 MAbs tested. Point mutations had a more modest effect on some viral strains: CNE4 with an inserted 171K gained sensitivity to just PG9, and CNE30-F164E/H169K gained sensitivity to both PG9 and PG16 but no others.
These observations confirm and extend the information gained from the crystal structures of PG9 with scaffolded V1/V2 from strains ZM109 and CAP45 (7). In these structures, V1/V2 residues 168, 169, and 171 are part of the cationic V1/V2 strand C that interacts directly with a number of negatively charged residues in the CDRH3 of PG9: sulfated tyrosines Tys 100g and Tys 100h and Asp 100i and Asp 100l (Kabat residue numbering). Negatively charged residues and deletions at positions 168, 169, and 171 likely disturb interactions and/or create charge repulsion with PG9 CDRH3 (Fig. 3). This interpretation is consistent with a recent study showing a correlation of net positive charge in V2 strand C with sensitivity to PG9 and PG16 (11). Similarly, mutagenesis studies have found that K169E confers resistance to PG9 and PG16, while the less drastic K171A mutation had a more moderate effect on neutralization by these antibodies (8, 13, 15).
Additional positions in strand C also affected sensitivity to V1/V2 antibodies. The E173Y mutation in 7165 effectively conferred sensitivity, in agreement with previous results showing loss of neutralization of Y173A in JR-CSF for both PG9 and PG16 (15). E173Y could potentially stabilize the positioning of glycan-156 and may thus have an indirect effect on interactions with PG9 (Fig. 3), though the mechanism of action of this mutation is less clear than that of the 168, 169, and 171 mutations.
Replacement of an 8-residue segment (residues 166 to 173, overlapping strand C) with the corresponding segment from CAP45 also conferred complete V1/V2 MAb sensitivity to strains 398, 6322, 6405, and CNE56. Sensitivity to at least one MAb, including PG9, was observed for the CAP45 C-strand chimeras of 0439, A03349M1, QH0515, QH209, RHPA, X2088, ZM135, and ZM651. For three of the strains for which both point mutants and CAP45 C-strand chimeras were tested, the strand C swap had the more dramatic effect. Strain CNE4 was resistant to all six MAbs, the PNG removal mutant CNE4-N128T.T130D had no effect on neutralization resistance, and CNE4-ins171K gained sensitivity only to PG9, but the CAP45 strand-C chimera was sensitive to PG9, PG16, and CH01. Similarly, on strain 6405, the point mutant N166R only gained sensitivity to PGT141, possibly indicating additional interactions with the longer PGT141 penetrating loop (7, 14), which may extend further toward the 166 region than that of PG9 (Fig. 3). In contrast, the CAP45 strand C swap provided sensitivity to all six MAbs. Finally, the point mutation in QH0515-ins171K had no effect on sensitivity, but the CAP45 strand C chimera conferred PG9 neutralization.
Paradoxically, in four cases, while the CAP45 strand C chimeras gained sensitivity to PG9 and PG16, a gain of resistance was noted for CH01 and CH04 (strain T251), PGT141 (RHPA and 7165), and PGT145 (QH209). This observation suggests that, despite overall similarity in the epitope recognized and the requirement for the N160 glycan, there is some variation in the mode of recognition by members of the V1/V2 class of neutralizing MAbs.
The mutations tested here did not cause global alterations in the neutralization sensitivity as assessed by MAbs to non-V1V2 epitopes (Table 1; also, see Table S1 in the supplemental material). The one exception was strain CNE4, for which the mutants increased accessibility to the CD4 binding site (targeted by control MAb F105) and CD4-induced epitopes (targeted by 17b) while decreasing the potency of PGT128 (glycans). The other 20 strains showed little change in sensitivity to the control MAbs, indicating that the effects of the mutations were likely specific for V1/V2 recognition.
These gain-of-sensitivity mutational analyses support the conclusions drawn from the scaffolded V1/V2-PG9 crystal structures, suggesting that the conformations observed for these engineered constructs are biologically and functionally relevant. For each of the PG9-resistant strains selected for gain-of-function experiments, at least one of the selected mutants gained sensitivity to one or more of the V1/V2 MAbs, thus validating the predictions based on structure and sequence. While correlations of PG9 resistance with other factors such as glycosylation and length of V2 have also been noted, our results suggest a general mechanism of resistance to V1/V2-directed broadly neutralizing antibodies that involves alteration of basic residues within strand C of the V1/V2 domain. Additionally, our observation that gain-of-sensitivity mutations generally affected not only PG9 but also antibodies PG16, CH01, CH04, PGT141, and PGT145 provides further evidence that the members of this class recognize a similar epitope on the native HIV-1 envelope glycoprotein.
ACKNOWLEDGMENTS
Support for this work was provided by the Intramural Research Program of the Vaccine Research Center, NIAID, NIH, by the Center for HIV/AIDS Vaccine Immunology (CHAVI) grant AI067854 from the Division of AIDS, NIAID, NIH, by grant AI 033292 from NIAID, NIH, and by the International AIDS Vaccine Initiative.
We are grateful to Brenda Hartman for assistance with graphics. We thank members of the Structural Biology Section, Structural Bioinformatics Core, Humoral Immunity Section, and BSL-3 Core at the NIH Vaccine Research Center for helpful discussions of the manuscript.
Footnotes
Published ahead of print 23 May 2012
Supplemental material for this article may be found at http://jvi.asm.org/.
REFERENCES
Articles from Journal of Virology are provided here courtesy of American Society for Microbiology (ASM)
Full text links
Read article at publisher's site: https://doi.org/10.1128/jvi.00696-12
Read article for free, from open access legal sources, via Unpaywall:
https://jvi.asm.org/content/jvi/86/15/8319.full.pdf
Citations & impact
Impact metrics
Article citations
mRNA-encoded HIV-1 Env trimer ferritin nanoparticles induce monoclonal antibodies that neutralize heterologous HIV-1 isolates in mice.
Cell Rep, 38(11):110514, 01 Mar 2022
Cited by: 31 articles | PMID: 35294883 | PMCID: PMC8922439
Structure-guided envelope trimer design in HIV-1 vaccine development: a narrative review.
J Int AIDS Soc, 24 Suppl 7:e25797, 01 Nov 2021
Cited by: 26 articles | PMID: 34806305 | PMCID: PMC8606863
Review Free full text in Europe PMC
Engineering well-expressed, V2-immunofocusing HIV-1 envelope glycoprotein membrane trimers for use in heterologous prime-boost vaccine regimens.
PLoS Pathog, 17(10):e1009807, 22 Oct 2021
Cited by: 10 articles | PMID: 34679128 | PMCID: PMC8565784
Immunogenicity Evaluation of N-Glycans Recognized by HIV Broadly Neutralizing Antibodies.
ACS Chem Biol, 16(10):2016-2025, 15 Aug 2021
Cited by: 2 articles | PMID: 34649433 | PMCID: PMC8526942
Systematic Assessment of Antiviral Potency, Breadth, and Synergy of Triple Broadly Neutralizing Antibody Combinations against Simian-Human Immunodeficiency Viruses.
J Virol, 95(3):e01667-20, 13 Jan 2021
Cited by: 3 articles | PMID: 33177194 | PMCID: PMC7925105
Go to all (61) article citations
Data
Data behind the article
This data has been text mined from the article, or deposited into data resources.
BioStudies: supplemental material and supporting data
Similar Articles
To arrive at the top five similar articles we use a word-weighted algorithm to compare words from the Title and Abstract of each citation.
Structure of HIV-1 gp120 V1/V2 domain with broadly neutralizing antibody PG9.
Nature, 480(7377):336-343, 23 Nov 2011
Cited by: 635 articles | PMID: 22113616 | PMCID: PMC3406929
Fragments of the V1/V2 domain of HIV-1 glycoprotein 120 engineered for improved binding to the broadly neutralizing PG9 antibody.
Mol Immunol, 77:14-25, 21 Jul 2016
Cited by: 8 articles | PMID: 27449907 | PMCID: PMC7418894
HIV-1 envelope proteins and V1/V2 domain scaffolds with mannose-5 to improve the magnitude and quality of protective antibody responses to HIV-1.
J Biol Chem, 289(30):20526-20542, 01 Jul 2014
Cited by: 17 articles | PMID: 24872420 | PMCID: PMC4110267
[Role of the HIV-1 gp120 V1/V2 domains in the induction of neutralizing antibodies].
Enferm Infecc Microbiol Clin, 27(9):523-530, 01 May 2009
Cited by: 2 articles | PMID: 19409660
Review
Funding
Funders who supported this work.
Intramural NIH HHS
NIAID NIH HHS (6)
Grant ID: R37 AI033292
Grant ID: U19 AI067854
Grant ID: AI067854
Grant ID: AI 033292
Grant ID: U01 AI067854
Grant ID: R01 AI033292