Abstract
Free full text

Schizophrenia in Translation: Dissecting Motivation in Schizophrenia and Rodents
Abstract
The negative symptoms of schizophrenia include deficits in motivation, for which there is currently no treatment available. Animal models provide a powerful tool for identifying the potential pathophysiological mechanisms underlying the motivation deficits of schizophrenia with the aim of discovering novel treatment targets. The success of such an approach critically depends on meticulously detailed analysis of motivational phenotypes in patients and in animal models. Here, we review the results of recent human behavioral and imaging studies of motivation, and we relate those findings to the results from animal studies, including a mouse model of striatal dopamine D2 receptor hyperfunction. The motivational deficit in patients with schizophrenia is not due to an inability to experience pleasure in the moment as hedonic reaction appears intact in patients. Instead, the motivation deficit represents a reduced capacity for anticipating future pleasure resulting from goal-directed action. The diminished anticipation appears to be a consequence of an inability to accurately represent the expected reward values of actions. A strikingly similar phenotype in incentive motivation has also been described in mice with striatal dopamine D2 receptor hyperfunction. These convergent findings identify potential pathophysiological mechanisms that underlie the deficit in anticipatory motivation, and importantly, the mouse model provides a tool for investigating novel treatment strategies, which we discuss here.
Apathy, amotivation, or avolition was described by both Kraeplin and Bleuler as a core feature of schizophrenia.1,2 Interest in this aspect of the disease has intensified over the last several years because a number of studies have shown that the severity of a patients’ deficit in motivation is the strongest predictor of functional outcome and quality of life.3,4 Currently, there are no effective treatments available for avolition. In order to develop new treatment strategies for this most disabling aspect of the disorder, the neurobiological basis of avolition must be understood.
Patients Exhibit Deficits in Anticipatory Motivation Without Anhedonia
Based on clinical scales involving questionnaires, patients with schizophrenia were previously considered to have a reduced capacity to experience pleasure, ie, suffer from anhedonia.5 The results of early neuroimaging studies of emotional processing in schizophrenia6,7 appeared to support these findings by showing that brain activity associated with hedonic experience was, in fact, disrupted. However, responses to questionnaires not only capture what the patient thinks and feels in the moment of the interview but are also influenced by the memory of pleasurable experiences in the past and the expectation of pleasure in the future. Similarly, most of these earlier imaging studies required subjects to remember feedback or emotional information across a delay, or use this information to guide choices. A revealing study by Gard et al8 circumvented these limitations by using experience sampling, where participants were asked at random times throughout the day to make notes of their experience in real time. Results from this study showed that patients have the capacity for normal hedonic reactions when they are experienced and measured in the moment, but reported anticipating less pleasure from future activities, specifically when the activities were goal directed, such as making dinner, doing an errand, working, or studying. Such dissociation of anticipatory motivation and hedonic reaction has also been shown using other approaches9–11 and is supported by a recent extensive review.12 Anticipatory pleasure is known to drive goal-directed behaviors aimed at achieving desired rewards.13 Consistently, Gard et al8 found that the degree of deficit in anticipatory pleasure correlates with decrement in goal-directed behavior and general functional outcomes.
In summary, when not required to remember emotional information, or use it to motivate actions, patients with schizophrenia exhibit hedonic responses in reward circuits comparable to control subjects.14,15
Consummatory Hedonia (Liking) and Anticipatory Motivation (Wanting) Involve Some Shared Neuroanatomical Substrates but Recruit Distinct Molecular Pathways
The neural networks supporting hedonic reactions share considerable anatomical overlap with the networks regulating anticipatory motivation. fMRI studies show increased activity in mesocorticolimbic circuitry, including dorsal and ventral striatum, midbrain, orbitofrontal cortex, medial PFC, amygdala, and insula cortex, in response to positive emotional or rewarding stimuli,16 and many of these regions are activated by anticipatory motivation (see below). However, wanting and liking also involve some distinct neuroanatomical substrates and are modulated by different neurochemical systems.
For example, hedonic reactions have been found to be regulated by opioid and cannabinoid signaling.17 The role of opioids and endocannabinoids in hedonic reactions and highly detailed anatomical maps of the sites involved in liking have been determined in rodents by exploiting the fact that the consumption of palatable foods elicits homologos patterns of facial affective reactions in primates (including humans) and rodents.18 Sweet tastes elicit positive facial “liking” expressions including lip licking and rhythmic tongue protrusions, whereas bitter tastes elicit negative “disliking” expressions including mouth gapes. Therefore, by measuring the facial reactions to substances that differ in palatability, hedonic reaction may be quantitatively assessed in rats and mice in a taste-reactivity paradigm.19,20 Studies using this paradigm have determined that injection of a µ-opioid receptor agonist or endocannabinoid into localized hotspots in the nucleus accumbens area of the ventral striatum and ventral pallidum significantly increase liking.21–23 In human imaging studies, both the ventral striatum and ventral pallidum have been shown to be activated in response to positive rewards.17
Wanting involves molecular substrates distinct from liking. Dopamine signaling is relatively uninvolved in hedonic reaction to reward20,24,25 but is critical for anticipatory motivation.26 Dopamine also plays a key role in processes related to wanting, such as reinforcement learning27,28 and the willingness to expend effort to obtain a goal.29 The brain regions known to participate in incentive motivation include the orbitofrontal cortex, which plays a key role in updating and maintaining value representations,30,31 as does the amygdala.32,33 The orbitofrontal cortex and the anterior cingulate cortex have been shown to be important in the computation of cost/benefit values that underlie the initiation and maintenance of action.34 In addition, the prefrontal cortex is involved in the construction, selection, and execution of specific action plans based on value representations.34–37 A recent review highlights how deficits in the cognitive processes supported by these structures may underlie the deficit in anticipatory motivation displayed in patients with schizophrenia.12
Wanting Is Disrupted in Schizophrenia by an Inability to Accurately Represent the Expected Reward Values of Actions
Given the evidence of intact liking in patients with schizophrenia, how might motivational deficits emerge? A deficit in “wanting” could reflect a reduced ability to translate hedonic experience into the expectation of value. This reduced ability to update representations of expected value could, in turn, be the result of faulty learning mechanisms, ie, difficulty in computing the reward prediction errors (RPEs) that drive reinforcement learning. However, data from a recent behavioral study suggests that this is not the case. Gold et al38 had patients with schizophrenia and controls perform a probabilistic reinforcement learning task, requiring subjects to learn to choose the best option from each of 4 pairs of stimuli. In 2 pairs, the best option resulted in a frequent monetary gain; in the other 2 pairs, the best option resulted in the frequent avoidance of a monetary loss. Once the pairs were acquired, stimuli were presented in novel combinations in a test phase to assess preferences among stimuli, based on their valence and reinforcement probability (figure 1A). In order to investigate relationships with negative symptoms, patients were split into two subgroups, based on the median rating for avolition/anhedonia in the sample (from the SANS).39 Gold et al (2012) found that patients with mild negative symptoms exhibited normal preferences, during both acquisition and test, for gain stimuli, relative to neutral stimuli, and for neutral stimuli, relative to loss stimuli. This result pointed to an intact ability to learn from RPEs in patients with low-negative symptoms. However, when patients with high-negative symptoms were compared with either controls or patients with low-negative symptoms they showed normal acquisition for loss-avoidance stimuli, but slower acquisition and lower accuracy for gain stimuli (figure 1B). High-negative patients also showed a reduced preference for “frequent-gain” stimuli, relative to “frequent-loss-avoidance” stimuli. Therefore negative symptoms were not associated with a general deficit in learning from all RPEs, but more specifically with a reduced ability to represent or utilize expected positive values to guide decisions. Computational modeling of the data suggested that the mechanisms for integrating expected value estimates with information derived from RPE calculations were disabled in patients with severe negative symptoms.
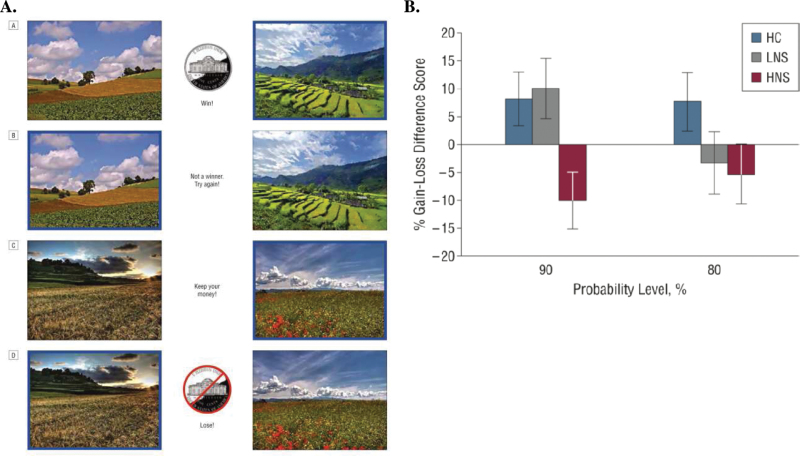
(A) Example of the stimuli pairings and feedback in the reinforcement learning task from.38 A, example of feedback delivered after a correct choice (choice indicated by a blue border) in a probabilistic gain (rewarded) trial. B, Feedback delivered following an incorrect choice in a gain trial. C, Feedback delivered following a correct choice in a loss-avoidance trial. D, Feedback delivered following an incorrect choice in a loss-avoidance trial. (B) To examine feedback valence effects on learning, the difference scores for gain-seeking trials and loss-avoidance trials for both the 90% and 80% probability conditions at the end of acquisition were computed. Difference scores above zero indicate better learning from gain than from loss avoidance, while scores below zero indicate better learning from loss avoidance than from gain. HC, healthy control; HNS, high-negative symptom; LNS, low-negative symptom group.38
Based on the findings reported by Gold et al the deficit in anticipatory motivation observed in patients with schizophrenia likely reflect dysfunction in orbitofrontal cortex and ventral striatum as these brain regions have been shown to participate in the representation of expected value.40,41 Accordingly, recent neuroimaging studies have produced evidence of relationships between clinical measures of avolition/anhedonia and striatal activity associated with reward anticipation in patients with schizophrenia.14,42,43
Dissecting Motivation in Animal Models of Disease
The animal studies described above have been critical in isolating circuits for liking distinct from those supporting wanting. Animal studies can also be used to explore how these distinct behaviors may be differentially disrupted in schizophrenia. We recently performed a detailed investigation into motivation and related behaviors in our own transgenic mouse model. The methods we used could be applied to dissect motivation in any transgenic, environmental, or pharmacological animal model or be used to screen potential drug treatments for amotivated patients.
To model the increase in striatal D2R activity observed in patients with schizophrenia,44 we generated mice that selectively and reversibly overexpress D2 receptors in the striatum (D2R-OE mice).45 We previously reported that D2R-OE mice display behavioral abnormalities that relate to both the cognitive and negative symptoms of schizophrenia.45–50 In relation to the negative symptoms, we initially observed that D2R-OE mice work less than control mice for appetitive rewards on a progressive ratio schedule in which each subsequent reward requires more effort to obtain than the previous reward.47 A series of studies has been carried out to dissect this observed reluctance to work in D2R-OE mice.
First, we determined that the decrease in effort is not due to the D2R-OE mice tiring more quickly or becoming sated by the milk rewards sooner.48 Another possible explanation for the deficit in the progressive ratio task could be an abnormal response to appetitive stimuli, ie, a reduction in liking. Therefore to obtain a measure of behavioral reaction to a palatable substance, we used a gustometer to record licking behavior as a function of sucrose concentration. In previous studies, both mice and rats show increased licking as sucrose concentration increases, and this is thought to reflect the palatability of the sucrose solution.51,52 We found that the vigor and sensitivity of consummatory behavior to changes in palatability is not altered in D2R-OE mice.50 We also directly measured hedonic reaction to sucrose in D2R-OE and control mice using the taste-reactivity paradigm described above (figure 2A).19,20 Using sucrose as the palatable food, we found that control and D2R-OE mice exhibited equivalent concentration-dependent increases in the number of positive hedonic reactions in response to sucrose (figure 2B).50
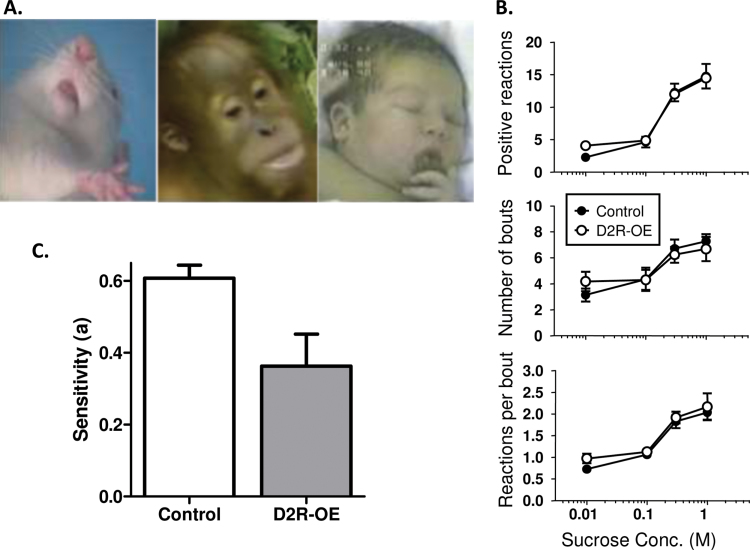
(A) Hedonic ‘liking’ reactions to pleasant tastes in rodents, nonhuman primates and human infants include rhythmic tongue protrusions (shown above) and lip licking. Such affective facial expressions provide an objective index of ‘liking’ to the hedonic impact of tastes.55 (B) Quantification of the hedonic response to increasing sucrose concentration in control (closed circles) and D2R-OE (open circles) mice is shown, including, Mean number of positive hedonic reactions (top), Mean number of bouts of drinking (middle) and Mean number of positive hedonic reactions per bout (bottom). Note the x-axis is on a log scale.50 (C) When tested on two concurrent schedules with different payoff rates, D2R-OE mice were significantly less sensitive to reward value than controls.50
Results from both the gustometer and taste-reactivity tests suggest that the hedonic reaction is intact in D2R-OE mice, as it is in patients with schizophrenia. Because D2R-OE mice appear to “like” the rewards as much as control mice, yet work less hard to obtain the rewards, we further explored their “wanting” or willingness to work. We employed an operant effort-related choice paradigm that indexes anticipatory (incentive) motivation.53 After determining that D2R-OE mice show as strong a preference as controls for evaporated milk vs home-cage chow when the two foods are freely available, mice were given a choice between pressing a lever to obtain the preferred evaporated milk or consuming freely available, less-preferred, home-cage chow. D2R-OE mice worked less for the preferred milk and consumed more of the freely available less-preferred chow, indicating that striatal overexpression of postsynaptic D2Rs can alter cost/benefit computations in a way that leads to a deficit in willingness to work for preferred rewards.50
Such a deficit in cost/benefit computations may arise from an impaired ability to represent the value of future outcomes. To assess whether D2R-OE mice and controls differed in their sensitivity to the value associated with different response options, we gave animals a choice of responding on two concurrently available response levers with a 5-fold variation in frequency at which each of the 2 available levers was rewarded. Control animals showed a good sensitivity to changes in payoff rates by adjusting their choices to fit the altered reward schedules. In contrast, D2R-OE mice were significantly less sensitive to variations in the distribution of rewards than controls (figure 2C), indicative of a deficit in either representing differences in future reward value or a deficit in being able to modulate behavior based on those values.50
In conclusion, overactivity of striatal D2Rs in mice, as found in patients, does not affect hedonic reaction to reward, but results in a deficit in incentive motivation evidenced by a decreased willingness to work. Furthermore, the behavioral studies suggest that decreased willingness to work in D2R-OE mice arises from deficits in the ability to represent or update the value of positive outcomes, similar to patients who show poor abilities in representing the positive value of future outcomes.38
It may be possible to document the deficit in ability to represent the value of future outcomes in the mouse model using electrophysiological recording methods. Midbrain dopamine neurons are known to play an important role in reward prediction error encoding; therefore, recording from these neurons or measuring dopamine release at their projection sites in limbic structures, under behavioral conditions presumed to induce anticipatory motivation, may determine the pathophysiological mechanism of this phenotype. Additionally, at the behavioral level the capacity to be guided by the current value of future rewards can be assessed in both patients and mouse models with several procedures such as postconditioning devaluation of rewards. Here the current value of a reward is lowered (say through satiation) and the sensitivity to this change in the value of future rewards should be reflected in a lowered willingness to perform the responses that previously produced this specific outcome.
A recent review of research in patients suggests that deficits in anticipatory motivation may result from impairments in cognition, including working memory.12 The D2R-OE mice also show deficits in cognitive functions including aspects of working memory,45 and the bidirectional relationship between cognition and motivation can be carefully explored in the mouse model.
Translations From Patients to Mice and Back to Novel Treatments
The striking parallel between the impairments in accurately representing the relative expected reward value of actions observed in patients and D2R-OE mice suggests that the D2R-OE mice can be used to identify and screen for new therapeutic treatment targets.
Gene expression analysis in the D2R-OE mouse has identified multiple potential pharmacological treatment targets, including the 5-HT2C receptor, whose expression levels are increased in D2R-OE mice. Our previous work has demonstrated that systemic application of a selective 5-HT2C receptor antagonist enhances willingness to work in mice.48 These findings suggest that 5-HT2C antagonism might also be a viable treatment target for patients. Additionally, we recently found that excitability of mediums spiny neurons (the GABAergic output neurons of the striatum) that overexpress D2Rs, is increased due to a downregulation of inwardly rectifying potassium (Kir) channels.54 The changes in excitability and Kir function are strongly associated with the motivational impairments because both can be reversed after switching off the excess expression of D2Rs in adulthood. We therefore hypothesize that the increase in spiny neuron excitability could be a mechanism underlying the motivation deficit. This hypothesis can directly be tested by reexpressing the downregulated Kir channels in D2R-OE mice. If decreasing excitability in D2R-OE mice indeed rescues their motivational deficit, targeting medium spiny neuron excitability could be a potential strategy to enhance motivation in patients with schizophrenia. Further explorations of the mechanism by which these, and other potential novel targets, may enhance incentive motivation are a first step to identifying novel treatment strategies for patients with schizophrenia.
The comparative consideration of the human and animal data we made here also demonstrates how a very careful analysis of motivational endophenotypes in patients and in rodent models can guide an analysis of the potential pathophysiological mechanisms of schizophrenia. This detailed analysis at the behavioral, molecular, electrophysiological, and neurochemical levels is a productive way to generate and test for much needed novel treatment targets.
Funding
National Institute of Mental Health; Silvio O. Conte Center for Schizophrenia Research: MH086404; (to E.H.S. and C.K.); R01MH093672 (to C.K. and P.B.).
Acknowledgments
The authors have declared that there are no conflicts of interest in relation to the subject of this study.
References
Articles from Schizophrenia Bulletin are provided here courtesy of Oxford University Press
Full text links
Read article at publisher's site: https://doi.org/10.1093/schbul/sbs114
Read article for free, from open access legal sources, via Unpaywall:
https://academic.oup.com/schizophreniabulletin/article-pdf/38/6/1111/5264536/sbs114.pdf
Citations & impact
Impact metrics
Citations of article over time
Article citations
Dampened motivation in schizophrenia: evidence from a novel effort-based decision-making task in social scenarios.
Eur Arch Psychiatry Clin Neurosci, 274(6):1447-1459, 28 Feb 2024
Cited by: 0 articles | PMID: 38413455
Vigor, Effort-Related Aspects of Motivation and Anhedonia.
Curr Top Behav Neurosci, 58:325-353, 01 Jan 2022
Cited by: 12 articles | PMID: 35505057
Review
Tracing Links Between Early Auditory Information Processing and Negative Symptoms in Schizophrenia: An ERP Study.
Front Psychiatry, 12:790745, 20 Dec 2021
Cited by: 9 articles | PMID: 34987433 | PMCID: PMC8721527
A Case for Thalamic Mechanisms of Schizophrenia: Perspective From Modeling 22q11.2 Deletion Syndrome.
Front Neural Circuits, 15:769969, 08 Dec 2021
Cited by: 13 articles | PMID: 34955759 | PMCID: PMC8693383
Review Free full text in Europe PMC
Investigating the Relationship between White Matter Connectivity and Motivational Circuits in Subjects with Deficit Schizophrenia: A Diffusion Tensor Imaging (DTI) Study.
J Clin Med, 11(1):61, 23 Dec 2021
Cited by: 8 articles | PMID: 35011803 | PMCID: PMC8745695
Go to all (38) article citations
Data
Similar Articles
To arrive at the top five similar articles we use a word-weighted algorithm to compare words from the Title and Abstract of each citation.
Dissociation of hedonic reaction to reward and incentive motivation in an animal model of the negative symptoms of schizophrenia.
Neuropsychopharmacology, 37(7):1699-1707, 14 Mar 2012
Cited by: 62 articles | PMID: 22414818 | PMCID: PMC3358738
Pharmacologic rescue of motivational deficit in an animal model of the negative symptoms of schizophrenia.
Biol Psychiatry, 69(10):928-935, 16 Mar 2011
Cited by: 56 articles | PMID: 21414604 | PMCID: PMC3170714
Insights About Striatal Circuit Function and Schizophrenia From a Mouse Model of Dopamine D2 Receptor Upregulation.
Biol Psychiatry, 81(1):21-30, 14 Jul 2016
Cited by: 25 articles | PMID: 27720388 | PMCID: PMC5121031
Review Free full text in Europe PMC
Mechanisms Underlying Motivational Deficits in Psychopathology: Similarities and Differences in Depression and Schizophrenia.
Curr Top Behav Neurosci, 27:411-449, 01 Jan 2016
Cited by: 102 articles | PMID: 26026289
Review
Funding
Funders who supported this work.
NIMH NIH HHS (4)
Grant ID: R01 MH080066
Grant ID: MH086404
Grant ID: R01 MH093672
Grant ID: R01MH093672