Abstract
Free full text

Knockout of SOD1 altered murine hepatic glycolysis, gluconeogenesis, and lipogenesis
Abstract
We previously observed a stronger effect of knockout of Cu, Zn superoxide dismutase (SOD1) than that of Se-dependent glutathione peroxidase-1(GPX1) on murine body weight and glucose homeostasis. Two experiments were conducted to determine how hepatic lipid profile and key metabolic regulators were corroborated with this difference. The SOD1−/−, GPX1−/−, and their respective wild-type (WT) littermates (n = 6 or 7/group, male) were fed a Se-adequate Torula yeast-sucrose diet and killed at 6 months of age to collect liver samples. In Exp. 1, fasted SOD1−/− mice displayed pyruvate intolerance and a 61% decrease (P < 0.05) in liver glycogen compared with the WT littermates. The former had lower (P < 0.05) activities of phosphoenolpyruvate carbooxykinase, total protein phosphatase, and protein phosphatase 2A, but a higher (P < 0.05) activity of glucokinase in the liver than those of the latter. In contrast, hepatic concentrations of total cholesterol, triglyceride, and non-esterified fatty acids were increased by 11 to 100% (P < 0.05) in the SOD1−/− mice. Meanwhile, these mice had elevated (P < 0.05) hepatic protein levels of sterol regulatory element binding proteins-1 and 2, p53 MAPK, total and phosphorylated AMP-activated protein kinase α1 protein, protein tyrosine phosphatase-1B, and protein phosphatase-2B. In Exp. 2, GPX1−/− and their WT littermates were compared, but showed no difference in any of the measures. In conclusion, knockout of SOD1, but not GPX1, led to a decreased liver glycogen storage synchronized with pyruvate intolerance and elevated hepatic lipid profiles in the adult mice. This striking comparison was possibly due to unique impacts of these two knockouts on intracellular tone of H2O2 and key regulators of liver gluconeogenesis, glycolysis, and lipogenesis.
Metabolic syndrome (MS) is a complex disorder with multiple interrelated disturbances of glucose and lipid homeostasis [1]. The prevalence of MS is increasing to epidemic magnitude, afflicting not only the United States and other urbanized countries but also developing nations. Major risk factors of MS include: abdominal obesity [2], atherogenic dyslipidemia [3], hepatic steatosis [4], dys-regulation of glucose metabolism [5]. Many studies show that MS and its associated diseases are often involved in oxidative stress, a condition featured as accelerated production and (or) attenuated removal of reactive oxygen species (ROS) [5, 6]. Despite its essential role in multiple physiological systems, ROS, at excessive levels, leads to cellular dysfunction in excess [7].
Cu, Zn-superoxide dismutase (SOD1) and Se-dependent cellular glutathione peroxidase-1 (GPX1) are widely considered to be two primary intracellular antioxidant enzymes in mammals. SOD1 can convert superoxide anion to hydrogen peroxide [8] which is subsequently reduced to water with the catalysis of GPX1 [9]. Because of this coupled reaction, SOD1 and GPX1 are thought to be cooperative in protecting cells against oxidative stress [10–12]. Our laboratory has applied SOD1−/− and GPX1−/− mice to study metabolic roles of these two enzymes in hepatic oxidative stress or drug toxicity [13], bone metabolism [14] and pancreatic physiology [15]. These studies reveal a greater impact by knockout of SOD1 than that of GPX1 on adult body weight, femoral mechanical characteristics, islet function, pancreas integrity, and glucose homeostasis than those of GPX1. While body glucose and lipid metabolisms are well-coordinated and liver represents a major organ for these metabolic events in mice [16], it is unclear if the previously-observed abnormal body weight and glucose status in the SOD1−/− mice [15] concur with altered lipid profiles and dys-regulation of key factors for glycolysis, gluconeogenesis, and lipogenesis.
As a master regulator of energy metabolism, AMP-activated protein kinase (AMPK) up-regulates the major energy-generating pathways (glycolysis), but down-regulates gluconeogenesis [17]. Phosphoenolpyruvate carbooxykinase (PEPCK) is the rate-limiting enzyme in gluconeogenesis [18], whereas glucokinase (GK) plays a key role in glucose oxidation (glycolysis) [19]. Meanwhile, sterol-regulatory binding protein-1c (SREBP-1c) and sterolregulatory binding protein 2 (SREBP2) are transcription factors regulating fatty acid, triglyceride, and cholesterol synthesis in liver [20]. Many studies have demonstrated that SREBP-1c mediates positive actions of insulin on glycolytic and lipogenic genes [21, 22]. Mueller et al. [23] have suggested that activation of protein tyrosine phosphatase-1B (PTP1B) stimulates the lipogenic pathway by inducing the SREBP-1c expression. Choi et al. [24] have shown that AMPK cascades are highly sensitive to oxidative stress, and the β1 and β2 subunits of AMPK are transcriptionally up-regulated via a p53-dependent signal pathway [25]. Nevertheless, it remains unclear as how these key factors are affected by changes in intracellular superoxide and hydrogen peroxide status associated with the SOD1 and GPX1 knockout. Theoretically, ROS or oxidative stress activates c-jun NH 2 -terminal kinase (JNK2) phosphorylation that can be inactivated by protein phosphatases (PPase) [26, 27]. While protein phosphatases 2A (PP2A) is known to be specific for dephosphorylation of PPase and another important enzyme, fructose- 2, 6-bisphosphatase, in controlling hepatic glycolysis [28]. In addition, oxidative stress enhances both the activity and phosphorylation of neutral sphingomyelinase 2 (nSMase2) that is bound directly by the phosphatase calcineurin (also known as protein phosphatase 2B -PP2B) which acts as an on/off switch for nSMase2 phosphorylation in the presence or absence of oxidative stress [29]. Therefore, it is confirmed that PP2B is relevant to oxidative stress. Furthermore, PP2B may be involved in regulating the lipogenesis pathway [30]. Subsequently, it is fascinating to find out if the metabolic impacts of the SOD1 and GPX1 knockout are initiated at these key stress and phosphorylation-related signal proteins.
Therefore, our objectives for the present study were to determine: 1) if hepatic activities of glycolysis and gluconeogenesis and profiles of lipids were affected differently by the GPX1 and SOD1 knockouts; and 2) how the resultant metabolic phenotypes were derived from dysregulations of key signal and (or) functional proteins involved in glycolysis, gluconeogenesis, and lipogenesis associated with the presumed changes of intracellular ROS status. Our results indicate that knockout of SOD1 impaired glucose homeostasis through the p53-AMPK pathway and lipid metabolism via the PTP1B-SREBP1 pathway. In contrast, knockout of GPX1 showed little effect on those pathways. Thus, we reveal a distinct difference in the physiological function and the underlying mechanism between these two major antioxidant enzymes in carbohydrate and lipid metabolism.
Materials and methods
Chemicals and antibodies
All chemicals were purchased from Sigma Chemical Co. (St. Louis, MO) unless otherwise indicated. The following antibodies were used: SREBP1, SREBP2, JNK2, PP2B, and p53 MAPK (Santa Cruz Biotechnology, Santa Cruz, CA); PTP1B (Abgent primary antibody company, San Diego, CA); AMPKα1 and phospho- AMPKα1 (Thr172) (Upstate Biotechnology, Lake Placid, NY); anti-rabbit IgG (Bio-Rad Laboratories, Hercules, CA); and anti-mouse IgG (Pierce Biotechnology Inc., Rockford, IL).
Animals and pyruvate tolerance test
Our experiments were approved by the Institutional Animal Care and Use Committee at Cornell University and were conducted in accordance with the National Institutes of Health (NIH) guidelines for animal care. The GPX1−/−, SOD1−/−, and their littermate WT mice were generated from C57BL/6 mice [31, 32]. All experimental mice (n = 6 or 7 per genotype) were male, 6-months old and were fed a Se-adequate (0.4 mg/kg) Torula yeast-sucrose diet [33]. Mice were given free access to food and distilled water, and housed in shoebox cages in a constant temperature (22) room with a 12-h light/dark cycle. For the pyruvate tolerance test (PTT), mice were fasted (overnight) for 18 h and then given an i.p. injection of sodium pyruvate at 2 g/kg of body weight (dissolved in PBS) [34]. Blood glucose concentrations were measured using a glucometer (Bayer, Elkhart, IN) via tail bleeding at 0 to 180 min after the injection.
Liver sample collection and biochemical analyses
Mice were fasted (overnight) for 8 h and killed as previously described to collect liver samples [35]. To extract lipid for the assay of total cholesterol (TC), total triglycerides (TG) and non-esterified fatty acid (NEFA), a total of 50 mg of liver was mixed with 0.5 ml of PBS and homogenized (Polytron PT3100; Brinkmann Instruments, Littau, Switzerland). After the homogenate was extracted with chloroform:methanol (5:2.5 mL) and centrifuged, the lower layer was transferred into a glass tube, dried under nitrogen gas, and dissolved into 200 mL of ethanol containing 1% Triton. Concentrations of TC, TG and NEFA in the ethanol solution were measured using kits (Wako Chemicals, Richmond, VA) [36]. Liver glycogen content was measured using a spectrophotometric assay [37].
Enzyme activity assays
Hepatic activity of PEPCK was determined using a spectrophotometric assay as described by Wimonwatwatee [38]. A unit of PEPCK activity is defined as one micromole of NADH oxidized per minute per milligram protein. Glucokinase activity was measured spectrophotometrically in a glucose-6-phosphate dehydrogenase-driven NAD(P)H fluorescence assay at 340 nm with the following modifications [39]. Mouse liver (50 mg) was homogenized in 500 µL of buffer containing 5 mM dithiothreitol, 5 mM EDTA, and 150 mM KCl. After the homogenate was centrifuged at 12,000×g for 10 min, the supernatant was used for glucokinase determination. The reaction mixture (200 µL) (15 µL sample) contained 250 mM Tris-HCl, pH 7.50, 750 mM glucose, 1 M KCl, 75 mM ATP, 75 mM MgSO4, 7.5 mM NADP, 40 units of glucose-6-P dehydrogenase/mL. A unit of enzyme activity is defined as the amount of glucokinase that catalyzes the formation of 1 micromole of glucose-6- phosphate per minute at 30. Total protein phosphatase (PPase) and PP2A activities were measured using p-nitrophenol phosphate (PNPP) as substrate and okadaic acid (OA) as an inhibitor at 405 nm according to the method of William [40]. One unit of total PPase activity is defined as the amounts of enzyme providing the formation of 1 micromole of p-nitrophenol per minute. Protein concentration was estimated using a BCA protein assay kit (Pierce Chemical Co., Rockford, IL).
Western blot analyses
Liver samples were homogenized (Polytron PT3100; Brinkmann Instruments, Littau, Switzerland) in buffer [50 mM potassium phosphate buffer, pH 7.8; 0.1% Triton X-100; 1.34 mM diethylenetriaminepentaacetic acid; 1 mM phenylmethylsulfonyl fluoride; 10 µg/ml peptstain A; 10 µg/ml leupeptin; 10 µg/ml aprotinin]. The homogenates were centrifuged at 12,000 g for 10 min at 4°C. Protein concentration was assayed using the BCA protein assay kit. The supernatants (30 µg of protein per lane) were loaded for Western blot analyses as described previously [41]. Relative densities of protein bands in individual blots were quantified using an IS-1000 Digital Imaging System (Alpha Innotech Co., San Leandro, CA).
Statistical analysis
Impacts of SOD1 and GPX1 knockout on all measures except for PTT were analyzed using the general linear model procedure of SAS (release 8.2; SAS Institute, Cary, NC) as a one-way analysis of variance against their respective WT littermate controls. The PTT data were analyzed using the same model but with time-repeated measurements. Data are presented as mean ± SEM, and significance was defined as P < 0.05.
Results
Knockout of SOD1 impacted body and hepatic glucose metabolism
The pyruvate injection resulted in less (P < 0.05) increases in blood glucose concentrations of the SOD −/− mice than those of the WT littermates (SOD +/+) (Fig. 1A). However, there was no such difference between the GPX1−/− mice and the WT littermates (GPX1+/+) (Fig.1B). After fasting for 8 h, the SOD1−/− mice showed 61% decrease (P < 0.05) in liver glycogen content compare with the SOD +/+ mice (Fig. 1C), Likewise, the reduction associated with the GPX1−/− did not reach statistical significance (Fig. 1D). Hepatic activities of PEPCK (Fig. 2A) and glucokinase (Fig. 2C) in the SOD1−/− mice were 61 lower and 79% higher (P < 0.05) than those of the SOD +/+ mice, respectively. Again, there was no GPX1 knockout effect on these two enzyme activities (Fig. 2B and 2D).
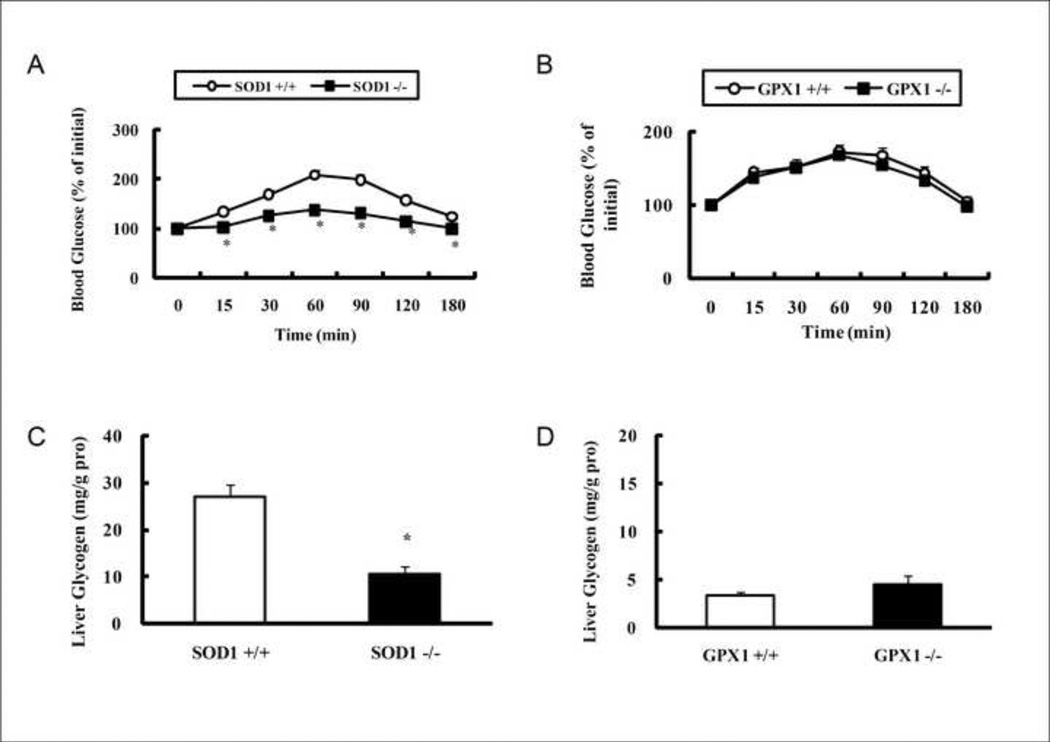
Effects of SOD1 and GPX1 knockouts on mouse (6 months of age) pyruvate tolerance (A and B, 2 g/kg of body weight, fasted for 18 h) and intrahepatic glycogen content (C and D, fasted for 8 h). Values are means ± SE (n = 6–7). The asterisks* indicate genotype differences (P ≤ 0.05).
Knockout of SOD1 up-regulated hepatic lipid profiles
Compared with the SOD +/+ controls, the SOD1−/− mice manifested with more than one-fold, 56%, and 11% increases (P < 0.05) in hepatic total TC, TG, and NEFA contents, respectively (Fig. 3A, C, and E). In contrast, GPX1−/− mice showed no statistically significant difference in these three measures from their own WT littermates (Fig. 3B, D, and F). Hepatic protein amounts of SREBP1 and SREBP2, along with p53 (Fig. 4) were twice higher (P < 0.05) in the SOD1−/− mice than in their WT controls. In contrast, the GPX1−/− mice and their WT littermates showed similar amounts of these three proteins.
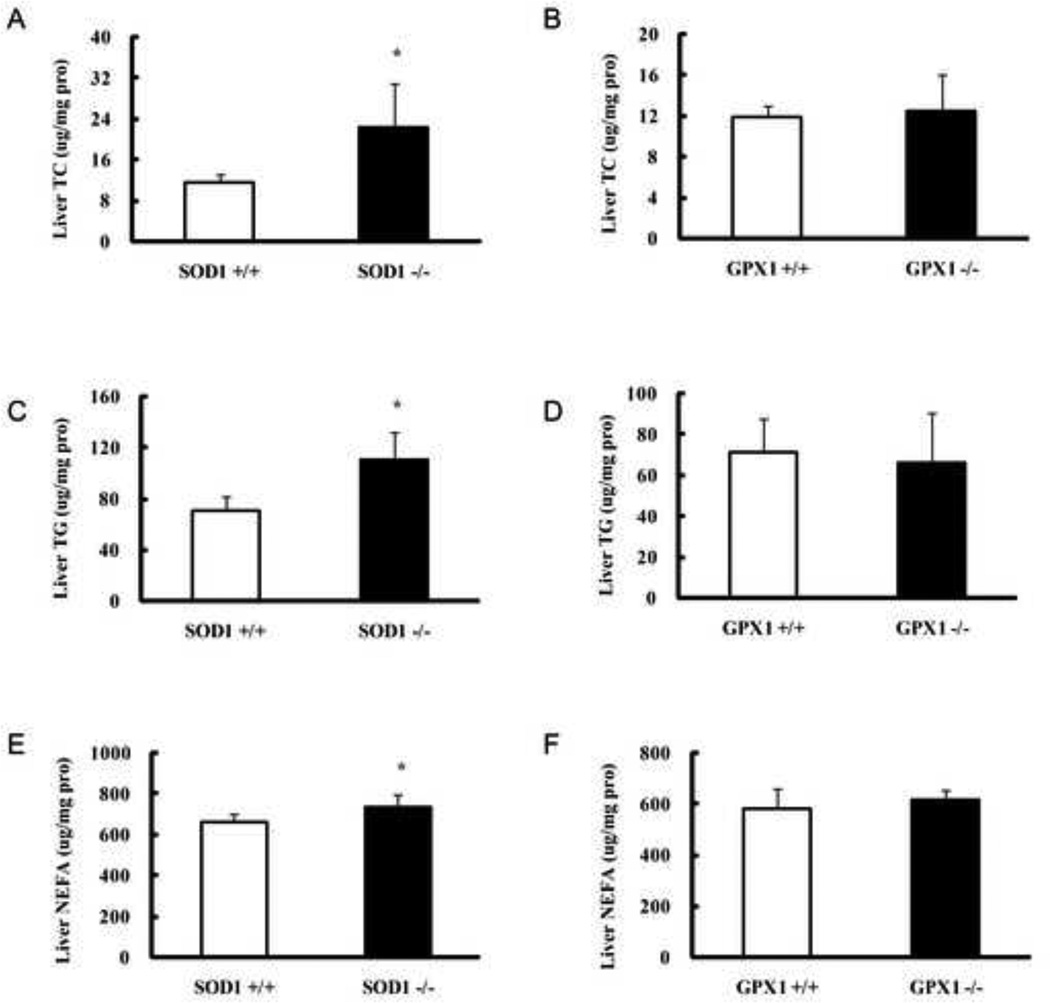
Effects of SOD1 and GPX1 knockouts on mouse (6 months of age, fasted for 8 h) hepatic total cholesterol (TC, A and B), total triglycerides (TG, C and D), and non-esterified fatty acid (NEFA, E and F). Values are means ± SE (n = 6–7). The asterisks* indicate genotype differences (P ≤ 0.05).

Effects of SOD1 and GPX1 knockouts on mouse (6 months of age, fasted for 8 h) hepatic total proteins of SREBP1, SREBP2, and p53. For a given protein, the upper band shows the representative images of three independent analyses (n = 3), and the lower value shows the relative density of protein bands (mean ± SE, n = 9). The superscript letters a and b indicate genotype differences (P ≤ 0.05).
Knockout of SOD1 altered hepatic protein kinase/phosphatase signaling
As shown in Fig. 5, there was a 50% increase (P < 0.05) in hepatic AMPKα1 protein in the SOD1−/− mice than their WT littermates (Fig. 5). This increase was concurrent with an elevation (P < 0.05) of hepatic phosphorylated AMPKα1 (on Thr-172). There was no difference in either form of these two proteins between the GPX1−/− and their WT littermates. Meanwhile, knockout of SOD1 led to a 22% decrease (P < 0.05) in hepatic activity of total PPase and to a 49% decrease in hepatic PP2A activity (Fig. 6A), although the latter difference was not statistically significant. Knockout of GPX1 essentially diminished hepatic GPX1 activity, but showed no effect on either phosphatase activity (Fig. 6B). The SOD1−/− mice also displayed as high as 4.4 and 2-fold of hepatic PTP1B and PP2B proteins (P < 0.05) compared with their WT controls (Fig. 6C). In addition, the SOD1−/− mice had 1.7-fold higher of hepatic JNK2 (P < 0.05) than their WT controls. Knockout of GPX1 exhibited no effect on any of these four proteins.
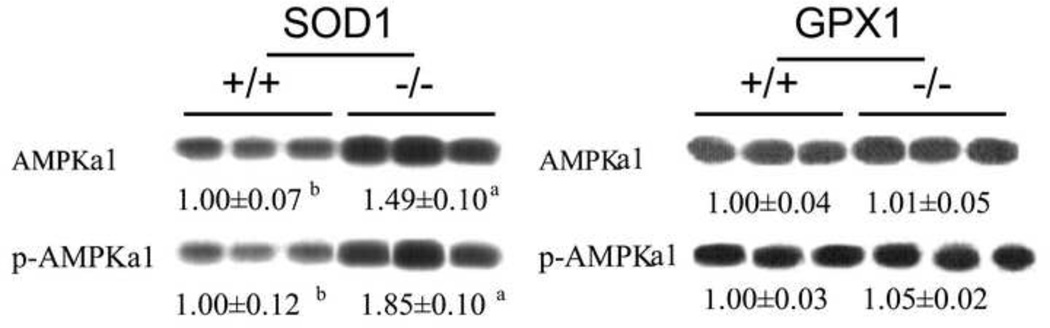
Effects of SOD1 and GPX1 knockouts on mouse (6 months of age, fasted for 8 h) hepatic AMPK proteins. For a given protein, the upper band shows the representative images of three independent analyses (n = 3), and the lower value shows the relative density of protein bands (mean ± SE, n = 9). The superscript letters a and b indicate genotype differences (P ≤ 0.05).
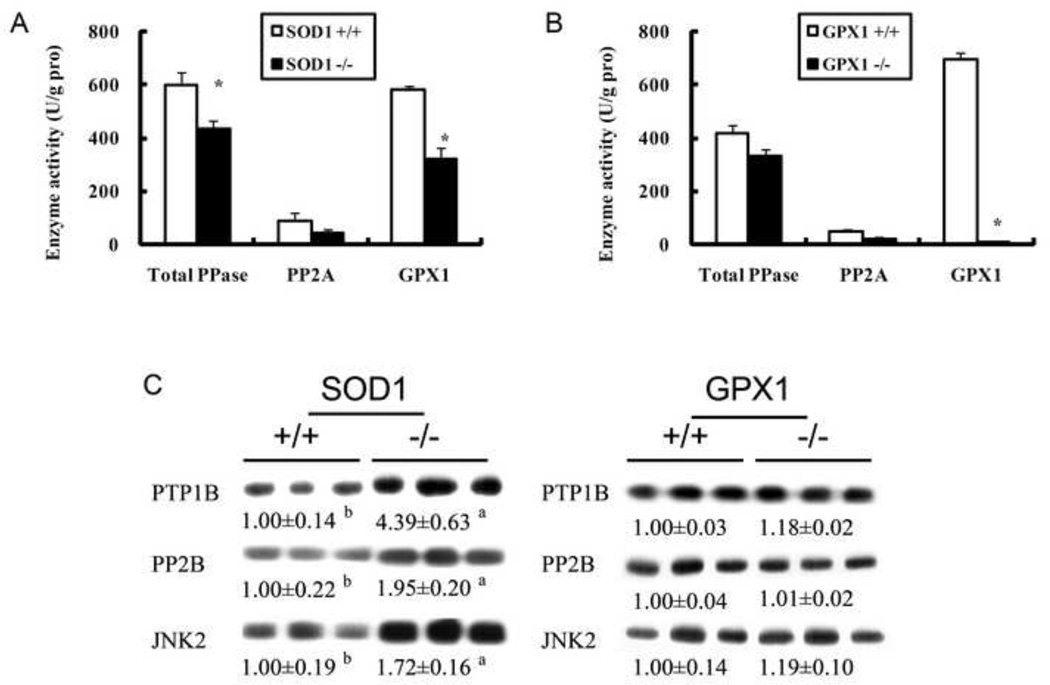
Effects of SOD1 and GPX1 knockouts on mouse (6 months of age, fasted for 8 h) hepatic total activities of protein phosphatase, PP2A and GPX1 (A and B) and hepatic protein amount of PTP1B, PP2B and JNK2 (C). In panels A and B, values are means ± SE (n = 6–7). The asterisks* indicate genotype differences (P ≤ 0.05). In panel C for a given protein, the upper band shows the representative images of three independent analyses (n = 3), and the lower value shows the relative density of protein bands (mean ± SE, n = 9). The superscript letters a and b indicate genotype differences (P ≤ 0.05).
Discussion
It is remarkable that knockout of SOD1, but not GPX1, resulted in dys-regulations of body pyruvate tolerance, hepatic glycolytic and gluconeogenesis activities, and hepatic lipid profiles in mice. Apparently, SOD1 plays a more crucial role in hepatic glucose and lipid metabolism than GPX1. In consistent, our previous study has illustrated much greater changes in body weight, glucose, and postmortem plasma insulin concentration caused by the SOD1 knockout than those by the GPX1 knockout [15]. These two knockouts also exerted a strikingly different impact on mouse susceptibility to acetaminophen toxicity [13]. Altogether, our present and past findings have revealed a consistent fact that the metabolic functions of SOD1 and GPX1 in regulating body energy metabolism, as in the case of coping with reactive nitrogen species-mediated oxidative stress, are quite unique or enzyme-specific. Consequently, the “prevailing” view of these two enzymes as indistinguishable intracellular antioxidant defenses [10–12] is somewhat mistakenly-conceived, and should be revised. Knockout of SOD1 presumably decreases, whereas knockout of GPX1 elevates intracellular hydroperoxide (H2O2) concentrations [15]. Our data implicate that diminishing intracellular hydroperoxide (H2O2) is more detrimental to glucose and lipid metabolisms than elevating it. This notion is also supported by the metabolic disturbance in insulin physiology and glucose homeostasis in the GPX1-overexpressing mice in which intracellular hydroperoxide (H2O2) was decreased [35, 42]. Coincidently, the metabolic disorders induced by attenuating intracellular H2O2 production in the SOD1−/− mice and accelerating its breakdown by the GPX1 overexpression underscore the physiological importance of “minimal” levels of intracellular H2O2.
Another exciting novel finding of the present study was the up-regulation of total AMPKα1 and p-AMPKα1 protein levels in the liver of SOD1−/− mice. This up-regulation might serve as the key mediator of the attenuated gluconeogenesis induced by the SOD1 knockout. Because activation of AMPK via phosphorylation inhibits and stimulates PEPCK and GK activity, respectively, an elevated AMPK activity has been shown to lower hepatic glycogen storage [43–45]. Consistently, impacts of SOD1 knockout on lowering hepatic gluconeogenic capacity in the present study were manifested by: 1) decreased PEPCK activity, 2) decreased pyruvate tolerance, and 3) decreased hepatic glycogen content. Because PEPCK is the rate-limiting enzyme in gluconeogenesis [18] and pyruvate represents an important substrate for the event, the 61% inhibition of the enzyme activity, presumably by the up-regulated p-AMPKα1, in the livers of SOD−/− mice, compared with their WT littermates, offers a good explanation to their reduced ability to convert the injected pyruvate into blood glucose. Subsequently, this down-regulated gluconeogenesis, along with a stimulated hepatic glycolysis associated with an increased GK activity, helps explain the decreased hepatic glycogen concentration in the SOD1−/− mice. From the free radical standpoint, the concurrent elevation of intracellular superoxide and diminishing of intracellular hydroperoxide in the SOD1−/− mice might additively stimulate these pathways. It is known that superoxide can cause intracellular alkalinization that activates glycolysis by increasing the activity of phosphofructokinase, a key regulatory enzyme for glycolysis [46]. Meanwhile, H2O2 inhibits several of the potentially glycolytic enzymes, i.e., GK and glyceraldehyde 3-phosphate dehydrogenase [47]. Removal of the dismutation of superoxide into H2O2 by the SOD1 knockout could lift such inhibitions.
Elevation of hepatic lipids including TG, TC, and NEFA by the SOD1 knockout in the mouse liver was concurrent with the up-regulation of SREBP-1c and SREBP2. It is well known that these transcription factors play key roles in regulating fatty acid, triglyceride, and cholesterol synthesis in liver [20]. Specifically, an isoform SREBP-1c is directly responsible for activating the synthesis of triglyceride and other lipids in liver cells [48]. Consistently, H2O2-stimulation increased SREBP-1c transcriptional activity and induced lipid accumulation in HepG2 cells [49], and genetic overexpression or knockdown of SREBP-1 in prostate cancer cells resulted in corresponding increased or decreased fatty acid synthase expression, fatty acid and lipid droplet accumulation [50]. Bettzieche et al [51] showed an increased mRNA expression of fatty acid synthase (FAS), glucose-6-phosphate dehydrogenase (G6PDH) and stearoyl-CoA desaturase (SCD)-1 in HepG2 cells treated with pro-oxidant CuSO4 compared with control cells. Interestingly, an adenovirus-mediated overexpression of SREBP-1c in the liver of streptozotocin- induced diabetic mice resulted in increased expressions of glycolytic enzymes (GK) and lipogenic enzymes (FAS, S14) and a dramatic decreased PEPCK expression [52]. Because all these changes mimicked perfectly the scenario of the SOD1−/− mice in the present study, the functional regulation of GK and PEPCK by SREBP-1c may serve as a general mechanism in coordinating the ROS-mediated simultaneous down-regulation of gluconeogenesis and up-regulation of lipogenesis.
To explore the potential intermediate link between the SOD1 knockout-induced superoxide stress and the up-regulations of hepatic AMPKα1/p-AMPKα1 and SREBP-1c/SREBP2, we determined two stress-related signal proteins (JNK2 and p53) located upstream in the cascade [53] and three protein phosphatases (PTP1B, PP2A, and PP2B) that regulate expression or function of AMPK and SREBP family proteins [54]. Similar to that reported by Bae et al. [55], we found an induction of hepatic JNK2 by the SOD1 knockout. Coronella-Wood et al [56] suggested that c-Fos might serve as the linker for the H2O2-induced responses of JNKs. Meanwhile, we also observed an increase in hepatic p53 protein in the SOD1−/− mice, compared with the WT. Although the current focus of p53 is on its central role in integrating various stress signals-related to cell death [52], Feng et al. [25] reported that the β1 and β2 subunits of AMPK were transcriptionally up-regulated via a p53-dependent signal pathway. Thus, it was possible that signaling for the up-regulation of hepatic AMPKα1/p-AMPKα1 proteins by the SOD1 knockout was initiated at JNK2 and transferred from the stimulation of p53. Alternatively, potential impacts of p53 on energy metabolism may be mediated by AMPK.
The SOD1 knockout caused a decrease in total PPase and PP2A activities although the latter change was not statistically significant. Because PP2A activity was enhanced by acute H2O2 treatment in cardiomyocytes [57] and myofilament– particulate fraction [58], its down-regulation in the SOD1−/− mice was likely related the deficiency of intracellular H2O2. This decrease might also result from inhibitions to these phosphatases by the elevated intracellular superoxide levels in the SOD1−/− mice [59]. An enhanced insulin signaling by 25–50 mM of H2O2 was accompanied by the suppression of PTP1B activity, and was strengthened by a co-administration with vanadate, a non-specific inhibitor for phosphatases [60]. Similarly, PP2B can be inhibited by H2O2 in a dose-dependent manner, and the inhibition is mediated by modifying its 2 cysteine residues through the oxidative formation of a disulfide bridge [61]. Consequently, an increased PTP1B [62] and (or) PP2B [23] activity (protein) might induce the expression of SREBP-1c. At the same time, an increased PTP1B might also contribute to the decrease of PP2A activity that could help maintain the activity (phosphorylation) of AMPK [63] and (or) JNK2 [64].
In summary, the two major intracellular antioxidant enzymes SOD1 and GPX1 did not seem to play the same roles in regulating carbohydrate and lipid metabolism as only the SOD1 knockout produced distinct metabolic impairments. Thus, normal SOD1 expression is essential to maintain hepatic glucose and lipid homeostasis. Figure 7 highlights possible pathways or mechanisms for the impacts of SOD1 knockout and links at least 4 key protein phosphatases as the direct targets of the attenuated intracellular H2O2. However, further studies are certainly required to determine how much of the regulation is directly employed by the initial changes of intracellular superoxide/H2O2 tone to the target functional proteins such as GK and PEPCK and how much is mediated through the proposed signal pathways. Caution should be taken that knockout of SOD1 might modify or disconnect normal pathways. A good example is that while an enhanced AMPK was supposed to down-regulate SREBP-1 [65], we saw simultaneous increases of both proteins in the SOD1−/− mouse liver. Thus, novel mechanisms may exist to be explored for revealing their interactions and their down-stream effect on lipid metabolism.
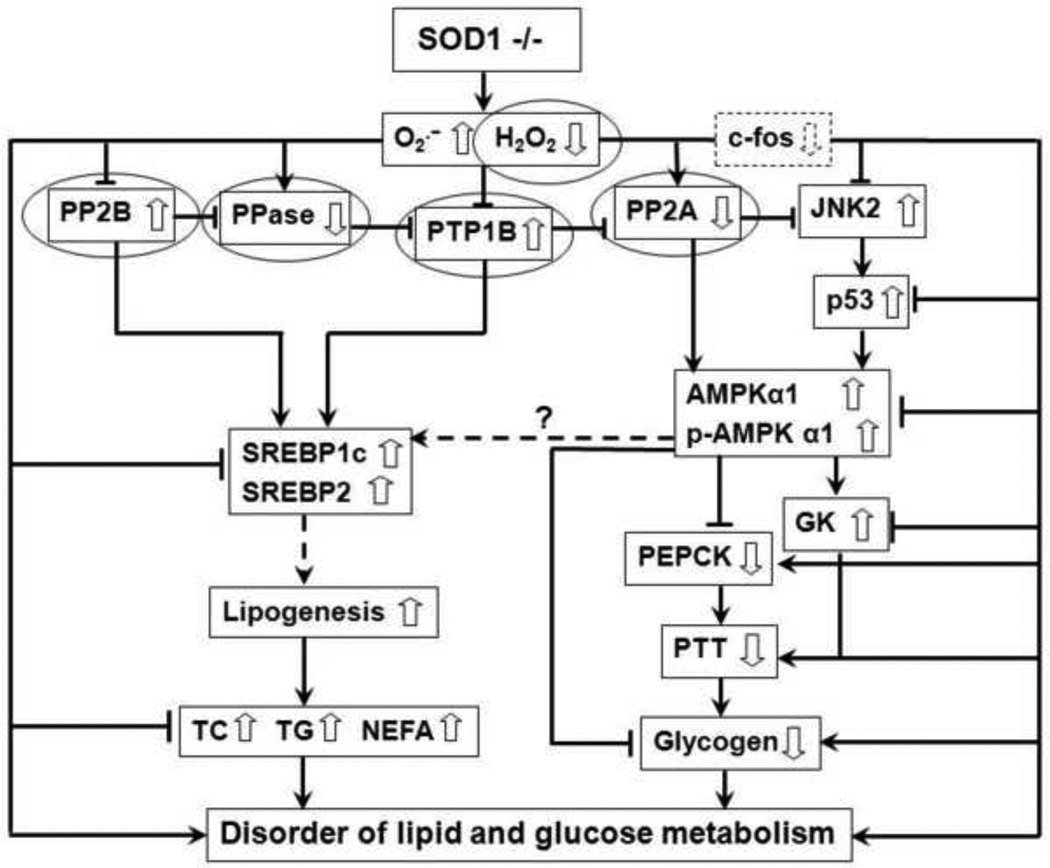
Schematic illustration of potential mechanisms of SOD1 knockout leading to the metabolic phenotype. →, Activation or increase; , Inhibition or decrease;
, Possibly affect;, Direct targets of H2O2; AMPKα1, AMP-activated protein kinase α1; c-fos, a component of transcription factor activator protein 1; GK, glucokinase; H2O2, hydrogen peroxide; JNK2, c-jun NH2 -terminal kinase; NEFA, non-esterified fatty acid; P, phosphorylation; p53, p53 mitogenactivated protein kinases; PEPCK, phosphoenolpyruvate carbooxykinase; PP2A, protein phosphatase 2A; PP2B, protein phosphatase 2B; PTP-1B, protein tyrosine phosphatase-1B; PTT, pyruvate tolerance test; SOD1, Cu, Zn-superoxide dismutase; −/−, knockout; SREBP, sterolregulatory binding protein; TC, total cholesterol; TG, total triglycerides.
Acknowledgments
We are grateful for the technical assistance of Xiaodan Wang and Carol A. Roneker. This study was supported in part by the National Institute of Health grant DK53108 to XGL.
Abbreviations
AMPK | AMP-activated protein kinase |
GPX1 | Se-glutathione peroxidase-1 |
JNK2 | c-jun NH 2 -terminal kinase |
MAPK | Mitogen-activated protein kinase |
MS | Metabolic syndrome |
NEFA | non-esterified fatty acid |
PEPCK | phosphoenolpyruvate carbooxykinase |
PP2A | protein phosphatases 2A |
PP2B | protein phosphatases 2B |
PPase | protein phosphatases |
PTP1B | Protein tyrosine phosphatase 1B |
PTT | pyruvate tolerance test |
ROS | reactive oxygen species |
SOD1 | Cu, Zn-superoxide dismutase |
−/− | knockout |
+/+ | wild-type |
SREBP-1c | sterol-regulatory binding protein-1c |
SREBP2 | sterol-regulatory binding protein 2 |
TC | total cholesterol |
TG | total triglycerides. |
Footnotes
Publisher's Disclaimer: This is a PDF file of an unedited manuscript that has been accepted for publication. As a service to our customers we are providing this early version of the manuscript. The manuscript will undergo copyediting, typesetting, and review of the resulting proof before it is published in its final citable form. Please note that during the production process errors may be discovered which could affect the content, and all legal disclaimers that apply to the journal pertain.
References
Full text links
Read article at publisher's site: https://doi.org/10.1016/j.freeradbiomed.2012.08.570
Read article for free, from open access legal sources, via Unpaywall:
https://europepmc.org/articles/pmc3472123?pdf=render
Citations & impact
Impact metrics
Citations of article over time
Article citations
Discovery of a novel homozygous SOD1 truncating variant bolsters infantile SOD1 deficiency syndrome.
Mol Biol Rep, 51(1):580, 26 Apr 2024
Cited by: 2 articles | PMID: 38668754
Non-Alcoholic Fatty Liver Disease: Translating Disease Mechanisms into Therapeutics Using Animal Models.
Int J Mol Sci, 24(12):9996, 10 Jun 2023
Cited by: 2 articles | PMID: 37373143 | PMCID: PMC10298283
Review Free full text in Europe PMC
Evidence and Metabolic Implications for a New Non-Canonical Role of Cu-Zn Superoxide Dismutase.
Int J Mol Sci, 24(4):3230, 06 Feb 2023
Cited by: 1 article | PMID: 36834640 | PMCID: PMC9966940
Role of necroptosis in chronic hepatic inflammation and fibrosis in a mouse model of increased oxidative stress.
Free Radic Biol Med, 164:315-328, 09 Jan 2021
Cited by: 44 articles | PMID: 33429022 | PMCID: PMC8845573
FGF21: An Emerging Therapeutic Target for Non-Alcoholic Steatohepatitis and Related Metabolic Diseases.
Front Endocrinol (Lausanne), 11:601290, 14 Dec 2020
Cited by: 80 articles | PMID: 33381084 | PMCID: PMC7767990
Review Free full text in Europe PMC
Go to all (25) article citations
Data
Similar Articles
To arrive at the top five similar articles we use a word-weighted algorithm to compare words from the Title and Abstract of each citation.
Lipopolysaccharide-induced hepatic oxidative injury is not potentiated by knockout of GPX1 and SOD1 in mice.
Biochem Biophys Res Commun, 404(1):559-563, 08 Dec 2010
Cited by: 13 articles | PMID: 21145306 | PMCID: PMC3018559
Knockouts of SOD1 and GPX1 exert different impacts on murine islet function and pancreatic integrity.
Antioxid Redox Signal, 14(3):391-401, 29 Sep 2010
Cited by: 57 articles | PMID: 20586612 | PMCID: PMC3026657
Hepatic mTORC2 activates glycolysis and lipogenesis through Akt, glucokinase, and SREBP1c.
Cell Metab, 15(5):725-738, 19 Apr 2012
Cited by: 336 articles | PMID: 22521878
Selenium and diabetes--evidence from animal studies.
Free Radic Biol Med, 65:1548-1556, 16 Jul 2013
Cited by: 96 articles | PMID: 23867154 | PMCID: PMC3859733
Review Free full text in Europe PMC
Funding
Funders who supported this work.
NIDDK NIH HHS (2)
Grant ID: DK53108
Grant ID: R01 DK053018
National Institutes of Health (1)
Grant ID: DK53108