Abstract
Free full text

Expression of MHC Class I on breast cancer cells correlates inversely with HER2 expression
Abstract
HER2 is a promising target for immunotherapeutic interventions with T cell-based approaches since it is amplified and overexpressed in 20–30% of breast cancers. However, several previous studies including ours showed that HER2-overexpressing tumors may escape cytotoxic T lymphocyte-mediated lysis by downregulating MHC Class I and components of the antigen-processing machinery. The aims of the present study were to analyze the relationship between HER2 and MHC Class I expression and to elucidate the mechanisms underlying MHC Class I downregulation in breast cancer. We explored expression of HER2, MHC Class I, PTEN, Ki67, estrogen and progesterone expression in 70 breast cancer patients by immunohistochemistry (IHC) and analyzed their correlation. We also explored the components of the signal transduction pathway that are involved in the regulation of MHC Class I expression using small-interfering RNAs targeting HER2 as well as an inhibitor of HER2 signaling. HER2 expression in breast cancers correlated inversely with MHC Class I expression analyzed by IHC. HER2 depletion by small-interfering RNAs resulted in MHC Class I upregulation. Moreover, MHC Class I expression on breast cancer cell lines was upregulated by PD98059, an inhibitor of mitogen-associated protein kinases, in a dose-dependent manner. Thus, agents that target the MAPK signaling pathway may increase MHC Class I expression in breast cancer cells.
Introduction
ERBB2 (best known as HER2 or neu) is a protooncogene encoding a 185-kDa transmembrane glycoprotein member of the epidermal growth factor receptor (EGFR) family of tyrosine kinases.1 HER2 is amplified and overexpressed in a variety of human tumors including 20–30% of ovarian and breast cancers, renal cell carcinomas, esophageal squamous cell carcinomas, gastric and colorectal cancers.2-5 Diverse experimental models and clinical trials indicate that HER2 is a promising target for immunotherapeutic interventions with both antibodies and T cell-based approaches.6,7
Previous studies have demonstrated that cytotoxic T lymphocytes (CTLs) specific for HER2 epitopes derived from breast cancer patients can recognize and kill HER2-expressing tumor cells.8-11 Nevertheless, HER2 expression is known to facilitate the escape of tumor cells from immune surveillance. For instance, an inverse correlation between HER2 and MHC class I expression levels has been observed in murine tumor models.12-14 Along similar lines, an inverse correlation between HER2 and TAP levels, resulting in reduced sensitivity to lysis by CTLs, has been highlighted in human tumors.12 Thus, HER2-overexpressing tumors may escape from CTL-mediated lysis by downregulating MHC class I and components of the antigen-processing machinery (APM). Our recent studies have also demonstrated that the overexpression of functional HER2 severely impairs MHC class I expression and CTL-mediated recognition of several HLA-A2 restricted tumor antigens, including HER2 epitopes.15
The overexpression of HER2 activates several signaling pathways including the PI3K/AKT, RAS/mitogen-activated protein kinase (MAPK), and STAT3 cascades.16 Compelling evidence on the link between MHC class I and HER2 expression is derived from the observation that the small-interfering RNA (siRNA)-mediated silencing of HER2 in tumor cell lines reportedly increases MHC class I surface expression.17 However, at present limited information is available on the relationship between HER2-associated signal transduction pathways and MHC class I expression on tumor cells.
Phosphatase and tensin homolog deleted on chromosome 10 (PTEN) is a lipid phosphatase that functionally antagonized PI3K/AKT signaling and is frequently mutated or inactivated in human tumors, including in 30% of breast cancer cases.18,19 Reduced or absent PTEN expression results in the hyperactivation PI3K/AKT signaling and is related to poor prognosis as well as resistance to chemotherapy and hormone therapy in breast cancer.18-21 However, it is unclear whether there is a direct correlation between PTEN expression and MHC class I levels in breast cancer.
The aim of the present study was to analyze the relationship between HER2 expression and MHC class I levels in breast cancer tissues. We have also explored the components of the signal transduction pathway that are involved in the regulation of MHC class I expression using siRNA-mediated HER2 silencing and chemical inhibitors of HER2-related signaling pathways.
Results
Expression of HER2, MHC class I, PTEN, Ki-67, estrogen receptor (ER) and progesterone receptor (PgR) on breast cancer tissues analyzed by immunohistochemistry
Seventy breast cancer patients were analyzed by immunohistochemistry for HER2, MHC class I, PTEN, Ki-67, ER and PgR expression. Representative immunohistochemical stainings on the serial section are shown in Figure 1A–C and the correlation between HER2 expression and each parameter are summarized in Table 1. Of note, while the majority of HER2 negative (HercepTest 0) cases showed strong MHC class I staining, most of HercepTest 3+ cases showed weak MHC Class I staining (Fig. 2). Collectively, these results indicate that there is an inverse correlation between HER2 and MHC class I expression levels (Table 2, p = 0.031, χ2 analysis). Furthermore, we observed an inverse correlations between HER2 expression and both ER and PgR levels (p = 0.013 and p = 0.021, respectively, χ2 analysis). PTEN and Ki67 levels neither correlated with MHC class I nor with HER2 expression. These observations suggest that HER2 expression in breast cancer inversely correlates with MHC Class I, ER and PgR expression, but not with PTEN and Ki67 levels.
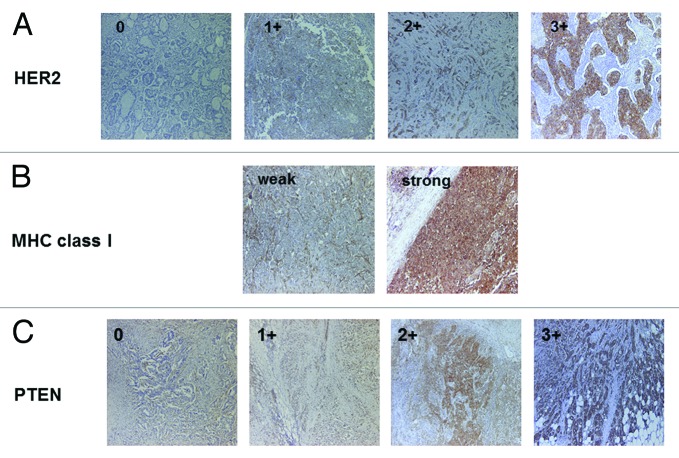
Figure 1. Representative immunostaining of HER2, MHC class I and PTEN. (A) HER2 staining (0, 1+, 2+, 3+). (B) MHC class I staining (weak and strong). (C) PTEN staining (0, 1+, 2+, 3+). Original magnification: 200x.
Age (years old) | |
---|---|
Mean | 57 |
Range | 31–86 |
Primary tumor#1 | |
---|---|
pTis | 1 |
pT1 | 40 |
pT2 | 25 |
pT3 | 2 |
pT4 | 2 |
Lymph node metastasis#1 | |
---|---|
pNx | 2 |
pN negative | 37 |
pN positive | 31 |
Estrogen receptor | |
---|---|
Negative | 28 |
Positive | 42 |
Progesterone receptor | |
---|---|
Negative | 27 |
Positive | 42 |
Histological Classification | |
---|---|
1. Ductal carcinoma in situ | 1 |
2. Invasive carcinoma | |
a. Invasive ductal carcinoma | |
Papillotubular | 17 |
Solid tubular carcinoma | 7 |
Scirrhous carcinoma | 33 |
b. Special types | |
Mucinous carcinoma | 6 |
Apocrine carcinoma | 1 |
Invasive micropapillary carcinoma | 4 |
3. Unclassified | 1 |
Nuclear grade #1 | |
---|---|
1 | 29 |
2 | 19 |
3 | 20 |
#1 The grade of tumor and stages were defined according to the UICC (TMN) classification.
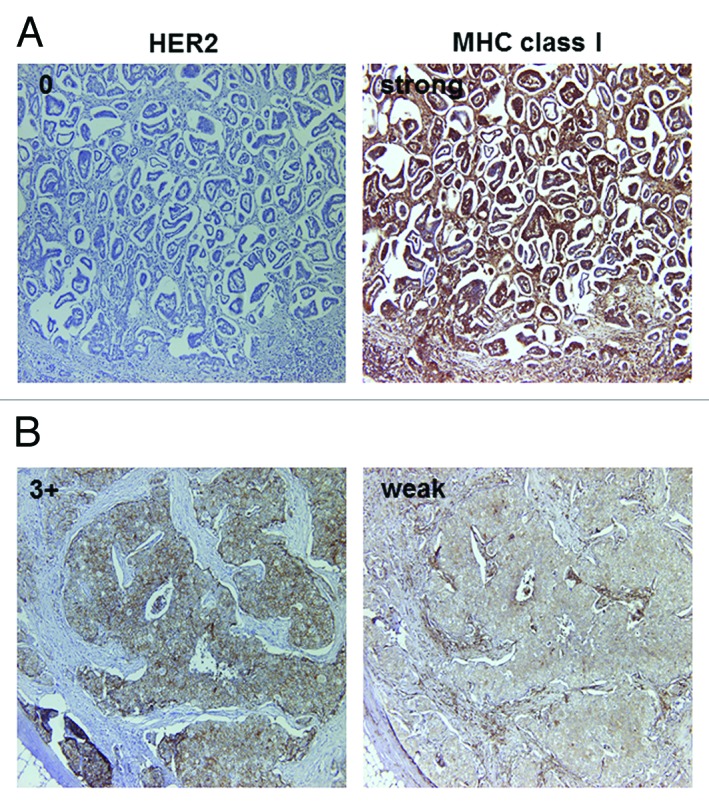
Figure 2. Representative MHC class I immunostaining of HercepTest 0 (A) and 3+ (B) cases. Serial sections were stained with antibodies specific for HER2 and MHC class I. (A) MHC class I staining was strong in HercepTest 0 case. (B) MHC class I staining was weak in HercepTest 3+ case. Original magnification: 200x.
| MHC Class l | PTEN | Ki67 | ER | PgR | |||||||
---|---|---|---|---|---|---|---|---|---|---|---|---|
Weak | Strong | 0 | 1+ | 2+ | 3+ | Low | High | - | + | - | + | |
HercpTest 0, 1+ | 30 | 18 | 11 | 19 | 13 | 5 | 37 | 11 | 14 | 34 | 14 | 34 |
Hercp Test 2+, 3+ | 20 | 2 | 3 | 12 | 7 | 0 | 16 | 6 | 16 | 6 | 13 | 3 |
p = 0.031 | no correlation | no correlation | p = 0.013 | p = 0.021 |
HER2 signaling affects MHC class I expression on human breast cancer cells
We used specific siRNAs to silence HER2 expression and to explore the relationship between MHC class I and HER2. MDA-MB-231 cells was transfected with a HER2-targeting or with a control non-silencing siRNA and were then examined by immunoblotting and cytofluorometry. HER2 expression in MDA-MB-231 cells transfected with the anti-HER2 siRNA was downregulated as compared with their control counterparts (Fig. 3A and B). Moreover, HER2-silenced cells exhibited increased MHC class I expression in comparison to cells receiving a control siRNA, as determined with two distinct antibodies specific for MHC Class I (Fig. 3C). Similar results were obtained in SK-BR-3 and BT549 breast cancer cells (Table 3). Altogether, these observations indicate that HER2 downregulates MHC Class I expression on breast cancer cells.
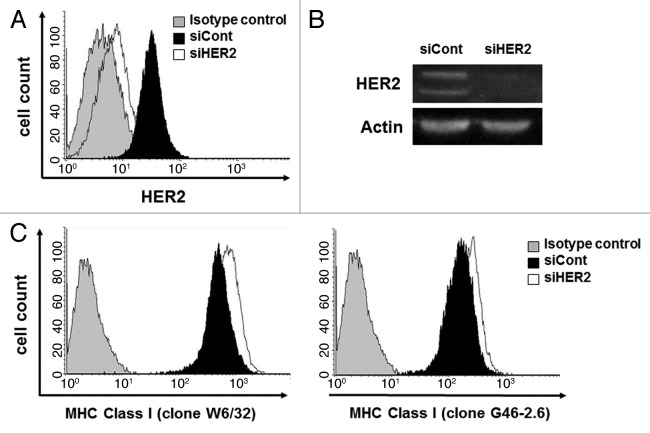
Figure 3. HER2 downregulation by siRNA upregulates MHC class I expression on breast cancer cells. MDA-MB-231 cells were transfected with a non-targeting siRNA (siCont) or with a siRNA for the downregulation of HER2 (siHER2). HER2 expression was downregulated in siHER2 transfectants, as assessed by flow cytometry (A) and immunoblotting (B). (C) MHC class I expression was increased in cells transfected with siHER2, as assessed by cytofluometry upon staining with two different antibodies specific for MHC class I molecules, clone W6/32 and clone G46–2.6. Representative results from n = 5 independent experiments are shown.
Cell line | Incubator time (hours) | HER2 (MFI) | MHC Class I (MFI) clone W6/32 | MHC Class I (MFI) clone G42–2.6 | |||
---|---|---|---|---|---|---|---|
siCont | siHER2 | siCont | siHER2 | siCont | siHER2 | ||
BT549 | 48 | 23.4 | 10.3 | 215.8 | 235.1 | 73.5 | 78.7 |
72 | 24.9 | 7.9 | 202.9 | 232.9 | 68.8 | 71.6 | |
SK-BR-3 | 48 | 527.7 | 303.7 | 25.8 | 29.3 | 13.9 | 15.5 |
72 | ND | ND | ND | ND | ND | ND |
Abbreviations: MFI, mean florescence intensity; siCont, control siRNA; siHER2, HER2 siRNA; ND, not determined.
The RAS/MAPK signaling pathway regulates MHC Class I expression on human breast cancer cells
In our cohort of breast cancer tissues, PTEN expression levels did not correlate to MHC Class I (data not shown) or HER2 expression (Table 2). Therefore, we focused on the RAS/MAPK pathway in order to evaluate the relationship between HER2-activated signaling pathway and MHC Class I expression. We used the MAPK inhibitor PD98059 to inhibit extracellular signal-regulated kinase (ERK) phosphorylation. ERK phosphorylation in MDA-MB-231, BT549, and SK-BR-3 cells was inhibited by PD98059 in a dose-dependent manner (Fig. 4). Furthermore, MDA-MB-231, BT549, and SK-BR-3 cells exposed to PD98059 upregulated MHC Class I in a dose-dependent manner, as assessed by flow cytometry analysis with two different antibodies specific for MHC Class I molecules (Fig. 4). These results indicate that the RAS/MAPK pathway negatively regulate MHC Class I expression and that inhibition of this pathway can result in the upregulation of MHC Class I expression on human breast cancer cells.
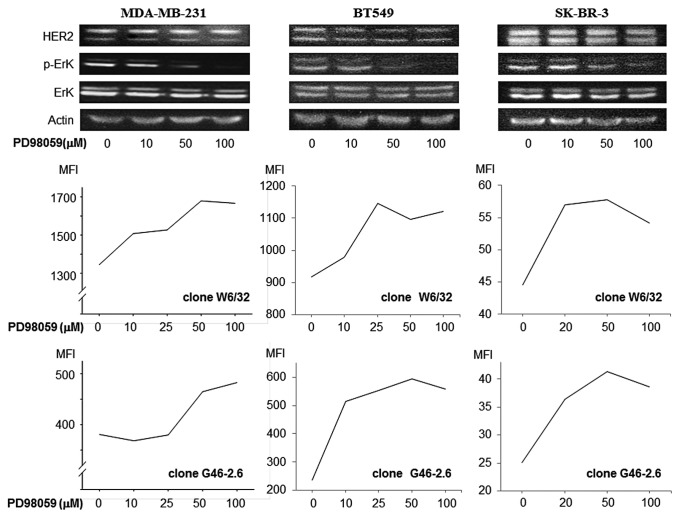
Figure 4. MAPK inhibiton upregulates MHC class I expression on breast cancer cells. MDA-MB-231, BT549 and SK-BR-3 were treated with the ERK inhibitor PD98059. Phospho-p44MAPK and phospho-p42MAPK levels were reduced in a dose-dependent manner, as assessed by immunoblotting. Conversely, MHC class I expression was increased upon exposure to PD98059 in a dose-dependent manner, as assessed by cytofluometry upon staining with two different antibodies specific for MHC class I molecules, clone W6/32 and clone G46–2.6. Non-viable (7-AAD+) cells were excluded from the analysis. Representative results from n = 4 independent experiments are shown. MFI, mean fluorescence intensity.
Discussion
Breast cancer is the most common malignancy in women. Despite aggressive treatment with surgery, chemotherapy and hormonal therapy as well as the advent of monoclonal antibodies, a significant proportion of breast cancer patients develop recurrent disease. Therefore, novel strategies are needed to improve the prognosis of breast cancer patients. One of these innovative approaches for treating breast cancer is represented by active immunotherapy, involving vaccination with tumor associated antigens. Results from a recent clinical trial with a vaccination based on the HER2-E75 peptide (HER2369–377, KIFGSLAFL) indicate that this procedure is safe, effective in eliciting an immune response, and may be clinically efficient with decreased recurrence rates among HER2-positive breast cancer patients.9-11,22
Recently, Bebavides et al. reported that patients expressing low levels of HER2 (IHC: 0 or 1+) respond better to the HER2-E75 vaccine than their HER2-overexpressing counterparts (IHC: 2+ or 3+), due to elements of immunologic tolerance developing in the latter group.10 In previous studies by Rosenberg and Conrad, human HER2-overexpressing tumors were not recognized by HER2369-specific CTLs,23,24 indicating that HER2 expression is associated with an immune escape phenotype. It is established that the downregulation of MHC Class I and components of the APM in tumor cells is one of the major reasons underlying the escape of cancer from CTL activity.2,25,26 We therefore speculated that the downregulation of MHC Class I could be one of causes of immunologic tolerance in HER2-overexpressing group patients, resulting in poor recognition of cancer cells by antigen specific CTLs.
Previous reports from our laboratory as well as from other investigators have demonstrated that HER2 signaling reduce the MHC Class I expression on cancer cells and that HLA-A2-restricted, tumor antigen-specific CTLs cannot recognize efficiently HER2 overexpressing cancer cells.12,15,17,27 In particular, it has been shown that the upregulation of HER2 results in the decreased sensitivity to HER2-specific CTLs15 and that the downregulation of HER2 by siRNA leads to restored tumor-specific CTL responses.27 In the present study, we showed that MHC Class I expression is upregulated upon the siRNA-mediated silencing of HER2 in 3 different breast cancer cell lines (Fig. 3C and Table 3). Our results confirm the hypothesis that HER2 signaling reduces the MHC Class I expression on breast cancer cells and that HER2 expression is associated with an immune escape phenotype. Thus, approaches for increasing MHC Class I expression in HER2-overexpressing breast cancer may boost clinical responses following vaccination therapies with MHC Class I restricted peptides. Concurrently, patients with low levels of HER2 (hence exhibiting higher levels of MHC Class I) may be more suitable for peptide vaccination than HER2-overexpressing patients, as efficient vaccination requires moderate HER2 expression for CTL induction and low levels of HER2 (even from an intracellular localization) suffice for CTL recognition.10 We suggest that the patients with reduced expression of HER2, which represents > 50% of breast cancer patients, will benefit from the HER2-E75 vaccine. Based on our findings, we also argue that vaccination approaches based on HER2-specific CTL epitopes alone may be less efficient than vaccines that can activate an integrated immune response involving CD4+ T cells and antibodies are induced.8,28
Although HER2 is associated with several downstream signaling pathways including the RAS/MAPK and the PI3K/AKT cascades,16,29 the signaling pathway activated by HER2 that may affect the expression of MHC Class I on breast cancer cells so far remained elusive. Although it has been shown that PTEN antagonizes PI3K function and negatively regulates AKT signaling pathway,18,19 we failed to observe a correlation between MHC Class I and PTEN expression in the present study (data not shown). However, inhibition of the RAS/MAPK pathway resulted in MHC Class I upregulation in 3 different breast cancer cell lines (Fig. 4). These observations suggest that the RAS/MAPK pathway may regulate MHC Class I expression on breast cancer cells and that HER2-overexpression may be linked to the downregulation of MHC Class I through this signaling cascade.
In the present study, we identified an inverse correlation between HER2 expression and MHC Class I expression in patients with breast cancer and showed that HER2-overexpression results in MHC Class I downregulation by a mechanism that involves the RAS/MAPK pathway. These findings suggest that RAS/MAPK pathway inhibitors may improve MHC Class I expression in breast cancer cells.
Materials and Methods
Patients and samples
Seventy consecutive patients with primary breast cancer who were histologically diagnosed and treated surgically in the First Department of Surgery, University of Yamanashi Hospital, were enrolled in the present study. None of the patients had received radiotherapy and/or chemotherapy before surgery and the characteristics of the patients are shown in Table 1. This study was approved by the ethical committee of University of Yamanashi. Patients were accrued with informed consent and research was conducted in keeping with the Helsinki declaration. Archived, formalin-fixed, paraffin-embedded tissue blocks of specimens obtained during resective surgery was used to obtain 4-μm thick serial sections for immunohistochemical analysis of HER2, MHC Class I, PTEN, Ki67, ER and PgR staining.
Reagents and antibodies
Penicillin/streptomycin and dimethylsulfoxide (DMSO) were purchased from Sigma-Aldrich. The MAPK inhibitor PD98059 was purchased from Cell Signaling Technology (#9900). 7-AAD, FITC- and PE-conjugated anti-HER2/neu (clone Neu 24.7) and HLA-ABC (clone G46–2.6) monoclonal antibodies, as well as the isotype control IgG1 κ (clone MOPC-21) were purchased from Becton Dickinson Biosciences. PE-conjugated HLA-ABC (clone W6/32) monoclonal antibody and the isotype control PE-conjugated IgG2a κ were purchased from eBioscience. Primary antibodies against HER2/ErbB2, phosphorylated HER2/ErbB2 (Tyr1221/1222), p44/42 MAPK, phospho-p44/42 MAPK, β-actin and secondary antibody, HRP-linked anti-rabbit IgG, for Western Blot were purchased from Cell Signaling Technology.
Immunohistochemistry (IHC) analysis
Deparaffinized and rehydrated sections of tumor tissue were incubated with the epitope retrieval solution (Dako) for 40 min at 95–99°C in a water bath or for 20 min at 121°C in an autoclave. For HER2 staining, we used the HercepTestTM (Dako) according to the manufacturer’s recommendations. Briefly, the sections were treated with 3% hydrogen peroxide for 5 min and incubated with a rabbit polyclonal antibody to human HER2, or a negative control antibody at room temperature for 30 min. Tissue sections were subsequently incubated with the visualization reagents for 30 min at room temperature. Diaminobenzidine was utilized as the chromogen for 10 min, and then the sections were counterstained with hematoxylin. Positive and negative control slides were provided by the HercepTestTM kit manufacturer and sections were observed and analyzed independently without prior knowledge of clinical and pathological data by two investigators (Y.M. and K.M.) according to the manufacturer’s criteria. For MHC Class I staining, tumor sections were treated with Chemmate Peroxidase Blocking Solution (Dako). Sections were incubated with the antibody EMR8–5 (Cosmo Bio Co.) or the isotype control (Dako) at 4°C overnight. They were then incubated with streptavidin-biotin complex (Nichirei) for 30 min followed by 3, 3′-diaminobenzidine (Dako) for 5 min, and counterstained with hematoxylin. The staining intensity was evaluated by following criteria: strong positive (strong), dark brown staining in more than 70% of tumor cells completely obscuring cytoplasm; weak positive (weak), any lesser degree of brown staining appreciable or no appreciable staining in tumor cells. IHC analysis was performed independently by two observers (Y.M. and T.M.) without prior knowledge of clinical and pathological data. For PTEN staining, the monoclonal mouse anti-PTEN clone 6H2.1 antibody (Dako) or the isotype control monoclonal antibody (Dako) was used to treat sections at 4°C overnight followed by the LSAB+ kit (Dako). The PTEN expression level was scored semi-quantitatively based on staining intensity and distribution using the immunoreactive score (IRS);30 IRS = SI (staining intensity) x PP (percentage of positive cells). Staining intensity was assigned as 0, negative; 1, weak; 2, moderate; 3, strong. Percentage of positive cells is defined as 0, < 1%; 1, 1–10%; 2, 11–50%; 3, 51–80%; and 4, > 80% positive cell. In all, 5 visual fields from different areas of each tumor were used for IRS evaluation. Based on the IRS score, staining intensity was graded – (IRS 0–3), 1+ (IRS 4–6), 2+ (IRS 7–9) or 3+ (IRS 10–12). For Ki-67 staining, the deparaffinized and rehydrated sections were incubated with the epitope retrieval solution (DaKo) for 10 min at 121°C in an autoclave. The sections were blocked with 3% hydrogen peroxide for 10 min and were incubated with the primary antibody, a mouse monoclonal antibody to human Ki-67 (Dako), or the primary negative control antibody at room temperature for 60 min followed by treatment with the EnVisionTM/HRP (Dako) and counterstained with hematoxylin. The Ki-67 staining intensity was evaluated according to previous report and was assigned as high and low.31 Percentage of tumor cell nuclei with positive immunostaining was defined as high, > 15%; low, 15%. IHC analysis was performed by two observers (M.I. and A.I.) without prior knowledge of clinical and pathological data.
Cell lines
MDA-MB-231 (HTB-26), BT549 and SK-BR-3 breast cancer cell lines were purchased from the American Type Culture Collection and maintained in RPMI 1640 (Invitrogen) with 5% FCS (Invitrogen), 50 U/mL penicillin and 2 mM L-glutamine.
Small interfering RNA (siRNA) transfection
Tumor cell lines were grown at approximately 60% confluence in 6-well plates. Cells were transfected with 100pmol siRNA (Thermo Fisher Scientific, ERBB2 ON-TARGETplus SMARTpool siRNA, L-003126–00–0005, or ON-TARGETplus siCONTROL non-targeting siRNA, D-001810–01–05) in Opti-MEN® I Reduced Serum Medium (Invitrogen) including 100 pmol, with 5 μl of LipofectamineTM 2000 (Invitrogen) according to manufacturer’s protocol. After 48 h, cells were analyzed by immunoblotting for HER2 and by flow cytometry for HER2 and MHC Class I expression. ERBB2 and non-silencing control siRNA transfectants were always cultured in parallel for the same period of time. Three identical but independent transfections were performed.
Cytofluorometry and immunolbotting
Cell staining was performed according to standard flow cytometric staining protocol. Samples were analyzed using a four-color FACS machine, FACSCalibur (Becton Dickinson). For immunoblotting, cell pellets were solubilized and sonicated, and then boiled for 10 min. The protein concentration of sample was measured and equal amounts (10μg) separated by SDS-PAGE followed by transfer to a polyvinylidene fluoride (PVDF) microporous membrane (MILLIPORE). Membrane was treated with primary antibodies, followed by HRP-linked goat anti-rabbit antibody (Cell Signaling Technology). The specific bands were visualized by ECL Plus (Amersham Pharmacia Biotech AB) and digitally captured by a LAS4000 Lumino Image Analyzer (Fuji Photo Film).
Data analysis
The χ2 test was used to examine the differences in HercepTest score and MHC Class I expression, PTEN expression, Ki-67 expression, ER expression, PgR expression. Statistical analyses were performed using Statview 5.0 for Windows software and statistically significant difference was considered as p values < 0.05.
Disclosure of Potential Conflicts of Interest
No potential conflicts of interest were disclosed.
Glossary
Abbreviations:
APM | antigen-processing machinery |
CTL | cytotoxic T lymphocyte |
ER | estrogen receptor |
ERK | extracellular signal-regulated kinase |
IHC | immunohistochemistry |
MAPK | mitogen-activated protein kinase |
PI3K | phosphoinosidite-3-kinase |
PgR | progesterone receptor |
PTEN | phosphatase and tensin homolog deleted on chromosome 10 |
siRNA | small interfering RNA |
Footnotes
Previously published online: www.landesbioscience.com/journals/oncoimmunology/article/21056
References
Articles from Oncoimmunology are provided here courtesy of Taylor & Francis
Full text links
Read article at publisher's site: https://doi.org/10.4161/onci.21056
Read article for free, from open access legal sources, via Unpaywall:
https://www.tandfonline.com/doi/pdf/10.4161/onci.21056?needAccess=true
Citations & impact
Impact metrics
Citations of article over time
Article citations
A Comprehensive Review of HER2 in Cancer Biology and Therapeutics.
Genes (Basel), 15(7):903, 11 Jul 2024
Cited by: 3 articles | PMID: 39062682 | PMCID: PMC11275319
Review Free full text in Europe PMC
Modification of the tumor microenvironment enhances immunity with plasmid gene therapy.
Cancer Gene Ther, 31(4):641-648, 09 Feb 2024
Cited by: 0 articles | PMID: 38337037
A novel prognostic model of breast cancer based on cuproptosis-related lncRNAs.
Discov Oncol, 15(1):35, 14 Feb 2024
Cited by: 1 article | PMID: 38353835 | PMCID: PMC10866837
HER2 Alterations in Non-Small Cell Lung Cancer: Biologico-Clinical Consequences and Interest in Therapeutic Strategies.
Life (Basel), 14(1):64, 29 Dec 2023
Cited by: 0 articles | PMID: 38255679 | PMCID: PMC10820545
Review Free full text in Europe PMC
Unveiling the Immunogenicity of Ovarian Tumors as the Crucial Catalyst for Therapeutic Success.
Cancers (Basel), 15(23):5694, 02 Dec 2023
Cited by: 1 article | PMID: 38067396 | PMCID: PMC10705691
Review Free full text in Europe PMC
Go to all (51) article citations
Similar Articles
To arrive at the top five similar articles we use a word-weighted algorithm to compare words from the Title and Abstract of each citation.
Inverse correlation of HER2 with MHC class I expression on oesophageal squamous cell carcinoma.
Br J Cancer, 103(4):552-559, 13 Jul 2010
Cited by: 29 articles | PMID: 20628381 | PMCID: PMC2939777
HER2/HER3 signaling regulates NK cell-mediated cytotoxicity via MHC class I chain-related molecule A and B expression in human breast cancer cell lines.
J Immunol, 188(5):2136-2145, 01 Feb 2012
Cited by: 38 articles | PMID: 22301547
MHC-class-I expression in human breast cancer correlates with nuclear localization of the 90 kDa heat-shock-protein.
Anticancer Res, 19(6b):5293-5297, 01 Nov 1999
Cited by: 4 articles | PMID: 10697551
T cell recognition of HLA-A2 restricted tumor antigens is impaired by the oncogene HER2.
Int J Cancer, 128(2):390-401, 13 Oct 2010
Cited by: 39 articles | PMID: 20715101